- 1Department of Horticultural Science, Imam Khomeini International University, Qazvin, Iran
- 2Department of Production Engineering and Plant Genetics, Faculty of Agriculture, Higher Education Complex of Saravan, Saravan, Iran
- 3Department of Genetic and Plant Breeding, Faculty of Agriculture and Natural Resources, Imam Khomeini International University, Qazvin, Iran
During postharvest life, broccoli suffers from floret yellowing confining its economic and nutritional value. The objective of the present study was to explore the mechanisms employed by phytosulfokine α (PSKα) at 150 nM for delaying floret yellowing in broccoli during storage at 4°C for 28 days. Our results showed that the higher endogenous accumulation of hydrogen sulfide (H2S) resulting from the higher gene expression and activities of L-cysteine desulfhydrase (LCD) and D-cysteine desulfhydrase (DCD) in broccoli floret treated with 150 nM PSKα may serve as an endogenous signaling molecule for delaying senescence. Moreover, the suppressed ethylene biosynthesis in broccoli floret treated with 150 nM PSKα might be ascribed to lower gene expression and activities of ACC synthase (ACS) and ACC oxidase (ACO). Furthermore, lower gene expression and activities of Mg2+ dechelatase (MDC), pheophytinase (PPH), and pheophorbide a oxygenase (PaO) might be the reasons for the higher accumulation of chlorophyll in broccoli floret treated with 150 nM PSKα. Based on our findings, exogenous PSKα application could be employed as signaling bioactive hormone for retarding floret yellowing of broccoli during storage at 4°C for 28 days.
Introduction
Owing to higher health-promoting bioactive molecules accumulation, broccoli has gained worldwide attention as a global healthy food crop, which is beneficial for ensuring human health by reducing the risk of chronic diseases, such as cancer, cardiovascular diseases, and neurodegenerative diseases in industrial countries (1).
By harvesting broccoli prematurely, a high accumulation of intracellular reactive oxygen species (ROS) (2), an insufficient intracellular supply of ATP (3, 4), an imbalance of intracellular hormones, signified by a higher biosynthesis of ethylene and a lower biosynthesis of cytokinin (5, 6), and chlorophyll degradation via the pheophorbide a oxygenase (PaO) pathway (2, 6–9) may be the mechanisms for the accelerated broccoli senescence, manifested by floret yellowing, which confines its commercial value. In recent years, postharvest procedures, such as 1-methylcyclopropene (1-MCP) (10), nitric oxide (2), brassinolide (6), putrescine (8), hydrogen sulfide (H2S) (4, 11), cytokinins (12), folic acid (13), melatonin (14), arginine, cysteine, and methionine (15), and phytosulfokine α (PSKα) (16–18), have been employed by researchers for delaying broccoli senescence manifested by floret yellowing during cold storage.
In plants, providing sufficient cysteine is crucial for H2S biosynthesis by L-cysteine desulfhydrase (LCD) enzyme activity in the cytosol and mitochondria and D-cysteine desulfhydrase (DCD) enzyme activity in the mitochondria (19–21). By exogenous H2S application, endogenous H2S accumulation resulting from higher LCD and DCD gene expressions and enzyme activities has been beneficial for improving the marketability in horticultural crops by sufficient intracellular ATP supply by promoting H+-ATPase, Ca2+-ATPase, cytochrome c oxidase (CCO), and succinate dehydrogenase (SDH) enzyme activities, preventing chlorophyll degradation by suppressing chlorophyll b reductase (CBR), chlorophyllase (Chlase), Mg2+ dechelatase (MDC), pheophytinase (PPH), and PaO gene expressions and enzyme activities, promoting endogenous proline accumulation by triggering pyrroline-5-carboxylate synthase (P5CS) and ornithine aminotransferase (OAT) gene expressions and enzyme activities along with hindering proline dehydrogenase (PDH) gene expression and enzyme activity, promoting ROS scavenging superoxide dismutase (SOD), catalase (CAT), ascorbate peroxidase (APX), and glutathione reductase (GR) gene expressions and enzyme activities leading to lower and H2O2 accumulation, keeping membrane integrity by presenting lower electrolyte leakage and malondialdehyde (MDA) accumulation resulting from lower phospholipase D (PLD) and lipoxygenase (LOX) enzyme activities, preventing ethylene biosynthesis by suppressing ACC synthase (ACS) and ACC oxidase (ACO) gene expressions and enzyme activities, promoting phenylpropanoid pathway activity by presenting higher phenylalanine ammonia lyase (PAL)/polyphenol oxidase (PPO) enzyme activity leading to higher phenols, flavonoids, and anthocyanins accumulation, promoting oxidative pentose phosphate pathway activity by presenting higher glucose 6-phosphate dehydrogenase (G6PDH) and 6-phosphogluconate dehydrogenase (6PGDH) enzyme activities for supplying NADPH and erythritol 4-phosphate, and promoting chitinase and β-1,3-glucanase gene expressions and enzyme activities (4, 22–30).
PSKα [Tyr(SO3H)-Ile-Tyr(SO3H)-Thr-Gln] is a bioactive signaling tyrosine-disulfate pentapeptide hormone biosynthesized from a prepropeptide of 80–120 amino acid prepropeptides encoded by the PSKs gene (31, 32). By promoting cytosolic second messenger guanosine 3′,5′-cyclic monophosphate (cGMP) accumulation by employing exogenous PSKα application resulting from phytosulfokine receptor 1 (PAR1) kinase encapsulating guanylate cyclase activity, triggering cytosolic Ca2+ accumulation may be responsible for triggering friendly extracellular ATP and intracellular SnRK1 signaling pathways, triggering SUMO E3 ligase SIZ1 system activity accompanied by suppressing NAD+ dissipating poly(ADP-ribose) polymerase 1 (PARP1) system activity for ensuring sufficient intracellular ATP supply resulting from higher H+-ATPase, Ca2+-ATPase, CCO, and SDH enzyme activities accompanied by sufficient intracellular NADPH supply resulting from higher G6PDH, 6PGDH, and methylenetetrahydrofolate dehydrogenase (MTHFD) enzyme activities, triggering ROS avoiding alternative oxidase (AOX) and uncoupling protein (UCP1) gene expressions and scavenging SOD, CAT, APX, and GR gene expressions and enzyme activities, promoting phenylpropanoid pathway activity by presenting higher PAL and chalcone synthase (CHS) gene expressions and enzyme activities leading to higher phenols, flavonoids, and anthocyanins accumulation, promoting ascorbic acid accumulation resulting from higher l-galactono-1,4-lactone dehydrogenase (GLDH) gene expression along with lower ascorbic acid oxidase (AAO) gene expression, promoting endogenous melatonin accumulation resulting from higher tryptophan decarboxylase (TDC), tryptamine 5-hydroxylase (T5H), serotonin N-acetyltransferase (SNAT), and N-acetylserotonin O-methyltransferase (ASMT) gene expressions, promoting endogenous cytokinin accumulation resulting from higher isopentenyl transferase (IPT) gene expression concomitant with lower cytokinin oxidase (CKO) gene expression, suppressing PLD and LOX gene expressions and enzyme activities, triggering heat shock protein (HSP70 and HSP90) gene expressions, and improving 2,2-diphenyl-1-picrylhydrazyl (DPPH), ferric ion reducing antioxidant power (FRAP), and DPPH scavenging capacity resulting from higher phenols, flavonoids, anthocyanins, and ascorbic acid accumulation may be responsible for attenuating chilling injury and fungal decay, delaying senescence and maintaining the nutritional quality of fruits and vegetables during cold storage (16–18, 33–37). However, there are ongoing attempts to introduce safe and operative procedures for avoiding floret yellowing and preserving the floret quality of broccoli during cold storage.
