- Nestlé Purina Research, One Checkerboard Square, St. Louis, MO, United States
Spirulina refers to two species of blue green algae (Arthrospira platensis, and A. maxima) consumed by humans as food for centuries. While, Spirulina has been shown to have immune enhancing properties in several animal and human studies, there are no systematic studies in dogs. The aim of this study was to evaluate the immunomodulatory effect of dietary supplementation with Spirulina in dogs. The study was conducted in two phases: Pre-test (8 wks.) and Test (42 wks.). Thirty adult dogs (mean 2.9 yrs.) were randomized into two groups and fed a nutritionally complete diet in the “Pre-test” phase. At the end of “Pre-test” phase all dogs received a rabies vaccine, and dogs in “test group” were switched to diet supplemented with dried Arthrospira platensis (Spirulina). Response to rabies vaccine was evaluated by Rapid Fluorescent Focus Inhibition Test (RFFIT). Gut immune response was assessed by measuring fecal IgA. Gut microbiota was evaluated by Temporal Temperature Gel Electrophoresis (TTGE) methodology. Repeated measures ANOVA was used to test for differences between groups and statistical significance considered to be p < 0.05. Dogs fed diets supplemented with Spirulina demonstrated enhanced immune status by showing significantly higher vaccine response and higher levels of fecal IgA as compared to the control group. Supplementing diets with Spirulina also resulted in significantly increased gut microbiota stability in the test group. In conclusion, diets supplemented with Spirulina significantly enhanced immune response and gut health in dogs.
Introduction
Spirulina are planktonic blue green algae that grows naturally in warm alkaline lakes in subtropical and tropical areas of America, Mexico, Asia and Central Africa. It has been the traditional diet of some in Africa and Mexico for many centuries (1). There are 3 main Spirulina algae—Spirulina platensis, Spirulina maxima, and Spirulina fusiformis- that have been most regularly investigated for their nutrition and functional properties (2, 3). Although these algae were firstly identified as belonging to the genus Spirulina, they are now accepted to be of the genus Arthrospira, however the name Spirulina has remained for historical reasons. Spirulina has been recognized as having a unique nutritional profile with a very high protein content (60–70% of dry matter content), and being rich in vitamins, minerals, essential fatty acids particularly gamma-linolenic acid, as well as other bioactive components (4). This rich nutritional profile has earned Spirulina an endorsement from both the National Aeronautics and Space Administration (NASA) and the European Space Agency (ESA) as a food suitable for long space missions. Over the last number of years, studies have identified many positive benefits of Spirulina, including immunostimulatory, antioxidant, anti-inflammatory, anti-viral, and anti-bacterial effects (4–6), to name some of the most important. We have published widely in the area of nutritional regulation of immune and gut health in dogs and cats and hypothesized that inclusion of Spirulina to companion animal pet foods could offer many health promoting benefits.
Studies have shown that Spirulina can modulate both cellular and humoral immune responses. In terms of cellular immune responses, there are several reports of Spirulina having a specific action on monocytes and natural killer (NK) cells, components of the innate immune system. In chickens and humans, macrophage phagocytic response was enhanced, and NK cell activity increased, in response to Spirulina administration (7–9). The phagocytic activity of macrophages isolated from cats was also found to be increased in response to antigen exposure in the presence of Spirulina (10). In dogs and mice, a polysaccharide extract of Spirulina platensis could increase white blood cell numbers when the haematopoietic system was damaged by irradiation (11). Mechanistically spray dried Spirulina platensis, orally administered to healthy male volunteers, increases IFN-γ production and phagocytic activity of isolated NK cells stimulated with IL-12/18. In the above study, Spirulina enhanced Toll like receptor (TLR)-2 and 4 mediated production of IL-12 from peripheral blood mononuclear cells, thus indicating Spirulina first activates monocytes and macrophages to produce cytokines that stimulate NK cells (9). An action through TLR-2 but not -4, leading to NF-κB activation, was further suggested in studies in human monocytes (6), while a very recent study has again implicated TLR-4 (12).
Spirulina also modulates humoral immune responses. Treatment of mice with Spirulina for 4 weeks enhanced ex vivo production of IgA from Peyer's patch cells on antigen presentation (13). A polysaccharide extract of Spirulina similarly increased mouse Peyer patch IgA production (6). The authors of this polysaccharide study suggested that the IgA stimulatory effect may have occurred via an increase in numbers of CD11b(+) dendritic cells or via increased IL-6 production (6). Broiler chickens fed a Spirulina supplemented diet demonstrated a higher antibody-specific response against injected sheep red blood cells (SRBC) (injected as an antigen) (7). Antibody production is critical in allergic reactions. Spirulina platensis phycocyanin extract may protect against allergy by suppressing antigen specific IgE and IgG responses and upregulating mucosal IgA response, while suppressing antigen induced small intestine inflammation (14). The above studies clearly indicated that Spirulina has strong potential to improve intestinal humoral immunity and thereby protecting against infection and potentially allergy.
