- 1Graduate Program in Health and Development in the Central-West Region, Federal University of Mato Grosso do Sul, Campo Grande, Brazil
- 2Spectroscopy and Bioinformatics Applied Biodiversity and Health - GEBABS, Federal University of Mato Grosso do Sul, Campo Grande, Brazil
- 3Graduate Program in Materials Science, Federal University of Mato Grosso do Sul, Campo Grande, Brazil
- 4Graduate Program in Biotechnology and Biodiversity in the Central-West Region, Federal University of Mato Grosso do Sul, Campo Grande, Brazil
- 5Medical School, Federal University of Mato Grosso do Sul, Campo Grande, Brazil
- 6Graduate Program in Biotechnology, S-Inova Biotech, Catholic University Dom Bosco-UCDB, Campo Grande, Brazil
Obesity is associated with the leading causes of death in the worldwide. On the other hand, the intake of vegetables, fruits and fish is related to the reduction of obesity and other metabolic syndromes. This review aims to highlight the role of ingestion of polyphenols and omega-3 polyunsaturated fatty acids (ω-3 PUFAs) in reducing obesity and related metabolic diseases (RMDs). The consumption of vegetables, fish and by-products rich in polyphenols and α-linolenic acid (ALA), as well as oils rich in eicosapentaenoic acid (EPA) and docosahexaenoic acid (DHA) are associated with a decrease in obesity and its RMDs in consumers. Furthermore, we discussed the adequate amount of extracts, powder, polyphenols, ω-3 PUFAs administrated in animal models and human subjects, and the relevant outcomes obtained. Thus, we appeal to the research institutions and departments of the Ministries of Health in each country to develop a food education joint project to help schools, businesses and families with the aim of reducing obesity and other metabolic diseases.
Introduction
Obesity is an abnormal accumulation of fat in cells that interferes with the maintenance of an individual's health. It is a chronic disease characterized by lower amounts of energy expenditure than ingestion, leading to body weight gain over time due to excessive increase in adipose tissue mass (1), triggering pro-inflammatory agents (2). Furthermore, obesity is linked with several diseases such as insulin resistance, systematic inflammation, diabetes mellitus (DM), hypertension, coronary heart diseases (CHD), adipocyte hypertrophy, non-alcoholic fatty liver disease (NAFLD), and others (3, 4). Weight can be calculated from the mathematical formula of the body mass index (BMI = mass/height × height), being considered overweight that can progress to obesity when BMI ≥ 25 and ≥ 30 kg/m2 (1, 2). In the adult population, the occurrence of obesity and overweight is 39 and 50%, and it is mainly explained by the easy access to high-calorie foods (fast food) and sedentary lifestyle (5).
Systemic complications in obese patients are associated with increased abdominal fat, severe organ and tissue failure due to an increased pro-inflammatory cytokine storm, lipopolysaccharide and oxidative stress conditions (6). In addition, several studies have reported a decrease in obesity and its RMDs due to consumption of vegetables (leaves, seeds, nuts, fruits, vegetable oils, by-products) and fish (mainly marine fish, oils, by-products) rich in polyphenols and ω-3 PUFAs: ALA, EPA, and DHA (7–13). Furthermore, obesity and its RMDs lowering can be explained by consequence of synergistic actions of polyphenols and ω-3 PUFAs improving several metabolic health pathways (14, 15). Due to the synergistic actions of the polyphenols and ω-3 PUFAs, some products that are found, like fish and vegetables, and their by-products can potentially improve and control obesity and its RMDs as anti-glucose tolerance, anti-oxidative, anti-atherosclerosis, anti-inflammation, anti-weight gain, hepato-protective, vascular-protective, cardiovascular-protective, anti-hypertension, anti-diabetic effects, thus improving the human health (13, 16–21). The beneficial effects of foods that contain polyphenols, ALA, EPA and DHA in their composition are summarized in Figure 1.
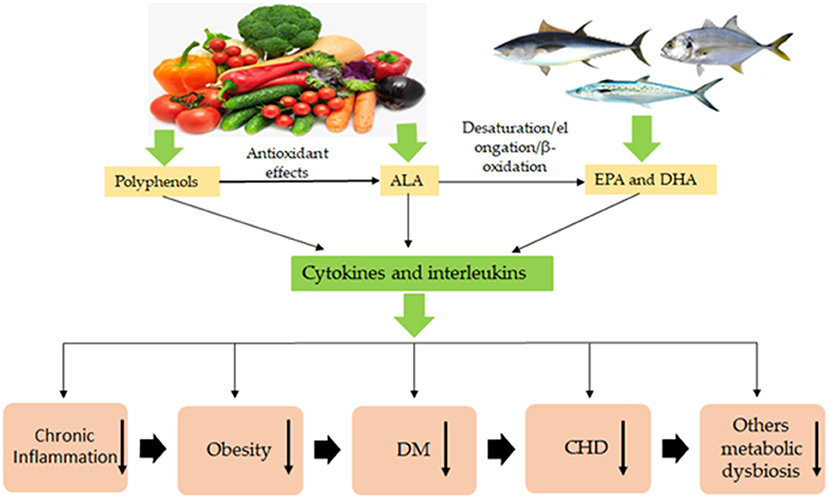
Figure 1. Overview of polyphenols, α-linolenic (ALA), eicosapentaenoic (EPA), and docosahexaenoic acids (DHA) natural sources. The polyphenol compounds obtained from vegetables are active natural antioxidants, which slow up or reduce the high speed of degradation of ALA, EPA, and DHA, quenching singlet oxygen and reacting or eliminating the free radicals, prolong the half-life of these acids during their storing and confection of food. The ingestion of polyphenols, ALA, EPA, and DHA in natural conditions prevent obesity and its related metabolic diseases, including these presented in the scheme. However, the benefit does not occur when polyphenols, ALA, EPA, and DHA are denaturated during the extraction process, storage, and food confection. Through the biosynthesis processes with the actions of enzymes, ALA is converted to EPA and DHA. The synergistic effects of polyphenols, EPA and DHA in the body promote health with preventing and reducing obesity and its related diseases for the consumers. ↓, significant decrease; DM, diabetes mellitus; CHD, cardiovascular heart diseases.
