- 1W.A. Franke College of Forestry & Conservation, University of Montana, Missoula, MT, United States
- 2Department of Forest Ecology and Management, Swedish University of Agricultural Sciences, Umeå, Sweden
- 3College of Agricultural Sciences, Pennsylvania State University, University Park, PA, United States
Pyrogenic carbon (PyC) is a chemically stable form of carbon (C) generated during fire events and is one of the few legacies of fire recorded in soil; however, the significance of this material as a form of C storage in forest ecosystems has received only limited scientific attention, and currently relatively little is known regarding the quantity of PyC generated during forest restoration efforts that include prescribed (Rx) fire. Quantifying the rate of PyC production during fire events is essential to estimating the potential for ecosystem C storage in forest systems where wildfire is a common natural disturbance and Rx fire is used as a fuel management practice. In this paper, we report on the average mass of PyC formation during wildfire and Rx fire events in forest ecosystems through synthesizing data across 12 published studies; and we report empirical data on the mass of PyC generated in a replicated Rx fire study in a ponderosa pine (Pinus ponderosa) forest ecosystem of the Rocky Mountain West. Our data synthesis showed that PyC (consisting of PyC from downed wood, O horizon, and mineral soil) was, on average, produced at a rate of 5.2 ± 2.5% of biomass exposed to fire in forest ecosystems, and a single wildfire or Rx fire event generated about 2.2 ± 1.7 Mg C ha−1 in the form of PyC. In our empirical study, we collected O horizon and surface mineral soil (0–10 cm) samples from the Fire and Fire Surrogates study plots in western Montana (treatments: control, thin, burn, thin&burn) that was burned in 2002 and analyzed for PyC content using a wet digestion method. The two Rx fire treatments had significantly higher PyC contents in the O horizon compared to the control. In the thin&burn treatment, PyC was formed at a rate of about 12% of total biomass consumed yielding ~2.24 Mg PyC C ha−1 in the O horizon. Mineral soil PyC contents were not affected by Rx fire. Wildfire and Rx fires generate a substantial amount of PyC which initially resides in the O horizon and serves as a stable form of ecosystem C.
Introduction
Charcoal and pyrogenic carbon (PyC) are general terms given to organic materials that have been subject to partial combustion during biomass burning events (Forbes et al., 2006; Zimmerman and Mitra, 2017). There is increasing interest in the role that PyC plays in long term carbon (C) storage in terrestrial and aquatic ecosystems, yet there is only limited understanding of the rate at which PyC accumulates in forest ecosystems during natural and prescribed (Rx) fire events. Of the PyC formed during forest fire events, much remains onsite as stored C (Lynch et al., 2004), eventually lost to depositional areas due via surface erosion (Abney et al., 2019) and if incorporated into mineral surface soils, the PyC may remain in surface soils an indefinite length of time (Abiven and Santín, 2019). Given this longevity in soil ecosystems, PyC has been identified as being an important C storage medium (Lehmann et al., 2006; DeLuca and Aplet, 2008; Santín et al., 2015) and receives a great deal of attention across a variety of research fields (Carcaillet, 2001; Gavin, 2004; Gavin et al., 2006; Power et al., 2006).
The role of PyC in the soil ecosystem extends beyond its capacity to serve as stored C, PyC can directly and indirectly influence soil microbial processes (Thies et al., 2015), increase rates of organic matter decomposition (Wardle et al., 2008; Pluchon et al., 2015) alter soil nutrient availability (Lehmann et al., 2011; Gul and Whalen, 2016; Gao and DeLuca, 2019; Gao et al., 2019), modify soil nutrient cycling (DeLuca and Sala, 2006; DeLuca et al., 2006, 2015; Gao et al., 2017; Gao and DeLuca, 2018), and adsorb organic compounds in plant root exudates, litter decomposition products, and microbial byproducts (Keech et al., 2005; Gundale and DeLuca, 2006; Quilliam et al., 2013). These facets of PyC have led to a significant increase in the number of studies devoted to the role of naturally formed PyC in terrestrial ecosystems (Pingree and DeLuca, 2017).
Despite the fact that PyC has widely been recognized as a highly chemically stable form of ecosystem C that is formed in all biomass burning events and is of great importance to global C cycle, to date there has been limited effort to quantify forest fire generated PyC and in particular that affiliated with Rx fire events in forest ecosystems (Alexis et al., 2012; Pingree et al., 2012; Wiechmann et al., 2015). In addition to wildfire events that commonly occur as a result of local climatic conditions at the seasonally dry forest ecosystems of the Rocky Mountain West (Agee, 1996), Rx fires are applied widely as a forest management practice throughout the region to reduce surface fuel loading and restore the historical fire regime (Ryan et al., 2013). Quantifying the rate of PyC production during fire events in this region is essential to estimating the potential of ecosystem C storage in other forest systems with similar climatic conditions and fire management practices. The objectives of the work reported here were to: (1) Evaluate the PyC generation rate and the percent fuel conversion to PyC during wildfire or Rx fire events of forest ecosystems through synthesizing existing literature; (2) Determine the amount of PyC present in soil following forest restoration treatments that include Rx fire in an empirical study in western Montana, and evaluate the relationship between PyC generation and fuel consumption using data collected on the same study sites. By combining a synthesis and an empirical study that both focus on the evaluation of PyC generation in forest fire events, our goal was to advance the understanding of total ecosystem C budgets and the C storage potential of post-fire forest systems. We believe the findings from this research could help inform more rational strategies toward assessment of the influence of fire and fuel treatments on ecosystem C storage.