Therefore, triggering endogenous PSKα signaling pathway by exogenous PSKα application or endogenous PSKα accumulation may be efficient for relieving chilling injury and fungal decay, delaying senescence and keeping the quality of horticultural crops during postharvest life (16–18, 33–37). The present study aimed to investigate the connection between exogenous PSKα application and broccoli floret yellowing and elucidate its mechanisms from the perspective of endogenous H2S accumulation by LCD and DCD gene expressions and enzyme activities, chlorophyll degradation by MDC, PPH, and PaO gene expressions, and enzyme activities accompanied by ethylene biosynthesis by ACS and ACO gene expressions and enzyme activities by employing exogenous PSKα application at 0 and 150 nM during storage at 4°C for 28 days.
Materials and Methods
Broccoli and PSKα Application
Broccoli (Brassica oleracea var. italica) was harvested at commercial maturity when the individual floret was still closed and was dark green and the inflorescence was compact. Healthy broccoli heads with uniform size, color, and maturity stage were selected for PSKα treatment. PSKα (soluble in sterile water, 1 mg/ml) was provided by Pepmic Co., Ltd., Suzhou, China. For PSKα treatment, 240 broccoli heads were allocated into two groups of 120 (40 heads per replicate) for the experimental treatments, applied by spraying the heads with 25 ml of PSKα at 0 (control; double distilled water, ddH2O) or 150 nM according to Aghdam et al. (16) and Aghdam and Luo (18). After drying overnight, the broccoli heads were packaged in polyethylene bags (50 ×80 cm, 0.04 mm) and stored at 4 ± 0.5°C and 85% relative humidity for 28 days. Then, every 7 days during storage at 4°C, floret from 10 broccolis was excised, immediately frozen in liquid nitrogen, powdered, and stored at −80°C for biochemical and gene expression analysis. For biochemical and gene expression analysis, three technical replications were carried out by three extractions from each biological replication to avoid instrumental error. The means of three technical replications are considered as one biological replication.
Broccoli Floret Yellowing
Broccoli floret yellowing (%) was assessed by a scale from 0 to 9 as stated by Shi et al. (2), where 0 indicates all dark green, 1 indicates 25% yellowing, 5 indicates 50% yellowing, 7 indicates 75% yellowing, and 9 indicates 100% yellowing of the broccoli floret.
Chlorophyll Degradation by MDC and PPH Enzyme Activities
Chlorophyll accumulation in broccoli floret was measured according to Gómez-Lobato et al. (38), by homogenizing 1 g of frozen powder with 10 ml of 80% (v/v) acetone and centrifugation at 10,000 × g for 10 min at 4°C. By measuring absorbance at 663 and 645 nm, chlorophyll accumulation was calculated using the formula:
Chlorophyll accumulation (g kg−1 FW) = (17.76 × A645nm) + (7.34 × A663nm).
For analyzing MDC and PPH enzyme activities, 1 g of frozen powder was homogenized with 10 ml of cold acetone. After centrifugation at 12,000 × g for 5 min at 4°C, the precipitate was used for assaying MDC and PPH enzyme activities according to 38. MDC enzyme activity was assayed by pheophorbide a formation at 686 nm and was expressed in mkatals produced per mass of protein, mkat kg−1. PPH enzyme activity was assayed by pheophorbide a formation at 667 nm and was expressed in mkatals produced per mass of protein, mkat kg−1.
Ethylene Biosynthesis by ACS and ACO Enzyme Activities
Ethylene production was measured using gas chromatography as stated by Fan et al. (39). Ethylene production was expressed as μg kg−1 h−1 on a fresh weight basis. For ACS enzyme activity, 1 g of frozen powder was homogenized with 5 ml of 100 mM sodium phosphate buffer (pH 9.0) containing 5 μM pyridoxal phosphate, 4 mM β-mercaptoethanol, 1 mM ethylenediaminetetraacetic acid (EDTA), and 10% (v/v) glycerol. After centrifugation at 16,000 × g for 20 min at 4°C, the supernatant was used for ACS enzyme activity assaying according to Suzuki et al. (40). ACS activity was expressed based on ACC production as μmol kg−1 h−1 on a fresh weight basis. For ACO enzyme activity, 1 g of frozen powder was homogenized with 10 ml of 100 mM Tris–HCl buffer (pH 7.2) containing 30% (w/v) glycerol, 10 mM sodium ascorbate, and 5 mM dithiothreitol (DTT). After centrifugation at 14,000 × g for 20 min at 4°C, the supernatant was used for ACO enzyme activity assaying according to Suzuki et al. (41). ACO activity was expressed based on ethylene production as μmol kg−1 h−1 on a fresh weight basis.
Endogenous H2S Accumulation by LCD and DCD Enzyme Activities
For endogenous H2S accumulation assaying by methylene blue method as stated by Li et al. (4), 1 g of frozen powder was homogenized with 10 ml of 50 mM phosphate buffer saline (pH 6.8) containing 0.2 M ascorbic acid and 0.1 M EDTA. After centrifugation at 10,000 × g for 20 min, the supernatants were mixed in a test tube containing 0.1 M phosphate buffer saline (pH 7.4), 2 mM phosphopyridoxal, and 10 mM L-cysteine. The released H2S was absorbed in a zinc acetate trap. The absorbance was measured at 667 nm, and the calibration curve was established with Na2S solution concentrations. Endogenous H2S accumulation was expressed as μmol kg−1 on a fresh weight basis. The LCD and DCD enzyme activities were analyzed as stated by Li et al. (4). Then, 1 g of frozen powder was homogenized with 20 mM Tris–HCl (pH 8.0). The homogenate was centrifuged at 12,000 × g for 20 min. For LCD and DCD enzyme activity assays, 1 ml of supernatant was mixed with 1 ml of mixture solution containing 100 mM Tris–HCl (pH 9.0 for LCD enzyme and 8.0 for DCD enzyme), 0.8 mM L-cysteine for LCD enzyme and D-cysteine for DCD enzyme, and 2.5 mM DTT. After incubation at 37°C for 15 min, the reaction was terminated by adding 100 μl of 30 mM FeCl3 dissolved in 1.2 M HCl and 100 μl of 20 mM N,N-dimethyl-p-phenylenediamine (DMPD) dissolved in 7.2 M HCl. Then, the formation of methylene blue was recorded at 670 nm. A calibration curve was established with Na2S solution concentrations. The LCD and DCD enzyme activities were expressed on a fresh weight basis as μmol kg−1 h−1.