In addition to its immunostimulatory effects, Spirulina is rich in β-carotene and tocopherols, nutrients of proven antioxidant and anti-inflammatory properties. Spirulina has been shown to reduce oxidation in brain, plasma and liver extracts (15). Phycoyanin extracted from Spirulina platensis can act as a free radical scavenger, iron chelator and protects the activity of anti-oxidant enzymes (5, 16). Further in vitro studies demonstrated Spirulina could alleviate oxidative damage associated with the cancer drug Flurouracil (17), as evidence by a reduction in oxidative production of malondialdehyde. Oxidation and inflammation play a key role in many diseases including degenerative diseases. In vivo, Spirulina can reduce markers of brain oxidative damage and reverse age-related increases in proinflammatory cytokines (18). While in a mouse model of Parkinson's disease, a Spirulina enriched diet was found to be neuroprotective (19). These antioxidant and anti-inflammatory effects seem to translate to clinical settings as studies in humans found the oral Spirulina administration helped to elevate symptoms of allergic rhinitis such as nasal congestion and itching (20, 21).
Given its immune enhancing, antioxidant and anti-inflammatory effects, it is not surprising that there is emerging evidence that Spirulina can modulate the gastrointestinal microbiota (22). We have previously investigated modulations of the gut microbiota as a result of dietary supplementation with bovine colostrum extract in both dogs (23) and cats (manuscript under review), and as a result of probiotic supplementation with Enterococcus faecium SF68 in dogs (24). Our investigations clearly demonstrated that dietary modulations are a highly effective route to not only stimulate both intestinal and systemic immune function, but also improve intestinal microbiota stability. In this current study, we evaluated the immune and gut health benefits of supplementing diets with Spirulina in dogs. To our knowledge a study of this type with Spirulina has never been carried out in dogs.
Materials and Methods
Animals and Diets
Thirty adult dogs (Husky crosses, 2–7 years, mean 2.9 years) were used in this study. During an 8-week pre-test period all dogs were fed a commercially available nutritional complete and balanced dog food [Nestlé Purina product: ~29% protein, 36% carbohydrate, 19% fat; 1.4% fiber, 3894 ME Kcal/Kg)] (Supplementary Table 1). At week 0, dogs were vaccinated with a rabies vaccine (IMRAB® 3, Merial Inc, Duluth, GA) as part of their normal veterinary care. At this time point dogs were randomly assigned into two feeding groups (n = 15 per group) based on sex, age, and fecal IgA (used as a marker of immune status). Dogs in the control group continued to be fed the “Control” pre-test diet and the other half fed the “Test diet” which was pre-test diet supplemented with 0.2% spray-dried Spirulina (Arthrospira platensis, Cyanotech Corporation, Kailua-Kona, Hawaii, USA) (Figure 1). Dogs were fed twice per day, once in the morning and once in the evening. Food intake was recorded daily, and body weight was recorded weekly. All dogs were fed their respective diet until the end of the study.
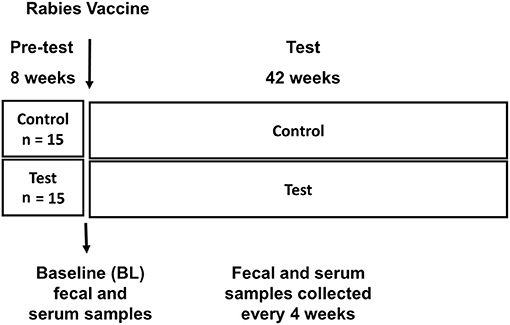
Figure 1. Experimental design: Pre-test phase and Test phase are depicted, along with timing of rabies vaccination, baseline (BL) sample collection, sample collection during test phase.
Dogs were exercised 3 days a week during the 42 week study, using a routine that was been previously described (23). The trial protocol was conducted in strict accordance with the guidelines established by the Nestlé Purina Pet Care (NPPC) Advisory Committee. Jugular or femoral blood samples were collected every 4 weeks from week 0 to week 42 (using BD Vacutainers with sodium citrate as the anticoagulant, Becton & Dickenson) (Figure 1). To obtain plasma, blood samples were centrifuged at 10,000 rpm for 10 min at 6°C and plasma stored at −80°C until assayed for immune markers. Fecal samples were collected every 4 weeks, processed and immediately stored in a −800C freezer (Figure 1). Fecal score was recorded daily and graded using a seven-point scale with a score of 1 representing firm, hard feces and a score of 7 representing liquid diarrhea (Supplementary Table 2). A score of 2 or 3 is considered ideal.
Measurements of Antibodies in Plasma
A Rapid Fluorescent Focus Inhibition Test (RFFIT) was used to measure serum rabies virus neutralizing antibodies. This is a functional assay and measures the ability of antibodies in the serum to neutralize rabies virus, and hence is a good reflection of how effectively the animal would be able to ward off a potential infection with the rabies virus. The test was carried out by the Rabies Laboratory of Kansas State University according to a method previously published (25).
