- 1Intercollege Graduate Degree Program in Integrative and Biomedical Physiology, Huck Institutes of the Life Sciences, The Pennsylvania State University, University Park, PA, United States
- 2Department of Nutritional Sciences, The Pennsylvania State University, University Park, PA, United States
- 3Center for Molecular Immunology and Infectious Disease, Huck Institutes of the Life Sciences, The Pennsylvania State University, University Park, PA, United States
- 4Penn State Cancer Institute, Hershey, PA, United States
There is strong evidence that physical activity (PA) reduces risk, recurrence, and mortality from breast cancer. Emerging data suggest that PA induces changes in inflammatory and immune mediators that may contribute to beneficial effects on breast cancer outcomes. Thus, the goal of this review was to evaluate the evidence linking the protective benefit of PA to modulation of immune responses in breast cancer. A literature search was conducted to identify studies that evaluated the impact of PA on tumor and immune outcomes in breast cancer patients and in mammary tumor models. Nineteen studies investigated the effect of PA interventions on cancer immune outcomes using preclinical breast cancer models. Tumor growth was reduced in 11 studies, unchanged in three studies, and increased in one study. Spontaneous metastasis was reduced in two studies and survival was improved in four studies. Frequently assessed immune outcomes include splenic cell number and function, circulating inflammatory cytokines, and intratumoral immune cells and inflammatory markers. Circulating inflammatory cytokine responses were heterogeneous in preclinical models. Within the tumor microenvironment (TME), several studies documented a change in the infiltration of immune cells with an increase in effector cells and a reduction in immune suppressive cells. Twenty-three studies investigated the effect of PA interventions on immune outcomes in breast cancer patients. Thirteen studies used aerobic PA interventions and 10 studies used a combination of aerobic and resistance exercise interventions. Cycling and treadmill activities were the most commonly used PA modalities. Circulating immune cells and inflammatory cytokines were the most frequently assessed immune outcomes in the clinical studies. Among the 19 studies that evaluated a PA intervention during the post treatment period, 10 reported a reduction in the levels of at least one inflammatory cytokine. No inflammatory cytokines were quantified in the three studies that evaluated a PA intervention during treatment with chemotherapy. Immune outcomes within the tumor were assessed in only one study performing a PA intervention prior to surgery. Results from preclinical and clinical studies suggest that PA exerts heterogeneous effects on inflammatory cytokines, but may alter the gene expression profile and immune infiltrates in the tumor which may result in a reduction in immunosuppressive factors. However, additional studies are needed to better understand the effect of PA on immune outcomes in the TME.
Introduction
Breast cancer is the most commonly diagnosed cancer and leading cause of cancer death among women worldwide (1). Compelling observational data suggest that higher levels of physical activity are associated with reduced risk of pre- and post-menopausal breast cancer (2, 3). Achieving the World Health Organization's recommendation for leisure time physical activity (≥10 MET- or 2.5 hours/week of moderate-intensity activity) is associated with 12% risk reduction for breast cancer (RR = 0.88; 95% CI 0.84–0.91) (4).
Increased physical activity may reduce body mass and/or change body composition, which are also breast cancer risk factors (2). Thus, physical activity and body mass index (BMI) are interrelated, and their independent contribution to breast cancer risk has been difficult to determine. Several studies demonstrate that the protective effect of physical activity on breast cancer risk varies based on BMI status (5–7). However, a number of observational studies demonstrate a beneficial effect of physical activity independent of changes in BMI (8–11). In particular, one prospective cohort study (n = 19,196) demonstrates a negative association between post-menopausal breast cancer incidence and physical activity across all BMI categories, and a positive association between post-menopausal breast cancer incidence and BMI across all levels of physical activity, suggesting independent effects of these risk factors (12). In addition, the relationship between physical activity and breast cancer risk reduction appears to be independent of estrogen receptor status, adult weight gain, or postmenopausal hormone therapy (5, 8, 11). Furthermore, physical activity is associated with a significantly delayed onset of breast cancer among BRCA1 and BRCA2 mutation carriers (13).
In addition to a beneficial effect of physical activity on breast cancer risk, physical activity significantly lowers the risk of breast cancer recurrence, breast cancer-specific and all-cause mortality. A recent meta-analysis including over 45,000 breast cancer patients from 32 prospective cohort studies and four randomized controlled trials demonstrates a significantly lower risk of breast cancer recurrence (pooled hazard ratios range from 0.79 to 0.76) and a lower relative risk of all-cause mortality (pooled hazard ratios range from 0.75 to 0.52) among women with higher physical activity levels (14). In addition, a meta-analysis including 22 prospective cohort studies demonstrates that participants who reported high lifetime physical activity had a significantly lower risk of all-cause (HR = 0.82, 95% CI 0.70–0.96) and breast cancer-related death (HR = 0.73, 95% CI 0.54–0.98) compared to the least active women (15). Women with higher recent recreational pre-cancer diagnosis physical activity, post-diagnosis physical activity levels, or meeting the recommended physical activity guidelines post-diagnosis (≥8 MET-hours/week), also had a significantly lower risk of all-cause mortality (HR = 0.73, 95% CI 0.65–0.82; HR = 0.52, 95% CI 0.43–0.64; HR = 0.54, 95% CI 0.38–0.76, respectively) and breast cancer-related mortality (HR = 0.84, 95% CI 0.73–0.97; HR = 0.59, 95% CI 0.45–0.78; HR = 0.67, 95% CI 0.50–0.90, respectively) compared to the least active women (15). Lastly, high compared to low post-diagnosis physical activity is also associated with a reduction in risk of breast cancer-specific mortality in estrogen receptor-positive/progesterone receptor-positive (ER+/PR+), ER−/PR−, and triple-negative breast cancer (TNBC) patients (16). Combined, these studies demonstrate that physical activity is beneficial in reducing breast cancer risk and in improving clinical outcomes after a breast cancer diagnosis independent of a variety of host factors (e.g., BMI strata, receptor subtype and presence of genetic risk factors). These results suggest that either there are multiple biological mechanisms underlying the protective effect of physical activity on cancer outcomes and/or the underlying biological mechanism(s) are universal to women of varying genotype or phenotype. Numerous biological mediators of the cancer prevention effects of physical activity have been proposed and include changes in body composition, improvements in metabolic function, a reduction in estrogen availability, and changes in inflammatory and immune mediators (17–19). The metabolic and endocrine pathways altered by physical activity have been evaluated in numerous studies and these results are reviewed elsewhere (17, 18). However, fewer studies have evaluated the potential link between changes in immune and inflammatory mediators and the protective effects of physical activity on breast cancer outcomes, which is the focus of the current review.
The immune system is an important component of endogenous cancer prevention. Cells of the immune system can prevent tumor growth and progression through the recognition and destruction of transformed, tumorigenic cells. Several mechanisms contributing to spontaneous anti-tumor immunity have been characterized in humans and animals models (20, 21). CD8+ cytotoxic T cells (CTLs) and natural killer (NK) cells are primarily responsible for killing tumor cells, while CD4+ helper T cells can enhance their cytotoxic function by producing cytokines such as IFNγ (21, 22). Immunodeficiency can increase the risk of tumor development, and once established, tumors produce many immunosuppressive factors that can impair anti-tumor immune responses (20, 21). Immunosuppressive cells from both the myeloid and the lymphoid lineages are recruited in response to tumor-derived signals to facilitate immune escape, including myeloid-derived suppressor cells (MDSCs) and regulatory T cells (Tregs), respectively (21, 22). In addition, a wide variety of cytokines, chemokines and other immune modulatory molecules also play important roles in dictating the immune response systemically and in the tumor microenvironment (TME) (21, 22). In healthy animals and humans, chronic, aerobic physical activity enhances T cell and NK cell function and vaccine responses (23–25) and also reduces infection rates, chronic inflammation and age-related immunosenescence (26–30). However, less is known about the effect of physical activity on anti-tumor immunity. Emerging evidence suggests that physical activity may result in an increase in effector cell number and function, a reduction in immunosuppressive cells, and an altered cytokine profile in animal models of breast cancer (31–35). However, a definitive link between physical activity and improved cancer immunity is yet to be established.