However, despite the reported benefit of polyphenols and ω-3 PUFAs reported, obesity and its RMDs high incidence can be correlated with inadequate food intake (22), the lower cost of unhealthy food acquisition (23) and cultural behaviors barriers (24) allied to unfavorable educational programs impact negatively on healthy food acquisition (25).
In this review, we aimed to emphasize the benefit of polyphenols and ω-3 PUFAs regular intake and their sources and to propose joint actions allied to consumer's behavior change for reducing obesity and its RMDs (systematic inflammation, cardiovascular diseases, hypertension, diabetes mellitus, high insulin level, metabolic syndrome, and others).
The Main Polyphenols Sources
Vegetables, fruits, seeds, almonds, and cereals are widely known in diets and supplementations for their enormous benefits on health improving, preventing, and reducing obesity and its RMDs (9, 13). Health benefits are associated with the effect of bioactive substances, mainly represented by compounds with antioxidant action that are responsible for functions such as the half-life of products and their by-products (residue products as peel, pulp and seed) (12, 16, 26–30). The main polyphenol substances occurs in leaves, flowers, roots, bulbs, and rhizomes of several wild edible plants (31, 32). In addition, polyphenol is present in fruits as apple, grapes, pear, cherries, berries, coffee, cereals and chocolate (33), citrus, mangoes, garlic, onions (34), tomatoes, potatoes, carrots, leaves (tea), and vegetables (broccoli, cabbages, pumpkin, spinach, and lettuce). In addition, these plants (35) are natural sources of anthocyanins and stilbenes (resveratrol and piceatannol) (26), catechin, quercetin, kaempferol (27), umbelliferone, epicatechin, phenolic acids (gallic, ellagic, chlorogenic, caffeic, and coumaric) (34), hydroxytyrosol, tyrosol (35), curcumin, rutin, chrysin (36), myricetin, isorhamnetin, hesperidin, narirutin, naringin, apigenin, luteolin, pelargonidin, cyanidin, delphinidin, genistein, daidzein (37), ellagitannins, and others (38). The effects of vegetables, fruits and polyphenols on obesity and its RMDs in animal models are summarized in Table 1.
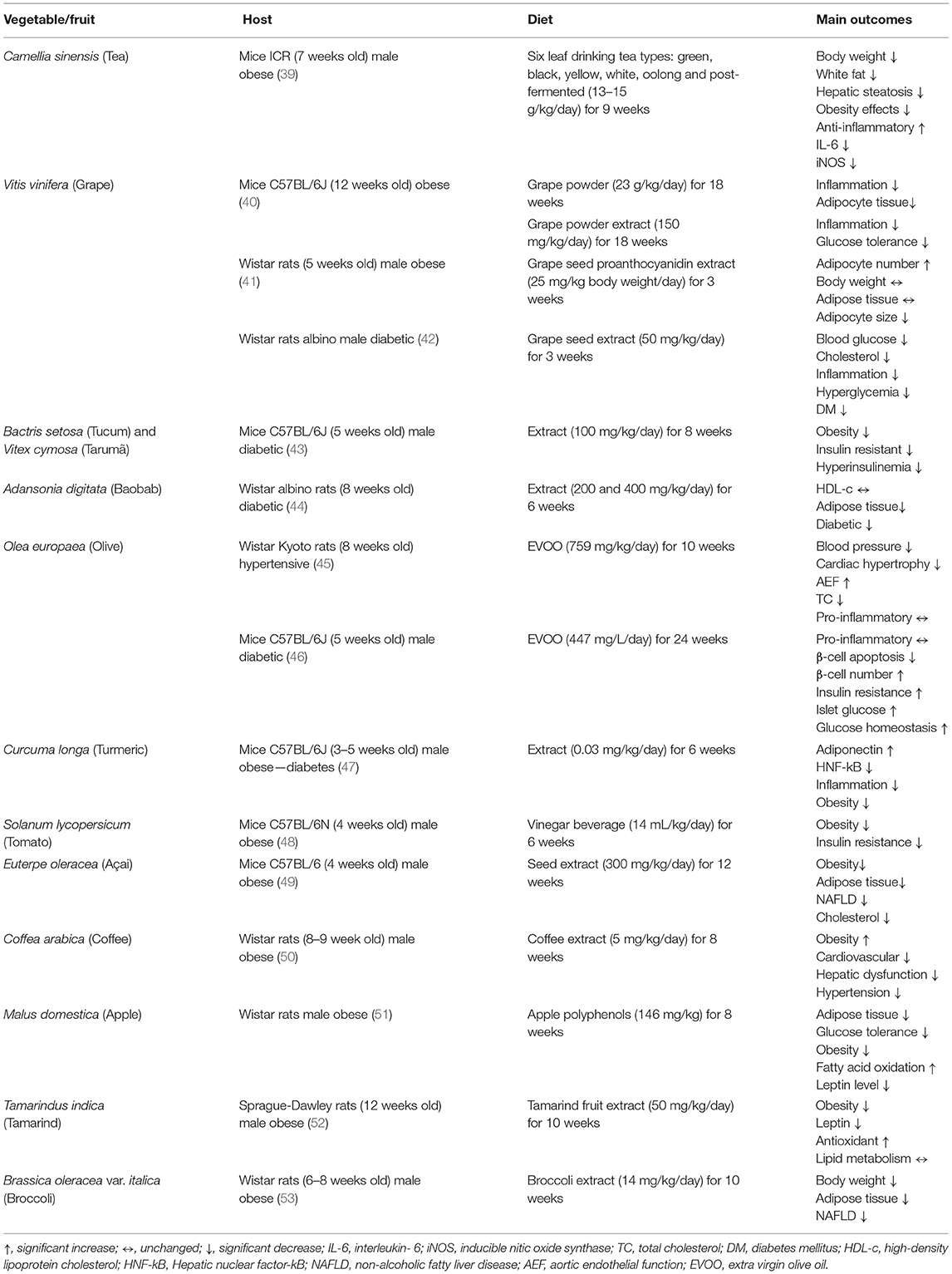
Table 1. Effects of polyphenols intake on obesity and its related metabolic diseases outcomes in animal model.