Genes Expression Assay by RT-qPCR
A 1 g of frozen powder was used for total RNA extraction from broccoli floret by Trizol reagent (Invitrogen, CA, USA). To confirm total RNA quantity and quality, we determined the absorbance at 260 nm by using a Thermo Scientific™ NanoDrop™ One Spectrophotometer and 1.0% agarose gel electrophoresis. The cDNA was synthesized from 2 μg of total RNA using the SuperScript® RT (Invitrogen, CA, USA) kit according to the manufacturer's instructions. The cDNA was used as a template for assaying the relative expression of genes by quantitative reverse transcription-PCR (RT-qPCR) utilizing a StepOne™ Real-Time PCR System. The final volume of 10 μl containing 1 μl of cDNA, 100 nM primers (Supplementary Table 1), and 5 μl of 2 × SYBR GREEN I Master Mix (TaKaRa, Japan) was prepared according to the instruction provided by the manufacturer. All primers' specificity was determined using the BLAST sequence alignment with Primer-BLAST software. To better quantify the relative expression of the genes, the threshold cycle (Ct) value was normalized to the Actin Ct value and calculated following 2−ΔΔCt according to Livak and Schmittgen (42).
Statistical Analysis
The experiment was planned using split plots in a time model based on a completely randomized design (CRD). For biochemical and genes expression analysis, three technical replications were carried out by three extractions from each biological replication to avoid instrumental error. The means of three technical replications are considered as one biological replication. All data were expressed as mean ± standard error (SE) from three biological replications. ANOVA was carried out, and the mean was compared using Tukey's test at a significance level of 0.01 using the SPSS software (version 19.0).
Results and Discussion
Broccoli Floret Yellowing and Ethylene Production
As depicted in Supplementary Figure 1, broccoli treated with PSKα at 150 nM exhibited the lowest floret yellowing during storage at 4°C for 28 days (P < 0.01). Additionally, retarding floret yellowing in broccoli treated with PSKα at 150 nM was concomitant with lower ethylene production (P < 0.01; Figure 1), which might be attributed to lower ACS1 and ACO1 gene expressions and enzyme activities (P < 0.01; Figure 1) during storage at 4°C for 28 days. During postharvest life, higher ethylene biosynthesis by ACS and ACO gene expressions and enzyme activities may be the reason for triggering floret yellowing in broccoli by promoting membrane phospholipids degradation by PLD gene expression and enzyme activity leading to free fatty acids supplying for peroxidation by LOX gene expression and enzyme activity. Higher LOX activity not only is liable for deteriorating membrane fluidity and integrity signifying higher MDA accumulation but also is liable for endogenous jasmonic acid accumulation for accelerating broccoli floret yellowing by motivating CBR, PPH, and PaO gene expressions and enzyme activities (38, 43, 44). Hence, suppressing ethylene biosynthesis accompanied by hampering membrane deteriorating LOX enzymes activity by exogenous 1-MCP and exogenous cytokinin application or IPT gene overexpression has been employed successfully for suppressing floret yellowing in broccoli during postharvest life (5, 40, 45, 46). According to our results, lower ethylene production in broccoli floret treated with PSKα at 150 nM might be attributed to lower ACS1 and ACO1 gene expressions and enzyme activities during storage at 4°C for 28 days. Hence, low temperature storage (4°C) synergistically by exogenous PSKα application may be crucial for suppressing ethylene production efficient for suppressing floret senescence manifested by yellowing.
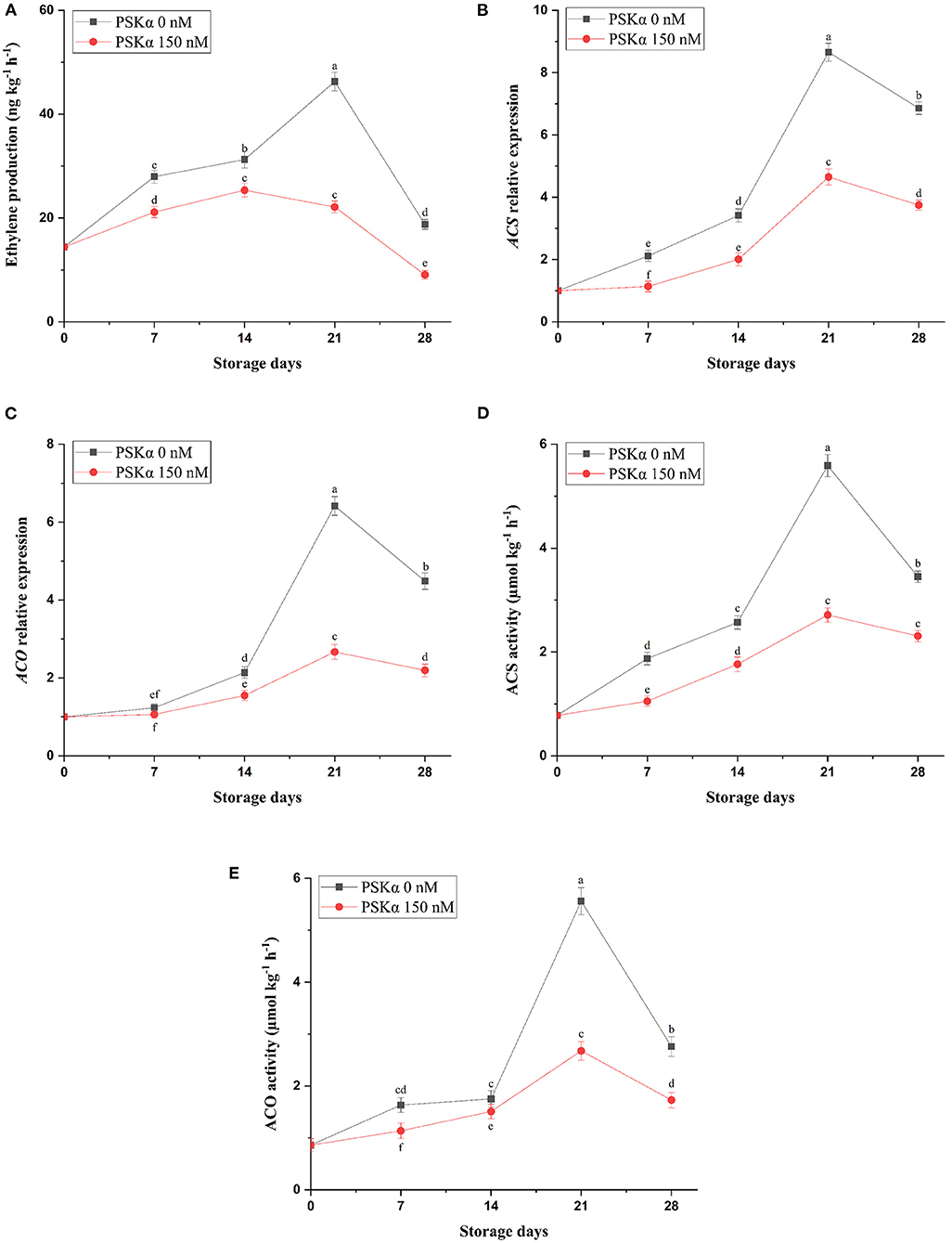
Figure 1. Ethylene production (A) accompanied by ACS and ACO gene expressions (B,C) and enzyme activities (D,E) in broccoli floret treated with PSKα at 0 and 150 nM during storage at 4°C for 28 days. Data shown are mean values of n = 3, and the error bars represent standard errors of the means. Tukey's test at P = 0.05 level.
Broccoli Floret Chlorophyll Degradation
As depicted in Figure 2, broccoli treated with PSKα at 150 nM exhibited higher chlorophyll accumulation (P < 0.01; Figure 2), which might be attributed to lower PPH and PaO gene expressions (P < 0.01; Figure 2) accompanied by lower MDC and PPH enzyme activities (P < 0.01; Figure 2) during storage at 4°C for 28 days. During postharvest senescence, PaO pathway activity is liable for chlorophyll degradation. During PAO pathway activity, Chlase enzyme activity is liable for phytol removal from chlorophyll a leading to chlorophyllide a producing in the thylakoid membrane. Chen et al. (47) reported that the silencing Chlase gene expression suppressed floret yellowing during postharvest life. After chlorophyll a dephytylation by Chlase, MDC enzyme activity is liable for Mg2+ removing from chlorophyllide a producing pheophorbide a in chloroplast stroma (48). In broccoli floret during senescence, demetallation may be the reason for producing pheophytin a from chlorophyll a by MDC enzyme activity. Then, PPH enzyme activity is liable for producing pheophorbide a in chloroplast stroma from pheophytin a (49–51). Suppressing floret yellowing in broccoli by cytokinin treatment might be ascribed to repressing PPH gene expression, and accelerating floret yellowing in broccoli by ethylene treatment might be ascribed to enhancing PPH gene expression (50). Then, PaO enzyme activity is liable for producing red chlorophyll catabolite (RCC) from pheophorbide a by oxidative chlorine ring-opening (48). Cai et al. (6) reported that suppressing floret yellowing in broccoli by exogenous brassinolide application might be ascribed to lower ethylene biosynthesis resulting from lower ACS and ACO gene expressions accompanied by higher chlorophyll accumulation resulting from lower Chlase, PPH, and PaO gene expressions. Hence, higher chlorophyll accumulation in broccoli floret treated with PSKα at 150 nM might be attributed to lower PPH and PaO gene expressions accompanied by lower MDC and PPH enzyme activities during storage at 4°C for 28 days.
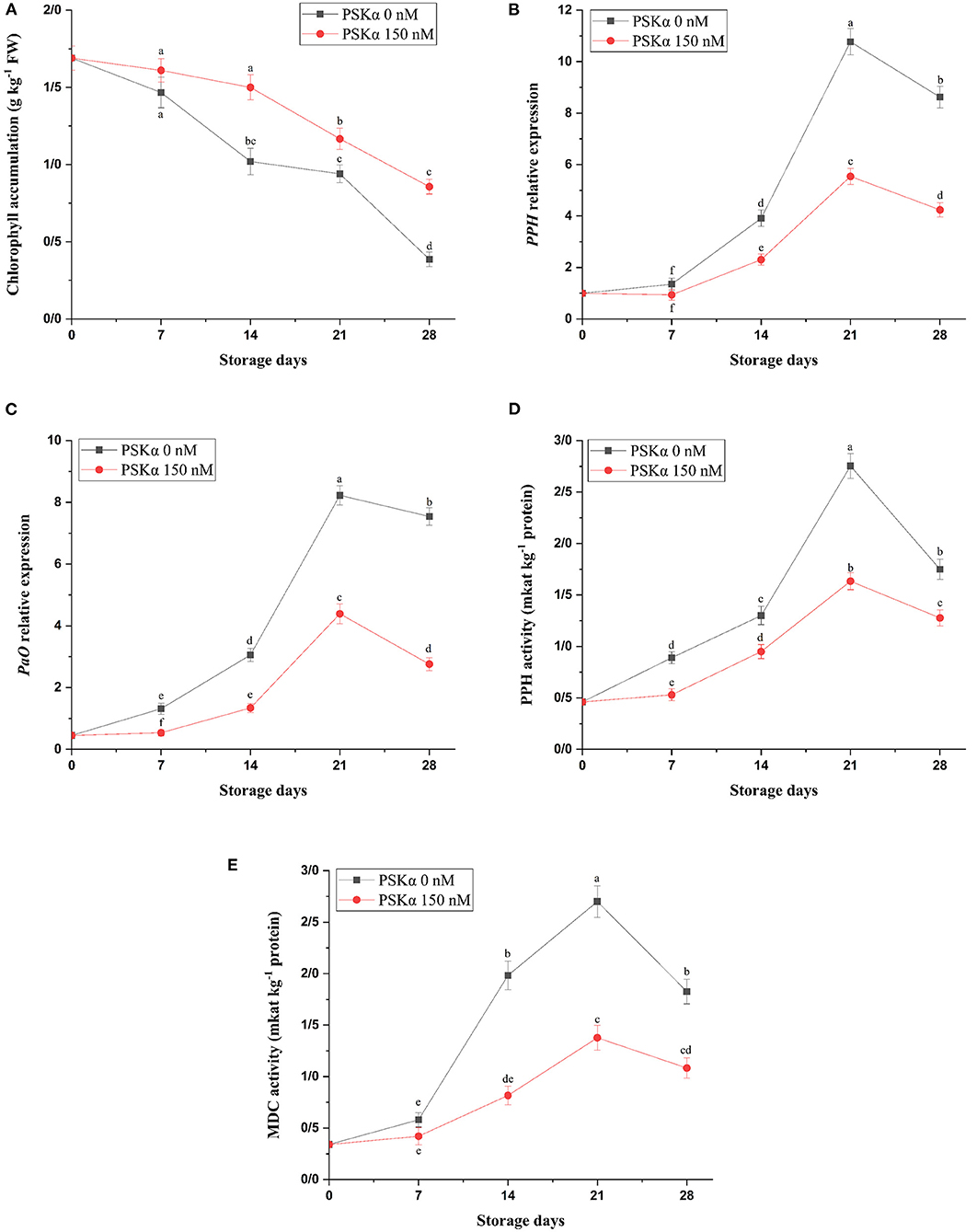
Figure 2. Chlorophyll accumulation (A) accompanied by PPH and PAO gene expressions (B,C) and MDC and PPH enzyme activities (D,E) in broccoli floret treated with PSKα at 0 and 150 nM during storage at 4°C for 28 days. Data shown are mean values of n = 3, and the error bars represent standard errors of the means. Tukey's test at P = 0.05 level.