To date, several reviews have summarized the effect of various types of physical activity (resistance, aerobic or the combination) on immune outcomes in patients of any cancer type (36, 37), and in breast cancer patients across the cancer continuum (38–40). However, no studies have comprehensively reviewed the evidence in animal models and breast cancer patients linking physical activity and immune modulation. Therefore, the objective of the current review was to evaluate and summarize both the preclinical and clinical evidence relating the protective benefit of chronic, aerobic physical activity on breast cancer outcomes to the modulation of immune responses, in both peripheral tissues/circulation and the TME.
Methods
Search Strategy
A systematic literature search was conducted using PubMed (Medline), Web of Science and CENTRAL to identify studies that evaluate the effect of physical activity (PA) interventions on tumor and immune outcomes in preclinical mammary tumor models and breast cancer patients. The search strategy included three components: (1) PA-related terms; (2) molecular and cellular components of the immune system and immunological processes important in cancer immunity; (3) breast cancer-related terms (complete search strategy provided in Supplementary Table 1). Supplemental hand searching was also conducted to identify additional studies from the reference lists of relevant review and primary research articles.
Inclusion and Exclusion Criteria
Only primary research articles written in the English language were included. This review aimed to evaluate the effect of chronic, aerobic PA interventions (such as running and swimming) in the context of breast cancer. Therefore, all observational studies and studies conducted in non-cancer animal or human subjects were excluded. Studies performing resistance exercise only, conditioning or stretching exercise (e.g., Tai Chi or yoga), or a single bout of exercise were also excluded. Because clinical studies frequently performed a combination of aerobic and resistance exercise interventions to prevent muscle wasting and cachexia in cancer patients, these studies were included. Preclinical and clinical studies that performed solely resistance exercise were excluded. Eligible preclinical studies must have reported the effect of PA interventions on tumor outcomes (tumor incidence, growth, metastasis, or survival). Clinical studies rarely investigated the effect of PA interventions on tumor outcomes, thus were not restricted by this criterion. Studies were included only if they assessed the effect of PA on immune outcomes, including immune cell number and/or function, cytokines, and other immune and inflammatory markers. Studies that used multiple interventions (such as those investigating the effect of PA and dietary supplements given alone and in combination) were included, as long as it was possible to compare groups receiving no intervention to PA intervention alone.
Study Selection and Quality Assessment
After de-duplication of the search results, a title/abstract screening was first performed on all records to identify potentially eligible studies, followed by a full-text screening to determine the eligibility of each study based on the inclusion and exclusion criteria. For preclinical studies, quality assessment was performed according to the SYstematic Review Center for Laboratory animal Experimentation's (SYRCLE's) risk of bias tool (41) (Supplementary Table 2). For clinical studies, quality assessment was performed according to National Institutes of Health Quality Assessment Tool for Controlled Intervention Studies, and for Before-After (Pre–Post) Studies With No Control Group (42) (Supplementary Table 3).
Data Extraction and Analysis
The following information was extracted from each eligible study for analysis: bibliographic information (author and publication year), animal model or human subject characteristics, PA intervention details, tumor outcomes and immune outcomes. Due to the heterogeneity in study design and immune outcomes assessed, data were synthesized in narrative form and a meta-analysis was not performed. However, a Preferred Reporting Items for Systematic Reviews and Meta-Analyses (PRISMA) flow diagram was created to describe the study selection process (43) (Supplementary Figure 1). Preclinical and clinical studies were summarized separately and were classified based on the timing of PA interventions in relation to tumor initiation or cancer treatment, respectively. For clinical studies, comparisons of immune outcomes between breast cancer patients performing PA intervention vs. sedentary control were recorded where possible. In studies using a single-group design where all participants performed PA interventions, comparisons between baseline and post-intervention values were recorded.
Results
Details regarding the study selection process are outlined in a PRISMA flow diagram (Supplementary Figure 1). In brief, 6,812 records were identified after duplicates were removed, and 6,577 were excluded following title and abstract screening. Of the 235 remaining studies, 42 met inclusion criteria and were included in the review. The 42 eligible studies included 19 preclinical and 23 clinical studies investigating the effect of PA interventions on immune outcomes in breast cancer. Results from preclinical and clinical studies were summarized separately below.
Preclinical Findings
Nineteen studies investigated the effect of PA interventions on cancer immune outcomes using preclinical breast cancer models (Table 1). Transplantable tumor models were used in 14 studies (31, 32, 34, 35, 44–50, 53–55), among which six used the 4T1 (or its subclone 4T1.2) TNBC murine mammary tumor model (32, 34, 35, 44, 48, 54). Carcinogen-induced (n = 3) (33, 56, 57) and genetically-engineered/spontaneous (n = 2) (51, 52) mammary tumor models were also used. Treadmill running (n = 11) was the most commonly used PA modality, followed by voluntary wheel running (n = 5), swimming (n = 3), and motorized wheel running (n = 2). Of these, two studies compared the effect of different PA modalities on tumor and immune outcomes (33, 46).
Included studies were highly heterogeneous in the frequency, duration and intensity of the PA intervention, as well as in the length and timeline of the PA intervention period. To evaluate if PA performed before or after tumor initiation may result in different tumor and immune outcomes, we classified the included studies into three categories: PA intervention prior to (n = 2) (32, 44), from pre to post (n = 10, including the spontaneous tumor models) (31, 34, 45–52), and post tumor initiation (n = 7) (33, 35, 53–57). Despite the heterogeneity in study designs, most studies reported positive effects of PA on tumor outcomes. Tumor incidence and/or multiplicity was reduced in three studies (33, 51, 56) and unchanged in two studies (50, 57). Tumor growth was reduced in 11 studies (31, 32, 34, 35, 44, 45, 49, 51–54), unchanged in three studies (48, 50, 55), and increased in one study (57). Spontaneous metastasis was reduced in two studies (34, 55) and unchanged in one study (35), and one additional study reported no change in experimental lung metastasis (46). Survival was improved in all four studies reporting cancer survival outcomes (31, 32, 34, 47).
Frequently assessed immune outcomes include splenic cell number and function, circulating inflammatory cytokines, and intratumoral immune cells and inflammatory markers. Among splenic effector cells, one study reported no change in the percentage of total T or NK cells, but an increase in proliferating (Ki-67+) and activated (CD69+) NK cells (35). Three other studies reported an increase in the number of NK cells (44) and a polarization of Th1/Th2 balance toward Th1 response (48, 54), respectively. Increased splenic CD4+ T cell proliferation [by PA plus energy restriction (ER)] (34), NK cell cytotoxicity (48) and lymphokine activated killer (LAK) activity (46) were each reported in one study. Among splenic immunosuppressive cells, one study reported a reduction in the number of MDSCs and no change in the number of Tregs (by PA+ER) (34), and two other studies reported a reduction in either the percentage or number of MDSCs (35) and Tregs (54), respectively.
Circulating inflammatory cytokine responses were heterogeneous in preclinical models. TNFα was reduced in one study (33) and increased in another study (31). IL-1(β) was reduced in one study (33) and increased in another study (31). MCP-1 was reduced in one study (51) and unchanged in another study (55). IL-6 was reduced (51), increased (33), or unchanged (56) in three individual studies. CRP was unchanged in two studies (33, 56). Fewer studies have evaluated circulating cytokines related to effector functions. Of those, IL-2 was increased in one study (33) and unchanged in another study (31), and IFNγ was increased in one study (33).
Within the TME, two studies reported an increase in the percentage of infiltrating CD4+ and CD8+ T cells (34) or activated (CD8+/CD69+) T cells (35), respectively. One study reported an increase in the percentage of tumor-infiltrating NK cells (44). Yet three studies reported no change in the percentage or number of CD4+ or CD8+ T cells (32, 54, 55). In addition, two studies assessed immune outcomes in the non-tumor-draining (mesenteric) lymph nodes and found an increase in lymphocyte proliferation (31, 47). Among the immune suppressive cells, the percentage of MDSCs was reduced in two studies (34, 35), and Treg number or Foxp3 gene expression were reduced in two studies (32, 34). Additionally, two studies reported a reduction in the percentage or number of tumor-infiltrating macrophages (49, 54), while a third study reported an increase in macrophage phagocytic activity (50). Intratumoral inflammatory cytokines were also assessed in several studies. One study reported no change in TNFα, IL-6, or IFNγ within the tumor (48), while three other studies reported a reduction in IL-6 (45, 53) or Ifng gene expression (34), respectively. Of note, one study evaluated the gene expression profile within the TME (34), and found global downregulation of genes important in metastasis, immune checkpoint molecules, and chemokines associated with MDSC and Treg recruitment (by PA alone or in combination with ER).