Measurements of Antibodies in Feces
Secretary IgA (sIgA) levels in fecal samples were measured as an indicator of gut-associated lymphoid tissue (GALT) activity using an ELISA method as previously described (23). Briefly, protein was extracted from fecal samples using extraction buffer and the supernatants were collected and frozen at 80°C until assayed for IgA by 96 well ELISA using mouse anti-canine IgA (Serotec, Raleigh, NC) and a secondary antibody of polyclonal goat anti-canine IgA conjugated with HRP (Serotec, Raleigh, NC). Color development was done with 3,3′,5,5′-Tetramethylbenzidine (TMB) peroxidase substrate (KPL, 50-76-00) according to the manufacturer's instructions. Color development was read at 450 nm and results were expressed as μg/ml using a feline IgA standard. Values for fecal IgA were normalized with the total protein content. The total protein content was measured using a BCA Protein Assay Kit (Pierce, 23225).
Measurement of C-Reactive Protein
C-reactive protein (CRP) is an acute phase protein produced by the liver in response to inflammation. It is recognized as a sensitive marker of tissue damage and inflammation. CRP was measured as a general marker of inflammation to confirm that any immune enhancement was not a result of, or did not lead to, a generalized inflammation or non-specific immunostimulation. Serum CRP level was measured in all dogs toward the end of the trial using a canine CRP kit (BD Canine CRP ELISA Kit; BD Bioscience), according to the manufacturer's instructions.
Measurement of the Effect of Spirulina on Gut Microbiota
At week 42, after 2 days of rest, all dogs participated in their standard exercise program. Dogs ran 10 miles in a harness pulling an unladen sled. Each team contain approximately equal numbers of control and Spirulina supplemented dogs. There was no difference in time to complete the task between teams (average 33 min and 30 s). This exercise was used as an inducer of physiological challenge to examine the effect of Spirulina on the gut microbiota stability. Rectal swabs were taken 24 h before (“pre”-samples) then 24 h after (“post”-samples) the exercise protocol. Samples were immediately snap frozen and later microbiota modulations were then assessed by Temporal Temperature Gel Electrophoresis (TTGE) (23). TTGE analysis allows the separation of 16S rRNA gene fragments that have been amplified by PCR and is a commonly used technique to identify microbiota microbial profiles (26) (described below). The “pre” samples collected prior to exercise were used to characterize the dogs baseline species diversity and evenness, as previously described (23). The effect of exercise in each of the dietary groups was assessed by comparing the per cent similarity of the “pre”– and “post”-exercise TTGE profiles. Similarity scores of the Spirulina supplemented group where compared to the control group (Supplementary Table 3).
Temporal Temperature Gel Electrophoresis
TTGE analysis was used to identify modulations in microbial profile of fecal samples in association with dietary supplementation with Spirulina as time points as described above. TTGE analysis was performed on extracted DNA according to the method that has been previously published (23). Gel images were captured and digitized using the FMBIOII (version 1.1) software (Hitachi). Digitized images were analyzed using the GelCompar II (version 2.0) gel analysis software (Applied Math). Band classes were established and band densities (based on height and band surface) within each class were tabulated. Each band class contained all the bands that migrated to the same adjusted location on the gels.
Statistical Analysis
Data are presented as mean ± SEM. Repeated measures ANOVA was carried out using Statistical Analysis Systems statistical software package SAS (SAS Institute, Cary, NC, USA), to test overall differences between groups for all measures. Dunnett's test was used to adjust for multiple comparisons with the control group. For all tests, significance was considered at P < 0.05.
Results
General Physiological Status
At the start of the trial the average weight of the dogs in the test group was 21.6 ± 0.6 kg and in the control group 22.05 ± 0.7 kg. Food intake and body weight did not differ between the two groups during the trial (data not shown). There was no significant difference between control and the Spirulina supplemented diet on all blood chemistry parameters measured (data not shown). No significant difference in fecal scores was also observed between dietary groups (data not shown). Levels of CRP, a marker of inflammation, measured toward the end of trial, were well within the normal range (0.8–16.4 μg/ml) (27) [0.04 ± 0.01 μg/ml for control, and 0.03 ± 0.01 μg/ml in the Spirulina supplemented group at end of trial].
Immune Response in the Gut
Fecal secretory IgA levels were analyzed by ELISA. Repeated measures ANOVA demonstrated a significant effect of diet on levels of sIgA (P < 0.05) (Figure 2). Fecal IgA levels were significantly increased at weeks 9, 30, and 42 in the Spirulina fed group compared to the control group (P < 0.05) (Figure 2).
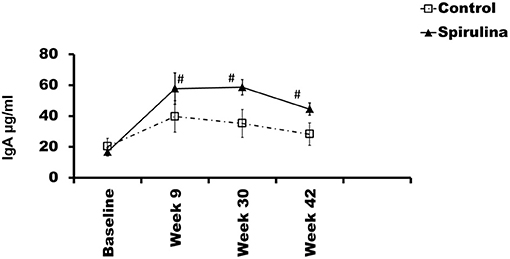
Figure 2. GALT response: Changes in fecal IgA in each group during the trial are depicted as mean fecal IgA values ± SEM. X-axis depicts time in weeks and Y-axis depicts mean fecal IgA values in μg/ml. #p < 0.05.