Several studies have been reported on obesity and its RMDs lowering using different extracts from leaves, flowers, fruits, seeds, rhizome, powder, and EVOO obtained from wild and cultivated plants regularly administrated at 3 mg/kg/day to 23 g/kg/day for 3–24 weeks to animal models (Table 1). Controversially, other studies using dairy dose administrated from the green tea polyphenols (10–29 mg/kg), catechin (200 and 400 mg/kg) (54), caffeic acid, quercetin (2 or 4%) (55), and proanthocyanin grape seed extract (4 g/kg/2 weeks) (56), reported liver, kidney and gastrointestinal toxicity, which can evolve to inflammation or death, due to high reactive oxygen species and oxidative stress formation. In addition, some studies with humans administered polyphenols showed the same results that can be explained by genetic effects, ethnicity, gender, eating habits, length of time, lifestyle, and others (57). Therefore, the reported high health benefits of regular consumption of polyphenol-rich plants and vegetables are widely recommended to prevent, control and reduce obesity and RMDs in humans and animals (57). Likewise, the health benefit for humans with obesity, that administered vegetables, fruits and polyphenols for 4–12 weeks are summarized in Table 2.
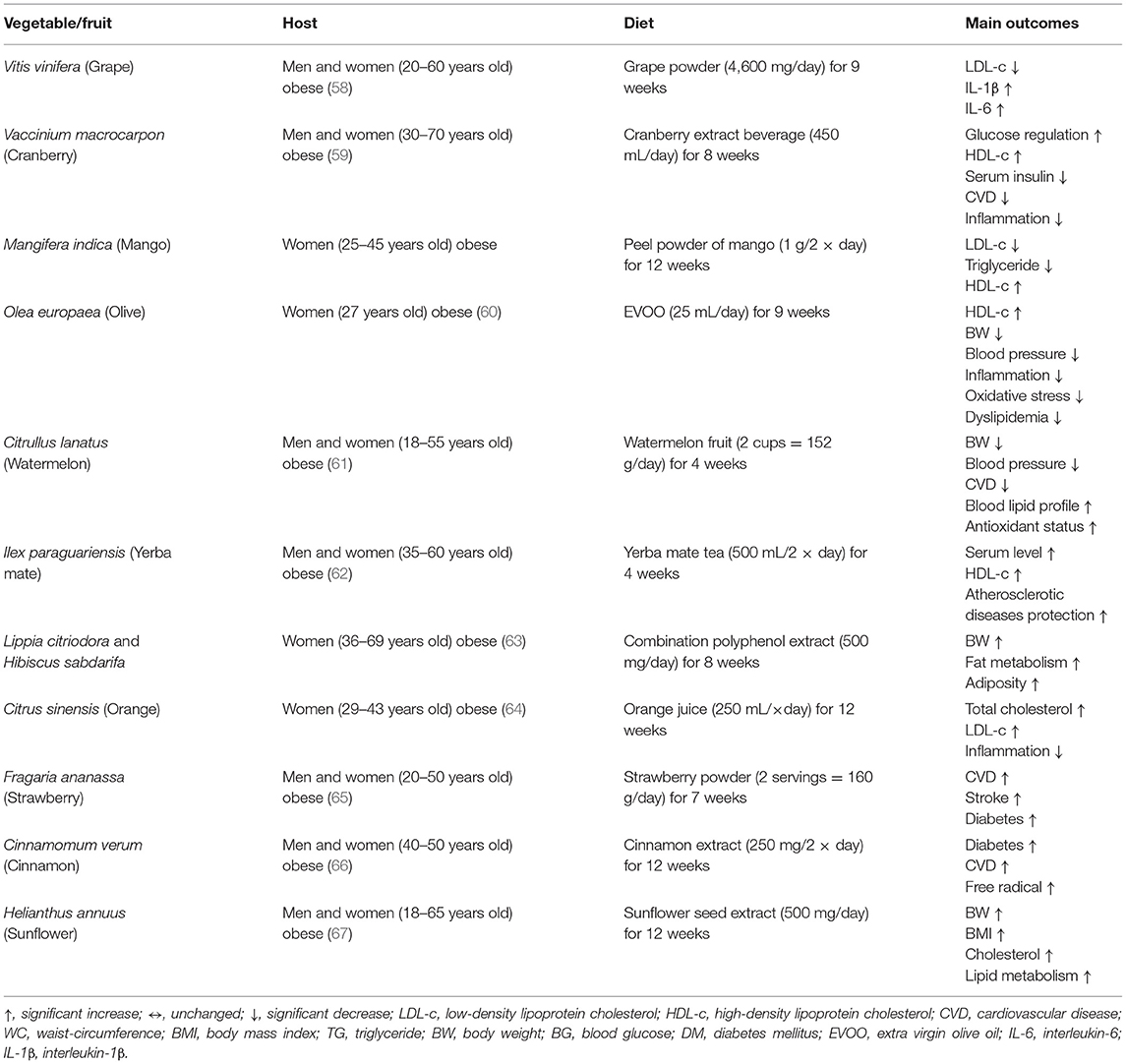
Table 2. Effects of polyphenols from vegetables and fruits intake on obesity and its related metabolic diseases outcomes in human subjects.
The Main ω-3 PUFAs Sources
The main sources of ω-3 PUFAs, including ALA, EPA and DHA are green leafy vegetables, seaweed, seeds, nuts, vegetable oils, fish and fish oils (68–77). The vegetable and fish origin ω-3 PUFAs are summarized in Table 3.
ALA is abundantly obtained in vegetable foodstuff and microalgae (7–94%) followed by vegetable oils (6–58%) and freshwater fish (1–4%) (69, 70, 73–77). While EPA and DHA are the majority in fish oil (7–13% and 9–18%), marine fish (3–6% and 13–33%), microalgae (13–31% and 2–14%), macroalgae (3–27% and 1–5%), and fish of freshwater (0.4–3% and 2–9%) (69, 71–74, 76).