Clinical Findings
Twenty-three studies investigated the effect of PA interventions on immune outcomes in breast cancer patients (Table 2). Thirteen studies used solely aerobic PA interventions and 10 studies used a combination of aerobic and resistance exercise interventions. Cycling and treadmill activities were the most commonly used aerobic PA modalities. Similar to preclinical studies, the frequency, duration, intensity and timeline of the PA interventions were highly heterogeneous in the clinical studies. To evaluate if the timing of the PA intervention in relation to cancer treatment may significantly impact immune outcomes, we classified the included studies into three categories: PA intervention performed prior to (n = 1) (58), during (n = 3) (39, 59, 60), and post treatment (n = 19) (61–79).
Circulating immune cells and inflammatory cytokines were the most frequently assessed immune outcomes in the clinical studies. In the 19 studies that evaluated a PA intervention during the post treatment period, 10 reported a reduction in at least one inflammatory cytokine including IL-6, IL-8, CRP, MIF, TNFα, and HMGB-1 (61, 62, 65, 66, 68, 70, 73, 74, 78, 79). However, no study measured all of these inflammatory mediators and some studies found no effect of the PA intervention on one or more of these cytokines. Two studies found an increase in NK cell cytotoxicity (63, 69) and mitogen-induced lymphocyte proliferation (67, 69) and one study reported an increase in monocyte phagocytosis (64). Four studies quantified immune cell populations in circulation (63, 64, 67, 76), but only one reported an increase in the percentage of CD4+/CD69+ cells (67).
No inflammatory cytokines were quantified in the three studies that evaluated a PA intervention during treatment with chemotherapy. Circulating immune cell populations including T cells, B cells, NK cells, total lymphocytes, neutrophils and monocytes were measured in all three studies and PA had no effect on the distribution of immune cells.
Immune outcomes within the tumor were assessed in only one study performing a PA intervention prior to treatment (58). In this study, one month of PA intervention prior to surgery resulted in the upregulation of multiple immune and inflammatory pathways within the TME, including cytokine-cytokine receptor interactions, NK-mediated cytotoxicity, T-cell receptor signaling, antigen processing and presentation. No change was found in tumor-infiltrating CD4+ or CD8+ T cells or macrophages, while a trend toward a reduction in tumor-infiltrating Tregs was observed.
Discussion
Gaining a better understanding of the biological mediators underlying the beneficial effect of physical activity on breast cancer outcomes is needed in order to make clearer recommendations regarding the dose, duration, and frequency of physical activity needed to achieve the beneficial effects observed to date, and to make evidence-based decisions about possible synergies between physical activity prescriptions and standard and emerging therapeutic strategies. Numerous studies have explored the biological mechanisms linking physical activity and improved breast cancer outcomes, including those related to inflammatory and immune responses. Thus, the goal of this review was to summarize the results from studies that have examined physical activity interventions and immune outcomes in animal models and women with breast cancer, in order to make conclusions regarding the role of physical activity on immune modulation in breast cancer.
Strong epidemiological evidence suggests that physical activity is associated with reduced breast cancer risk (2–4). Consistent with data collected in observational studies, 13 out of the 15 preclinical studies that assessed primary tumor growth demonstrated that physical activity reduces mammary tumor growth or volume. This physical activity-induced reduction in tumor growth occurred regardless of whether the physical activity interventions occurred prior to, from pre to post, and only post tumor initiation. Tumor incidence and/or multiplicity were most commonly assessed in spontaneous and carcinogen-induced breast cancer models. Among these studies, tumor incidence was reduced in one study (33), while tumor multiplicity was reduced in three studies (33, 51, 56) and unchanged in another study (57). In the two studies that utilized PA interventions of the shortest duration (16–17 days), no change in tumor incidence and/or growth was observed (50, 55). These data suggest that longer exposure to regular bouts of physical activity may be needed to achieve a beneficial effect on tumor outcomes.
Strong evidence from observational and randomized controlled trials also suggests that physical activity is associated with reduced breast cancer recurrence and cancer-specific and all-cause mortality (14–16). Metastasis contributes significantly to the mortality of breast cancer patients (80). Events in the metastatic pathway include the release of cancer cells from the primary tumor; invasion of surrounding tissue including stroma, lymphatics, and/or blood vessels (as circulating tumor cells, or CTCs); and colonization of target organs as disseminated tumor cells (DTCs) (81). DTCs may undergo cell death via recognition by immune effector cells; initiate rapid cell proliferation; or enter a state of tumor dormancy. Dormant cells have been proposed to either exist as single cells in cell cycle arrest (82), or to be actively dividing, but with restricted proliferation due to either lack of angiogenesis (83) or immune surveillance (84, 85). Metastatic dormancy is widespread in breast cancer, although the molecular basis is not completely known. Metastatic burden was evaluated in four preclinical studies where physical activity was performed from pre to post, or post tumor initiation. Two studies found a reduction in spontaneous metastasis to the lung, femur or abdominal cavity (34, 55), while two others found no change in spontaneous or experimental lung metastasis, respectively (35, 46). In addition, four studies reported an improvement in survival in physically active animals (PA alone or in combination with ER) (31, 32, 34, 47). In all four studies, mice or rats were exposed to eight weeks of a PA intervention prior to tumor inoculation, but were removed from the PA intervention at varying intervals post tumor inoculation (e.g., at the time of, or two or five weeks after tumor inoculation). These results suggest that PA prior to tumor development, at least in the mammary tumor models studied to date, may be important in reducing metastatic burden and preventing tumor-related death. Thus, it is plausible that physical activity may be reducing recurrence and increasing survival in breast cancer patients by suppressing metastases to distant sites. However, additional studies are needed to confirm these findings and to explore the biological mechanisms contributing to a PA-induced reduction in metastases and mortality in mammary tumor models.
Both preclinical and clinical studies that have explored the effect of PA on cancer immune outcomes report highly variable results. A wide range of immunological parameters has been assessed, yet each parameter is only assessed in a small number of studies with mixed results. Due to limitations in the accessibility of human samples, peripheral blood is often the only tissue compartment from which immune cells or inflammatory mediators are assessed in clinical studies. Over half (53%) of the studies evaluating a PA intervention in the post treatment period reported a reduction in at least one inflammatory cytokine (IL-6, IL-8, CRP, MIF, TNFα, and HMGB-1) in plasma or serum (61, 62, 65, 66, 68, 70, 73, 74, 78, 79). However, no study measured all of these inflammatory mediators in the same patients and some studies found no effect of the PA intervention on one or more of these cytokines within the same study. Preclinical studies that evaluated circulating inflammatory markers reported mixed results. This heterogeneity in the response of inflammatory mediators in preclinical models may be attributed to differences in the timing of sample collection with respect to the last bout of activity, the use of different mammary tumor models that may have different cytokine profiles, and/or the presence of growing tumors that can be a direct source of inflammatory cytokines. Cytokines and chemokines play a critical role in cancer-related inflammation, regulating both host and malignant cells in the tumor microenvironment (86). Furthermore, metastasis is driven by inflammatory signals and the infiltration of inflammatory cells into the primary tumor (87). PA can reduce inflammatory mediators associated with obesity and other chronic diseases (88, 89) in the absence of tumor. Thus, evaluating the cytokine milieu in response to physical activity interventions in both preclinical models and in breast cancer patients is an important outcome to pursue in future studies to determine if changes in inflammatory mediators correlate with clinical outcomes.