Response to Rabies Virus Vaccine
All dogs received a rabies vaccination at week 0. The vaccine is commercially available, approved for use in dogs, routinely used in veterinary practice, and was chosen in consultation with the veterinarian. Repeated measures ANOVA demonstrated a significant effect of diet on antibody response (P < 0.05). In comparison to the control diet, the Spirulina supplemented group had significantly higher antibody levels at weeks 9, 15, and 30 (Figure 3, P < 0.05).
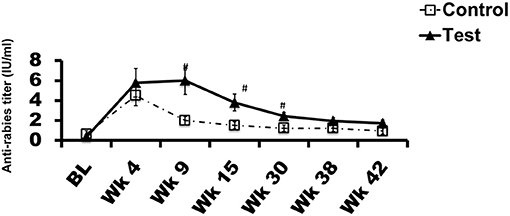
Figure 3. Rabies virus vaccine response: Antibody level ± SEM in each group during the trial is plotted. X-axis represents time in weeks and Y-axis represents anti-rabies virus titers in IU/ml. #p < 0.05. BL denotes baseline value.
Effects of Spirulina on Gut Microbiota
Using TTGE microbial profiling, dogs' gut microbiota patterns were compared before and after exercise. Exercising induces a certain amount of physiological stress on the dogs. When the “pre”-exercise microbiota pattern (collected 24 h before exercise) was compared with the “post”-exercise microbiota pattern, dogs fed the test diet supplemented with Spirulina had increased gut microbiota stability as evidence by increased similarity in the “pre” and “post” banding pattern 77.8 ± 2.3 vs. 48.6 ± 3.0% compared to the control group, P < 0.05).
Discussion
In this study we have demonstrated that 0.2% Spirulina platensis powder supplemented in the diet of dogs was associated with significantly enhanced immune and gut health. Spirulina increased fecal IgA levels after just 9 weeks of feeding, and this effect was maintained throughout the study. Gut microbiota maintained enhanced stability following a challenging exercise in the Spirulina fed dogs as compared to the control group. Systemic immune responsiveness was found to be fortified with Spirulina feeding leading to a faster and stronger induction of rabies vaccine titer. Together these results suggest that Spirulina fed dogs have a healthier and more robust immune system.
We previously demonstrated that increased fecal sIgA levels could be induced with the dietary inclusion of a immunomodulating agents such as bovine colostrum (23) or the lactic acid probiotic Enterococcus faecium SF68 (24). We now extend this finding to Spirulina platensis and provide further evidence of the health benefits of immunonutrition for companion animals. IgA is the most abundant immunoglobulin produced by the GALT, and increased fecal sIgA content is indicative of increased GALT activity (28). IgA plays an important role in defending against pathogenic infection, in preventing antigens from entering the epithelium, and in the selection and maintenance of colonizing bacteria (29). Intestinal IgA's primary role is to maintain intestinal homeostasis and to protect mucosal membranes from infection by pathogenic microorganisms and enteric toxins by inducing a process known as immune exclusion (30). IgA maintains immune exclusion through a number of ways, for example, by binding to antigens trapping them in the mucus and preventing their binding to cell surface receptors, and by reducing bacterial virulence (31). In dogs, a reduction in fecal sIgA has been shown to be associated with some chronic enteropathies (32, 33), suggesting fecal sIgA level is an indicator of intestinal immune health. At a mechanistic level, it is known that Spirulina can have a direct action on intestinal immune cell function and one mechanism explored includes the activation of intestinally located TLR's (6, 9). A previous study has demonstrated that constitutive activity of TLR-4 in the intestinal epithelium of mice lead to B cell recruitment, their switching to IgA+ cells, and consequently increased fecal IgA levels (34).
Previous studies have also shown that Spirulina or extracts of Spirulina could enhance gut IgA levels (13, 14). In mice orally administered shrimp extract as an antigen, intestinal IgA levels were shown to be increased in Spirulina as compared to control fed mice (13). In another study, ovalbumin was administered to mice to induce antigen-specific antibodies in their lymphoid tissues. This induced both antigen specific IgA and total IgA in the Peyer's patch however, the effect was significantly enhanced in an ovalbumin and phycocyanin combined group compared to ovalbumin alone. In the serum, phycocyanin prevented the antigen induced increase in IgG1 and IgE levels, and suppressed ovalbumin induced inflammation of the intestine (14). The authors of this mouse study proposed that phycocyanin, extracted from Spirulina platanis, may reduce allergic inflammation by supressing antigen specific IgE and augmenting intestinal IgA production. The production of antigen specific sIgA from IgA-secreting cells in Peyer's patches, and accompanying higher fecal sIgA levels, has been suggested to be a mechanism for the development of food tolerance (35). Therefore, by supporting intestinal immune function, Spirulina, may not only help reduce opportunistic infections but may also help prevent food intolerance. In our current study, fecal sIgA levels were increased after just 2 months of feeding Spirulina and the positive enhancement was maintained to the end of the 42-week study. Interestingly in a human study of oral Spirulina administration, immunostimulatory effects of Spirulina were evident up to 4 weeks after the end of administration (9), indicating the dogs included in this study could have continued gut health benefits even after the end of the trial. In this study, Hirahashi et al., demonstrated that spirulina likely acts monocytes to induce IL-12, which in turn drives NK cells to produce IFN gamma and activate downstream T cell function.