Broccoli Floret Endogenous H2S Accumulation
As depicted in Figure 3, broccoli treated with PSKα at 150 nM exhibited higher endogenous H2S accumulation (P < 0.01; Figure 3), which might be attributed to higher LCD and DCD gene expressions and enzyme activities (P < 0.01; Figure 3) during storage at 4°C for 28 days. Hu et al. (22) reported that suppressing leaf yellowing in spinach treated with H2S might be ascribed to endogenous H2S accumulation resulting from higher LCD and DCD enzyme activities efficient for sufficient intracellular ATP supply resulting from electron transferring from sulfide to CCO for accelerating electron transport system activity accompanied by higher chlorophyll accumulation resulting from lower Chlase and MDC enzyme activities along with higher SOD and CAT enzyme activities leading to higher DPPH and scavenging capacity, keeping membrane integrity signifying lower electrolyte leakage and MDA accumulation. Li et al. (4) reported that suppressing floret yellowing in broccoli treated with H2S might be ascribed to endogenous H2S accumulation resulting from higher LCD and DCD enzyme activities efficient for sufficient intracellular ATP supply resulting from higher H+-ATPase, Ca2+-ATPase, SDH, and CCO enzyme activities accompanied by sufficient intracellular NADPH supply resulting from higher G6PDH and 6PGDH enzyme activities. Liu et al. (26) reported that suppressing daylily flower senescence treated with H2S might be ascribed to endogenous H2S accumulation resulting from higher LCD and DCD enzyme activities efficient for sufficient intracellular ATP supply resulting from higher H+-ATPase, Ca2+-ATPase, SDH, and CCO enzyme activities accompanied by higher SOD, CAT, and APX enzyme activities leading to lower and H2O2 accumulation, keeping membrane integrity signifying lower MDA accumulation. Aghdam et al. (27) reported that relieving chilling damage in hawthorn fruit treated with H2S might be ascribed to endogenous H2S accumulation resulting from higher LCD and DCD enzyme activities efficient for higher SOD, CAT, and APX enzyme activities leading to lower H2O2 accumulation; higher phenols, flavonoids, and anthocyanins accumulation; and higher DPPH scavenging activity resulting from higher PAL enzyme activity, keeping membrane integrity signifying lower MDA accumulation. Therefore, higher endogenous H2S accumulation in broccoli floret treated with PSKα at 150 nM might be attributed to higher LCD and DCD gene expressions and enzyme activities during storage at 4°C for 28 days efficient for suppressing floret yellowing by suppressing ethylene biosynthesis and chlorophyll degradation.
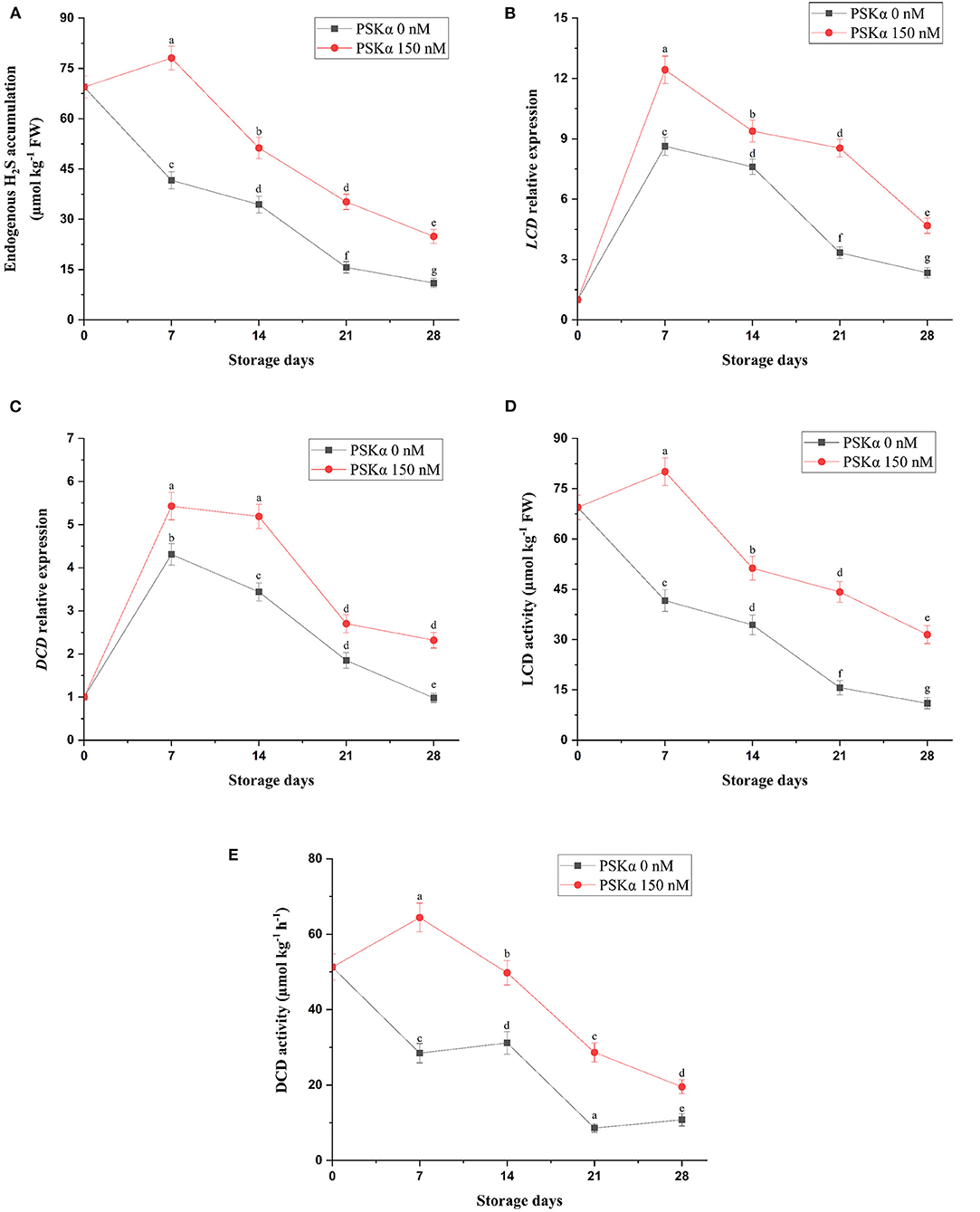
Figure 3. Endogenous H2S accumulation (A) accompanied by LCD and DCD gene expressions (B,C) and enzyme activities (D,E) in broccoli floret treated with PSKα at 0 and 150 nM during storage at 4°C for 28 days. Data shown are mean values of n = 3, and the error bars represent standard errors of the means. Tukey's test at P = 0.05 level.