In addition to circulating factors, preclinical studies often assess immune outcomes in the spleen and tumor microenvironment. Emerging evidence suggests that PA may induce beneficial changes in splenic immunity, represented by an increase in T cell proliferation and Th1 cytokine production (34, 48, 54), NK cell number and cytotoxicity (44, 46), and a reduction in the immunosuppressive populations induced by tumors including MDSCs and Tregs (34, 35, 54). Within the TME, there is a similar trend of increased effector cells (CD4+ and CD8+ T cells and NK cells) and a reduction in pro-tumor immune suppressors (MDSCs and Tregs) (32, 34, 35, 44) in animals exposed to PA interventions. In a study using the 4T1.2 murine mammary tumor model (34), PA alone or in combination with ER induced global changes in the gene expression profile within the TME, featured by a downregulation of chemokines important in MDSC and Treg recruitment (CCL5, CCL20, CCL22), immune checkpoint (programmed cell death protein 1, PD-1) and other inhibitory molecules (indoleamine 2,3-dioxygenase, IDO). Consistent with this, a clinical study performing a pre-surgical PA intervention (58) reported an upregulation in multiple pathways within the TME, including those involved in effector cell function and inflammatory signaling. These findings suggest that PA may be preventing the escalation of immunosuppression while promoting anti-tumor immune response within the TME, which may improve responses to both chemotherapy and immunotherapy.
Overall, it has been hypothesized that the modulation of the immune system is a candidate for the development of more effective therapies in breast cancer. However, breast cancer is a heterogeneous disease, with HER2+ and TNBC being more immunogenic compared to hormone receptor (HR)-positive subtypes (90–92). HER2+ and TNBC are more likely to be infiltrated by tumor-infiltrating lymphocytes (TILs) and to express programmed death ligand-1 (PD-L1) in the TME than ER- or PR-expressing luminal tumors (93–95). Moreover, the quantity of TILs is a prognostic indicator of disease recurrence-free survival and overall survival, especially for TNBC (96–98). Higher TIL concentration is also predictive of response to neoadjuvant chemotherapy in all subtypes of breast cancer (99). The clinical success of immune checkpoint inhibitors (ICI) in several other cancer types has established immunotherapy as a fundamental pillar of cancer treatment (100). However, recent clinical trials in breast cancer immunotherapy utilizing ICIs have yielded mixed results (90, 101). Initial phase I/II trials using PD-1/PD-L1 inhibitors as single-agent therapy report an overall response rate (ORR) under 10% in unselected populations (102–104). However, higher ORRs (12–24%) are observed in several trials in which enriched subgroups of TNBC patients were involved, including PD-L1+ patients with high TIL level, and when the PD-1/PD-L1 inhibitor was administered as an earlier line of therapy (90, 103, 105). In an effort to improve response rates and overcome acquired resistance, various combination strategies have been developed to explore the potential synergy between ICI and radiation, chemotherapy, targeted therapy, and other immunotherapies (90, 101, 106). This combinatorial approach has led to improved clinical outcomes and FDA approval for the use of a PD-L1 inhibitor (atezolizumab) in combination with paclitaxel protein-bound for patients with unresectable locally advanced or metastatic TNBC whose tumors express PD-L1 (107). Given the potential for physical activity to modulate both systemic immunity and immune infiltration in the TME, physical activity may be a possible strategy to use in combination with chemotherapy and emerging immunotherapies. To date, no clinical trials have explored the effect of physical activity on immunotherapy outcomes. However, a preclinical study using the 4T1 murine breast cancer model demonstrates that PA enhances the anti-tumor effect of a combination of PD-1 blockade and focal radiotherapy (35). The improved therapeutic response was associated with reduced PD-1 expression by splenic CD8+ T cells, splenic and tumor-infiltrating NK cells, and a reduction in tumor-infiltrating MDSCs. Despite the limited amount of evidence, emerging data suggest that PA may be altering the TME, which could enhance the efficacy of immunotherapies. However, additional studies are needed to explore the use of ICI alone or in combination with other standard therapies, with and without physical activity, to determine if any additive or synergistic effects are observed.
The current review has several limitations. There is much heterogeneity in the prescription of PA interventions (modality, frequency, duration, intensity, and timing), as well as the preclinical cancer models used and characteristics of human participants. Additionally, many of the clinical studies are exploratory trials with immune outcomes assessed in a small sample size. Thus, limited definitive conclusions can be made on the potential effect of PA on cancer immune outcomes.
In summary, existing preclinical studies consistently demonstrate a beneficial effect of PA on breast cancer outcomes, with the most abundant evidence on reducing primary mammary tumor growth. Emerging preclinical evidence also suggests a potential role of PA in reducing tumor incidence, metastasis and improving survival. However, exploration on the potential immunological mechanisms underlying such beneficial effect is still in its early phase. Findings from clinical studies suggest that physical activity may have an impact on circulating inflammatory cytokines. However, additional studies are warranted to better understand which cytokines might be impacted by activity and if these changes correlate with clinical outcomes. Physical activity may modulate immune responses in circulation resulting in enhanced immune surveillance, and/or alter the immune landscape within the TME leading to greater infiltration of effector cells and a reduction in immune suppression (Figure 1). Additional mechanistic studies are needed to determine the potential immune mediators contributing to physical activity-induced improvement in tumor outcomes, particularly within the TME, and if a causal relationship exists. Well-designed clinical trials are also warranted to confirm if immunological mechanisms underlie the cancer prevention effect of physical activity, as well as to evaluate if physical activity interventions may be used in combination with standard and emerging immunotherapies to improve clinical outcomes in breast cancer patients.
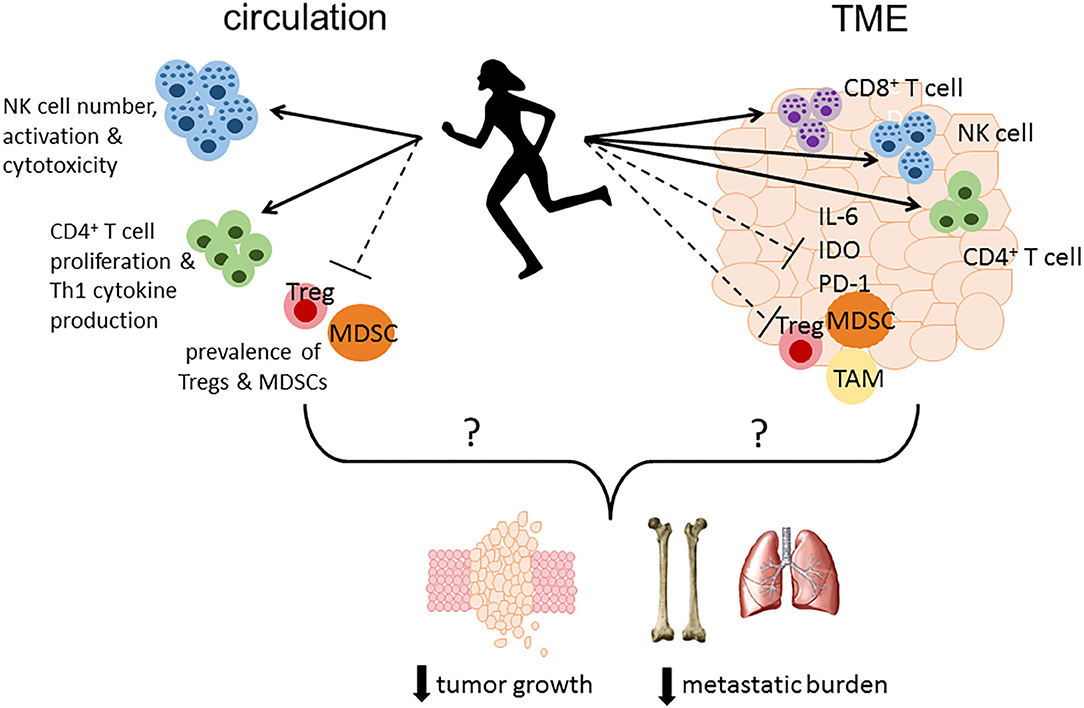
Figure 1. Proposed immune mechanisms altered by physical activity that may play a role in reducing tumor growth, metastatic burden and mortality. Physical activity may modulate immune responses in circulation resulting in enhanced immune surveillance by increasing the number, activation status, and cytotoxicity of NK cells, enhancing CD4+ T cell proliferation and Th1 cytokine production, and reducing the number or percentage of immunosuppressive MDSCs and Tregs. Furthermore, physical activity may alter the immune landscape within the TME leading to greater infiltration of effector cells (CD4+ and CD8+ T cells, NK cells) concurrent with a reduction in immunosuppressive factors including IL-6, PD-1, and IDO, and less accumulation of MDSCs, TAMs, and Tregs. TME, tumor microenvironment; IL-6, interleukin-6; PD-1, programmed cell death protein 1; IDO, indoleamine 2,3-dioxygenase; MDSC, myeloid-derived suppressor cell; TAM, tumor-associated macrophage; Treg, regulatory T cell.