The health of the immune system can be evaluated by how subjects respond to exercise (23), as exercise can temporally lower immune status (36). The intestinal microbiota is dynamic and temporal variations of content occur over time in response to life events such as stress, age, illness, and even exercise (37). We have previously shown that a more stable gut microbiota can resist exercise induced changes in gut microbiota (23). Like our previous study, we were also interested to determine if Spirulina could improve gut microbiota stability. At 42 weeks, all dogs participated in an exercise protocol involving a 2-day rest period followed by a 10-mile sled run. The Spirulina fed group had greater gut microbiota stability following exercise compared to the control group (P < 0.05), as measured by a greater degree of microbiota similarity before and after exercise. Although we did not determine which specific species of gut microbes were modulated by Spirulina, a previous in vitro study has shown that Spirulina can increase the growth of lactic acid bacteria (38). Lactic acid bacteria are normal residents of canine gut microbiota (39) and lactic acid probiotics have a safe and effective history in dogs (24, 40). By contrast, gut microbiota species diversity was not modulated by Spirulina supplementation, which is in line with the only other previous study that examined the effect of Spirulina platensis on the gut microbiota and were mice similarly showed no change in gut microbiota diversity (22). Interestingly, Spirulina has also previously been shown to prevent oxidative damage to skeletal muscle normally associated with exercise in untrained subjects (41), which together with this current finding indicates Spirulina is of significant benefit to negate the negative impacts of high endurance activity.
It is currently unclear through which mechanism Spirulina would influence the gut microbiota, however, the overall improvement in gut microbiota stability is likely to be connected its improvement gut immune status. The gut microbiota and gut immune system are critically interlinked. For example, the intestinal microbiota increases the proliferation of IgA synthesizing plasma cells in the intestine (42), while mucosal IgA enhances the homeostasis of gut commensal microbiota (43). Thus, beneficial effects of Spirulina on gut immunity will reciprocate on the gut microbiota, and vice versa. Marine algae are known to produce soluble polysaccharides which escape conventional digestion and are fermented by the gut microbiota (44). Extracts of Spirulina platensis have been shown to stimulate lactic acid bacteria growth (38) and can increase probiotic yields in a dairy products (45), thus indicating soluble polysaccharides of Spirulina may similarly escape conventional digestion, but rather reach the colon and provide substrates for microbiota growth.
Here we vaccinated the dogs with a rabies vaccine just prior to them starting the dietary trial with Spirulina. Dogs in both the control and Spirulina dietary groups mounted an immune response to the vaccination as evidenced by the production of rabies vaccine specific antibodies. However, the response antibody response was faster and stronger in the Spirulina group (P < 0.05). Similarly, one previous study in mice demonstrated an increased tetanus toxoid vaccine response 21 days post vaccination, in mice orally administered with Spirulina powder for 7 days prior to, and thereafter, vaccination compared to control mice who did not receive Spirulina (46). We have previously used co-incident vaccination protocols with dietary manipulations to understand how a given diet could influence systemic immunity as vaccine responses are a relevant biomarkers of an immunological response to challenge (23, 24), and can be interpreted as a surrogate marker of a typical immune response to infection (47). A poor response to vaccination can lead to higher rates of clinical illness (48, 49). Here Spirulina supplemented dogs are likely to have a better resilience to infection through the strengthening of their immune system. However, the normal CRP levels found in both control and Spirulina supplemented dogs clearly demonstrates that there was no overt pro-inflammatory response occurring, indicating the enhancement of immune functioning occurs in a targeted and challenge specific manner.
In conclusion, this study demonstrates for the first time that Spirulina can regulate the mucosal and systemic immune response of dogs. Gut microbiota stability is also regulated by its supplementation. This study further supports the use of functional ingredients in the diets of companion animals.
Data Availability Statement
The original contributions presented in the study are included in the article/Supplementary Material, further inquiries can be directed to the corresponding author/s.
Ethics Statement
The animal study was reviewed and approved by Nestlé Purina Pet Care (NPPC) Advisory Committee.
Author Contributions
ES and AR designed the study, interpreted the results, and prepared the manuscript. RE, PS, and JL carried out the assays described in the study. ES and AR had primary responsibility for final content. All authors read and approved the final manuscript.
Conflict of Interest
All authors are employed by Nestle Purina Research which funded the study in full.
Acknowledgments
The authors wish to acknowledge Julie Spears for help with analyzing the microbiota data, Wendell Kerr for statistical analysis, and Lisa Conboy-Schmidt for assistance in compiling the manuscript.
Supplementary Material
The Supplementary Material for this article can be found online at: https://www.frontiersin.org/articles/10.3389/fnut.2021.667072/full#supplementary-material
Abbreviations
GALT, gut-associated lymphoid tissue; TTGE, temporal temperature gel electrophoresis.
References
1. Khan Z, Bhadouria P, Bisen PS. Nutritional and therapeutic potential of Spirulina. Curr Pharm Biotechnol. (2005) 6:373–9. doi: 10.2174/138920105774370607
2. Karkos PD, Leong SC, Karkos CD, Sivaji N, Assimakopoulos DA. Spirulina in clinical practice: evidence-based human applications. Evid Based Complement Alternat Med. (2011) 2011:531053. doi: 10.1093/ecam/nen058
3. Khan M, Shobha JC, Mohan IK, Naidu MU, Sundaram C, Singh S, et al. Protective effect of Spirulina against doxorubicin-induced cardiotoxicity. Phytother Res. (2005) 19:1030–7. doi: 10.1002/ptr.1783
4. Deng R, Chow TJ. Hypolipidemic, antioxidant, and antiinflammatory activities of microalgae Spirulina. Cardiovasc Ther. (2010) 28:e33–45. doi: 10.1111/j.1755-5922.2010.00200.x
5. Romay C, Armesto J, Remirez D, Gonzalez R, Ledon N, Garcia I. Antioxidant and anti-inflammatory properties of C-phycocyanin from blue-green algae. Inflamm Res. (1998) 47:36–41. doi: 10.1007/s000110050256
6. Balachandran P, Pugh ND, Ma G, Pasco DS. Toll-like receptor 2-dependent activation of monocytes by Spirulina polysaccharide and its immune enhancing action in mice. Int Immunopharmacol. (2006) 6:1808–14. doi: 10.1016/j.intimp.2006.08.001
7. Qureshi MA, Garlich JD, Kidd MT. Dietary Spirulina platensis enhances humoral and cell-mediated immune functions in chickens. Immunopharmacol Immunotoxicol. (1996) 18:465–76. doi: 10.3109/08923979609052748
8. Al-Batshan HA, Al-Mufarrej SI, Al-Homaidan AA, Qureshi MA. Enhancement of chicken macrophage phagocytic function and nitrite production by dietary Spirulina platensis. Immunopharmacol Immunotoxicol. (2001) 23:281–9. doi: 10.1081/IPH-100103866
9. Hirahashi T, Matsumoto M, Hazeki K, Saeki Y, Ui M, Seya T. Activation of the human innate immune system by Spirulina: augmentation of interferon production and NK cytotoxicity by oral administration of hot water extract of Spirulina platensis. Int Immunopharmacol. (2002) 2:423–34. doi: 10.1016/S1567-5769(01)00166-7
10. Qureshi MA, Ali RA. Spirulina platensis exposure enhances macrophage phagocytic function in cats. Immunopharmacol Immunotoxicol. (1996) 18:457–63. doi: 10.3109/08923979609052747
11. Zhang HQ, Lin AP, Sun Y, Deng YM. Chemo- and radio-protective effects of polysaccharide of Spirulina platensis on hemopoietic system of mice and dogs. Acta Pharmacol Sin. (2001) 22:1121–4.
12. Kawanishi Y, Tominaga A, Okuyama H, Fukuoka S, Taguchi T, Kusumoto Y, et al. Regulatory effects of Spirulina complex polysaccharides on growth of murine RSV-M glioma cells through Toll-like receptor 4. Microbiol Immunol. (2013) 57:63–73. doi: 10.1111/1348-0421.12001
13. Hayashi O, Hirahashi T, Katoh T, Miyajima H, Hirano T, Okuwaki Y. Class specific influence of dietary Spirulina platensis on antibody production in mice. J Nutr Sci Vitaminol. (1998) 44:841–51. doi: 10.3177/jnsv.44.841
14. Nemoto-Kawamura C, Hirahashi T, Nagai T, Yamada H, Katoh T, Hayashi O. Phycocyanin enhances secretary IgA antibody response and suppresses allergic IgE antibody response in mice immunized with antigen-entrapped biodegradable microparticles. J Nutr Sci Vitaminol. (2004) 50:129–36. doi: 10.3177/jnsv.50.129
15. Miranda MS, Cintra RG, Barros SB, Mancini FJ. Antioxidant activity of the microalga Spirulina maxima. Braz J Med Biol Res. (1998) 31:1075–9. doi: 10.1590/S0100-879X1998000800007
16. Bermejo-Bescos P, Pinero-Estrada E, Villar del Fresno AM. Neuroprotection by Spirulina platensis protean extract and phycocyanin against iron-induced toxicity in SH-SY5Y neuroblastoma cells. Toxicol In Vitro. (2008) 22:1496–502. doi: 10.1016/j.tiv.2008.05.004
17. Ray S, Roy K, Sengupta C. In vitro evaluation of protective effects of ascorbic acid and water extract of Spirulina plantesis (blue green algae) on 5-fluorouracil-induced lipid peroxidation. Acta Pol Pharm. (2007) 64:335–44.