Endogenous H2S accumulation serves as a signaling molecule by persulfidation, a protein post-translational modification (PTM). By exogenous H2S application or endogenous H2S accumulation, NADPH oxidase, LCD, APX, and glyceraldehyde 3-phosphate dehydrogenase (GAPDH) activity upregulation and ACO, NADP-isocitrate dehydrogenase (NADP-ICDH), NADP-malic enzyme (NADP-ME), and CAT activity downregulation have been attributed to persulfidation (52). González-Gordo et al. (53) suggested that the endogenous H2S accumulation regulates the metabolism of plant organelles cytosol, chloroplast, mitochondrion, and peroxisome by persulfidation. Jia et al. (28) reported that the endogenous accumulation of H2S suppresses ethylene biosynthesis by inhibiting the activity of ACO following cysteine persulfidation. Recently, Li et al. (54) reported that the H2S conferred oxidative stress tolerance to tomato plants by persulfidation of ROS scavenging CAT and APX. Therefore, possibly, the endogenous accumulation of H2S suppresses ethylene biosynthesis, due to lower activities of ACS and ACO and a suppressed chlorophyll degradation due to lower activities of MDC and PPH by protein persulfidation. However, this assumption needs to be validated in future studies by employing other methods, such as an improved tag-switch method for the in situ labeling of intracellular persulfides (55).
Conclusion
To sum up, our results demonstrated that the exogenous PSKα application at 150 nM may be employed as a promising procedure for delaying senescence of broccoli floret by promoting endogenous H2S accumulation resulting from higher LCD and DCD gene expressions and enzyme activities giving to suppressing ethylene production resulting from lower ACS1 and ACO1 gene expressions and enzyme activities accompanied by higher chlorophyll accumulation resulting from lower PPH and PaO gene expressions concomitant with lower MDC and PPH enzyme activities during storage at 4°C for 28 days. The present study demonstrated the efficiency of 150 nM PSKα treatment as a preservation procedure for postharvest broccoli to delay floret yellowing, and thus the use of 150 nM PSKα may have broad application prospects. Thus, exogenous PSKα application can be employed as a beneficial strategy for delaying the senescence of broccoli floret during low temperature storage.
Data Availability Statement
The raw data supporting the conclusions of this article will be made available by the authors, without undue reservation.
Author Contributions
MSA conceived the idea and wrote the manuscript. MSA, MA-K, and RK performed the experiments and supported the data analysis. All the authors read, discussed, and approved the manuscript.
Conflict of Interest
The authors declare that the research was conducted in the absence of any commercial or financial relationships that could be construed as a potential conflict of interest.
Acknowledgments
The authors are grateful to Imam Khomeini International University (IKIU) for providing financial support.
Supplementary Material
The Supplementary Material for this article can be found online at: https://www.frontiersin.org/articles/10.3389/fnut.2021.609217/full#supplementary-material
References
1. Duarte-Sierra A, Nadeau F, Angers P, Michaud D, Arul J. UV-C hormesis in broccoli florets: preservation, phyto-compounds and gene expression. Postharvest Biol Technol. (2019) 157:110965. doi: 10.1016/j.postharvbio.2019.110965
2. Shi J, Gao L, Zuo J, Wang Q, Wang Q, Fan L. Exogenous sodium nitroprusside treatment of broccoli florets extends shelf life, enhances antioxidant enzyme activity, and inhibits chlorophyll-degradation. Postharvest Biol Technol. (2016) 116:98–104. doi: 10.1016/j.postharvbio.2016.01.007
3. Silva-Sanzana C, Balic I, Sepúlveda P, Olmedo P, León G, Defilippi BG, et al. Effect of modified atmosphere packaging (MAP) on rachis quality of ‘Red Globe’ table grape variety. Postharvest Biol Technol. (2016) 119:33–40. doi: 10.1016/j.postharvbio.2016.04.021
4. Li D, Li L, Ge Z, Limwachiranon J, Ban Z, Yang D, et al. Effects of hydrogen sulfide on yellowing and energy metabolism in broccoli. Postharvest Biol Technol. (2017) 129:136–42. doi: 10.1016/j.postharvbio.2017.03.017
5. Liu M-S, Li H-C, Lai Y-M, Lo H-F, Chen L-FO. Proteomics and transcriptomics of broccoli subjected to exogenously supplied and transgenic senescence-induced cytokinin for amelioration of postharvest yellowing. J Proteomics. (2013) 93:133–44. doi: 10.1016/j.jprot.2013.05.014
6. Cai J-H, Luo F, Zhao Y-B, Zhou Q, Wei B-D, Zhou X, et al. 24-Epibrassinolide treatment regulates broccoli yellowing during shelf life. Postharvest Biol Technol. (2019) 154:87–95. doi: 10.1016/j.postharvbio.2019.04.019
7. Aiamla-Or S, Shigyo M, Yamauchi N. UV-B treatment controls chlorophyll degradation and related gene expression in broccoli (Brassica oleracea L. Italica Group) florets during storage. Sci Hortic. (2019) 243:524–7. doi: 10.1016/j.scienta.2018.09.009
8. Zheng Q, Zuo J, Gu S, Gao L, Hu W, Wang Q, et al. Putrescine treatment reduces yellowing during senescence of broccoli (Brassica oleracea L. var italica) Postharvest Biol Technol. (2019) 152:29–35. doi: 10.1016/j.postharvbio.2019.02.014
9. Yan Z, Shi J, Gao L, Wang Q, Zuo J. The combined treatment of broccoli florets with kojic acid and calcium chloride maintains post-harvest quality and inhibits off-odor production. Sci Hortic. (2020) 262:109019. doi: 10.1016/j.scienta.2019.109019
10. Xu F, Wang H, Tang Y, Dong S, Qiao X, Chen X, et al. Effect of 1-methylcyclopropene on senescence and sugar metabolism in harvested broccoli florets. Postharvest Biol Technol. (2016) 116:45–9. doi: 10.1016/j.postharvbio.2016.01.004
11. Li S-P, Hu K-D, Hu L-Y, Li Y-H, Jiang A-M, Xiao F, et al. Hydrogen sulfide alleviates postharvest senescence of broccoli by modulating antioxidant defense and senescence-related gene expression. J Agric Food Chem. (2014) 62:1119–29. doi: 10.1021/jf4047122
12. Reyes Jara AM, Gómez-Lobato ME, Civello PM, Martínez GA. Effects of hormonal and physical treatments on the expression of a putative chlorophyll b reductase gene (BoNYC1) during postharvest senescence of broccoli. Postharvest Biol Technol. (2019) 147:107–12. doi: 10.1016/j.postharvbio.2018.09.010
13. Xu D, Zuo J, Fang Y, Yan Z, Shi J, Gao L, et al. Effect of folic acid on the postharvest physiology of broccoli during storage. Food Chem. (2021) 339:127981. doi: 10.1016/j.foodchem.2020.127981
14. Wu C, Cao S, Xie K, Chi Z, Wang J, Wang H, et al. Melatonin delays yellowing of broccoli during storage by regulating chlorophyll catabolism and maintaining chloroplast ultrastructure. Postharvest Biol Technol. (2021) 172:111378. doi: 10.1016/j.postharvbio.2020.111378
15. Sohail M, Wills RBH, Bowyer MC, Pristijono P. Beneficial impact of exogenous arginine, cysteine and methionine on postharvest senescence of broccoli. Food Chem. (2021) 338:128055. doi: 10.1016/j.foodchem.2020.128055
16. Aghdam MS, Sayyari M, Luo Z. Exogenous application of phytosulfokine α (PSKα) delays yellowing and preserves nutritional quality of broccoli florets during cold storage. Food Chemistry. (2020) 333:127481. doi: 10.1016/j.foodchem.2020.127481
17. Aghdam MS, Flores FB. Employing phytosulfokine α (PSKα) for delaying broccoli florets yellowing during cold storage. Food Chem. (2021) 129626. doi: 10.1016/j.foodchem.2021.129626
18. Aghdam MS, Luo Z. Exogenous application of phytosulfokine α (PSKα) delays senescence in broccoli florets during cold storage by ensuring intracellular ATP availability and avoiding intracellular ROS accumulation. Sci Hortic. (2021) 276:109745. doi: 10.1016/j.scienta.2020.109745
19. Huo J, Huang D, Zhang J, Fang H, Wang B, Wang C, et al. Hydrogen sulfide: a gaseous molecule in postharvest freshness. Front Plant Sci. (2018) 9:1172. doi: 10.3389/fpls.2018.01172
20. Ziogas V, Molassiotis A, Fotopoulos V, Tanou G. Hydrogen sulfide: a potent tool in postharvest fruit biology and possible mechanism of action. Front Plant Sci. (2018) 9:1375. doi: 10.3389/fpls.2018.01375
21. Ali S, Nawaz A, Ejaz S, Haider ST-A, Alam MW, Javed HU. Effects of hydrogen sulfide on postharvest physiology of fruits and vegetables: an overview. Sci Hortic. (2019) 243:290–9. doi: 10.1016/j.scienta.2018.08.037
22. Hu H, Liu D, Li P, Shen W. Hydrogen sulfide delays leaf yellowing of stored water spinach (Ipomoea aquatica) during dark-induced senescence by delaying chlorophyll breakdown, maintaining energy status and increasing antioxidative capacity. Postharvest Biol Technol. (2015) 108:8–20. doi: 10.1016/j.postharvbio.2015.05.003
23. Luo Z, Li D, Du R, Mou W. Hydrogen sulfide alleviates chilling injury of banana fruit by enhanced antioxidant system and proline content. Sci Hortic. (2015) 183:144–51. doi: 10.1016/j.scienta.2014.12.021
24. Li D, Limwachiranon J, Li L, Du R, Luo Z. Involvement of energy metabolism to chilling tolerance induced by hydrogen sulfide in cold-stored banana fruit. Food Chem. (2016) 208:272–8. doi: 10.1016/j.foodchem.2016.03.113
25. Módis K, Ju Y, Ahmad A, Untereiner AA, Altaany Z, Wu L, et al. S-Sulfhydration of ATP synthase by hydrogen sulfide stimulates mitochondrial bioenergetics. Pharmacol Res. (2016) 113:116–24. doi: 10.1016/j.phrs.2016.08.023
26. Liu D, Xu S, Hu H, Pan J, Li P, Shen W. Endogenous hydrogen sulfide homeostasis is responsible for the alleviation of senescence of postharvest daylily flower via increasing antioxidant capacity and maintained energy status. J Agric Food Chem. (2017) 65:718–26. doi: 10.1021/acs.jafc.6b04389
27. Aghdam MS, Mahmoudi R, Razavi F, Rabiei V, Soleimani A. Hydrogen sulfide treatment confers chilling tolerance in hawthorn fruit during cold storage by triggering endogenous H2S accumulation, enhancing antioxidant enzymes activity and promoting phenols accumulation. Sci Hortic. (2018) 238:264–71. doi: 10.1016/j.scienta.2018.04.063
28. Jia H, Chen S, Liu D, Liesche J, Shi C, Wang J, et al. Ethylene-induced hydrogen sulfide negatively regulates ethylene biosynthesis by persulfidation of ACO in tomato under osmotic stress. Front Plant Sci. (2018) 9:1517. doi: 10.3389/fpls.2018.01517
29. Jin Z, Sun L, Yang G, Pei Y. Hydrogen sulfide regulates energy production to delay leaf senescence induced by drought stress in arabidopsis. Front Plant Sci. (2018) 9:1722. doi: 10.3389/fpls.2018.01722
30. Munoz-Vargas M A, Gonzalez-Gordo S, Canas A, Lopez-Jaramillo J, Palma JM, Corpas FJ. Endogenous hydrogen sulfide (H2S) is up-regulated during sweet pepper (Capsicum annuum L.) fruit ripening. In vitro analysis shows that NADP-dependent isocitrate dehydrogenase (ICDH) activity is inhibited by H2S and NO. Nitric Oxide. (2018) 81:36–45. doi: 10.1016/j.niox.2018.10.002
31. Sauter M. Phytosulfokine peptide signalling. J Exp Bot. (2015) 66:5161–9. doi: 10.1093/jxb/erv071
32. Kaufmann C, Sauter M. Sulfated plant peptide hormones. J Exp Bot. (2019) 70:4267–77. doi: 10.1093/jxb/erz292
33. Song H, Wang X, Hu W, Yang X, Diao E, Shen T, et al. A cold-induced phytosulfokine peptide is related to the improvement of loquat fruit chilling tolerance. Food Chem. (2017) 232:434–42. doi: 10.1016/j.foodchem.2017.04.045
34. Zhang H, Hu Z, Lei C, Zheng C, Wang J, Shao S, et al. A plant phytosulfokine peptide initiates auxin-dependent immunity through cytosolic Ca2+ signaling in tomato. The Plant Cell. (2018) 30:652–67. doi: 10.1105/tpc.17.00537
35. Aghdam MS, Alikhani-Koupaei M. Exogenous phytosulfokine α (PSKα) applying delays senescence and relief decay in strawberry fruits during cold storage by sufficient intracellular ATP and NADPH availability. Food Chem. (2021) 336:127685. doi: 10.1016/j.foodchem.2020.127685
36. Aghdam MS, Flores FB, Sedaghati B. Exogenous phytosulfokine α (PSKα) application delays senescence and relieves decay in strawberry fruit during cold storage by triggering extracellular ATP signaling and improving ROS scavenging system activity. Sci Hortic. (2021) 279:109906. doi: 10.1016/j.scienta.2021.109906
37. Aghdam MS, Sayyari M, Luo Z. Exogenous phytosulfokine α application delays senescence and promotes antioxidant nutrient accumulation in strawberry fruit during cold storage by triggering endogenous phytosulfokine α signaling. Postharvest Biol Technol. (2021) 175:111473. doi: 10.1016/j.postharvbio.2021.111473
38. Gomez-Lobato ME, Civello PM, Martínez GA. Expression of a lipoxygenase encoding gene (BoLOX1) during postharvest senescence of broccoli. Postharvest Biol Technol. (2012) 64:146–53. doi: 10.1016/j.postharvbio.2011.07.003
39. Fan L, Shi J, Zuo J, Gao L, Lv J, Wang Q. Methyl jasmonate delays postharvest ripening and senescence in the non-climacteric eggplant (Solanum melongena L.) fruit. Postharvest Biol Technol. (2016) 120:76–83. doi: 10.1016/j.postharvbio.2016.05.010
40. Suzuki Y, Asoda T, Matsumoto Y, Terai H, Kato M. Suppression of the expression of genes encoding ethylene biosynthetic enzymes in harvested broccoli with high temperature treatment. Postharvest Biol Technol. (2005) 36:265–71. doi: 10.1016/j.postharvbio.2005.02.005
41. Suzuki Y, Uji T, Terai H. Inhibition of senescence in broccoli florets with ethanol vapor from alcohol powder. Postharvest Biol Technol. (2004) 31:177–82. doi: 10.1016/j.postharvbio.2003.08.002
42. Livak KJ, Schmittgen TD. Analysis of relative gene expression data using real-time quantitative PCR and the 2– ΔΔCT method. Methods. (2001) 25:402–8. doi: 10.1006/meth.2001.1262
43. Hunter DA, Pinkney TT, Watson LM, Trivellini A, Janssen BJ, Brummell DA, et al. Effect of postharvest water deficit stress on gene expression in heads of broccoli (Brassica oleracea var. italica) Postharvest Biol Technol. (2011) 59:113–23. doi: 10.1016/j.postharvbio.2010.09.010
44. Fang H, Luo F, Li P, Zhou Q, Zhou X, Wei B, et al. Potential of jasmonic acid (JA) in accelerating postharvest yellowing of broccoli by promoting its chlorophyll degradation. Food Chem. (2020) 309:125737. doi: 10.1016/j.foodchem.2019.125737
45. Gómez-Lobato ME, Hasperué JH, Civello PM, Chaves AR, Martínez GA. Effect of 1-MCP on the expression of chlorophyll degrading genes during senescence of broccoli (Brassica oleracea L.). Sci Hortic. (2012) 144:208–11. doi: 10.1016/j.scienta.2012.07.017
46. Gómez-Lobato ME, Mansilla SA, Civello PM, Martínez GA. Expression of Stay-Green encoding gene (BoSGR) during postharvest senescence of broccoli. Postharvest Biol Technol. (2014) 95:88–94. doi: 10.1016/j.postharvbio.2014.04.010
47. Chen LFO, Lin CH, Kelkar SM, Chang YM, Shaw JF. Transgenic broccoli (Brassica oleracea var. italica) with antisense chlorophyllase (BoCLH1) delays postharvest yellowing. Plant Sci. (2008) 174:25–31. doi: 10.1016/j.plantsci.2007.09.006
48. Hortensteiner S, Krautler B. Chlorophyll breakdown in higher plants. Biochim Biophys Acta. (2011) 1807:977–88. doi: 10.1016/j.bbabio.2010.12.007
49. Aiamla-Or S, Kaewsuksaeng S, Shigyo M, Yamauchi N. Impact of UV-B irradiation on chlorophyll degradation and chlorophyll-degrading enzyme activities in stored broccoli (Brassica oleracea L. Italica Group) florets. Food Chem. (2010) 120:645–51. doi: 10.1016/j.foodchem.2009.10.056
50. Buchert AM, Civello PM, Martinez GA. Chlorophyllase versus pheophytinase as candidates for chlorophyll dephytilation during senescence of broccoli. J Plant Physiol. (2011) 168:337–43. doi: 10.1016/j.jplph.2010.07.011
51. Aiamla-Or S, Nakajima T, Shigyo M, Yamauchi N. Pheophytinase activity and gene expression of chlorophyll-degrading enzymes relating to UV-B treatment in postharvest broccoli (Brassica oleracea L. Italica Group) florets. Postharvest Biol Technol. (2012) 63:60–6. doi: 10.1016/j.postharvbio.2011.08.003
52. Corpas FJ, Palma JM. H2S signaling in plants and applications in agriculture. J Adv Res. (2020) 24:131–7. doi: 10.1016/j.jare.2020.03.011
53. González-Gordo S, Palma JM, Corpas FJ. Appraisal of H2S metabolism in Arabidopsis thaliana: In silico analysis at the subcellular level. Plant Physiol Biochem. (2020) 155:579–88. doi: 10.1016/j.plaphy.2020.08.014
54. Li J, Shi C, Wang X, Liu C, Ding X, Ma P, et al. Hydrogen sulfide regulates the activity of antioxidant enzymes through persulfidation and improves the resistance of tomato seedling to copper oxide nanoparticles (CuO NPs)-induced oxidative stress. Plant Physiol Biochemi. (2020) 156:257–66. doi: 10.1016/j.plaphy.2020.09.020
Keywords: chlorophyll degradation, ethylene biosynthesis, floret yellowing, hydrogen sulfide, pheophorbide a oxygenase, phytosulfokine α
Citation: Aghdam MS, Alikhani-Koupaei M and Khademian R (2021) Delaying Broccoli Floret Yellowing by Phytosulfokine α Application During Cold Storage. Front. Nutr. 8:609217. doi: 10.3389/fnut.2021.609217
Received: 22 September 2020; Accepted: 03 March 2021;
Published: 01 April 2021.
Edited by:
Tie Liu, University of Florida, United StatesReviewed by:
Tao Feng, Shanghai Institute of Technology, ChinaKarin Albornoz, University of Concepcion, Chile
Copyright © 2021 Aghdam, Alikhani-Koupaei and Khademian. This is an open-access article distributed under the terms of the Creative Commons Attribution License (CC BY). The use, distribution or reproduction in other forums is permitted, provided the original author(s) and the copyright owner(s) are credited and that the original publication in this journal is cited, in accordance with accepted academic practice. No use, distribution or reproduction is permitted which does not comply with these terms.
*Correspondence: Morteza Soleimani Aghdam, c29sZWltYW5pYWdoZGFtQGVuZy5pa2l1LmFjLmly