Author Contributions
CR and YX participated in the conception and design of the work, collection and assembly of data, data analysis and interpretation, and manuscript writing. All authors approved the final version for submission.
Funding
YX was supported by a T32 Training Program (T32GM108563) Research training in Physiological Adaptations to Stress while at The Pennsylvania State University.
Conflict of Interest
The authors declare that the research was conducted in the absence of any commercial or financial relationships that could be construed as a potential conflict of interest.
Supplementary Material
The Supplementary Material for this article can be found online at: https://www.frontiersin.org/articles/10.3389/fnut.2020.557997/full#supplementary-material
References
2. Chan DSM, Abar L, Cariolou M, Nanu N, Greenwood DC, Bandera EV, et al. World Cancer Research Fund International: Continuous Update Project-systematic literature review and meta-analysis of observational cohort studies on physical activity, sedentary behavior, adiposity, and weight change and breast cancer risk. Cancer Causes Control. (2019) 30:1183–200. doi: 10.1007/s10552-019-01223-w
3. Rezende LFM, Sa TH, Markozannes G, Rey-Lopez JP, Lee IM, Tsilidis KK, et al. Physical activity and cancer: an umbrella review of the literature including 22 major anatomical sites and 770 000 cancer cases. Br J Sports Med. (2018) 52:826–33. doi: 10.1136/bjsports-2017-098391
4. Liu L, Shi Y, Li T, Qin Q, Yin J, Pang S, et al. Leisure time physical activity and cancer risk: evaluation of the WHO's recommendation based on 126 high-quality epidemiological studies. Br J Sports Med. (2016) 50:372–8. doi: 10.1136/bjsports-2015-094728
5. Leitzmann MF, Moore SC, Peters TM, Lacey JV Jr, Schatzkin A, Schairer C, et al. Prospective study of physical activity and risk of postmenopausal breast cancer. Breast Cancer Res. (2008) 10:R92. doi: 10.1186/bcr2190
6. Maruti SS, Willett WC, Feskanich D, Rosner B, Colditz GA. A prospective study of age-specific physical activity and premenopausal breast cancer. J Natl Cancer Inst. (2008) 100:728–37. doi: 10.1093/jnci/djn135
7. McTiernan A, Kooperberg C, White E, Wilcox S, Coates R, Adams-Campbell LL, et al. Recreational physical activity and the risk of breast cancer in postmenopausal women: the Women's Health Initiative Cohort Study. JAMA. (2003) 290:1331–6. doi: 10.1001/jama.290.10.1331
8. Breslow RA, Ballard-Barbash R, Munoz K, Graubard BI. Long-term recreational physical activity and breast cancer in the National Health and Nutrition Examination Survey I epidemiologic follow-up study. Cancer Epidemiol Biomarkers Prev. (2001) 10:805–8. Available online at: https://cebp.aacrjournals.org/content/10/7/805.long
9. Moore SC, Lee IM, Weiderpass E, Campbell PT, Sampson JN, Kitahara CM, et al. Association of leisure-time physical activity with risk of 26 types of cancer in 1.44 million adults. JAMA Intern Med. (2016) 176:816–25. doi: 10.1001/jamainternmed.2016.1548
10. Sesso HD, Paffenbarger RS Jr, Lee IM. Physical activity and breast cancer risk in the College Alumni Health Study (United States). Cancer Causes Control. (1998) 9:433–9. doi: 10.1023/a:1008827903302
11. Hildebrand JS, Gapstur SM, Campbell PT, Gaudet MM, Patel AV. Recreational physical activity and leisure-time sitting in relation to postmenopausal breast cancer risk. Cancer Epidemiol Biomarkers Prev. (2013) 22:1906–12. doi: 10.1158/1055-9965.EPI-13-0407
12. Bellocco R, Marrone G, Ye W, Nyren O, Adami HO, Mariosa D, et al. A prospective cohort study of the combined effects of physical activity and anthropometric measures on the risk of post-menopausal breast cancer. Eur J Epidemiol. (2016) 31:395–404. doi: 10.1007/s10654-015-0064-z
13. King MC, Marks JH, Mandell JB. New York Breast Cancer Study G. Breast and ovarian cancer risks due to inherited mutations in BRCA1 and BRCA2. Science. (2003) 302:643–6. doi: 10.1126/science.1088759
14. Cormie P, Zopf EM, Zhang X, Schmitz KH. The impact of exercise on cancer mortality, recurrence, and treatment-related adverse effects. Epidemiol Rev. (2017) 39:71–92. doi: 10.1093/epirev/mxx007
15. Lahart IM, Metsios GS, Nevill AM, Carmichael AR. Physical activity, risk of death and recurrence in breast cancer survivors: a systematic review and meta-analysis of epidemiological studies. Acta Oncol. (2015) 54:635–54. doi: 10.3109/0284186X.2014.998275
16. Friedenreich CM, Neilson HK, Farris MS, Courneya KS. Physical activity and cancer outcomes: a precision medicine approach. Clin Cancer Res. (2016) 22:4766–75. doi: 10.1158/1078-0432.CCR-16-0067
17. Friedenreich CM. Physical activity and breast cancer: review of the epidemiologic evidence and biologic mechanisms. Recent Results Cancer Res. (2011) 188:125–39. doi: 10.1007/978-3-642-10858-7_11
18. McTiernan A. Mechanisms linking physical activity with cancer. Nat Rev Cancer. (2008) 8:205–11. doi: 10.1038/nrc2325
19. Neilson HK, Friedenreich CM, Brockton NT, Millikan RC. Physical activity and postmenopausal breast cancer: proposed biologic mechanisms and areas for future research. Cancer Epidemiol Biomarkers Prev. (2009) 18:11–27. doi: 10.1158/1055-9965.EPI-08-0756
20. Dunn GP, Old LJ, Schreiber RD. The three Es of cancer immunoediting. Annu Rev Immunol. (2004) 22:329–60. doi: 10.1146/annurev.immunol.22.012703.104803
21. Swann JB, Smyth MJ. Immune surveillance of tumors. J Clin Invest. (2007) 117:1137–46. doi: 10.1172/JCI31405
22. Ostrand-Rosenberg S. Immune surveillance: a balance between protumor and antitumor immunity. Curr Opin Genet Dev. (2008) 18:11–8. doi: 10.1016/j.gde.2007.12.007
23. Rogers CJ, Berrigan D, Zaharoff DA, Hance KW, Patel AC, Perkins SN, et al. Energy restriction and exercise differentially enhance components of systemic and mucosal immunity in mice. J Nutr. (2008) 138:115–22. doi: 10.1093/jn/138.1.115
24. Rogers CJ, Zaharoff DA, Hance KW, Perkins SN, Hursting SD, Schlom J, et al. Exercise enhances vaccine-induced antigen-specific T cell responses. Vaccine. (2008) 26:5407–15. doi: 10.1016/j.vaccine.2008.07.081
25. Simpson RJ, Kunz H, Agha N, Graff R. Exercise and the Regulation of Immune Functions. Prog Mol Biol Transl Sci. (2015) 135:355–80. doi: 10.1016/bs.pmbts.2015.08.001
26. Nicklas BJ, Brinkley TE. Exercise training as a treatment for chronic inflammation in the elderly. Exerc Sport Sci Rev. (2009) 37:165–70. doi: 10.1097/JES.0b013e3181b7b3d9
27. Woods JA, Ceddia MA, Wolters BW, Evans JK, Lu Q, McAuley E. Effects of 6 months of moderate aerobic exercise training on immune function in the elderly. Mech Ageing Dev. (1999) 109:1–19. doi: 10.1016/s0047-6374(99)00014-7
28. Nieman DC, Wentz LM. The compelling link between physical activity and the body's defense system. J Sport Health Sci. (2019) 8:201–17. doi: 10.1016/j.jshs.2018.09.009
29. Bartlett DB, Duggal NA. Moderate physical activity associated with a higher naive/memory T-cell ratio in healthy old individuals: potential role of IL15. Age Ageing. (2020) 49:368–73. doi: 10.1093/ageing/afaa035
30. Turner JE, Brum PC. Does regular exercise counter T cell immunosenescence reducing the risk of developing cancer and promoting successful treatment of malignancies? Oxid Med Cell Longev. (2017) 2017:4234765. doi: 10.1155/2017/4234765
31. Bacurau AV, Belmonte MA, Navarro F, Moraes MR, Pontes FL Jr, Pesquero JL, et al. Effect of a high-intensity exercise training on the metabolism and function of macrophages and lymphocytes of walker 256 tumor bearing rats. Exp Biol Med. (2007) 232:1289–99. doi: 10.3181/0704-rm-93
32. Hagar A, Wang Z, Koyama S, Serrano JA, Melo L, Vargas S, et al. Endurance training slows breast tumor growth in mice by suppressing Treg cells recruitment to tumors. BMC Cancer. (2019) 19:536. doi: 10.1186/s12885-019-5745-7
33. Thompson HJ, Wolfe P, McTiernan A, Jiang W, Zhu Z. Wheel running-induced changes in plasma biomarkers and carcinogenic response in the 1-methyl-1-nitrosourea-induced rat model for breast cancer. Cancer Prev Res. (2010) 3:1484–92. doi: 10.1158/1940-6207.capr-10-0078
34. Turbitt WJ, Xu Y, Sosnoski DM, Collins SD, Meng H, Mastro AM, et al. Physical activity plus energy restriction prevents 4T1.2 mammary tumor progression, MDSC accumulation, and an immunosuppressive tumor microenvironment. Cancer Prev Res. (2019) 12:493–506. doi: 10.1158/1940-6207.capr-17-0233
35. Wennerberg E, Lhuillier C, Rybstein MD, Dannenberg K, Rudqvist NP, Koelwyn GJ, et al. Exercise reduces immune suppression and breast cancer progression in a preclinical model. Oncotarget. (2020) 11:452–61. doi: 10.18632/oncotarget.27464
36. Koelwyn GJ, Quail DF, Zhang X, White RM, Jones LW. Exercise-dependent regulation of the tumour microenvironment. Nat Rev Cancer. (2017) 17:620–32. doi: 10.1038/nrc.2017.78
37. Khosravi N, Stoner L, Farajivafa V, Hanson ED. Exercise training, circulating cytokine levels and immune function in cancer survivors: a meta-analysis. Brain Behav Immun. (2019) 81:92–104. doi: 10.1016/j.bbi.2019.08.187
38. Schmidt T, van Mackelenbergh M, Wesch D, Mundhenke C. Physical activity influences the immune system of breast cancer patients. J Cancer Res Ther. (2017) 13:392–8. doi: 10.4103/0973-1482.150356
39. Schmidt T, Jonat W, Wesch D, Oberg HH, Adam-Klages S, Keller L, et al. Influence of physical activity on the immune system in breast cancer patients during chemotherapy. J Cancer Res Clin Oncol. (2018) 144:579–86. doi: 10.1007/s00432-017-2573-5
40. de Jesus Leite MAF, Puga GM, Arantes FJ, Oliveira CJF, Cunha LM, Bortolini MJS, et al. Effects of combined and resistance training on the inflammatory profile in breast cancer survivors: a systematic review. Complement Ther Med. (2018) 36:73–81. doi: 10.1016/j.ctim.2017.11.023
41. Hooijmans CR, Rovers MM, de Vries RB, Leenaars M, Ritskes-Hoitinga M, Langendam MW. SYRCLE's risk of bias tool for animal studies. BMC Med Res Methodol. (2014) 14:43. doi: 10.1186/1471-2288-14-43
42. National Heart, Lung, and Blood Institute. Study Quality Assessment Tools. (2019). Available online at: https://www.nhlbi.nih.gov/health-topics/study-quality-assessment-tools
43. Liberati A, Altman DG, Tetzlaff J, Mulrow C, Gotzsche PC, Ioannidis JP, et al. The PRISMA statement for reporting systematic reviews and meta-analyses of studies that evaluate health care interventions: explanation and elaboration. J Clin Epidemiol. (2009) 62:e1–34. doi: 10.1016/j.jclinepi.2009.06.006
44. Wang B, Xu H, Hu X, Ma W, Zhang J, Li Y, et al. Synergetic inhibition of daidzein and regular exercise on breast cancer in bearing-4T1 mice by regulating NK cells and apoptosis pathway. Life Sci. (2020) 245:117387. doi: 10.1016/j.lfs.2020.117387
45. Shalamzari SA, Agha-Alinejad H, Alizadeh S, Shahbazi S, Khatib ZK, Kazemi A, et al. The effect of exercise training on the level of tissue IL-6 and vascular endothelial growth factor in breast cancer bearing mice. Iranian J Basic Med Sci. (2014) 17:231–58.
46. Hoffman-Goetz L, May KM, Arumugam Y. Exercise training and mouse mammary tumour metastasis. Anticancer Res. (1994) 14(6b):2627–31.
47. Bacurau RF, Belmonte MA, Seelaender MC, Costa Rosa LF. Effect of a moderate intensity exercise training protocol on the metabolism of macrophages and lymphocytes of tumour-bearing rats. Cell Biochem Funct. (2000) 18:249–58. doi: 10.1002/1099-0844(200012)18:4<249::aid-cbf879>3.0.co;2-2
48. Molanouri Shamsi M, Chekachak S, Soudi S, Gharakhanlou R, Quinn LS, Ranjbar K, et al. Effects of exercise training and supplementation with selenium nanoparticle on T-helper 1 and 2 and cytokine levels in tumor tissue of mice bearing the 4 T1 mammary carcinoma. Nutrition. (2019) 57:141–7. doi: 10.1016/j.nut.2018.05.022
49. Almeida PW, Gomes-Filho A, Ferreira AJ, Rodrigues CE, Dias-Peixoto MF, Russo RC, et al. Swim training suppresses tumor growth in mice. J Appl Physiol. (2009) 107:261–5. doi: 10.1152/japplphysiol.00249.2009
50. Woods JA, Davis JM, Kohut ML, Ghaffar A, Mayer EP, Pate RR. Effects of exercise on the immune response to cancer. Med Sci Sports Exerc. (1994) 26:1109–15.
51. Murphy EA, Davis JM, Barrilleaux TL, McClellan JL, Steiner JL, Carmichael MD, et al. Benefits of exercise training on breast cancer progression and inflammation in C3(1)SV40Tag mice. Cytokine. (2011) 55:274–9. doi: 10.1016/j.cyto.2011.04.007
52. Goh J, Tsai J, Bammler TK, Farin FM, Endicott E, Ladiges WC. Exercise training in transgenic mice is associated with attenuation of early breast cancer growth in a dose-dependent manner. PLoS ONE. (2013) 8:e80123. doi: 10.1371/journal.pone.0080123
53. Khori V, Shalamzari SA, Isanejad A, Alizadeh AM, Alizadeh S, Khodayari S, et al. Effects of exercise training together with tamoxifen in reducing mammary tumor burden in mice: possible underlying pathway of miR-21. Eur J Pharmacol. (2015) 765:179–87. doi: 10.1016/j.ejphar.2015.08.031
54. Bianco TM, Abdalla DR, Desiderio CS, Thys S, Simoens C, Bogers JP, et al. The influence of physical activity in the anti-tumor immune response in experimental breast tumor. Immunol Lett. (2017) 190:148–58. doi: 10.1016/j.imlet.2017.08.007
55. Buss LA, Dachs GU. Voluntary exercise slows breast tumor establishment and reduces tumor hypoxia in ApoE(-/-) mice. J Appl Physiol. (2018) 124:938–49. doi: 10.1152/japplphysiol.00738.2017
56. Faustino-Rocha AI, Gama A, Oliveira PA, Alvarado A, Neuparth MJ, Ferreira R, et al. Effects of lifelong exercise training on mammary tumorigenesis induced by MNU in female Sprague-Dawley rats. Clin Exp Med. (2017) 17:151–60. doi: 10.1007/s10238-016-0419-0
57. Saez Mdel C, Barriga C, Garcia JJ, Rodriguez AB, Ortega E. Exercise-induced stress enhances mammary tumor growth in rats: beneficial effect of the hormone melatonin. Mol Cell Biochem. (2007) 294:19–24. doi: 10.1007/s11010-005-9067-5
58. Ligibel J, Dillon DA, Giobbie-Hurder A, McTiernan A, Frank ES, Cornwell M, et al. Impact of a pre-operative exercise intervention on breast cancer proliferation and gene expression: results from the Pre-Operative Health and Body (PreHAB) Study. Clin Cancer Res. (2019) 25:5398–406. doi: 10.1158/1078-0432.ccr-18-3143
59. Mijwel S, Bolam KA, Gerrevall J, Foukakis T, Wengstrom Y, Rundqvist H. Effects of exercise on chemotherapy completion and hospitalization rates: the OptiTrain breast cancer trial. Oncologist. (2019) 24:1–10. doi: 10.1634/theoncologist.2019-0262
60. Kim JJ, Shin YA, Suk MH. Effect of a 12-week walking exercise program on body composition and immune cell count in patients with breast cancer who are undergoing chemotherapy. J Exerc Nutr Biochem. (2015) 19:255–62. doi: 10.5717/jenb.2015.15092812
61. Giallauria F, Gentile M, Chiodini P, Mattiello A, Maresca L, Vitelli A, et al. Exercise training reduces high mobility group box-1 levels in women with breast cancer: the DIANA (diet and androgens)-5 project. Eur J Prev Cardiol. (2014) 21(1 Suppl. 1):S112. doi: 10.1177/2047487314534582
62. Sturgeon KM, Foo W, Heroux M, Schmitz K. Change in inflammatory biomarkers and adipose tissue in BRCA1/2(+) breast cancer survivors following a yearlong lifestyle modification program. Cancer Prev Res. (2018) 11:545–50. doi: 10.1158/1940-6207.capr-18-0098
63. Peters C, Lotzerich H, Niemeier B, Schule K, Uhlenbruck G. Influence of a moderate exercise training on natural-killer cytotoxicity and personality-traits in cancer-patients. Anticancer Res. (1994) 14(3A):1033–6.
64. Peters C, Lotzerich H, Niemeir B, Schule K, Uhlenbruck G. Exercise, cancer and the immune-response of monocytes. Anticancer Res. (1995) 15:175–9.
65. Loo LWM, Nishibun K, Welsh L, Makolo T, Chong CD, Pagano I, et al. Using a cultural dance program to increase sustainable physical activity for breast cancer survivors-A pilot study. Complement Ther Med. (2019) 47:102197. doi: 10.1016/j.ctim.2019.102197
66. Dethlefsen C, Lillelund C, Midtgaard J, Andersen C, Pedersen BK, Christensen JF, et al. Exercise regulates breast cancer cell viability: systemic training adaptations versus acute exercise responses. Breast Cancer Res Treat. (2016) 159:469–79. doi: 10.1007/s10549-016-3970-1
67. Hutnick NA, Williams NI, Kraemer WJ, Orsega-Smith E, Dixon RH, Bleznak AD, et al. Exercise and lymphocyte activation following chemotherapy for breast cancer. Med Sci Sports Exerc. (2005) 37:1827–35. doi: 10.1249/01.mss.0000175857.84936.1a
68. Dieli-Conwright CM, Parmentier JH, Sami N, Lee K, Spicer D, Mack WJ, et al. Adipose tissue inflammation in breast cancer survivors: effects of a 16-week combined aerobic and resistance exercise training intervention. Breast Cancer Res Treat. (2018) 168:147–57. doi: 10.1007/s10549-017-4576-y
69. Fairey AS, Courneya KS, Field CJ, Bell GJ, Jones LW, Mackey JR. Randomized controlled trial of exercise and blood immune function in postmenopausal breast cancer survivors. J Appl Physiol. (2005) 98:1534–40. doi: 10.1152/japplphysiol.00566.2004
70. Fairey AS, Courneya KS, Field CJ, Bell GJ, Jones LW, Martin BS, et al. Effect of exercise training on C-reactive protein in postmenopausal breast cancer survivors: a randomized controlled trial. Brain Behav Immun. (2005) 19:381–8. doi: 10.1016/j.bbi.2005.04.001
71. Rogers LQ, Fogleman A, Trammell R, Hopkins-Price P, Vicari S, Rao K, et al. Effects of a physical activity behavior change intervention on inflammation and related health outcomes in breast cancer survivors: pilot randomized trial. Integrative Cancer Ther. (2013) 12:323–35. doi: 10.1177/1534735412449687
72. Rogers LQ, Vicari S, Trammell R, Hopkins-Price P, Fogleman A, Spenner A, et al. Biobehavioral factors mediate exercise effects on fatigue in breast cancer survivors. Med Sci Sports Exer. (2014) 46:1077–88. doi: 10.1249/mss.0000000000000210
73. Alizadeh AM, Isanejad A, Sadighi S, Mardani M, Kalaghchi B, Hassan ZM. High-intensity interval training can modulate the systemic inflammation and HSP70 in the breast cancer: a randomized control trial. J Cancer Res Clin Oncol. (2019) 145:2583–93. doi: 10.1007/s00432-019-02996-y
74. Gómez AM, Martínez C, Fiuza-Luces C, Herrero F, Pérez M, Madero L, et al. Exercise training and cytokines in breast cancer survivors. Int J Sports Med. (2011) 32:461–7. doi: 10.1055/s-0031-1271697
75. Jones SB, Thomas GA, Hesselsweet SD, Alvarez-Reeves M, Yu H, Irwin ML. Effect of exercise on markers of inflammation in breast cancer survivors: the Yale exercise and survivorship study. Cancer Prev Res. (2013) 6:109–18. doi: 10.1158/1940-6207.capr-12-0278
76. Nieman DC, Cook VD, Henson DA, Suttles J, Rejeski WJ, Ribisl PM, et al. Moderate exercise training and natural killer cell cytotoxic activity in breast cancer patients. Int J Sports Med. (1995) 16:334–7. doi: 10.1055/s-2007-973015
77. Tizdast N, Ghazalian F, Gholami M. The effect of exercise type on inflammatory markers in obese survivors with breast cancer: randomized control trial. Health Scope. (2016) 5:1–5. doi: 10.17795/jhealthscope-33421
78. Karimi N, Dabidi Roshan V, Fathi Bayatiyani Z. Individually and combined water-based exercise with ginger supplement, on systemic inflammation and metabolic syndrome indices, among the obese women with breast neoplasms. Iranian J Cancer Prev. (2015) 8:e3856. doi: 10.17795/ijcp-3856
79. Zimmer P, Baumann FT, Oberste M, Schmitt J, Joisten N, Hartig P, et al. Influence of personalized exercise recommendations during rehabilitation on the sustainability of objectively measured physical activity levels, fatigue, and fatigue-related biomarkers in patients with breast cancer. Integrative Cancer Ther. (2018) 17:306–11. doi: 10.1177/1534735417713301
80. Pineiro R. Introduction - biology of breast cancer metastasis and importance of the analysis of CTCs. Adv Exp Med Biol. (2020) 1220:1–10. doi: 10.1007/978-3-030-35805-1_1
81. Eckhardt BL, Francis PA, Parker BS, Anderson RL. Strategies for the discovery and development of therapies for metastatic breast cancer. Nat Rev Drug Discov. (2012) 11:479–97. doi: 10.1038/nrd2372
82. Barkan D, Kleinman H, Simmons JL, Asmussen H, Kamaraju AK, Hoenorhoff MJ, et al. Inhibition of metastatic outgrowth from single dormant tumor cells by targeting the cytoskeleton. Cancer Res. (2008) 68:6241–50. doi: 10.1158/0008-5472.CAN-07-6849
83. Almog N, Ma L, Raychowdhury R, Schwager C, Erber R, Short S, et al. Transcriptional switch of dormant tumors to fast-growing angiogenic phenotype. Cancer Res. (2009) 69:836–44. doi: 10.1158/0008-5472.CAN-08-2590
84. Koebel CM, Vermi W, Swann JB, Zerafa N, Rodig SJ, Old LJ, et al. Adaptive immunity maintains occult cancer in an equilibrium state. Nature. (2007) 450:903–7. doi: 10.1038/nature06309
85. Eyles J, Puaux AL, Wang X, Toh B, Prakash C, Hong M, et al. Tumor cells disseminate early, but immunosurveillance limits metastatic outgrowth, in a mouse model of melanoma. J Clin Invest. (2010) 120:2030–9. doi: 10.1172/JCI42002
86. Candido J, Hagemann T. Cancer-related inflammation. J Clin Immunol. (2013) 33(Suppl. 1):S79–84. doi: 10.1007/s10875-012-9847-0
87. Smith HA, Kang Y. The metastasis-promoting roles of tumor-associated immune cells. J Mol Med. (2013) 91:411–29. doi: 10.1007/s00109-013-1021-5
88. Walsh NP, Gleeson M, Shephard RJ, Woods JA, Bishop NC, Fleshner M, et al. Position statement. Part one: Immune function and exercise. Exerc Immunol Rev. (2011) 17:6–63.
89. Pedersen BK, Febbraio MA. Muscles, exercise and obesity: skeletal muscle as a secretory organ. Nat Rev Endocrinol. (2012) 8:457–65. doi: 10.1038/nrendo.2012.49
90. Adams S, Gatti-Mays ME, Kalinsky K, Korde LA, Sharon E, Amiri-Kordestani L, et al. Current landscape of immunotherapy in breast cancer: a review. JAMA Oncol. (2019) 5:1205–14. doi: 10.1001/jamaoncol.2018.7147
91. de la Cruz-Merino L, Palazon-Carrion N, Henao-Carrasco F, Nogales-Fernandez E, Alamo-de la Gala M, Vallejo-Benitez A, et al. New horizons in breast cancer: the promise of immunotherapy. Clin Transl Oncol. (2019) 21:117–25. doi: 10.1007/s12094-018-1907-3
92. Diana A, Carlino F, Franzese E, Oikonomidou O, Criscitiello C, De Vita F, et al. Early triple negative breast cancer: conventional treatment and emerging therapeutic landscapes. Cancers. (2020) 12:819. doi: 10.3390/cancers12040819
93. Savas P, Salgado R, Denkert C, Sotiriou C, Darcy PK, Smyth MJ, et al. Clinical relevance of host immunity in breast cancer: from TILs to the clinic. Nat Rev Clin Oncol. (2016) 13:228–41. doi: 10.1038/nrclinonc.2015.215
94. Cimino-Mathews A, Thompson E, Taube JM, Ye X, Lu Y, Meeker A, et al. PD-L1 (B7-H1) expression and the immune tumor microenvironment in primary and metastatic breast carcinomas. Hum Pathol. (2016) 47:52–63. doi: 10.1016/j.humpath.2015.09.003
95. Li X, Li M, Lian Z, Zhu H, Kong L, Wang P, et al. Prognostic role of programmed death ligand-1 expression in breast cancer: a systematic review and meta-analysis. Target Oncol. (2016) 11:753–61. doi: 10.1007/s11523-016-0451-8
96. Loi S, Drubay D, Adams S, Pruneri G, Francis PA, Lacroix-Triki M, et al. Tumor-infiltrating lymphocytes and prognosis: a pooled individual patient analysis of early-stage triple-negative breast cancers. J Clin Oncol. (2019) 37:559–69. doi: 10.1200/JCO.18.01010
97. Park JH, Jonas SF, Bataillon G, Criscitiello C, Salgado R, Loi S, et al. Prognostic value of tumor-infiltrating lymphocytes in patients with early-stage triple-negative breast cancers (TNBC) who did not receive adjuvant chemotherapy. Ann Oncol. (2019) 30:1941–9. doi: 10.1093/annonc/mdz395
98. Kovacs A, Stenmark Tullberg A, Werner Ronnerman E, Holmberg E, Hartman L, Sjostrom M, et al. Effect of radiotherapy after breast-conserving surgery depending on the presence of tumor-infiltrating lymphocytes: a long-term follow-up of the SweBCG91RT randomized trial. J Clin Oncol. (2019) 37:1179–87. doi: 10.1200/JCO.18.02157
99. Denkert C, von Minckwitz G, Darb-Esfahani S, Lederer B, Heppner BI, Weber KE, et al. Tumour-infiltrating lymphocytes and prognosis in different subtypes of breast cancer: a pooled analysis of 3771 patients treated with neoadjuvant therapy. Lancet Oncol. (2018) 19:40–50. doi: 10.1016/S1470-2045(17)30904-X
100. Ribas A, Wolchok JD. Cancer immunotherapy using checkpoint blockade. Science. (2018) 359:1350–5. doi: 10.1126/science.aar4060
101. Barroso-Sousa R, Tolaney SM. Clinical development of PD-1/PD-L1 inhibitors in breast cancer: still a long way to go. Curr Treat Options Oncol. (2020) 21:59. doi: 10.1007/s11864-020-00756-6
102. Dirix LY, Takacs I, Jerusalem G, Nikolinakos P, Arkenau HT, Forero-Torres A, et al. Avelumab, an anti-PD-L1 antibody, in patients with locally advanced or metastatic breast cancer: a phase 1b JAVELIN Solid Tumor study. Breast Cancer Res Treat. (2018) 167:671–86. doi: 10.1007/s10549-017-4537-5
103. Emens LA, Cruz C, Eder JP, Braiteh F, Chung C, Tolaney SM, et al. Long-term clinical outcomes and biomarker analyses of atezolizumab therapy for patients with metastatic triple-negative breast cancer: a phase 1 study. JAMA Oncol. (2019) 5:74–82. doi: 10.1001/jamaoncol.2018.4224
104. Adams S, Schmid P, Rugo HS, Winer EP, Loirat D, Awada A, et al. Pembrolizumab monotherapy for previously treated metastatic triple-negative breast cancer: cohort A of the phase II KEYNOTE-086 study. Ann Oncol. (2019) 30:397–404. doi: 10.1093/annonc/mdy517
105. Nanda R, Chow LQ, Dees EC, Berger R, Gupta S, Geva R, et al. Pembrolizumab in patients with advanced triple-negative breast cancer: phase Ib KEYNOTE-012 study. J Clin Oncol. (2016) 34:2460–7. doi: 10.1200/JCO.2015.64.8931
106. Emens LA. Breast cancer immunotherapy: facts and hopes. Clin Cancer Res. (2018) 24:511–20. doi: 10.1158/1078-0432.CCR-16-3001
107. U.S. Food and Drug Administration. FDA Approves Atezolizumab for PD-L1 Positive Unresectable Locally Advanced or Metastatic Triple-Negative Breast Cancer. (2019). Available online at: https://www.fda.gov/drugs/drug-approvals-and-databases/fda-approves-atezolizumab-pd-l1-positive-unresectable-locally-advanced-or-metastatic-triple-negative
Keywords: exercise, immunity, inflammation, recurrence, survival
Citation: Xu Y and Rogers CJ (2020) Physical Activity and Breast Cancer Prevention: Possible Role of Immune Mediators. Front. Nutr. 7:557997. doi: 10.3389/fnut.2020.557997
Received: 01 May 2020; Accepted: 21 August 2020;
Published: 08 October 2020.
Edited by:
Ornella Ida Selmin, University of Arizona, United StatesReviewed by:
Henry J. Thompson, Colorado State University, United StatesCharikleia Stefanaki, National and Kapodistrian University of Athens, Greece
Copyright © 2020 Xu and Rogers. This is an open-access article distributed under the terms of the Creative Commons Attribution License (CC BY). The use, distribution or reproduction in other forums is permitted, provided the original author(s) and the copyright owner(s) are credited and that the original publication in this journal is cited, in accordance with accepted academic practice. No use, distribution or reproduction is permitted which does not comply with these terms.
*Correspondence: Connie J. Rogers, Y2pyMTAyQHBzdS5lZHU=