18. Gemma C, Mesches MH, Sepesi B, Choo K, Holmes DB, Bickford PC. Diets enriched in foods with high antioxidant activity reverse age-induced decreases in cerebellar beta-adrenergic function and increases in proinflammatory cytokines. J Neurosci. (2002) 22:6114–20. doi: 10.1523/JNEUROSCI.22-14-06114.2002
19. Pabon MM, Jernberg JN, Morganti J, Contreras J, Hudson CE, Klein RL, et al. A spirulina-enhanced diet provides neuroprotection in an alpha-synuclein model of Parkinson's disease. PLoS ONE. (2012) 7:e45256. doi: 10.1371/journal.pone.0045256
20. Mao TK, Van de Water J, Gershwin ME. Effects of a Spirulina-based dietary supplement on cytokine production from allergic rhinitis patients. J Med Food. (2005) 8:27–30. doi: 10.1089/jmf.2005.8.27
21. Cingi C, Conk-Dalay M, Cakli H, Bal C. The effects of spirulina on allergic rhinitis. Eur Arch Otorhinolaryngol. (2008) 265:1219–23. doi: 10.1007/s00405-008-0642-8
22. Rasmussen HE, Martinez I, Lee JY, Walter J. Alteration of the gastrointestinal microbiota of mice by edible blue-green algae. J Appl Microbiol. (2009) 107:1108–18. doi: 10.1111/j.1365-2672.2009.04288.x
23. Satyaraj E, Reynolds A, Pelker R, Labuda J, Zhang P, Sun P. Supplementation of diets with bovine colostrum influences immune function in dogs. Br J Nutr. (2013) 110:2216–21. doi: 10.1017/S000711451300175X
24. Benyacoub J, Czarnecki-Maulden GL, Cavadini C, Sauthier T, Anderson RE, Schiffrin EJ, et al. Supplementation of food with Enterococcus faecium (SF68) stimulates immune functions in young dogs. J Nutr. (2003) 133:1158–62. doi: 10.1093/jn/133.4.1158
25. Briggs DJ, Smith JS, Mueller FL, Schwenke J, Davis RD, Gordon CR, et al. A comparison of two serological methods for detecting the immune response after rabies vaccination in dogs and cats being exported to rabies-free areas. Biologicals. (1998) 26:347–55. doi: 10.1006/biol.1998.0162
26. Tsai YL, Olson BH. Rapid method for separation of bacterial DNA from humic substances in sediments for polymerase chain reaction. Appl Environ Microbiol. (1992) 58:2292–5. doi: 10.1128/AEM.58.7.2292-2295.1992
27. Otabe K, Sugimoto T, Jinbo T, Honda M, Kitao S, Hayashi S, et al. Physiological levels of C-reactive protein in normal canine sera. Vet Res Commun. (1998) 22:77–85. doi: 10.1023/A:1006071211779
28. Salminen S, Bouley C, Boutron-Ruault MC, Cummings JH, Franck A, Gibson GR, et al. Functional food science and gastrointestinal physiology and function. Br J Nutr. (1998) 1:S147–71. doi: 10.1079/BJN19980108
29. Suzuki K, Kawamoto S, Maruya M, Fagarasan S. GALT: organization and dynamics leading to IgA synthesis. Adv Immunol. (2010) 107:153–85. doi: 10.1016/B978-0-12-381300-8.00006-X
30. Mantis NJ, Rol N, Corthesy B. Secretory IgA's complex roles in immunity and mucosal homeostasis in the gut. Mucosal Immunol. (2011) 4:603–11. doi: 10.1038/mi.2011.41
31. Pabst O. New concepts in the generation and functions of IgA. Nat Rev Immunol. (2012) 12:821–32. doi: 10.1038/nri3322
32. Littler RM, Batt RM, Lloyd DH. Total and relative deficiency of gut mucosal IgA in German shepherd dogs demonstrated by faecal analysis. Vet Rec. (2006) 158:334–41. doi: 10.1136/vr.158.10.334
33. Maeda S, Ohno K, Uchida K, Nakashima K, Fukushima K, Tsukamoto A, et al. Decreased immunoglobulin A concentrations in feces, duodenum, and peripheral blood mononuclear cells of dogs with inflammatory bowel disease. J Vet Intern Med. (2013) 27:47–55. doi: 10.1111/jvim.12023
34. Shang L, Fukata M, Thirunarayanan N, Martin AP, Arnaboldi P, Maussang D, et al. Toll-like receptor signaling in small intestinal epithelium promotes B-cell recruitment and IgA production in lamina propria. Gastroenterology. (2008) 135:529–38. doi: 10.1053/j.gastro.2008.04.020
35. Frossard CP, Hauser C, Eigenmann PA. Antigen-specific secretory IgA antibodies in the gut are decreased in a mouse model of food allergy. J Allergy Clin Immunol. (2004) 114:377–82. doi: 10.1016/j.jaci.2004.03.040
36. Romeo J, Jimenez-Pavon D, Cervantes-Borunda M, Warnberg J, Gomez-Martinez S, Castillo MJ, et al. Immunological changes after a single bout of moderate-intensity exercise in a hot environment. J Physiol Biochem. (2008) 64:197–204. doi: 10.1007/BF03178842
37. Matsumoto M, Inoue R, Tsukahara T, Ushida K, Chiji H, Matsubara N, et al. Voluntary running exercise alters microbiota composition and increases n-butyrate concentration in the rat cecum. Biosci Biotechnol Biochem. (2008) 72:572–6. doi: 10.1271/bbb.70474
38. Parada JL, Zulpa de CG, Zaccaro de Mule MC, Storni de Cano MM. Lactic acid bacteria growth promoters from Spirulina platensis. Int J Food Microbiol. (1998) 45:225–8. doi: 10.1016/S0168-1605(98)00151-2
39. Handl S, Dowd SE, Garcia-Mazcorro JF, Steiner JM, Suchodolski JS. Massive parallel 16S rRNA gene pyrosequencing reveals highly diverse fecal bacterial and fungal communities in healthy dogs and cats. FEMS Microbiol Ecol. (2011) 76:301–10. doi: 10.1111/j.1574-6941.2011.01058.x
40. Vahjen W, Manner K. The effect of a probiotic Enterococcus faecium product in diets of healthy dogs on bacteriological counts of Salmonella spp., Campylobacter spp. and Clostridium spp. in faeces. Arch Tierernahr. (2003) 57:229–33. doi: 10.1080/0003942031000136657
41. Lu HK, Hsieh CC, Hsu JJ, Yang YK, Chou HN. Preventive effects of Spirulina platensis on skeletal muscle damage under exercise-induced oxidative stress. Eur J Appl Physiol. (2006) 98:220–6. doi: 10.1007/s00421-006-0263-0
42. Crabbe PA, Bazin H, Eyssen H, Heremans JF. The normal microbial flora as a major stimulus for proliferation of plasma cells synthesizing IgA in the gut. The germ-free intestinal tract. Int Arch Allergy Appl Immunol. (1968) 34:362–75. doi: 10.1159/000230130
43. Suzuki K, Meek B, Doi Y, Muramatsu M, Chiba T, Honjo T, et al. Aberrant expansion of segmented filamentous bacteria in IgA-deficient gut. Proc Natl Acad Sci USA. (2004) 101:1981–6. doi: 10.1073/pnas.0307317101
44. Michel C, Macfarlane GT. Digestive fates of soluble polysaccharides from marine macroalgae: involvement of the colonic microflora and physiological consequences for the host. J Appl Bacteriol. (1996) 80:349–69. doi: 10.1111/j.1365-2672.1996.tb03230.x
45. Varga L, Szigeti J, Kovacs R, Foldes T, Buti S. Influence of a Spirulina platensis biomass on the microflora of fermented ABT milks during storage (R1). J Dairy Sci. (2002) 85:1031–8. doi: 10.3168/jds.S0022-0302(02)74163-5
46. Chu WL, Quynh l, V, Radhakrishnan AK. Effect of Spirulina (Arthrospira) supplementation on the immune response to tetanus toxoid vaccination in a mouse model. J Diet Suppl. (2013) 10:229–40. doi: 10.3109/19390211.2013.822452
47. Satyaraj E. Emerging paradigms in immunonutrition. Top Companion Anim Med. (2011) 26:25–32. doi: 10.1053/j.tcam.2011.01.004
48. Burns EA, Lum LG, Seigneuret MC, Giddings BR, Goodwin JS. Decreased specific antibody synthesis in old adults: decreased potency of antigen-specific B cells with aging. Mech Ageing Dev. (1990) 53:229–241. doi: 10.1016/0047-6374(90)90041-D
Keywords: Spirulina, Spirulina (Arthrospira) platensis, nutritional immunology, immunity, gut health and function, dog
Citation: Satyaraj E, Reynolds A, Engler R, Labuda J and Sun P (2021) Supplementation of Diets With Spirulina Influences Immune and Gut Function in Dogs. Front. Nutr. 8:667072. doi: 10.3389/fnut.2021.667072
Received: 11 February 2021; Accepted: 03 May 2021;
Published: 28 May 2021.
Edited by:
Reinaldo B. Oria, Federal University of Ceara, BrazilReviewed by:
Marie Van Der Merwe, University of Memphis, United StatesJunpeng Wang, Henan University, China
Copyright © 2021 Satyaraj, Reynolds, Engler, Labuda and Sun. This is an open-access article distributed under the terms of the Creative Commons Attribution License (CC BY). The use, distribution or reproduction in other forums is permitted, provided the original author(s) and the copyright owner(s) are credited and that the original publication in this journal is cited, in accordance with accepted academic practice. No use, distribution or reproduction is permitted which does not comply with these terms.
*Correspondence: Ebenezer Satyaraj, ZWJlbmV6ZXIuc2F0eWFyYWpAcmQubmVzdGxlLmNvbQ==