The ω-3 PUFAs and ω-6 PUFAs are essential fatty acids (cannot be biosynthesized by the mammalian body, including humans) are required from the diet (78, 79). In the human body, through to physiology mechanism reactions, which ALA is converted to long chain PUFAs (LC-PUFAs, fatty acids ≤ C20) and very-long-chain fatty acids (VLCFAs, fatty acids ≥ C22) (78, 79), which the ALA converted rate to EPA and DHA is 5–8% (80). The biosynthetic process of VLCFAs production, starting by ALA from the diet to the bloodstream is illustrated in Figure 2.
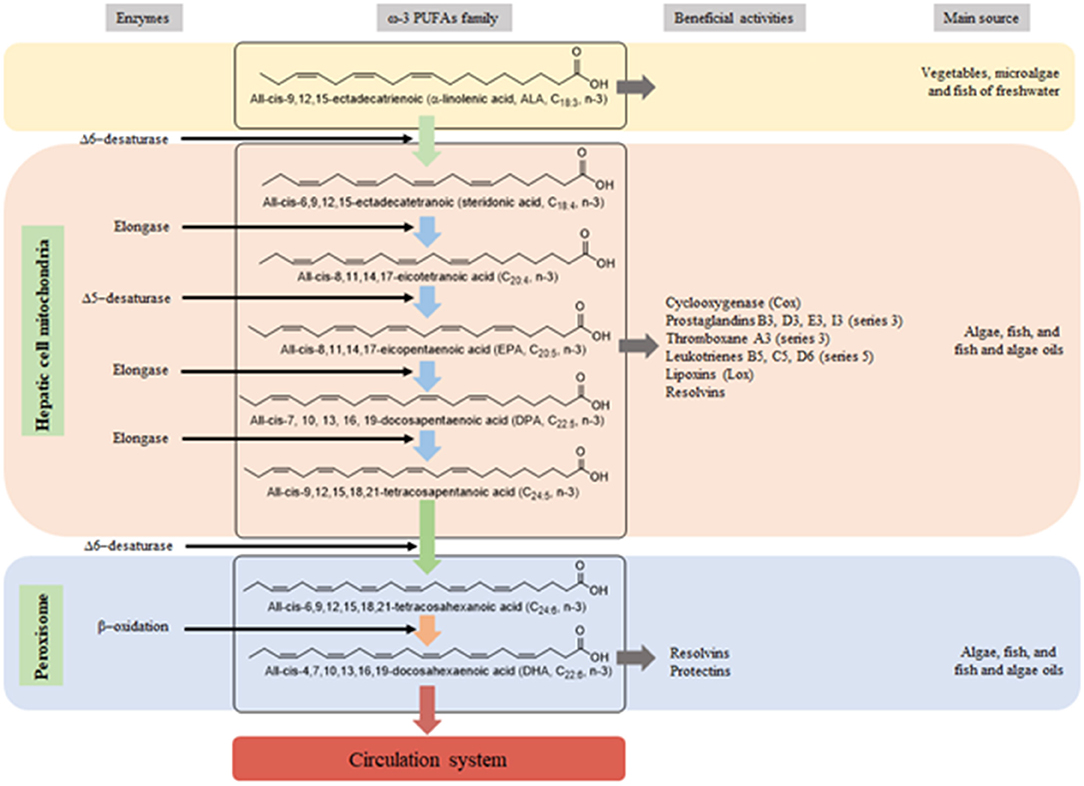
Figure 2. Biosynthesis pathway of very-long-chain polyunsaturated fatty acids (LC-PUFAs) and very long-chain fatty acids (VLCFAs) in the human body starting by the α-linolenic acid (ALA) obtained from the diet. The LC-PUFAs and VLCFAs biosynthesis process occurs in hepatic cell mitochondria and peroxisome. These acids reach the bloodstream, which are conducted to different body parts for health benefits.
When consumed and going through several physiological reactions in the body, EPA and DHA present positive effects such as anti-inflammation, vasodilation, bronchodilation and antiplatelet aggregation (78). Beyond, both acids are correlated with cyclooxygenase, prostacyclin, thromboxane, leukotrienes, lipoxins, and resolvins, which play a crucial role in several beneficial physiologic actions (78, 79, 81). The consumption of an ω-3 PUFAs-rich balanced diet, including ALA, EPA, and DHA is correlated with health-improving and decreasing and or preventing obesity and its RMDs, such as adipose tissue fat accumulation, insulin resistance, inflammation, hypertension, atherosclerosis, CVD, CHD, and DM (4, 78, 79).
However, due to the presence of double bond in carbon-3 of methyl end (ω-3), including ALA, EPA, and DHA, ω-3 PUFAs family is susceptible to oxidation by light, temperature, metal ions and microorganism degradation during oil extraction and storage by autoxidation reactions (photochemical and photosensitized oxidation) with 4-Hydroxy-2-hexenal production (82, 83). These reactions result in enzymatic oxidation with increase the production of E-series resolvins from EPA, and D-series Resolvins (DHA), prostaglandins, thromboxanes, leukotrienes, epoxy products (84, 85). Besides, the ω-3 PUFAs decrease in amount during food confections by thermal processing, while in inversely proportion occurs the increasing of degradation and hazard oxidized substances that damage cell membranes (86, 87). The oxidation products are higher in fried, followed by roasted, and boiled foods, which present the same proportion of oxidative products when compared to raw food (88, 89). The frying and roasting food confections release the most oxidative products (4(RS)-4-F4t-NeuroP, 4-Hydroxy-2-hexenal production, and others), which are correlated with obesity, CVD, inflammation, hypertension, and others diseases (82, 83).
Therefore, the application of natural antioxidant compounds such as carotenoids, tocopherols, tocotrienols, phytostanols, phytosterols, and ascorbic acid are recommended due to their symbiotic and synergistic interactions decrease oxidation and thermal degradation, prolonging the shelf life of ω-3 PUFAs during the period of storage (90–92).
Dietary EPA and DHA Diets Benefits on Obesity and its RMDs
Diets consumption rich in vegetables and fish and their by-products are correlated with reducing obesity and its RDMs effects for presenting ALA, EPA, and DHA in their composition (4, 78, 79), and for animal models are summarizing in Table 4.
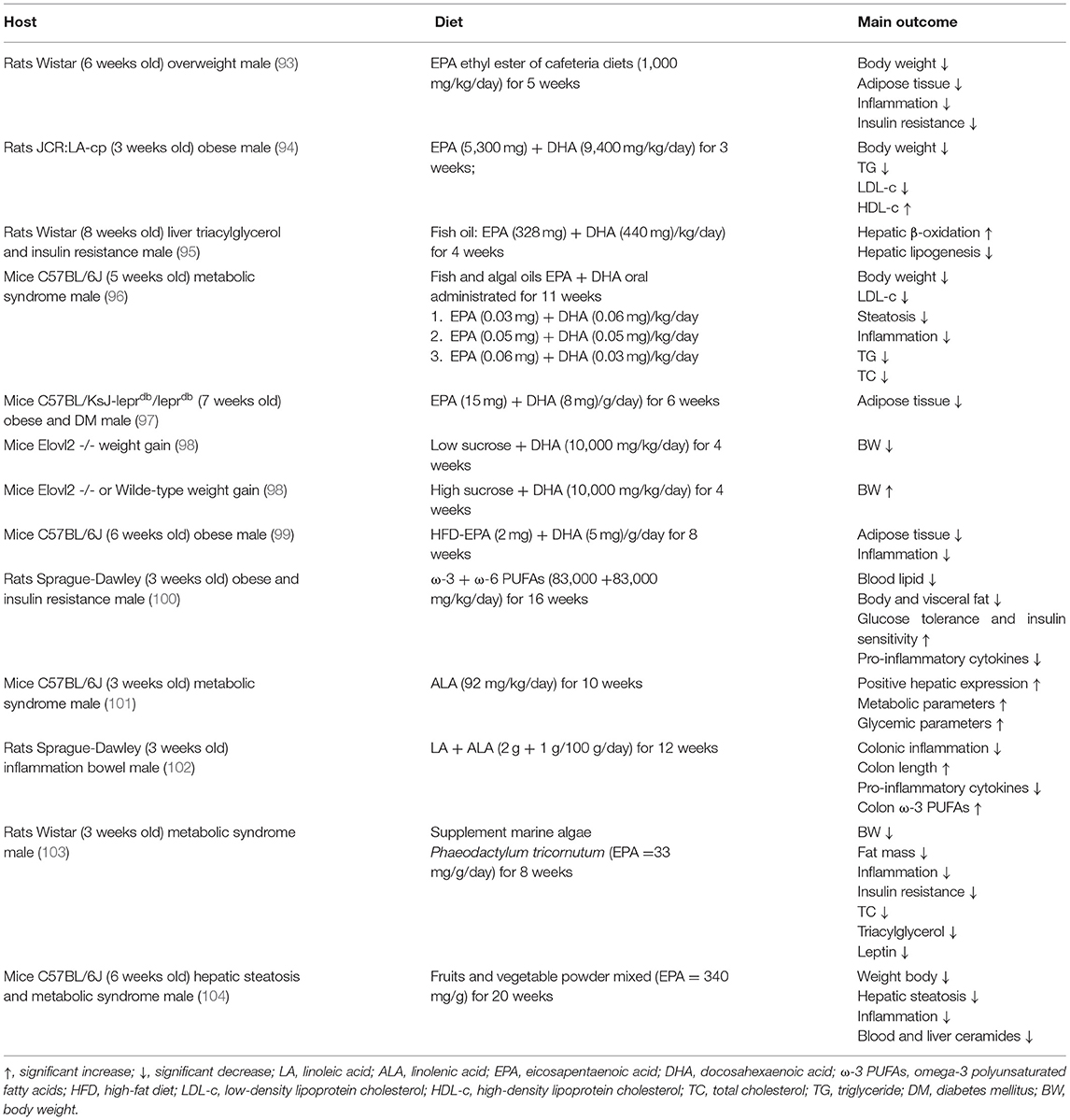
Table 4. Effects of EPA and DHA intake on obesity and related metabolic diseases outcomes in animal models.
Obesity and its reduction in RMDs have been reported in animal studies that consumed for 3–20 weeks EPA from vegetable/fruit and cafeteria diets (33 mg/g/day to 1,000 mg/kg/day), EPA mixed with DHA (2–5,300 mg/g and 3–9,400 mg/g/day), ALA (92 g/kg/day), ω-3/ω-6 (1:1), and linoleic acid (LA) plus ALA (2:1) (93, 94, 99, 100, 102–104). These positive effects observed are correlated with ω-3 PUFAs that improve and repair several organs for normal function linked to hepatic organ for better lipogenesis, insulin resistance, lipid homeostasis, adipocytes function, β-oxidation, and increasing leptin and adiponectin production, pro-inflammatory mediators reducing from LA and arachidonic (AA) acids (78, 79, 81). However, some studies reported a discrepancy effect of ω-3 PUFAs to diabetes, cholesterol, plasma glucose (105), overweight and obesity (106), inflammatory cytokines (107), cardiovascular diseases, and others (108). These ω-3 PUFAs fail results can be associated with its preparation, doses quantity, administration duration period, subject target, statistics, and other factors (109, 110). Therefore, ω-3 PUFAs regular consumption is recommended due to numerous studies that demonstrated strong positive effects against several metabolic diseases in animal models and human subjects, as summarized in Table 5.
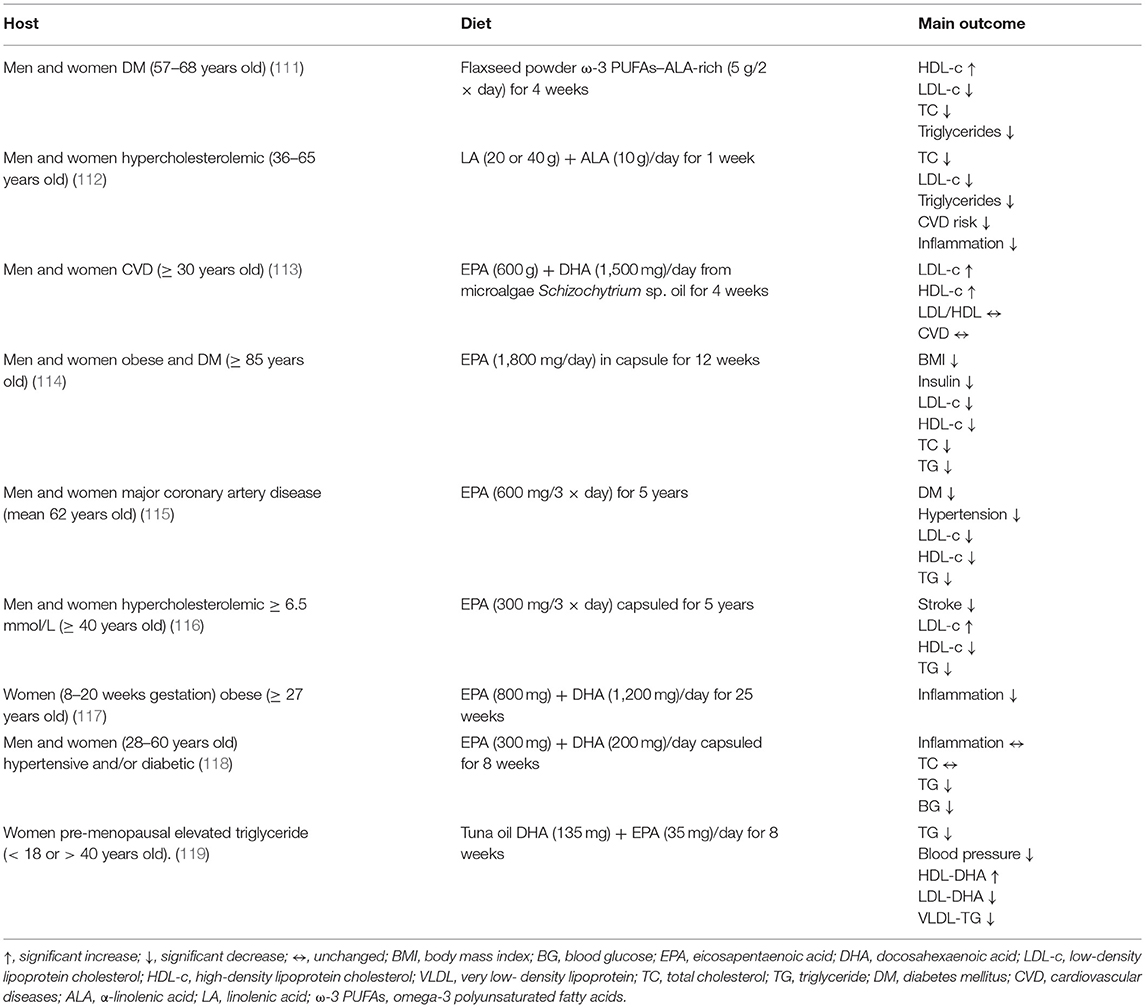
Table 5. Effects of EPA and DHA intake on obesity and its related metabolic diseases outcomes in human subjects.
Furthermore, lowering obesity and its RMDs were observed for human subjects daily administered 2 ×2 g of flaxseed powder, as well as in proportion of 4:1 and 2:1 of LA and ALA for 1 and 2 weeks (111, 112), EPA daily dosed 3 ×300 mg or 3 ×600 mg, dose of 1,800 mg during 12 weeks and 5 years (114–116), and doses of EPA and DHA during 8–25 weeks in proportions of 1:1.5, 1.5:1, and 1:4 (117–119).
Polyphenols and ω-3 PUFAs Mechanisms on Obesity and its RMDs
Increasing of obesity and its RMDs are already observed from childhood to elderly individuals and have become a public health problem in modern society (120, 121). A practical alternative against obesity and its RDMs in humans can be associated with diet-rich in polyphenols and ω-3 PUFAs in composition, including their by-products (112). In the body, polyphenols and ω-3 PUFAs (DHA and EPA) physiologically act protecting and inhibiting cascade inflammatory reaction processes that can evolve into obesity, diabetes, CVD, hypercholesterolemia, and others metabolic diseases (122, 123). Thus, mechanisms that polyphenols and ω-3 PUFAs are involved in the body, which are crucial to prevent several metabolic diseases, which can be used as adjuvant therapy, are summarized in Figure 3.
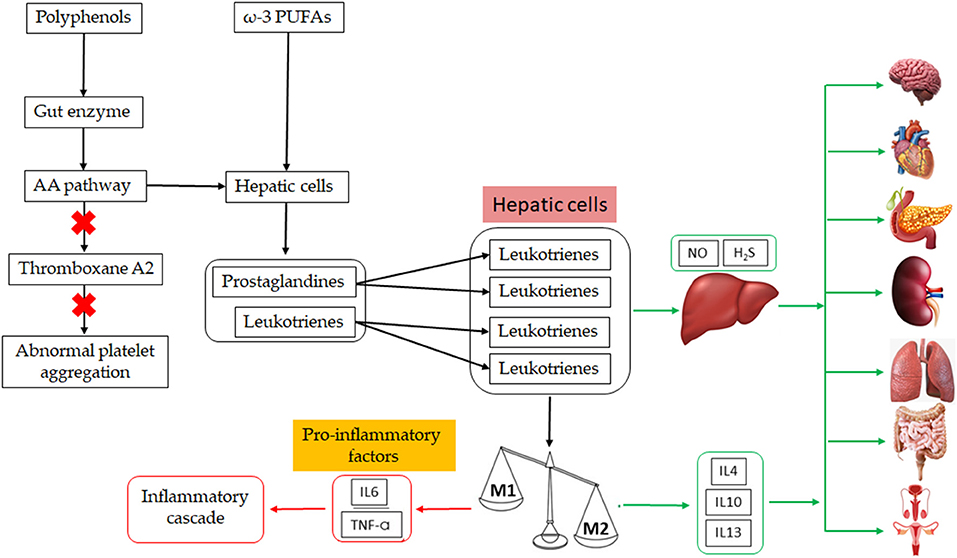
Figure 3. Mechanism involved in an inflammatory condition and its resolution using ω-3 PUFAs and polyphenols dietary. The action of products from PUFAs metabolization (hepatic biosynthesis or tissue under inflammation), lipoxins, resolvins, protectins, and maresins on macrophage profile change and the endothelial cells. As a result, there are anti-inflammatory interleukins, nitric oxide (NO) and hydrogen sulfite (H2S) being produced, which will provide the resolution and tissue regeneration. Products from polyphenols metabolization are also connected with this anti-inflammatory pathway to several organs in the body. M1, type 1 macrophages; M2, type 2 macrophages; IL, interleukin; TNF-α, tumor necrosis factor-alpha. Green lines mean resolution of the inflammatory process and red lines mean the uncontrolled inflammatory process leading to an inflammatory cascade.
In the liver, PUFAs are metabolized and converted into prostaglandins (PGE2) and leukotrienes, which reach the inflammation site being converted into lipoxins, resolvins, protectins, and maresins, which will stimulate type 2 macrophages more so than the type 1 kind, leading to the production of anti- inflammatory interleukins (124, 125). Likewise, polyphenols are absorbed in the intestine after being hydrolyzed by intestine enzymes and the host's microbiota (126). Then, the resulting molecules can interact with free radicals and inhibit enzymes involved in the AA pathway, modulating the inflammatory response and blocking the AA pathway (14). Besides that, endothelial cells are also being stimulated by both products from polyphenols and ω-3 PUFAs metabolization to produce NO and H2S in the first case, which will aid the resolution of the inflammatory situation and the tissue regeneration, or trigger signaling cascades by interacting with cell membrane receptors such as vascular endothelial growth factor (VEGF) or blocking p-AKT, NF-κB, and MMP-9 activities (122, 127).
The mechanisms involved in balancing the inflammatory process are the change of the phospholipid fatty acid composition of the cell membrane, inhibition of the NF-κβ activation, thus reducing the expression of pro-inflammatory genes and production of resolving mediators by macrophages (122).
Consumer Behavior Changes on Obesity and its RMDs
Choosing daily healthy food type intake is the chief component and managed by humans to improve their own and all family healthy lifestyle (128). Among the several factors of healthy lifestyle or prevalence of obesity and its RMDs can be associated with regular or irregularly and healthy or unhealthy daily food consumed in each meal (22, 129). In addition, it may also be associated with the lower purchase price of unhealthy foods on the market compared with healthy ones, whose edible parts (leaves, peel, flesh, seeds, and others) are wasted in homes, restaurants and other food enterprises due to their lack of nutritional knowledge (130, 131). In addition, also it is known that refined sugar is often always added to edible vegetables, fruits, natural juices and other by-products and other beverages, which can be associated with obesity, overweight, CVD, and other metabolic diseases prevalence (132).
Thereby, Figure 4 summarizes food types that improve healthy life (green line), which oil rich in ω-3 PUFAs, oleic acid and short-chain fatty acid are widely recommended (4). Daily at least 400 mg of natural and/or native fruits and vegetables (133), while weekly 3 ×150 g of fish are recommended (134). Furthermore, fruits, vegetables and fishes are natural sources of macro- and microelements, vitamins, resistant nutrients, free sugars and fibers, which play a crucial role in microbiota balance, satiety, gut health and act as antioxidants in the body, improving and/or impeding obesity and others prevalent metabolic diseases (135–137).
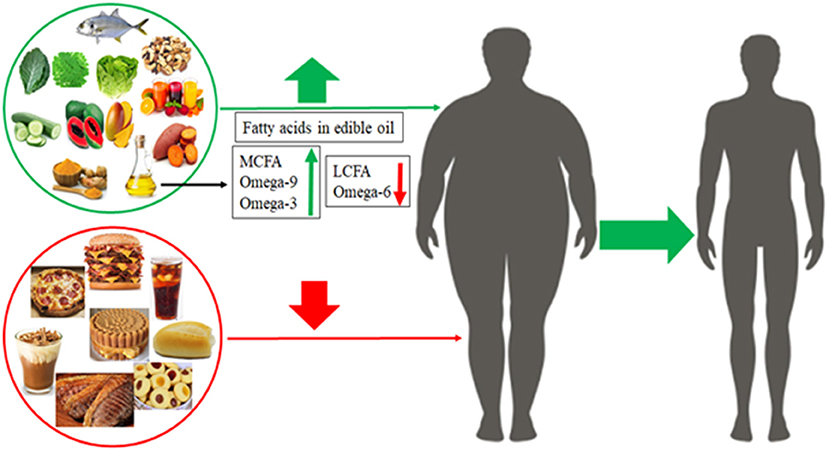
Figure 4. Healthy food (green line) intake reduce obesity to normal conditions, while unhealthy food (red line) conduces to obesity and its related metabolic diseases.
Paradoxically, nowadays, meals rich in vegetables and fruits are associated with poor and traditional peoples, while meat and sweetened ones are associated with rich and modern life (24, 138, 139). The consumption of foods marked by the red line (Figure 4) must be reduced, because they are sweetened and fatted, including long-chain saturated fatty acids (mainly myristic and palmitic acids), ω-6 PUFAs and industrialized trans-fatty acids present high amounts of calories in their composition, which are primarily associated with obesity and its prevalent RDMs (134).
Hence, for human behavior changes, joint activities between Universities, Research Centers, Health Ministries, and others will be legally necessary constitution of Departments that could be responsible by outline joint projects and approaches for health promotion through seminars, and lectures to implement in schools (Primary and Secondary), enterprises and families to promote healthy food cooking, sale, and intake to pave the way to reduce obesity and its RMDs prevalence (140–144).
Conclusion
The consumption of vegetables, fruits, seed and fish and/or supplements rich in polyphenols and ω-3 PUFAs is widely correlated with reducing of obesity and its related metabolic diseases prevalence. Thus, for behavior change, it is necessary to draw out a joint projects of research institutions and the Health Ministries to schools, enterprises and families to promote healthy food intake to reduce obesity and its related metabolic diseases.
Author Contributions
TS, DM, VZ-P, DB, AP, and RG conceptualized the topic, researched and analyzed the literature, wrote the manuscript, and including interpretation. PF, GM, PH, MV, RF, EC, and VN contributed with draft and interpretation and revised the manuscript critically for intellectual content. All authors have read and approved the final version of the manuscript, ensure the accuracy and integrity of the work, and agree to be accountable for all appearance.
Funding
This research was funded by Federal University of Mato Grosso do Sul (UFMS) and Coordination of Higher Education Personnel Improvement (CAPES)-Portaria 2016/2018. This study was financed in part by the CAPES-finance code 001. The study was also supported by research grants from the National Council for Scientific and Technological Development (Conselho Nacional de Desenvolvimento Científico e Tecnológico-CNPq).
Conflict of Interest
The authors declare that the research was conducted in the absence of any commercial or financial relationships that could be construed as a potential conflict of interest.
Publisher's Note
All claims expressed in this article are solely those of the authors and do not necessarily represent those of their affiliated organizations, or those of the publisher, the editors and the reviewers. Any product that may be evaluated in this article, or claim that may be made by its manufacturer, is not guaranteed or endorsed by the publisher.
Acknowledgments
We thank the Graduate Program in Material Sciences, Graduate Program in Biotechnology and Biodiversity, and the Graduate Program in Health and Development in the Central-West Region, Federal University of Mato Grosso do Sul-UFMS for support.
References
1. Rogero MM, Calder P. Obesity, inflammation, toll-like receptor 4 and fatty acids. Nutrients. (2018) 10:432. doi: 10.3390/nu10040432
2. Lyons CL, Kennedy EB, Roche HM. Metabolic inflammation-differential modulation by dietary constituents. Nutrients. (2016) 8:247. doi: 10.3390/nu8050247
3. Figueiredo PS, Inada AC, Marcelino G, Cardozo CML, Freitas KC, Guimarães RCA, et al. Fatty acids consumption: the role metabolic aspects involved in obesity and its associated disorders. Nutrients. (2017) 9:1158. doi: 10.3390/nu9101158
4. Machate DJ, Figueiredo PS, Marcelino G, Guimarães RCA, Hiane PA, Bogo D, et al. Fatty acid diets: regulation of gut microbiota composition and obesity and its related metabolic dysbiosis. Int J Mol Sci. (2020) 21:4093. doi: 10.3390/ijms21114093
5. World Health Organization. Health Diet. (2021). Available online at: https://www.who.int/news-room/fact-sheets/detail/healthy-diet#:~:text=Eating%20at%20least%20400%20g,aily%20intake%20of%20dietary%20fibre (accessed August 4, 2021).
6. Mafort TT, Rufino R, Costa CH, Lopes AJ. Obesity: systemic and pulmonary complications, biochemical abnormalities, and impairment of lung function. Multidiscip Respir Med. (2016) 11:28. doi: 10.1186/s40248-016-0066-z
7. Kris-Etherton PM, Grieger JA, Etherton TD. Dietary reference intakes for DHA and EPA. Prostaglandins Leukot Essent Fatty Acids. (2009) 81:99–104. doi: 10.1016/j.plefa.2009.05.011
8. Slavin JL, Lloyd B. Health benefits of fruits and vegetables. Adv Nutr. (2012) 3:506–16. doi: 10.3945/an.112.002154
9. Hogan S, Canning C, Sun S, Sun X, Zhou K. Effects of grape pomace antioxidant extract on oxidative stress and inflammation in diet induced obese mice. J Agric Food Chem. (2010) 58:11250–6. doi: 10.1021/jf102759e
10. Van Hul M, Geurts L, Plovier H, Druart C, Everard A, Stahlman M, et al. Reduced obesity, diabetes, and steatosis upon cinnamon and grape pomace are associated with changes in gut microbiota and markers of gut barrier. Am J Physiol Endocrinol Metab. (2018) 314:E334–52. doi: 10.1152/ajpendo.00107.2017
11. Violi F, Loffredo L, Pigatelli P, Angelico F, Bartimoccia S, Nocella C, et al. Extra virgin olive oil use is associated with improved post-prandial blood glucose and LDL cholesterol in healthy subjects. Nutr Diabetes. (2015) 5:e172. doi: 10.1038/nutd.2015.23
12. Carnevale R, Loffredo L, Del Ben M, Angelico F, Nocella C, Petruccioli A, et al. Extra virgin olive oil improves post-prandial glycemic and lipid profile in patients with impaired fasting glucose. Clin Nutr. (2017) 36:782–7. doi: 10.1016/j.clnu.2016.05.016
13. Chuang CC, McIntosh MK. Potential mechanisms by which polyphenol-rich grapes prevent obesity-mediated inflammation and metabolic diseases. Annu Rev Nutr. (2011) 31:155–76. doi: 10.1146/annurev-nutr-072610-145149
14. Méndez L, Medina I. Polyphenols and fish oils for improving metabolic health: a revision of the recent evidence for their combined nutraceutical effects. Molecules. (2021) 26:2438. doi: 10.3390/molecules26092438
15. Pagliaro M, Pizzone DM, Scurria A, Lino C, Paone E, Mauriello F, et al. Sustainably sourced olive polyphenols and ômega-3 marine lipids: a synergy fostering public health. ACS Food Sci Technol. (2021) 1:139–45. doi: 10.1021/acsfoodscitech.0c00082
16. Pan H, