- 1Institute of Human Nutrition and Food Science, University of Kiel, Kiel, Germany
- 2Institute of Animal Breeding and Husbandry, University of Kiel, Kiel, Germany
- 3GMA-Gesellschaft für Marine Aquakultur mbH, Büsum, Germany
- 4Department of Nutritional, Food, and Consumer Sciences, University of Applied Sciences Fulda, Fulda, Germany
- 5Department of Metabolism and Nutrition, Institute of Food Science, Food Technology and Nutrition (ICTAN-CSIC), Madrid, Spain
This study aimed to evaluate whether resveratrol (RSV) and its microbial metabolites dihydro-resveratrol (DHR) and lunularin (LUN) affected fatty acid metabolism and omega-3 polyunsaturated fatty acid (n3-PUFA) synthesis in cultured hepatocytes. To this end, cultured human HepG2 hepatocytes were treated with non-toxic concentrations of these polyphenols (40 μM) and Δ5- and Δ6-desaturase (FADS1 and FADS2, respectively) expression was measured. Resveratrol induced both genes but DHR and LUN showed no effect. Co-incubation of RSV with α-linolenic acid (ALA) also induced FADS1 and FADS2 expression. Moreover, transcription of carnitine palmitoyltransferase 1A and fatty acid synthase expression was increased, indicating induction of β-oxidation and fatty acid synthesis, respectively. Using gas chromatography to measure fatty acid levels, we observed the impact of RSV with and without ALA treatment on fatty acid composition. However, RSV reduced unsaturated while increasing saturated fatty acid levels. We found lower amounts of monounsaturated fatty acids (16:1n-7c, 18:1n-9c, 18:1n7c, and 20:1n-9) and n3-PUFA docosahexaenoic acid whereas unsaturated fatty acid levels, especially of stearic acid, were elevated. Of interest, once we co-incubated the cells with RSV together with bovine serum albumin, we found no differences in gene expression compared to cells without RSV treatment. Although we found no positive effect of RSV on n3-PUFA synthesis, the stilbene could possibly prevent cellular stress by decreasing unsaturated fatty acid levels.
Introduction
Consumption of omega-3-fatty-acid-rich foods (1), such as fish, walnuts, or algae (2, 3) may decrease cardiovascular disease risk. The most important omega-3 long-chain polyunsaturated fatty acids (n3-PUFA) are eicosapentaenoic acid (C20:5n-3; EPA) and docosahexaenoic acid (C22:6n-3; DHA). General recommendations of EPA and DHA consumption vary between 250 and 1,000 mg per day for healthy adults (4). Atlantic salmon (Salmo salar) contains approximately two grams of long chain PUFAs per 100 g, with farmed fish usually containing higher lipid and thus PUFA levels than wild fish (5). However, aquaculture cannot fully compensate for the decreasing amounts of wild fish and the increasing demand due to growing world population (6). Therefore, developing supplements or functional foods that favor the synthesis of n3-PUFAs from their essential precursor α-linolenic acid (C18:3n-3; ALA) (7) in humans could possibly be an alternative to fish oil consumption per se.
Synthesis of PUFAs (Figure 1) mainly occurs in liver (11). Here, fatty acid desaturases 1 and 2 (FADS1 and FADS2, respectively) insert double bonds into the carbon chains of EPA and DHA precursors. FADS1 adds a double bond to C20:4n-3 whereas FADS2 is responsible for desaturating ALA, C20:3n-3, and C24:5n-3 (12). FADS2 can desaturate at the Δ6- and Δ8-position and thus convert ALA to C18:4n-3 and C20:3n-3 to C20:4n-3 (13, 14). Desaturation at the Δ8-position is exclusively conducted by FADS2 (15).
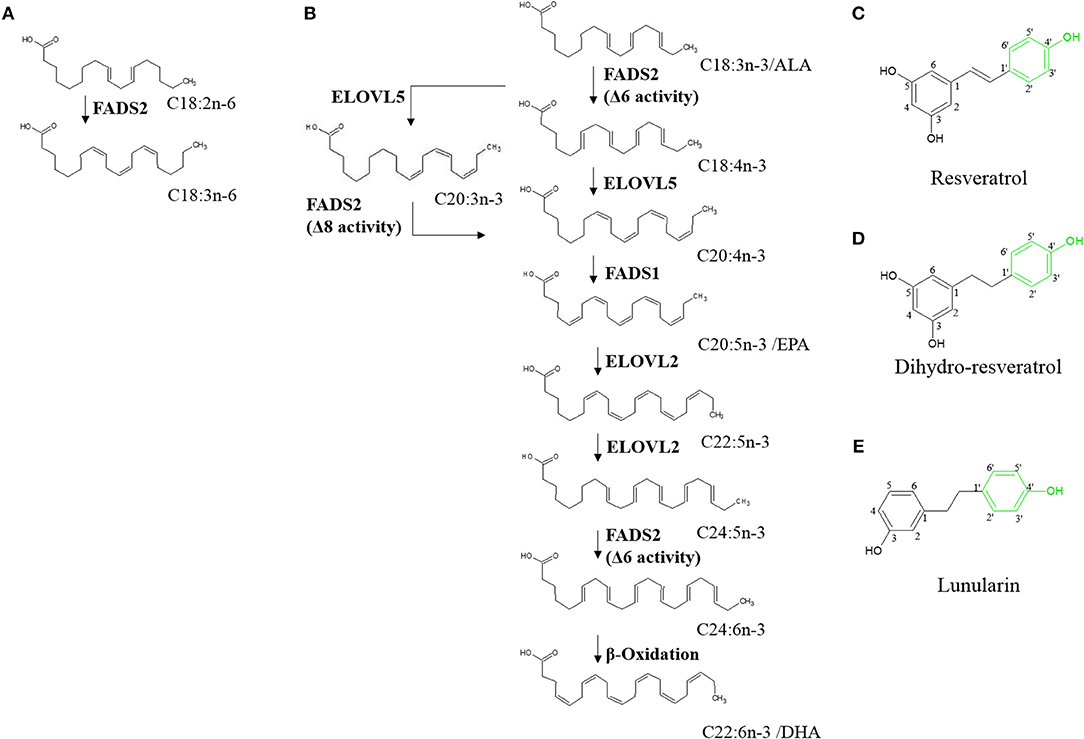
Figure 1. Hepatic endogenous synthesis of long-chain polyunsaturated fatty acids (PUFA) via fatty acid desaturases 1 and 2 (FADS1 and FADS2, respectively) (A,B) and test compounds used in the study (C–E). (A) step of omega-6 PUFA synthesis regulated via FADS2; (B) steps of the two possible pathways of omega-3 PUFA synthesis from α-linolenic acid (C18:3n-3/ALA). α-linolenic acid is either first desaturated by fatty acid desaturase 6 (FADS2) and then elongated by elongase of very long-chain fatty acids 5 (ELOVL5) or first elongated and then desaturated before being desaturated to eicosapentaenoic acid (C20:5n-3/EPA). Synthesis of docosahexaenoic acid (C22:6n-3/DHA) requires further elongation and desaturation and a final peroxisomal step of β-oxidation (8–10). (C–E) Chemical structures of resveratrol, dihydro-resveratrol and lunularin. Green marking indicates potentially responsible OH-groups for induction of peroxisome proliferator-activated receptor alpha.
Transcription factors regulating FADS1 and FADS2 gene expression are proliferator-activated receptor alpha (PPARα) (8), liver X receptor and sterol regulatory element binding protein-1c (SREBP-1c) (16). They are regulated by external ligands and cofactors and may induce or inhibit each other (17–20). Moreover, EPA and fasting conditions possibly activate PPARα, whereas C18:1n-9, ALA, C22:5n-3, and DHA impede its action (20). Interestingly and in contrast to regulation of PPARα, EPA and states of hunger have been shown to inhibit SREBP-1c (21). Further PPARα targets have been reported to code for proteins involved in β-oxidation such as carnitine palmitoyltransferase 1A (CPT1) (8). Thereby, the risks because of high levels of unsaturated phospholipids in the cell membrane and, consequently, oxidative damage may be lowered (22, 23).
Resveratrol (RSV) is a plant derived stilbene (Figure 1C) found in grapes and other fruits [reviewed by (24)]. Studies on the polyphenol have reported protective effects against coronary heart diseases [reviewed by (25)], antioxidant activities (26) and other effects that partly mimic those of caloric restriction [reviewed by (27)]. Furthermore, it has been hypothesized that RSV may activate PPARα (via the 4'-OH group; Figure 1C) (28). Thus, RSV may affect n3-PUFA synthesis (29–32).
In the gut, RSV was shown to be metabolized with type and amount of derivatives varying greatly inter- and intraspecifically. In humans, depending on the microbiota composition, RSV is metabolized to dihydro-resveratrol (DHR) and may be further metabolized to lunularin (LUN), both of which contain the potentially PPARα-mediating 4'-OH group (Figures 1D,E) (33). Low concentrations of DHR have been reported as showing proliferative actions whereas higher amounts, similar to RSV, are possibly rather antiproliferative agents (34). Furthermore, low doses of DHR may decrease cholesterol accumulation by inhibiting fatty acid binding protein 4 (35).
In order to compare how RSV and its gut metabolites DHR and LUN affect FADS1 and FADS2 expression, we included all three polyphenols in our experiments. In addition, due to the positive effects reported for RSV on fatty acid metabolism, we aimed to determine the potential of RSV and its derivatives DHR and LUN to modulate fatty acid metabolism and especially n3-PUFA synthesis in vitro.
Materials and Methods
Materials
α-Linolenic acid was obtained from Sigma-Aldrich, Buchs, Switzerland. Resveratrol (IUPAC name: 5-[(E)-2-(4-hydroxyphenyl)ethenyl] benzene-1,3-diol; C14H12O3; CAS number 501-36-0; purity ≥98%) was provided by Carl Roth (Karlsruhe, Germany). Dihydro-resveratrol (IUPAC name: 5-[2-(4-hydroxyphenyl)ethyl] benzene-1,3-diol; C14H14O3; CAS number 58436-28-5; purity ≥98%) was synthesized according to Faragher et al. (36) and LUN (IUPAC name: 3-[2-(4-hydroxyphenyl)ethyl] phenol; C14H14O2; CAS number 37116-80-6; purity >97%) was obtained from Ark Pharm, Inc. (Illinois, USA).
Cell Culture
HepG2 cells (IAZ, Munich, Germany; ATCC No: CCL-23) were cultured in RPMI-1640 with L-glutamine (PAN Biotech, Aidenbach, Germany). The passage number was ≤25. The medium was supplemented with 10% (vol/vol) heat inactivated fetal bovine serum (Gibco™ by Thermo Fisher Scientific GmbH, life technologies™, Darmstadt, Germany) and 1% penicillin/streptomycin (PAN Biotech, Aidenbach, Germany). Cells were grown at 37°C in a humidified 5% CO2 incubator in T75 or T175 flasks (37). Cytotoxicity was tested with a neutral red assay (38). To make results comparable, RSV, DHR, and LUN were used at the same non-toxic concentration of 40 μM. Cells were treated at 80% confluence for periods indicated in the subsequent sections. Test compounds were dissolved in dimethyl sulfoxide (DMSO). For co-incubation experiments, 40 mM stock solutions of ALA in EtOH were used to give a final ALA concentration of 50 μM. In all cases, incubations were carried out in duplicate. Experiments were independently performed three times.
RNA-Isolation and Quantitative Real Time (qRT) Polymerase Chain Reaction (PCR)
Cells were treated with 40 μM compounds; in the case of RSV with or without co-incubation with ALA. After 24 h of treatment, cells were harvested with peqGOLD TriFast and RNA was isolated according to the manufacturer's protocol (peqGOLD TriFast, VWR International, Radnor, USA). Sample RNA-concentrations and quality were determined with a Nano Drop 2000 (Thermo Fisher Scientific GmbH, life technologies™, Darmstadt, Germany). The A260 was used to calculate RNA concentrations. The ratio of absorbance at 260 and 280 nm was used to assess of RNA purity. Samples were frozen at −80°C at a concentration of 100 ng/μl and the 260/280 ratio was ≥1.8. The ratios for all samples can be found in Supplementary Material. Gene expression was analyzed via quantitative real time polymerase chain reaction (qRT-PCR) with SensiFAST™ SYBR® No-ROX One-Step Kit (Bioline GmbH, Luckenwalde, Germany) using a Rotorgene 6000 cycler (Corbett Life Science, Sydney, Australia). To this end, samples were diluted with nuclease free water and the qRT-PCR reagents to a final concentration of 0.2 ng/μl. Sequences of used primers FADS1, FADS2, CPT1, fatty acid synthase (FASN), and housekeeping gene glyceraldehyde-3-phosphate dehydrogenase (GAPDH) are given in Table 1. Primers for FADS1, FADS2, CPT1, and GAPDH were self-designed using Primer3web. The FASN primer was obtained from the Harvard Primer Bank (PrimerBank ID 41872630c1) (39).
The expression levels of the target genes were determined by normalizing the calculated relative concentrations to the housekeeping gene GAPDH.
Western Blotting Analyses
Cells were treated with 40 μM of RSV with or without ALA for 24 h. For protein analysis, whole cell protein lysates from HepG2 were harvested with RIPA buffer (50 mmol/l Tris, 150 mmol/l NaCl, 0.5% sodium deoxycholate (v/v), 0.1% SDS (w/v), 1% NP-40 (v/v), pH 7.4) supplemented with proteinase inhibitors. Protein concentrations were determined with the Pierce™ BCA assay (Thermo Fisher Scientific GmbH, life technologies™, Darmstadt, Germany) according to the manufacturer's instructions and analyzed by Western Blotting analysis as described before (40). Briefly, 30 μg per samples were heated with loading buffer and applied to Mini-PROTEAN® Stainfree™ Precast Gels (4–20%, BioRad laboratories GmbH, Munich, Germany). Samples were transferred to a polyvinylidenedifluoride membrane (Bio-Rad laboratories GmbH, Munich, Germany) and blocked with skim milk dissolved in Tris-buffered saline + 0.05% (v/v) Tween 20. Membranes were then incubated with the primary antibodies FADS1, FADS2, CPT1, GAPDH (sc-134337, sc-98480, sc-48357, and sc-20357, respectively; Santa Cruz Biotechnology Inc., Dallas, USA) and FASN (bs-5045R, Bioss Inc., Massachusetts, USA). Secondary antibodies were from Santa Cruz Biotechnology Inc., Dallas, USA. Results were analyzed using Image Lab 5.0 (Bio-Rad laboratories GmbH, Munich, Germany).
Analysis of Fatty Acid Composition
Cells were treated for either 24 or 48 h and harvested by adding 1 mL of Dulbecco's phosphate buffered saline to each well, scratching the cells gently off the plate and transferring the suspension to 2 mL cups, maintaining the samples always on ice. After centrifugation at 2,000 g and 4°C for 5 min, the supernatant was discarded and pellet was resuspended in 200 μl of 0.2M sodium chloride. Samples were frozen at −80°C until further analysis. Fatty acid composition was analyzed according to Vauzour et al. (41) by gas chromatography with a flame ionization detector. Briefly, samples were lyophilized at −60°C for 19 h followed by 1 h at −70°C. Fatty acid methyl esters (FAMEs) were synthesized by incubation with 0.5 M sodium methoxide and incubated at 60°C for 15 min, followed by extraction with acetyl chloride in methanol 1:10 (v/v) and incubation at 60°C for 60 min. One milliliter purified water, 1.5 mL hexan, and sodium sulfate were added to each sample, followed by a centrifugation step (4°C, 1,500 rpm for 5 min). One milliliter of the supernatant was used for further analysis. Samples were evaporated in a Savant™ SPD131DDA SpeedVac™ Concentrator (Thermo Fisher Scientific, Madrid, Spain) and resuspended in hexane. FAMEs analysis was conducted in a 7820A Agilent gas chromatograph (Agilent Technologies Spain) equipped with an Agilent HP-23 capillary column (60 m × 250 μm × 0.25 μm, Agilent Technologies Spain) and helium (1.0 mL/min) as the carrier gas. The temperature protocol was the following: initial temperature 100°C, ramp 8°C/min to 145°C (20 min), ramp 5°C/min to 195°C (5 min), ramp 5°C to 215°C (15 min), ramp 5°C to 230°C (5 min). Chromatograms were recorded and analyzed using Agilent EZChrom Elite software (Agilent Technologies Spain) with 13:0 as the internal standard. Fatty acid composition was calculated as a percentage of the total identified FAMEs. The method was validated with original standards for every fatty acid quantified. Additionally, tridecanoic acid (C13:0) was used as internal standard and in every case a response factor of 1 was used. The limit of quantification was calculated as being below 0.02 mg/g dry matter and the limit of detection was 0.005 mg/mL for every fatty acid.
Statistics
Statistical analyses were performed with the software RStudio (RStudio, Version 1.0.136, Inc., Boston, USA) (42), using an appropriate mixed model (43, 44). Normal distribution was determined before the analyses with the Shapiro-Wilk-Test and by graphical interpretation using a residual plot. The treatment was regarded as a fixed factor and the replicate as a random factor. Based on this model, a Pseudo R2 was calculated (45) and an analysis of variances (ANOVA) was conducted, followed by a post-hoc multiple comparison test of Dunnett (46) to compare the the effects to the DMSO control or a post-hoc multiple comparison test of Tukey to determine differences between the means of all treatments.
Results
Compound cytotoxicity was tested in a neutral red assay (Supplementary Figure S1) and only a non-toxic dose was applied in the following experiments.
Treating HepG2 cells with 40 μM of RSV induced transcription of FADS1 and FADS2 while 40 μM of DHR and LUN showed no effect (Figures 2A,B). On the protein level, RSV slightly lowered FADS1 and did not have any impact on FADS2 (Figure 2C; Supplementary Figure S3).
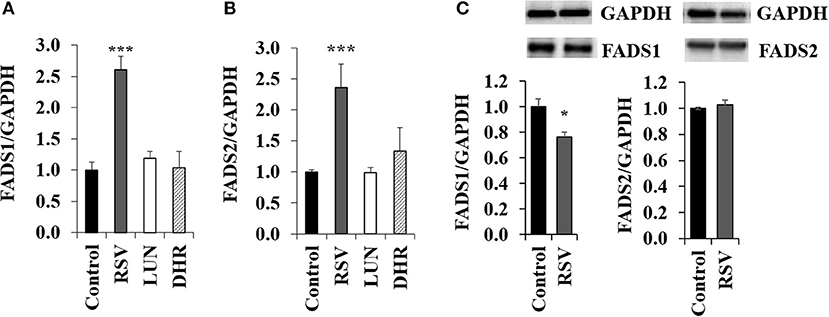
Figure 2. Effects of treating HepG2 for 24 h with 40 μM of resveratrol (RSV), lunularin (LUN) or dihydro-resveratrol (DHR) on the mRNA level of (A) fatty acid desaturase 1 (FADS1) and (B) fatty acid desaturase 2 (FADS2). Values are relative to the solvent control DMSO set to be 1.0. Expression levels were normalized to glyceraldehyde 3-phosphate dehydrogenase (GAPDH) and are shown as means + standard error (n = 3; * P ≤ 0.05, ***P ≤ 0.001; with post-hoc multiple comparison test of Dunnett). Effects of treating HepG2 for 24 h with 40 μM of resveratrol on protein levels of FADS1 and FADS2 (C), analyzed by Western Blotting analysis. Protein levels were normalized to the housekeeping protein GAPDH.
We conducted a second experiment to determine whether application of external ALA influenced RSV action. In our first attempt, we applied ALA complexed with BSA. When applying RSV together with BSA, the induction of FADS1 and FADS2 disappeared (Supplementary Figures S2, S4), possibly because of RSV binding to BSA (47). Therefore, we chose to apply ALA dissolved in EtOH for our experiments of co-incubation with RSV. When adding EtOH-dissolved ALA, FADS2 expression was inhibited compared to the EtOH solvent control. Induction of FADS1 and FADS2 after 40 μM RSV co-incubation with 50 μM of ALA (Figures 3A,B) was almost as strong as when applying RSV alone (Figures 2A,B). However, protein analysis showed a tendency toward lower FADS1 levels due to treatment with RSV and ALA (Figures 3E,F; Supplementary Figure S5).
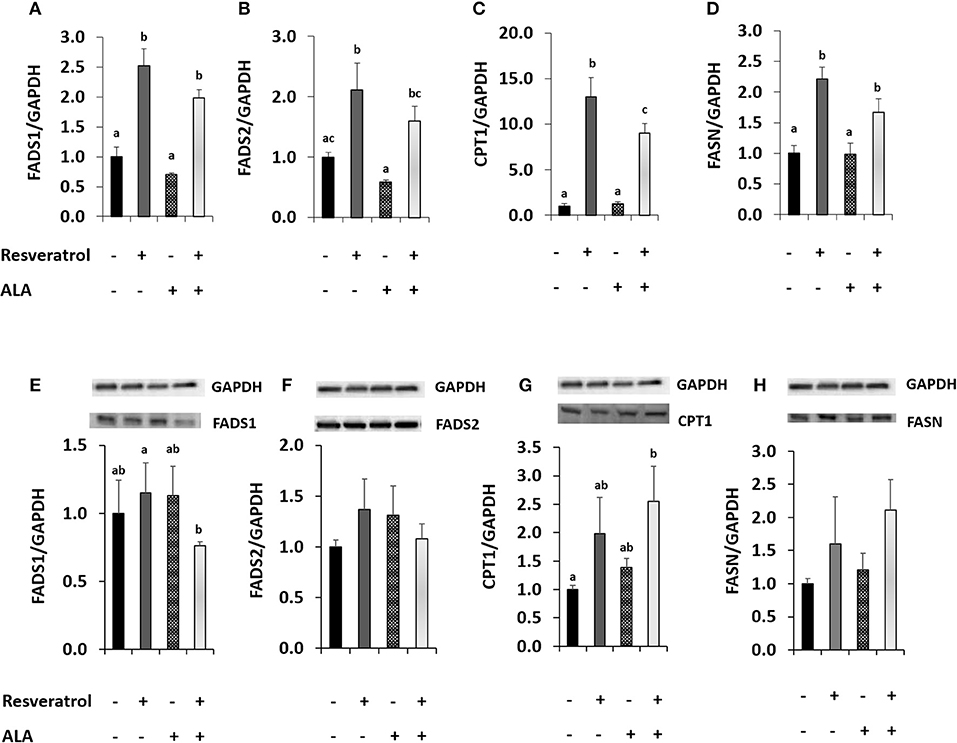
Figure 3. Effects of treating HepG2 for 24 h with 40 μM of resveratrol and 50 μM α-linolenic acid (ALA) in ethanol (EtOH) or 0.125% EtOH + 0.04% DMSO solvent control on (A) fatty acid desaturase 1 (FADS1), (B) fatty acid desaturase 2 (FADS2), (C) carnitine palmitoyltransferase 1A (CPT1), and (D) fatty acid synthase (FASN) mRNA levels. Values are relative to DMSO + EtOH control, which was set to be 1.0. Expression levels were normalized to the housekeeping gene glyceraldehyde 3-phosphate dehydrogenase (GAPDH). n = 3; mean + standard error. Values with different superscript letters differ significantly with p-values < 0.05; with post-hoc multiple comparison test of Tukey. Effects of treating HepG2 for 24 h with 40 μM of resveratrol and 50 μM ALA in EtOH or EtOH + DMSO solvent control on protein levels of FADS1, FADS2, CPT1, or FASN (E-H), analyzed by Western Blotting analysis. Protein levels were normalized to the housekeeping protein GAPDH.
Resveratrol-induced CPT1 transcription was more than 10-fold higher than in the solvent control and remained around 9-fold higher when comparing ALA with ALA and RSV (Figure 3C). Resveratrol also induced FASN (Figure 3D). Levels of both proteins appeared to be elevated by RSV and ALA treatment in Western blots, albeit effects on FASN were not significant (Figures 3G,H).
Determining fatty acid composition with gas chromatography, we found that in cells treated with RSV and ALA, stearic acid and total amounts of saturated fatty acids were enhanced. In contrast, levels of monounsaturated fatty acids C16:1n-7, C18:1n-9c, and C18:1n-7c were decreased. Total levels of PUFA were affected due to the addition of ALA but not the treatment with RSV. While α-linolenic acid levels tended to and amounts of C20:3n-3 were increased, C22:5n-3 and DHA levels were reduced compared to non-ALA treated cells. With exception of control ALA treated cells, EPA levels were under the detectable limit. Fatty acid composition after 24 and 48 h was very similar (Tables 2, 3).
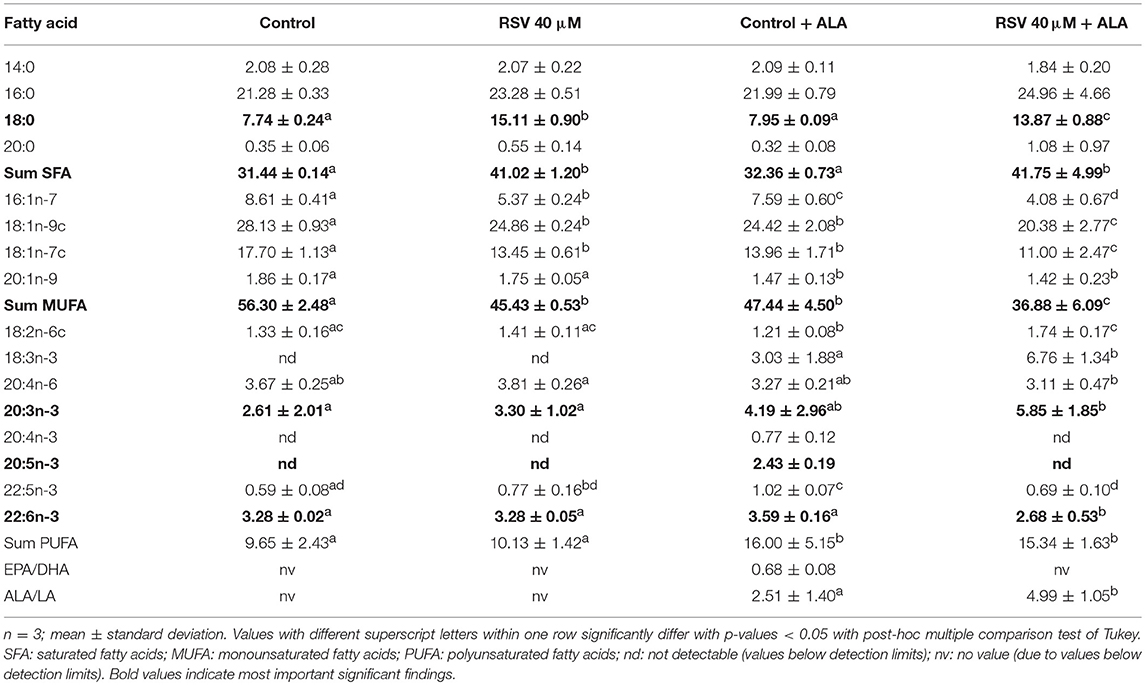
Table 2. Fatty acid composition (in % of total fatty acid methyl esters) of HepG2 cells treated for 24 h with 40 μM of resveratrol (RSV) and 50 μM of α-linolenic acid (ALA) dissolved in ethanol (EtOH) or solvent control EtOH + DMSO.
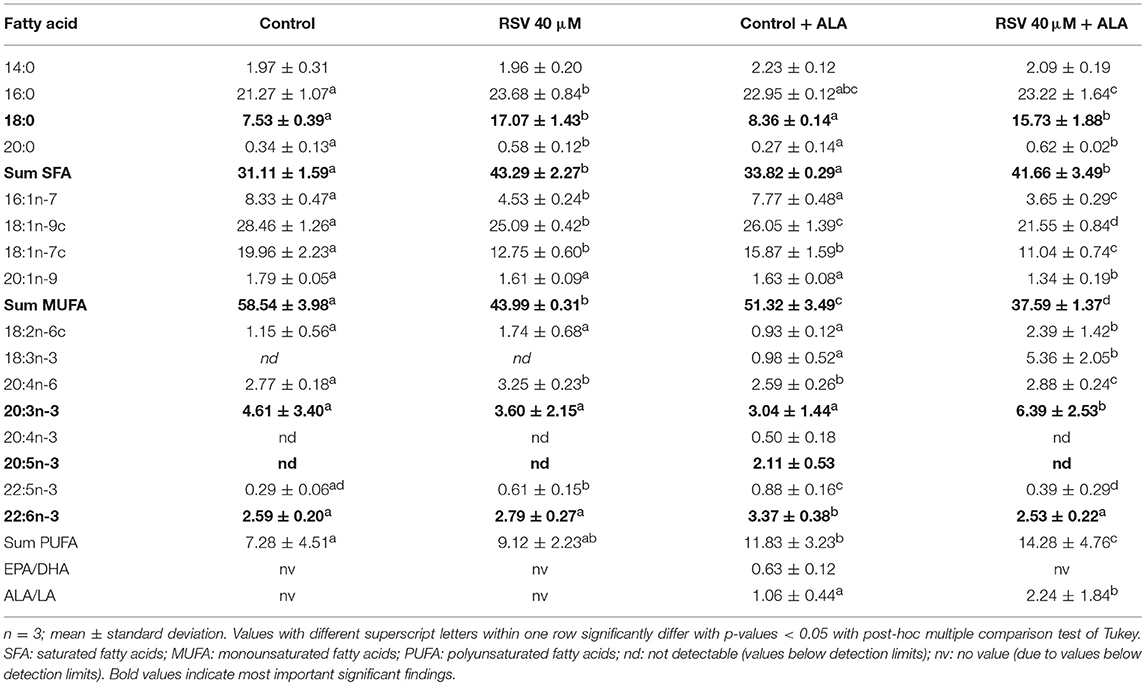
Table 3. Fatty acid composition (in % of total fatty acid methyl esters) of HepG2 cells treated for 48 h with 40 μM of resveratrol (RSV) and 50 μM of α-linolenic acid (ALA) dissolved in ethanol (EtOH) or solvent control EtOH + DMSO.
When complexing ALA with BSA (Supplementary Tables S1, S2), the impact of RSV was similar albeit less strong than with EtOH dissolved ALA. In both experiments, total lipid contents did not differ significantly between the treatments (data not shown).
Discussion
Resveratrol but not its metabolites LUN or DHR induced the expression of FADS1 and FADS2 when used at a concentration of 40 μM. While the 4′-OH group in RSV was shown to be important for PPARα activation (28), cell cycle and antioxidant effects potentially further depend on the 3- and 5-OH groups and the important trans double bond in the stilbenic skeleton (48). Thus, the missing impacts of LUN and DHR on FADS1 and FADS2 transcription may be due to the saturated instead of trans-unsaturated bond in both compounds and, additionally, the absence of a 3-OH group in the structure of LUN (Figures 1C–E). Furthermore, it has to be considered that we did not measure intracellular concentrations of the test compounds and therefore cannot exclude lowered bioavailability of LUN and DHR compared to RSV.
ALA-addition to the cells did not significantly change FADS1 or FADS2 protein levels compared to non-ALA treated cells with or without RSV.
However, as can be seen in the fatty acid analyses (Tables 2, 3), ALA appears to be taken up by the cells. This coincides with a previously reported dose-dependent increase of intracellular ALA after ALA co-treatment (49). Furthermore, RSV treatment increased ALA levels even more, which has been found in rat hepatocytes treated with 50 μM RSV (30) and Atlantic salmon supplemented with 2.5 g/kg RSV (32).
Interestingly, in the cells, levels of total saturated fatty acids, especially stearic acid, were increased by RSV treatment, whereas amounts of monounsaturated fatty acids were lowered. Application of RSV and ALA increased C20:3n-3 levels while lowering EPA, C22:5n-3, and DHA levels. Eicosatetraenoic acid (C20:4n-3) was not detectable in cells treated with RSV and ALA. Based on our mRNA data for FADS2, we did not expect a decrease of fatty acids that are synthesized by this gene product. However, enhanced levels of C20:3n-3 but non-detectable levels of C20:4n-3 after application with RSV and ALA may be caused by decreased FADS2/Δ8-desaturase activity (1) or increased β-oxidation (2):
1. The initial elongation of ALA may be initiated but activity of FADS2 may be inhibited by RSV. As a multi-pass membrane protein, FADS2 (50) possibly interacts with RSV which would be expected to accumulate in membrane departments due to its low solubility in aqueous environments. If RSV somehow inhibited FADS2, it might not be able to act at the Δ6-position and instead, ELOV5 might induce the alternative pathway of synthesis of n3-PUFA (13). However, Δ8-desaturase activity of FADS2 may also be repressed, making C20:3n-3 potentially an inhibitor of further fatty acid desaturation and elongation (Figure 1). Additionally, it has been hypothesized that animals lack Δ8-desaturase activity (51). Missing C20:4n-3 and no effects on FADS2 protein levels after treatment with RSV and EtOH-dissolved ALA supports the theory of missing or blocked Δ8-desaturase activity. This may explain, why C20:3n-3 accumulates in cells treated with ALA, although mRNA level of FADS2 is elevated.
In contrast, detectable levels of C20:4n-3 being the product of C20:3n-3 desaturation and C18:4n-3 elongation in control samples indicate an induction and execution of the pathway of n3-PUFA synthesis in the absence of RSV. In accordance with this hypothesis of inhibited n3-PUFA synthesis after C18:3n-3 elongation in RSV-treated cells, EPA, C22:5n-3, and DHA levels were lowered in these samples.
2. Another explanation may be an increased β-oxidation and thereby degradation of mono- and polyunsaturated fatty acids. Potential induction of β-oxidation is indicated by enhanced CPT1 expression and protein levels, CPT1 being essential for the passage of fatty acids through the mitochondrial membrane (52). As reported before (53), we found RSV to highly increase CPT1 expression. Thus, via β-oxidation, unsaturated fatty acids may directly be metabolized to saturated fatty acids (54). Additionally, unsaturated fatty acids may be broken down to acetyl CoA, which in turn can be used as a precursor for de novo synthesis of saturated fatty acids (55). De novo synthesis of fatty acids seems to be induced by RSV, since we found it to increase the expression and protein levels of FASN, which is central in fatty acid synthesis (56).
In contrast to PUFAs, saturated fatty acids are rather related to increased inflammation and high low density lipoprotein cholesterol levels (57, 58). However, it has been hypothesized that stearic acid may improve fitness and lower free fatty acid levels in fruit flies (59). In laboratory mice, dietary stearic acid was reported as reducing visceral adipose tissue. The authors related the effect to apoptosis induction of fat cells due to stearic acid application, which they confirmed in vitro in cultured preadipocytes (60). Furthermore, stearic acid may also decrease cholesterol absorption and inflammatory response (61, 62).
Resveratrol may reduce inflammation by lowering the levels of high-sensitivity C-reactive protein and CC-chemokine ligand 5 in vivo (63, 64). In human plasma, these markers were found to be modulated by different fatty acids. Stearic acid and C18:2n-6, which we found to be increased by RSV treatment (Tables 2, 3), may also lower high-sensitivity C-reactive protein and CC-chemokine ligand 5 levels [reviewed by Hunter et al. (65)]. Resveratrol-induced C18:2n-6 increase is in line with previous results in Atlantic salmon (32). The positive effects reported for RSV on cholesterol levels and inflammation (66, 67) may hypothetically, in part, be related to its alteration of fatty acid profiles. Additionally, C16:1n-7, C18:1n-9c, and C18-1n-7c, which were decreased by RSV (Tables 2, 3), have been related positively to the induction of inflammatory enzymes, such as high-sensitivity C-reactive protein and CC-chemokine ligand 5 (62).
An increase of C20:3n-3 may lower inflammation since it has been reported as dose dependently inhibiting inflammatory markers. Of interest, this n3-fatty acid was shown to improve skin barrier function and health in UV-irradiated hairless mice, possibly via the nuclear factor kappa-light-chain-enhancer of activated B cells pathway (68). However, C20:3n-3 has a lower anti-inflammatory potential than EPA (69).
Since unsaturated carbon chains of n3-PUFA enhance susceptibility to oxidative damage (23), RSV may hypothetically protect cells by lowering levels of EPA, C22:5n-3, and DHA. Of interest, RSV has been shown to induce effects similar to caloric restriction [reviewed by Pallauf et al. (27)]. One of the putatively life-span prolonging mechanisms, which RSV and caloric restriction share, could possibly be lowering unsaturated FA content of membrane lipids (70). If such a mechanism contributed to lifespan extension, one could possibly explain species-dependent effects of RSV on longevity, since saturation indexes of membrane very between species [reviewed by Hector et al. (24)].
The increase of FADS1 and FADS2 expression may be due to RSV inducing their transcription via PPARα (8, 71). Conversely, although we found elevated mRNA levels of FADS1 and FADS2 after RSV treatment, neither protein levels nor fatty acid concentrations of n3-PUFA were elevated after 24 or 48 h. We cannot exclude a long-term enhancing effect of RSV on n3-PUFA levels. However, decreased EPA due to RSV treatment but no influence on FADS expression was reported before for salmon fed 1.5 g RSV per kg feed (32). This was in contrast to previous findings in trout fed 0.3% dry matter RSV (increased n3-PUFA but no effect on FADS2) and rat hepatocytes exposed to 50 μM RSV (increased n3-PUFA in phospholipids) (30, 31), indicating potential species- and/or dose-specific effects.
It needs to be considered that concentrations of the test compounds, as used in the present cell culture study, were manifold higher than plasma concentrations reported in humans (72).
In conclusion, albeit application of 40 μM RSV to human hepatocytes increased the expression of genes involved in n3-PUFA synthesis, levels of EPA and DHA were reduced. Resveratrol, furthermore, led to elevated amounts of stearic acid, a finding, which should be validated in studies in laboratory rodents and humans. RSV metabolites LUN and DHR did not show an impact on FADS1 or FADS2.
Data Availability
The raw data of this study may be requested of the corresponding author of this article.
Author Contributions
The experimental work was conducted by GK and BD-R under the supervision of KP, GR, and SdP-T. MB synthesized the test compound dihydro-resveratrol. The study was designed by GR, KP and CS. All authors contributed to the methods and interpretation of the results. The text was written by GK, KP, and GR and revised by the other authors. All authors have given approval to its final version.
Conflict of Interest Statement
CS was employed by Gesellschaft für Marine Aquakultur mbH (Büsum, Germany).
The remaining authors declare that the research was conducted in the absence of any commercial or financial relationships that could be construed as a potential conflict of interest.
Acknowledgments
We acknowledge financial support by Land Schleswig-Holstein within the funding programme Open Access Publikationsfonds. GK was kindly funded by a fellowship from the Wilhelm Schaumann foundation (Hamburg, Germany). SdP-T was funded by the Spanish MICINN through the grant AGL2016-76832-R.
Supplementary Material
The Supplementary Material for this article can be found online at: https://www.frontiersin.org/articles/10.3389/fnut.2018.00106/full#supplementary-material
Abbreviations
ALA, α-linolenic acid; BSA, bovine serum albumin; CPT1, carnitine palmitoyltransferase 1A; DHA, docosahexaenoic acid; DHR, dihydro-resveratrol; EPA, eicosapentaenoic acid; EtOH, ethanol; FADS, fatty acid desaturase; FAME, Fatty acid methyl ester; FASN, fatty acid synthase; GAPDH, glyceraldehyde 3-phosphate dehydrogenase; n3-PUFA, omega-3 polyunsaturated fatty acid; LUN, lunularin; PPAR, peroxisome proliferator-activated receptor; RSV, resveratrol; SREBP-1c, sterol regulatory element binding protein-1c.
References
1. DeFilippis AP, Sperling LS. Understanding omega-3's. Am Heart J. (2006) 151:564–70. doi: 10.1016/j.ahj.2005.03.051
2. Zhao G, Etherton TD, Martin KR, West SG, Gillies PJ, Kris-Etherton PM. Dietary alpha-linolenic acid reduces inflammatory and lipid cardiovascular risk factors in hypercholesterolemic men and women. J Nutr. (2004) 134:2991–7. doi: 10.1093/jn/134.11.2991
3. Arterburn LM, Oken HA, Hoffman JP, Bailey-Hall E, Chung G, Rom D et al. Bioequivalence of Docosahexaenoic acid from different algal oils in capsules and in a DHA-fortified food. Lipids (2007) 42:1011–24. doi: 10.1007/s11745-007-3098-5
4. Global Organization for EPA and DHA and Omega-3s. Global Recommendations for EPA and DHA Intake. Salt Lake City, UT (2015). p. 17.
5. Zárate R, El Jaber-Vazdekis N, Tejera N, Pérez JA, Rodríguez C. Significance of long chain polyunsaturated fatty acids in human health. Clin Transl Med. (2017) 6:25. doi: 10.1186/s40169-017-0153-6
6. FAO. (2016). The State of World Fisheries and Aquaculture. Rome: Food and Agriculture Organization of the United Nations.
7. Burr GO, Burr MM. Nutrition classics from The Journal of Biological Chemistry 82:345-67, 1929. a new deficiency disease produced by the rigid exclusion of fat from the diet. Nutr Rev. (1973) 31:248–9. doi: 10.1111/j.1753-4887.1973.tb06008.x
8. Rakhshandehroo M, Knoch B, Müller M, Kersten S. Peroxisome proliferator-activated receptor alpha target genes. PPAR Res. (2010) 2010:612089. doi: 10.1155/2010/612089
9. Buzzi M, Henderson RJ, Sargent JR. Biosynthesis of docosahexaenoic acid in trout hepatocytes proceeds via 24-carbon intermediates. Comp Biochem Physiol B Biochem Mol Biol. (1997) 116:263–7. doi: 10.1016/S0305-0491(96)00210-6
10. Leaver MJ, Bautista JM, Björnsson BT, Jönsson E, Krey G, Tocher DR et al. Towards fish lipid nutrigenomics: current state and prospects for fin-fish aquaculture. Rev Fish Sci. (2008) 16:73–94. doi: 10.1080/10641260802325278
11. Sibbons CM, Brenna JT, Lawrence P, Hoile SP, Clarke-Harris R, Lillycrop KA et al. Effect of sex hormones on n-3 polyunsaturated fatty acid biosynthesis in HepG2 cells and in human primary hepatocytes. Prostaglandins Leukot Essent Fatty Acids (2014) 90:47–54. doi: 10.1016/j.plefa.2013.12.006
12. Reardon HT, Hsieh AT, Park WJ, Kothapalli KSD, Anthony JC, Nathanielsz PW et al. Dietary long-chain polyunsaturated fatty acids upregulate expression of FADS3 transcripts. Prostaglandins Leukot Essent Fatty Acids (2013) 88:15–9. doi: 10.1016/j.plefa.2012.02.003
13. Park WJ, Kothapalli KSD, Lawrence P, Tyburczy C, Brenna JT. An alternate pathway to long-chain polyunsaturates: the FADS2 gene product Delta8-desaturates 20:2n-6 and 20:3n-3. J Lipid Res. (2009) 50:1195–202. doi: 10.1194/jlr.M800630-JLR200
14. Monroig Ó, Tocher DR, Hontoria F, Navarro JC. Functional characterisation of a Fads2 fatty acyl desaturase with Δ6/Δ8 activity and an Elovl5 with C16, C18 and C20 elongase activity in the anadromous teleost meagre (Argyrosomus regius). Aquaculture (2013) 412–413:14–22. doi: 10.1016/j.aquaculture.2013.06.032
15. Stroud CK, Nara TY, Roqueta-Rivera M, Radlowski EC, Lawrence P, Zhang Y et al. Disruption of FADS2 gene in mice impairs male reproduction and causes dermal and intestinal ulceration. J Lipid Res. (2009) 50:1870–80. doi: 10.1194/jlr.M900039-JLR200
16. Minghetti M, Leaver MJ, Tocher DR. Transcriptional control mechanisms of genes of lipid and fatty acid metabolism in the Atlantic salmon (Salmo salar L.) established cell line, SHK-1. Biochim Biophys Acta (2011) 1811:194–202. doi: 10.1016/j.bbalip.2010.12.008
17. Yoshikawa T, Ide T, Shimano H, Yahagi N, Amemiya-Kudo M, Matsuzaka T et al. Cross-talk between peroxisome proliferator-activated receptor (PPAR) alpha and liver X receptor (LXR) in nutritional regulation of fatty acid metabolism. I. PPARs suppress sterol regulatory element binding protein-1c promoter through inhibition of LXR signaling. Mol Endocrinol. (2003) 17:1240–54. doi: 10.1210/me.2002-0190
18. Baranowski M. Biological role of liver X receptors. J Physiol Pharmacol. (2008) 59(Suppl 7):31–55.
19. Xiaoping Z, Fajun Y. Regulation of SREBP-mediated gene expression. Sheng Wu Wu Li Hsueh Bao (2012) 28:287–94. doi: 10.3724/SP.J.1260.2012.20034
20. Jump DB, Tripathy S, Depner CM. Fatty acid-regulated transcription factors in the liver. Annu Rev Nutr. (2013) 33:249–69. doi: 10.1146/annurev-nutr-071812-161139
21. Nakamura MT, Nara TY. Gene regulation of mammalian desaturases. Biochem Soc Trans. (2002) 30:1076–9. doi: 10.1042/BST0301076
22. Garg ML, Clandinin MT. Alpha-linolenic acid and metabolism of cholesterol and long-chain fatty acids. Nutrition (1992) 8:208–10.
23. Menoyo D, López-Bote CJ, Obach A, Bautista JM. Effect of dietary fish oil substitution with linseed oil on the performance, tissue fatty acid profile, metabolism, and oxidative stability of Atlantic salmon. J Anim Sci. (2005) 83:2853–62. doi: 10.2527/2005.83122853x
24. Hector KL, Lagisz M, Nakagawa S. The effect of resveratrol on longevity across species: a meta-analysis. Biol Lett. (2012) 8:790–3. doi: 10.1098/rsbl.2012.0316
25. Bonnefont-Rousselot D. Resveratrol and cardiovascular diseases. Nutrients (2016) 8:8050250. doi: 10.3390/nu8050250
26. Mikulski D, Molski M. Quantitative structure-antioxidant activity relationship of trans-resveratrol oligomers, trans-4,4'-dihydroxystilbene dimer, trans-resveratrol-3-O-glucuronide, glucosides: trans-piceid, cis-piceid, trans-astringin and trans-resveratrol-4'-O-beta-D-glucopyranoside. Eur J Med Chem. (2010) 45:2366–80. doi: 10.1016/j.ejmech.2010.02.016
27. Pallauf K, Giller K, Huebbe P, Rimbach G. Nutrition and healthy ageing: calorie restriction or polyphenol-rich “mediterrasian” diet? Oxid Med Cell Longev. (2013) 2013:707421. doi: 10.1155/2013/707421
28. Takizawa Y, Nakata R, Fukuhara K, Yamashita H, Kubodera H, Inoue H. The 4'-hydroxyl group of resveratrol is functionally important for direct activation of PPARα. PLoS ONE (2015) 10:e0120865. doi: 10.1371/journal.pone.0120865
29. Gnoni GV, Paglialonga G. Resveratrol inhibits fatty acid and triacylglycerol synthesis in rat hepatocytes. Eur J Clin Invest. (2009) 39:211–8. doi: 10.1111/j.1365-2362.2008.02077.x
30. Momchilova A, Petkova D, Staneva G, Markovska T, Pankov R, Skrobanska R et al. Resveratrol alters the lipid composition, metabolism and peroxide level in senescent rat hepatocytes. Chem Biol Interact. (2014) 207:74–80. doi: 10.1016/j.cbi.2013.10.016
31. Torno C, Staats S, Pascual-Teresa SD, Rimbach G, Schulz C. Fatty acid profile is modulated by dietary resveratrol in rainbow trout (Oncorhynchus mykiss). Mar Drugs (2017) 15:15080252. doi: 10.3390/md15080252
32. Menoyo D, Kühn G, Ruiz-Lopez N, Pallauf K, Stubhaug I, Pastor JJ et al. Dietary resveratrol impairs body weight gain due to reduction of feed intake without affecting fatty acid composition in Atlantic salmon. Animal (2018) 114–115:1–8. doi: 10.1017/S1751731118000812
33. Bode LM, Bunzel D, Huch M, Cho G-S, Ruhland D, Bunzel M et al. In vivo and in vitro metabolism of trans-resveratrol by human gut microbiota. Am J Clin Nutr. (2013) 97:295–309. doi: 10.3945/ajcn.112.049379
34. Anisimova NY, Kiselevsky MV, Sosnov AV, Sadovnikov SV, Stankov IN, Gakh AA. Trans-, cis-, and dihydro-resveratrol: a comparative study. Chem Cent J. (2011) 5:88. doi: 10.1186/1752-153X-5-88
35. Azorín-Ortuño M, Yáñez-Gascón MJ, González-Sarrías A, Larrosa M, Vallejo F, Pallarés FJ et al. Effects of long-term consumption of low doses of resveratrol on diet-induced mild hypercholesterolemia in pigs: a transcriptomic approach to disease prevention. J Nutr Biochem. (2012) 23:829–37. doi: 10.1016/j.jnutbio.2011.04.007
36. Faragher RGA, Burton DGA, Majecha P, Fong NSY, Davis T, Sheerin A et al. Resveratrol, but not dihydroresveratrol, induces premature senescence in primary human fibroblasts. Age (2011) 33:555–64. doi: 10.1007/s11357-010-9201-5
37. Wagner AE, Huck G, Stiehl DP, Jelkmann W, Hellwig-Bürgel T. Dexamethasone impairs hypoxia-inducible factor-1 function. Biochem Biophys Res Commun. (2008) 372:336–40. doi: 10.1016/j.bbrc.2008.05.061
38. Borenfreund E, Puerner JA. A simple quantitative procedure using monolayer cultures for cytotoxicity assays (HTD/NR-90). J Tissue Cult Methods (1985) 9:7–9. doi: 10.1007/BF01666038
39. Spandidos A, Wang X, Wang H, Seed B. PrimerBank: a resource of human and mouse PCR primer pairs for gene expression detection and quantification. Nucleic Acids Res. (2010) 38:D792–9. doi: 10.1093/nar/gkp1005
40. Schloesser A, Campbell G, Glüer C-C, Rimbach G, Huebbe P. Restriction on an energy-dense diet improves markers of metabolic health and cellular aging in mice through decreasing hepatic mTOR activity. Rejuvenation Res. (2015) 18:30–9. doi: 10.1089/rej.2014.1630
41. Vauzour D, Tejera N, O'Neill C, Booz V, Jude B, Wolf IMA et al. Anthocyanins do not influence long-chain n-3 fatty acid status: studies in cells, rodents and humans. J Nutr Biochem. (2015) 26:211–8. doi: 10.1016/j.jnutbio.2014.09.005
44. Verbeke G, Molenberghs G. Linear Mixed Models for Longitudinal Data. New York, NY: Springer (2000).
45. Nakagawa S, Schielzeth H, O'Hara RB. A general and simple method for obtaining R2 from generalized linear mixed-effects models. Methods Ecol Evol. (2013) 4:133–42. doi: 10.1111/j.2041-210x.2012.00261.x
46. Bretz F, Westfall PH, Hothorn T. Multiple Comparisons Using R. Boca Raton, FL: Chapman and Hall/CRC (2010).
47. Bourassa P, Kanakis CD, Tarantilis P, Pollissiou MG, Tajmir-Riahi HA. Resveratrol, genistein, and curcumin bind bovine serum albumin. J Phys Chem B (2010) 114:3348–54. doi: 10.1021/jp9115996
48. Stivala LA, Savio M, Carafoli F, Perucca P, Bianchi L, Maga G et al. Specific structural determinants are responsible for the antioxidant activity and the cell cycle effects of resveratrol. J Biol Chem. (2001) 276:22586–94. doi: 10.1074/jbc.M101846200
49. Rao YPC, Lokesh BR. Modulatory effects of alpha-linolenic acid on generation of reactive oxygen species in elaidic acid enriched peritoneal macrophages in rats. Indian J Exp Biol. (2014) 52:860–9.
50. Cho HP, Nakamura MT, Clarke SD. Cloning, expression, and nutritional regulation of the mammalian Δ-6 desaturase. J Biol Chem. (1999) 274:471–7. doi: 10.1074/jbc.274.1.471
51. Sprecher H, Lee CJ. The absence of an 8-desaturases in rat liver: a reevaluation of optional pathways for the metabolism of linoleic and linolenic acids. Biochim Biophys Acta (1975) 388:113–25. doi: 10.1016/0005-2760(75)90067-3
52. Lopaschuk GD, Ussher JR, Folmes CDL, Jaswal JS, Stanley WC. Myocardial fatty acid metabolism in health and disease. Physiol Rev. (2010) 90:207–58. doi: 10.1152/physrev.00015.2009
53. Shimoda H, Tanaka J, Kikuchi M, Fukuda T, Ito H, Hatano T et al. Effect of polyphenol-rich extract from walnut on diet-induced hypertriglyceridemia in mice via enhancement of fatty acid oxidation in the liver. J Agric Food Chem. (2009) 57:1786–92. doi: 10.1021/jf803441c
54. Sheaff Greiner RC, Zhang Q, Goodman KJ, Giussani DA, Nathanielsz PW, Brenna JT. Linoleate, alpha-linolenate, and docosahexaenoate recycling into saturated and monounsaturated fatty acids is a major pathway in pregnant or lactating adults and fetal or infant rhesus monkeys. J Lipid Res. (1996) 37:2675–86.
55. Burdge GC, Wootton SA. Conversion of α-linolenic acid to palmitic, palmitoleic, stearic and oleic acids in men and women. Prostaglandins Leukot Essent Fatty Acids (2003) 69:283–90. doi: 10.1016/S0952-3278(03)00111-X
56. Smith S. The animal fatty acid synthase: One gene, one polypeptide, seven enzymes. FASEB J. (1994) 8:1248–59. doi: 10.1096/fasebj.8.15.8001737
57. Keys A, Anderson JT, Grande F. Serum cholesterol response to changes in the diet. Metabolism (1965) 14:776–87. doi: 10.1016/0026-0495(65)90004-1
58. Milanski M, Degasperi G, Coope A, Morari J, Denis R, Cintra DE et al. Saturated fatty acids produce an inflammatory response predominantly through the activation of TLR4 signaling in hypothalamus: implications for the pathogenesis of obesity. J Neurosci. (2009) 29:359–70. doi: 10.1523/JNEUROSCI.2760-08.2009
59. Bajracharya R, Bustamante S, Ballard JWO. Stearic acid supplementation in high protein to carbohydrate (P:C) ratio diet improves physiological and mitochondrial functions of Drosophila melanogaster parkin null mutants. J Gerontol A Biol Sci Med Sci. (2017). doi: 10.1093/gerona/glx246. [Epub ahead of print].
60. Shen M-C, Zhao X, Siegal GP, Desmond R, Hardy RW. Dietary stearic acid leads to a reduction of visceral adipose tissue in athymic nude mice. PLoS ONE (2014) 9:e104083. doi: 10.1371/journal.pone.0104083
61. Feldman EB, Russell BS, Schnare FH, Moretti-Rojas I, Miles BC, Doyle EA. Effects of diets of homogeneous saturated triglycerides on cholesterol balance in rats. J Nutr. (1979) 109:2237–46. doi: 10.1093/jn/109.12.2237
62. Perreault M, Roke K, Badawi A, Nielsen DE, Abdelmagid SA, El-Sohemy A et al. Plasma levels of 14:0, 16:0, 16:1n-7, and 20:3n-6 are positively associated, but 18:0 and 18:2n-6 are inversely associated with markers of inflammation in young healthy adults. Lipids (2014) 49:255–63. doi: 10.1007/s11745-013-3874-3
63. Samsami-Kor M, Daryani NE, Asl PR, Hekmatdoost A. Anti-inflammatory effects of resveratrol in patients with ulcerative colitis: a randomized, double-blind, placebo-controlled pilot study. Arch Med Res. (2015) 46:280–5. doi: 10.1016/j.arcmed.2015.05.005
64. Moussa C, Hebron M, Huang X, Ahn J, Rissman RA, Aisen PS et al. Resveratrol regulates neuro-inflammation and induces adaptive immunity in Alzheimer's disease. J Neuroinflamm. (2017) 14:1. doi: 10.1186/s12974-016-0779-0
65. Hunter JE, Zhang J, Kris-Etherton PM. Cardiovascular disease risk of dietary stearic acid compared with trans, other saturated, and unsaturated fatty acids: a systematic review. Am J Clin Nutr. (2010) 91:46–63. doi: 10.3945/ajcn.2009.27661
66. Di Renzo L, Marsella LT, Carraro A, Valente R, Gualtieri P, Gratteri S et al. Changes in LDL oxidative status and oxidative and inflammatory gene expression after red wine intake in healthy people: a randomized trial. Mediators Inflamm. (2015) 2015:317348. doi: 10.1155/2015/317348
67. Ali MHH, Messiha BAS, Abdel-Latif HA-T. Protective effect of ursodeoxycholic acid, resveratrol, and N-acetylcysteine on nonalcoholic fatty liver disease in rats. Pharm Biol. (2016) 54:1198–208. doi: 10.3109/13880209.2015.1060247
68. Jin X-J, Kim EJ, Oh IK, Kim YK, Park C-H, Chung JH. Prevention of UV-induced skin damages by 11,14,17-eicosatrienoic acid in hairless mice in vivo. J Korean Med Sci. (2010) 25:930–7. doi: 10.3346/jkms.2010.25.6.930
69. Chen S-J, Chuang L-T, and Chen S-N. Incorporation of eicosatrienoic acid exerts mild anti-inflammatory properties in murine RAW264.7 cells. Inflammation (2015) 38:534–45. doi: 10.1007/s10753-014-9960-8
70. Pamplona R, Portero-Otín M, Riba D, Ruiz C, Prat J, Bellmunt MJ et al. Mitochondrial membrane peroxidizability index is inversely related to maximum life span in mammals. J Lipid Res. (1998) 39:1989–94.
71. Inoue H, Jiang X-F, Katayama T, Osada S, Umesono K, Namura S. Brain protection by resveratrol and fenofibrate against stroke requires peroxisome proliferator-activated receptor α in mice. Neurosci Lett. (2003) 352:203–6. doi: 10.1016/j.neulet.2003.09.001
Keywords: resveratrol, HepG2 cells, omega-3, desaturase, stearic acid
Citation: Kühn G, Pallauf K, Schulz C, Birringer M, Diaz-Rica B, de Pascual-Teresa S and Rimbach G (2018) Resveratrol Modulates Desaturase Expression and Fatty Acid Composition of Cultured Hepatocytes. Front. Nutr. 5:106. doi: 10.3389/fnut.2018.00106
Received: 20 July 2018; Accepted: 23 October 2018;
Published: 14 November 2018.
Edited by:
Alessandra Durazzo, Consiglio per la ricerca in agricoltura e l'analisi dell'economia agraria (CREA), ItalyReviewed by:
Zhaojun Wei, Hefei University of Technology, ChinaArturo Anadón, Complutense University of Madrid, Spain
Ciprian Iliescu, National University of Singapore, Singapore
Copyright © 2018 Kühn, Pallauf, Schulz, Birringer, Diaz-Rica, de Pascual-Teresa and Rimbach. This is an open-access article distributed under the terms of the Creative Commons Attribution License (CC BY). The use, distribution or reproduction in other forums is permitted, provided the original author(s) and the copyright owner(s) are credited and that the original publication in this journal is cited, in accordance with accepted academic practice. No use, distribution or reproduction is permitted which does not comply with these terms.
*Correspondence: Gianna Kühn, a3VlaG5AZm9vZHNjaS51bmkta2llbC5kZQ==