Materials and Methods
Data Synthesis
A search for peer-reviewed papers (published between January 2000 and December 2018) on PyC generation rate was conducted using the “Web of Science” database with a variety of keywords (“charcoal,” “PyC,” “pyrogenic carbon,” “black carbon”; “generation,” “production,” “formation”; and “forest fire,” “fire,” “wildfire,” “prescribed fire,” “Rx fire,” “fuel reduction,” “forest management practices”). The title and abstract were first evaluated to determine if the publication was relevant to our inquiry and contained original data. Papers that met these criteria were then examined in detail for information and data “extraction” prior to analysis. We excluded studies that report PyC production data at the continental to global scale (either existing synthesis data or modeling data). We also excluded studies associated with landscape scale grassland or peatland fires, and we specifically focused on PyC generated in forest systems at a local to sub-regional scale. If a study was conducted at an ecotone transitioning from grassland to woodland, we only included PyC data collected at sites with dominant species being woody plants to match the common scenarios of Rx fire in most fuel treatment practices. A total of 12 published papers were identified, which presented PyC data for a total of 22 individual fire events, and a total of 31 sampling sites (N = 31) were selected and finalized for our further analysis. It is important to note that for studies that report PyC data by comparing pre- and post-fire treatments, we did not separate data by time-since-fire given the small sample size in this synthesis; similarly, studies that report PyC formation by using data collected at paired burned and unburned sites were also included in our synthesis regardless of time-since-fire. For studies that only provided a PyC production range, both minimum and maximum numbers were included in our analysis, but treated as two observations for one single fire event site.
All PyC production data were converted to the same unit (Mg PyC C ha−1) according to the C content of PyC reported in individual studies. We also recorded the “percent fuel conversion to PyC (PyC mass: fuel mass)” data when it was provided by the study. Mean value and 1× standard deviation (SD) were calculated for both PyC production data (Mg C ha−1) and fuel conversion rate (%) data across all observations associated with wildfire or Rx fire events of the forest ecosystems. Given that the sample size in this synthesis is rather small, we limited the focus of our examination to only these two response variables (i.e., PyC production, fuel conversion%) and eliminated any analysis examining their relationships with potential covariates (e.g., fire characteristics, site and soil conditions, time-since-fire, etc.). However, detailed descriptive statistics for all observations across studies are provided in Supplementary Information (Figure S1, Table S1). Data homogeneity and normality were tested before conducting any analysis. We used ANOVA and Tukey's post-hoc test to identify differences between PyC generation rate under wildfires and prescribed fires at a significant level of P = 0.05. All statistical analyses were performed in R (R Core Team, 2016).
Empirical Study
The empirical study was conducted using soil samples collected from the Montana installment of the Fire and Fire Surrogates (FFS) national study network (Table 1). The FFS study is a multiyear interdisciplinary study investigating the effectiveness of thinning and Rx fire treatments aimed at reducing wildfire hazard and restoring more natural stand structure which has been described in detail elsewhere (Gundale et al., 2005; Metlen and Fiedler, 2006). Briefly, this study was implemented on an ~100-years-old second-growth ponderosa pine/Douglas-fir forest (100 years since last fire except one experimental block which is 60 years old) at the University of Montana's Lubrecht Experimental Forest in western Montana (46° 53′N 113° 27′W). Elevation ranges from 1,230 to 1,388 m, mean annual air temperature is 7°C and mean annual precipitation is 50 cm, with 44% falling as snow (Nimlos, 1986; Gundale et al., 2005). Soils at the study site are Eutric Haplocryalfs and Typic Dystrocryepts. The study was established as a semi-randomized block design (n = 3), with each block quartered into square 9 ha units, and assigned one of four treatments (control, burn-only, thin-only, and thin&burn). Selection harvest treatments reduced stand basal area from ~22–11 m2 ha−1 (trees >10 cm diameter), with harvest taking place in the winter of 2000/2001. Prescribed fires were conducted in May to June 25, 2002 and resulted in an average of 1–3.5 Mg ha−1 O horizon consumption and 12–27 Mg ha−1 woody fuel consumption. More detailed description of the study system can be found in Gundale et al. (2005).
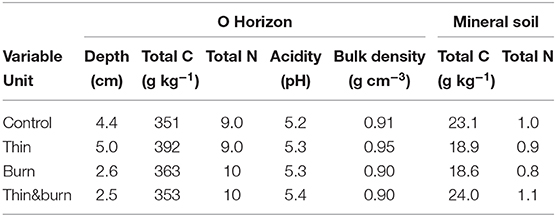
Table 1. Soil physical and chemical characteristics (mean) on plots at the Fire and Fire Surrogates study site at Lubrecht Experimental Forest (see Gundale et al., 2005).
Soil samples were collected from 10 stratified sub-sampling points within each of the 9 ha plots during July 2003. We collected mineral soil samples from a depth of 0–10 cm, using a standard 2.5 cm stainless steel soil probe with seven subsamples creating an individual sample. Complete organic horizon (Oi, Oe, and Oa) samples were collected within a 15.2 cm diameter PVC ring. Two mineral soil samples and two O horizon samples were collected from opposing corners of each plot and composited. The 10 composite samples were then independently analyzed for total PyC and averaged, yielding a single datum from each treatment replicate. All samples were dried at 60°C and stored until used for analysis.
We measured mineral soil and O horizon bulk density and organic horizon thickness to allow reporting of results on an area basis. Mineral soil bulk density was measured using a 5 cm diameter by 5 cm depth bulk density core with the dry weight of the sample divided by the volume of the core to estimate bulk density (Blake and Hartge, 1986). Organic horizon depth was measured from the top of the Oi horizon to the bottom of the Oa horizon (as above). Samples were then returned to lab and oven-dried (65°C) and weighed. Bulk density was calculated by dividing the dry mass of the material by the sample volume.
Both mineral soil samples and O horizon materials were ground using a shatterbox and passed through a 0.76 μm sieve. The PyC content of mineral soil samples and O horizon samples were determined by using a peroxide, weak nitric acid digestion procedure (the “KMD” method) as described by Kurth et al. (2006). The high organic matter content of the O horizon samples necessitated the modification of this digestion procedure wherein 0.5 g samples were pretreated with 30% H2O2 and allowed to stand for 24 h prior to starting the actual digestion. Samples of 1.0 g sample of mineral soil or 0.5 g of pre-treated O horizon material were placed into a 250 ml Erlenmeyer flask and amended with 20 ml of 30% H2O2 and 10 ml of 1.0 M HNO3. The mouth of the flasks were fitted with a 5 cm diameter glass sphere which allowed for gentle reflux, and the flasks were occasionally swirled at room temperature over a 30 min period, then heated to 100°C on a heating plate for 16 h. Samples were swirled occasionally and returned to the heating plate until the digestion was complete (no further effervescence). Samples were filtered through Whatman #2 filter paper, and then dried. Samples were scraped from the filter, homogenized with a mortar and pestle for total C determination by dry combustion (Elemental Analyzer 1500, Fissions Instruments, Milano, Italy). The total C measured after the digestion is reported as total PyC following the assumption that all non-PyC is consumed in the digestion process. In many of the O horizon samples, there was clearly some quantity of non-PyC organic matter remaining at the end of the digestion, in which case the PyC appeared black and non-PyC organic material appeared white. This incomplete digestion precluded analysis for total C on the digests as this approach would have overestimated total PyC content of the O horizon. The total mass was weighed and adjusted to a PyC content based on an ocular (at 20 × magnification) assessment of PyC as a percent of the total mass remaining. PyC samples from this study site averaged 750 g C kg−1 PyC, thus PyC mass in O horizon digests was converted to PyC as C by multiplying the mass by 0.75.
A generalized linear model was initially performed at a significant level of P = 0.05 where treatment was entered as a fixed factor and block was entered as a random factor. Because block was not a significant factor (P > 0.05), the comparison was repeated using a one-factor ANOVA followed by Tukey's post-hoc tests to identify differences among treatments at P = 0.05. A Pearson's correlation analysis was conducted to determine the relationship between total fuel consumption and PyC generation, where the goodness-of-fit R2 and associated P-value are presented. Data were checked for homogeneity and normality and were log-transformed when necessary to meet model assumptions. All analyses were conducted using SPSS (version 17.0, SPSS Inc., Chicago, IL, USA).
Results
Data Synthesis
Our analysis involving 22 independent fire event sites that were previously published on PyC generation rates (N = 31) showed that PyC was generated at an average rate of 2.17 (± SD 1.72) Mg C ha−1 in a single fire event that ranging from 0.05 to 6.4 Mg C ha−1, regardless of wildfire or prescribed (Rx) fire, with or without fuel reduction treatments (Figure 1A). There was no difference in the amount of PyC produced by wildfire or Rx fire; however, the amount of PyC produced by wildfire seemed to exhibit a higher degree of variation than Rx fire (Figure 1A). Thinning + Rx fire appeared to generate more PyC (1.95 ± SD 1.84 Mg C ha−1, Figure S1) than Rx fire only, but there was only one data point for this condition (1.40 Mg C ha−1, Figure S1). Of the 12 studies included in this synthesis, only nine (14 sites; N = 14) provided the % fuel conversion to PyC data (Figure 1B). On average, a single fire event converted 5.2 (± SD 2.5) % of biomass to PyC (Figure 1B).
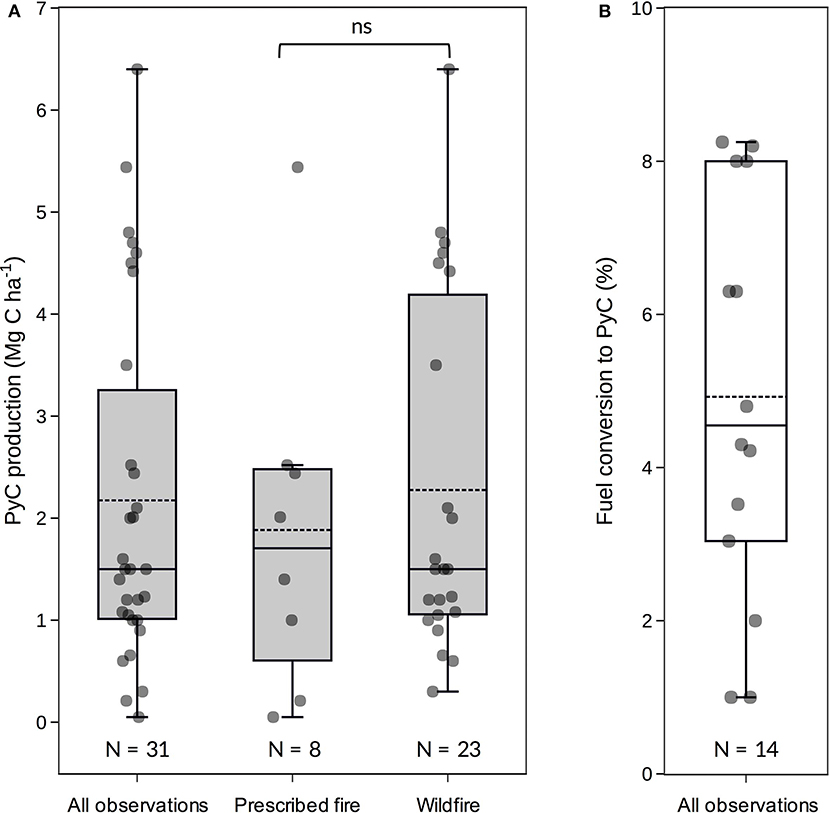
Figure 1. (A) Pyrogenic carbon (PyC) production (Mg C ha−1) and (B) fuel conversion to PyC (%) during wildfire and/or prescribed fire events as recorded at 22 individual fire event sites from 12 published studies associated with forest ecosystems (N = 31). Solid line indicates median and dashed line indicates mean, ns indicates no significant differences at P = 0.05. Detailed information on specific observation of PyC production rate can be found in Supplementary Material.
Empirical Study
Total PyC mass in the O horizon was directly influenced by restoration treatments that included Rx fire. The thin&burn treatment had the greatest PyC quantities present in the O horizon (Figure 2A). PyC mass in the forest floor was ~2.24 ± 0.72 Mg PyC ha−1 in the thin&burn treatment, these levels were significantly greater than the burn alone, thin alone, or control treatments (Figure 2A).
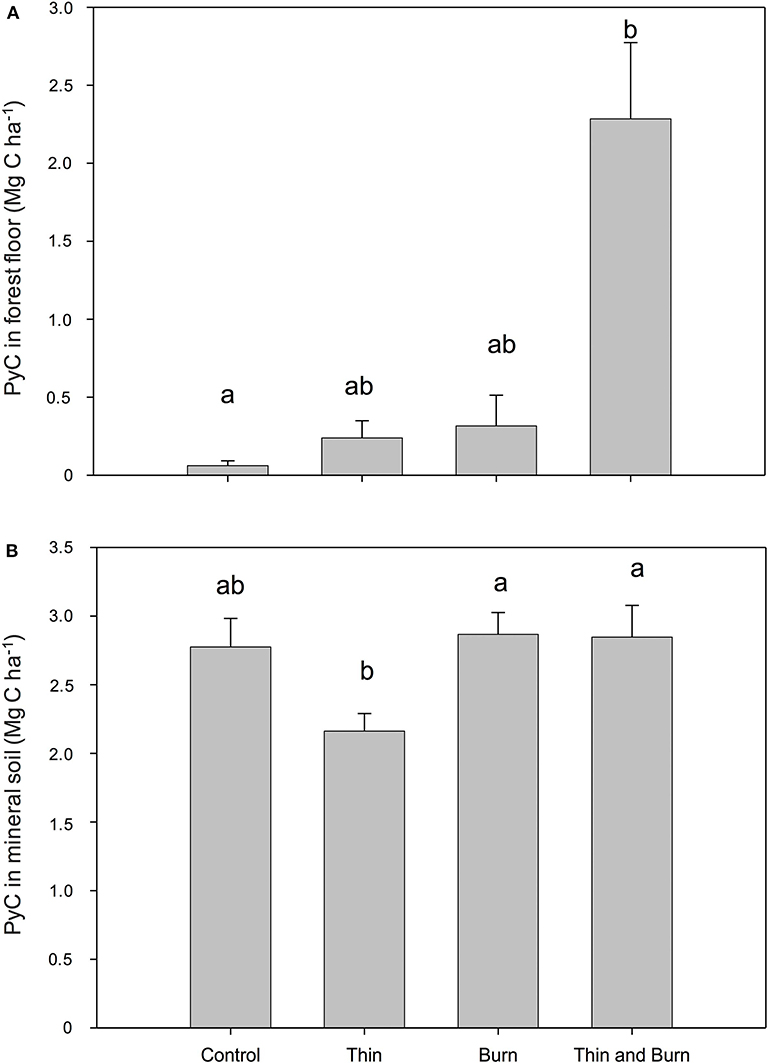
Figure 2. Pyrogenic carbon (PyC) (Mg C ha−1) in the (A) O horizon and (B) mineral soil as influenced by thinning with or without prescribed fire in the Fire and Fire Surrogates study in Western Montana, USA. Data were compared using Tukey's postdoc test following ANOVA. Error bar indicates 1 × standard error. Columns with the same letter are not significantly different at P = 0.05.
PyC in the mineral soil was significantly higher when comparing the burn and thin&burn plots to the thin alone plots, but there was no significant difference in PyC concentrations between the control and the burn or thinning plots (Figure 2B). Total PyC in the O horizon was found to generally follow fuel consumption rates with about 44% of the variation in PyC production being accounted for by fuel consumption (Figure 3). Fuel consumption was nearly 14 Mg ha−1 (fuel and forest floor) in the burn only treatments, where the total mass of fine fuel and forest floor consumed in the thin&burn plots was over 30 Mg ha−1.
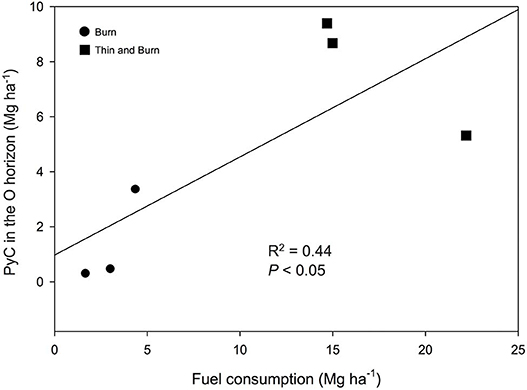
Figure 3. Correlation (R2 and P-value) between PyC in the O horizon (Mg ha−1) and the amount of fuel consumed (Mg ha−1) in “burn” and “thin&burn” treatments in the Fire and Fire Surrogates study in Western Montana, USA.
Discussion
In this study, we combined an assessment of previously published data on PyC production in forest wildfires or prescribed (Rx) fires with an empirical study from a Rx fire study in western Montana. The data synthesis from existing literature provides a range for biomass conversion to PyC during Rx fire and wildfire events with Rx fire events producing similar amounts of PyC, but a far lower degree of variation than wildfire events (Figure 1). This is somewhat expected given the relative low severity of most Rx fires. Previous studies have estimated PyC generation per unit fuel consumption in coniferous forest ecosystems to range from <1% to nearly 20% (Tinker and Knight, 2000; Lynch et al., 2004; Forbes et al., 2006) and more commonly reported as being between 1 and 5% (Preston and Schmidt, 2006). In a more recent detailed analysis of PyC generation in a boreal forest wildfire with pre and post-fire sampling canopy, understory, and surface soils, Santín et al. (2015) reported an average ecosystem conversion rate of 27% of forest biomass with a conversion rate of 50% for downed woody debris. These estimates are far higher than the average value of 5.2% of fuel consumption observed in our data synthesis, but may reflect the fact that most studies have not had the benefit of pre-and post-fire sampling (Miesel et al., 2018). Further, visual estimates can overestimate PyC generation rates compared to wet chemical digestion methods.
Importantly, there is little consistency between individual studies and the method employed to estimate PyC content generated during fire events, therefore the PyC production rate across studies was highly variable which creates challenges, particularly when modeling at larger spatial scale (Wei et al., 2018). By compiling published data of soil PyC content in the whole soil profile to estimate global PyC stock across a variety of environmental constraints (e.g., climate, soil pH, site net primary production), Reisser et al. (2016) found that soil properties, rather than fire characteristics best explained PyC content at global scales where high clay soils (clay content over 50%) and alkaline soils had more PyC content than low clay soils and acidic soils. The relatively small sample size of this data synthesis limits our ability to extrapolate the results; however, we report an average value of 2.2 Mg PyC ha−1 for a single fire event. From these data it was hard for us to further generalize correlations between environmental variables and PyC production per single fire event. It is possible that the differences in soil and site properties across geographically distinct sites contributed to the variation in PyC persistence; however, it is more likely that fire and fuel characteristics including burn temperature, fuel composition and loading, and fuel moisture were the strongest drivers of variation in PyC production across different studies (Huang et al., 2018). This is supported by our data synthesis which showed that the higher amount of PyC production was observed under “wildfire” compared with “Rx fire” (Figure 1A), and under “thinning + Rx fire” when compared with “Rx fire only” (Figure S1). The average value of 2.2 ± 1.7 Mg C ha−1 generated per fire event in the form of PyC is a close fit with the Rx fire data in our empirical study and suggests that PyC generation during Rx events is a significant contributor to the stable C pool in western forest ecosystems.
In our empirical study, the Rx fire treatments generated PyC in the O horizon at a rate of about 12% of fuel consumed in the thin plus burn treatments which is slightly higher than the average of our synthesis findings and midway between the estimate of Preston and Schmidt (2006) and that of Santín et al. (2015). Alexis et al. (2007) reported relatively lower PyC generation rates (3.2% of stem, leaf and litter converted to PyC post fire) in a sub-tropical scrub oak ecosystem and indicated that PyC accumulates at lower rates than conservative estimates of stable organic matter formation (7% of stem, leaf and litter). Insights from that ecosystem led the authors to suggest that fire and PyC generation lead to a long-term decline in soil C. However, this argument does not account for differences in the longevity of stable organic matter and PyC, which may be over 10 times greater for PyC compared to soil organic matter (DeLuca and Aplet, 2008). Further evidence for longevity of PyC in soils and an indication of the limited impact of a single burn event on soil PyC stocks is captured in the recent study by Santos et al. (2017) which investigated PyC stocks in litter layers (significantly different from a 100-years post-burn site to an unburned site) and dissolved PyC leaching from soil horizons (not significantly different from burned to unburned). At our FFS sites, the lack of differences in mineral soil PyC content across treatments is likely a product of historical fires, including a fire in the 1930's that covered portions of Block 1 and 3 (Gundale et al., 2005), whereas recent fire activity resulted in measurable changes in the O horizon PyC content.
Recent studies have pointed to PyC generation as offsetting C emissions during wildfire (Wei et al., 2018; Jones et al., 2019) requiring us to further assess the influence of fire on the global C balance. Importantly, these studies demonstrate that PyC accumulation in ecosystems is most directly related to fire severity and frequency (Sawyer et al., 2018; Adkins et al., 2019). So, although PyC generation in individual fire events in Savanah ecosystems is low, the net accumulation can be high. The same thinking must go into how we approach Rx fire in western forest ecosystems of the Inland Northwest, where multiple Rx fires over a 100 years period will store more PyC than a single high severity fire over the same time period (DeLuca and Aplet, 2008).
Conclusion
This study provides a combination of original empirical data on PyC generation as well as a data synthesis from prior studies that address PyC generation in forest wildfire and Rx fire events. The results presented here support previous studies showing that fires generate a significant quantity of PyC, a relatively passive form of organic C. Our empirical results further demonstrate that the majority of PyC mass resides in the mineral soil, even though PyC storage from recent fires resides in the O horizon (Brimmer, 2006; DeLuca and Sala, 2006; Kurth et al., 2006). Charcoal left on site likely disintegrates into smaller particles over time further affording incorporation into the mineral soil as a result of physical and biological pedoturbation (DeLuca and Aplet, 2008). This fine particulate C may also leach into deeper soil horizons over time (Santos et al., 2017). The reintroduction of fire using these restoration treatments in the region represents the first potential addition of PyC to these ecosystems over a 100-years period. Although the primary reasons for implementing restoration or fuel reduction treatments are for reducing wildfire risk and restoring historical ecosystem structure and function, there is clearly an additional benefit in that Rx fire generates a surprisingly large pool of highly stable C, particularly when it is applied in combination with forest thinning. And while the immediate effect of Rx fire is to release C, in the long term this approach may promote C sequestration. Future efforts to restore forest ecosystems in the Inland Northwest should incorporate Rx fire to further reduce surface fuel loading, reestablish more natural fire regimes, and support long-term ecosystem C storage.
Data Availability Statement
The datasets generated for this study are available on request to the corresponding author.
Author Contributions
TD, MG, and RB were involved in designing the empiric study, conducting field experiments, and laboratory analyses. TD and SG conducted data synthesis and analysis. TD, SG, and MG drafted the manuscript.
Funding
This research was partially conducted on the Montana Fire and Fire Surrogates plots, a study funded by the US Joint Fire Science Program and is part of the National Fire and Fire Surrogates Project (JSFP 04-S-02).
Conflict of Interest
The authors declare that the research was conducted in the absence of any commercial or financial relationships that could be construed as a potential conflict of interest.
Acknowledgments
The authors wish to thank Melissa Pingree and Casey Rossetto for their assistance in the laboratory.
Supplementary Material
The Supplementary Material for this article can be found online at: https://www.frontiersin.org/articles/10.3389/ffgc.2020.00024/full#supplementary-material
References
Abiven, S., and Santín, C. (2019). Editorial: from fires to oceans: dynamics of fire-derived organic matter in terrestrial and aquatic ecosystems. Front. Earth Sci. 7:31. doi: 10.3389/feart.2019.00031
Abney, R. B., Kuhn, T. J., Chow, A., Hockaday, W., Fogel, M. L., and Berhe, A. A. (2019). Pyrogenic carbon erosion after the rim fire, yosemite national park: the role of burn severity and slope. J. Geophys. Res. Biogeosci. 124, 432–449. doi: 10.1029/2018JG004787
Adkins, J., Sanderman, J., and Miesel, J. (2019). Soil carbon pools and fluxes vary across a burn severity gradient three years after wildfire in sierra nevada mixed-conifer forest. Geoderma 333, 10–22. doi: 10.1016/j.geoderma.2018.07.009
Alexis, M. A., Rasse, D. P., Knicker, H., Anquetil, C., and Rumpel, C. (2012). Evolution of soil organic matter after prescribed fire: a 20-year chronosequence. Geoderma 189–190, 98–107. doi: 10.1016/j.geoderma.2012.05.003
Alexis, M. A., Rasse, D. P., Rumpel, C., Bardoux, G., Péchot, N., Schmalzer, P., et al. (2007). Fire impact on C and N losses and charcoal production in a scrub oak ecosystem. Biogeochemistry 82, 201–216. doi: 10.1007/s10533-006-9063-1
Blake, G. R., and Hartge, K. H. (1986). “Bulk Density,” in Methods of Soil Analysis: Part 1—Physical and Mineralogical Methods, SSSA B. Ser. 5.1. ed A. Klute (Madison, WI: SSSA,ASA), 363–375.
Brimmer, R. J. (2006). Sorption Potential of Naturally Occurring Charcoal in Ponderosa Pine Forests of Western Montana (Master's Thesis). University of Montana, Missoula, MT, USA.
Carcaillet, C. (2001). Are holocene wood-charcoal fragments stratified in alpine and subalpine soils? Evidence from the alps based on AMS 14C dates. Holocene 11, 231–242. doi: 10.1191/095968301674071040
DeLuca, T. H., and Aplet, G. H. (2008). Charcoal and carbon storage in forest soils of the rocky mountain west. Front. Ecol. Environ. 6, 18–24. doi: 10.1890/070070
DeLuca, T. H., Gundale, M. J., MacKenzie, M. D., and Jones, D. L. (2015). “Biochar effects on soil nutrient transformations,” in Biochar for Environmental Management: Science, Technology and Implementation, eds J. Lehmann and S. Joseph (London: Routledge), 421–454.
DeLuca, T. H., MacKenzie, M. D., Gundale, M. J., and Holben, W. E. (2006). Wildfire-produced charcoal directly influences nitrogen cycling in ponderosa pine forests. Soil Sci. Soc. Am. J. 70, 448–453. doi: 10.2136/sssaj2005.0096
DeLuca, T. H., and Sala, A. (2006). Frequent fire alters nitrogen transformations in ponderosa pine stands of the inland northwest. Ecology 87, 2511–2522. doi: 10.1890/0012-9658(2006)87[2511:FFANTI]2.0.CO;2
Forbes, M. S., Raison, R. J., and Skjemstad, J. O. (2006). Formation, transformation and transport of black carbon (charcoal) in terrestrial and aquatic ecosystems. Sci. Total Environ. 370, 190–206. doi: 10.1016/j.scitotenv.2006.06.007
Gao, S., and DeLuca, T. H. (2018). Wood biochar impacts soil phosphorus dynamics and microbial communities in organically-managed croplands. Soil Biol. Biochem. 126, 144–150. doi: 10.1016/j.soilbio.2018.09.002
Gao, S., and DeLuca, T. H. (2019). Use of microdialysis to assess short-term soil soluble N dynamics with biochar additions. Soil Biol. Biochem. 136:107512. doi: 10.1016/j.soilbio.2019.06.008
Gao, S., DeLuca, T. H., and Cleveland, C. C. (2019). Biochar additions alter phosphorus and nitrogen availability in agricultural ecosystems: a meta-analysis. Sci. Total Environ. 654, 463–472. doi: 10.1016/j.scitotenv.2018.11.124
Gao, S., Hoffman-Krull, K., and DeLuca, T. H. (2017). Soil biochemical properties and crop productivity following application of locally produced biochar at organic farms on Waldron Island, WA. Biogeochemistry 136, 31–46. doi: 10.1007/s10533-017-0379-9
Gavin, D. G. (2004). Forest soil disturbance intervals inferred from soil charcoal radiocarbon dates. Can. J. For. Res. 33, 2514–2518. doi: 10.1139/x03-185
Gavin, D. G., Feng, S. H., Lertzman, K., and Corbett, P. (2006). Weak climatic control of stand-scale fire history during the late holocene. Ecology 87, 1722–1732. doi: 10.1890/0012-9658(2006)87[1722:WCCOSF]2.0.CO;2
Gul, S., and Whalen, J. K. (2016). Biochemical cycling of nitrogen and phosphorus in biochar-amended soils. Soil Biol. Biochem. 103, 1–15. doi: 10.1016/j.soilbio.2016.08.001
Gundale, M. J., and DeLuca, T. H. (2006). Temperature and source material influence ecological attributes of ponderosa pine and douglas-fir charcoal. For. Ecol. Manage. 231, 86–93. doi: 10.1016/j.foreco.2006.05.004
Gundale, M. J., DeLuca, T. H., Fiedler, C. E., Ramsey, P. W., Harrington, M. G., and Gannon, J. E. (2005). Restoration treatments in a montana ponderosa pine forest: effects on soil physical, chemical and biological properties. For. Ecol. Manage. 213, 25–38. doi: 10.1016/j.foreco.2005.03.015
Huang, W., Hu, Y., Chang, Y., Liu, M., Li, Y., Ren, B., et al. (2018). Effects of fire severity and topography on soil black carbon accumulation in boreal forest of Northeast China. Forests 9:408. doi: 10.3390/f9070408
Jones, M. W., Santín, C., van der Werf, G. R., and Doerr, S. H. (2019). Global fire emissions buffered by the production of pyrogenic carbon. Nat. Geosci. 12, 742–747. doi: 10.1038/s41561-019-0403-x
Keech, O., Carcaillet, C., and Nilsson, M. C. (2005). Adsorption of allelopathic compounds by wood-derived charcoal: the role of wood porosity. Plant Soil 272, 291–300. doi: 10.1007/s11104-004-5485-5
Kurth, V. J., MacKenzie, M. D., and DeLuca, T. H. (2006). Estimating charcoal content in forest mineral soils. Geoderma 137, 135–139. doi: 10.1016/j.geoderma.2006.08.003
Lehmann, J., Gaunt, J., and Rondon, M. (2006). Bio-char sequestration in terrestrial ecosystems–a review. Mitig. Adapt. Strateg. Glob. Chang. 11, 395–419. doi: 10.1007/s11027-005-9006-5
Lehmann, J., Rillig, M. C., Thies, J., Masiello, C. A., Hockaday, W. C., and Crowley, D. (2011). Biochar effects on soil biota—a review. Soil Biol. Biochem. 43, 1812–1836. doi: 10.1016/j.soilbio.2011.04.022
Lynch, J. A., Clark, J. S., and Stocks, B. J. (2004). Charcoal production, dispersal, and deposition from the fort providence experimental fire: interpreting fire regimes from charcoal records in boreal forests. Can. J. For. Res. 34, 1642–1656. doi: 10.1139/x04-071
Metlen, K. L., and Fiedler, C. E. (2006). Restoration treatment effects on the understory of ponderosa pine/douglas-fir forests in western Montana, USA. For. Ecol. Manage. 222, 355–369. doi: 10.1016/j.foreco.2005.10.037
Miesel, J., Reiner, A., Ewell, C., Maestrini, B., and Dickinson, M. (2018). Quantifying changes in total and pyrogenic carbon stocks across fire severity gradients using active wildfire incidents. Front. Earth Sci. 6:41. doi: 10.3389/feart.2018.00041
Nimlos, T. J. (1986). Soils of Lubrecht Experimental Forest. Misc. Publ. No. 44. Missoula, MT: Montana Forest and Conservation Experiment Station, School of Forestry, University of Montana. 36 p.
Pingree, M. R. A., and DeLuca, T. H. (2017). Function of wildfire-deposited pyrogenic carbon in terrestrial ecosystems. Front. Environ. Sci. 5:53. doi: 10.3389/fenvs.2017.00053
Pingree, M. R. A., Homann, P. S., Morrissette, B., and Darbyshire, R. (2012). Long and short-term effects of fire on soil charcoal of a conifer forest in Southwest Oregon. Forests 3, 353–369. doi: 10.3390/f3020353
Pluchon, N., Casetou, S. C., Kardol, P., Gundale, M. J., Nilsson, M.-C., and Wardle, D. A. (2015). Influence of species identity and charring conditions on fire-derived charcoal traits. Can. J. For. Res. 45, 1669–1675. doi: 10.1139/cjfr-2015-0076
Power, M. J., Whitlock, C., Bartlein, P., and Stevens, L. R. (2006). Fire and vegetation history during the last 3800 years in northwestern Montana. Geomorphology 75, 420–436. doi: 10.1016/j.geomorph.2005.07.025
Preston, C. M., and Schmidt, M. W. I. (2006). Black (pyrogenic) carbon: a synthesis of current knowledge and uncertainties with special consideration of boreal regions. Biogeosciences 3, 397–420. doi: 10.5194/bg-3-397-2006
Quilliam, R. S., Rangecroft, S., Emmett, B. A., Deluca, T. H., and Jones, D. L. (2013). Is biochar a source or sink for polycyclic aromatic hydrocarbon (PAH) compounds in agricultural soils? GCB Bioenergy 5, 96–103. doi: 10.1111/gcbb.12007
Reisser, M., Purves, R. S., Schmidt, M. W. I., and Abiven, S. (2016). Pyrogenic carbon in soils: a literature-based inventory and a global estimation of its content in soil organic carbon and stocks. Front. Earth Sci. 4:80. doi: 10.3389/feart.2016.00080
Ryan, K. C., Knapp, E. E., and Varner, J. M. (2013). Prescribed fire in North American forests and woodlands: history, current practice, and challenges. Front. Ecol. Environ. 11:120329. doi: 10.1890/120329
Santín, C., Doerr, S. H., Preston, C. M., and González-Rodríguez, G. (2015). Pyrogenic organic matter production from wildfires: a missing sink in the global carbon cycle. Glob. Chang. Biol. 21, 1621–1633. doi: 10.1111/gcb.12800
Santos, F., Wagner, S., Rothstein, D., Jaffe, R., and Miesel, J. R. (2017). Impact of a historical fire event on pyrogenic carbon stocks and dissolved pyrogenic carbon in spodosols in northern michigan. Front. Earth Sci. 5:80. doi: 10.3389/feart.2017.00080
Sawyer, R., Bradstock, R., Bedward, M., and Morrison, R. J. (2018). Fire intensity drives post-fire temporal pattern of soil carbon accumulation in Australian fire-prone forests. Sci. Total Environ. 610–611, 1113–1124. doi: 10.1016/j.scitotenv.2017.08.165
Thies, J. E., Rillig, M. C., and Graber, E. R. (2015). “Biochar effects on the abundance, activity and diversity of the soil biota,” in Biochar for Environmental Management: Science, Technology and Implementation, eds J. Lehmann and S. Joseph (London: Routledge), 327–389.
Tinker, D. B., and Knight, D. H. (2000). Coarse woody debris following fire and logging in wyoming lodgepole pine forests. Ecosystems 3, 472–483. doi: 10.1007/s100210000041
Wardle, D. A., Nilsson, M.-C., and Zackrisson, O. (2008). Fire-derived charcoal causes loss of forest humus. Science 320:629. doi: 10.1126/science.1154960
Wei, X., Hayes, D. J., Fraver, S., and Chen, G. (2018). Global pyrogenic carbon production during recent decades has created the potential for a large, long-term sink of atmospheric CO2. J. Geophys. Res. Biogeosci. 123, 3682–3696. doi: 10.1029/2018JG004490
Wiechmann, M. L., Hurteau, M. D., Kaye, J. P., and Miesel, J. R. (2015). Macro-particle charcoal C content following prescribed burning in a mixed-conifer forest, Sierra Nevada, California. PLoS ONE 10:e0135014. doi: 10.1371/journal.pone.0135014
Keywords: black carbon, carbon storage, fire, forest restoration, PyC, pyrogenic carbon
Citation: DeLuca TH, Gundale MJ, Brimmer RJ and Gao S (2020) Pyrogenic Carbon Generation From Fire and Forest Restoration Treatments. Front. For. Glob. Change 3:24. doi: 10.3389/ffgc.2020.00024
Received: 24 October 2019; Accepted: 24 February 2020;
Published: 10 March 2020.
Edited by:
Chengrong Chen, Griffith University, AustraliaReviewed by:
Blanca Cespedes, New Mexico Highlands University, United StatesMaría Florencia Urretavizcaya, National Council for Scientific and Technical Research (CONICET), Argentina
Jessica R. Miesel, Michigan State University, United States
Copyright © 2020 DeLuca, Gundale, Brimmer and Gao. This is an open-access article distributed under the terms of the Creative Commons Attribution License (CC BY). The use, distribution or reproduction in other forums is permitted, provided the original author(s) and the copyright owner(s) are credited and that the original publication in this journal is cited, in accordance with accepted academic practice. No use, distribution or reproduction is permitted which does not comply with these terms.
*Correspondence: Thomas H. DeLuca, dG9tLmRlbHVjYUB1bW9udGFuYS5lZHU=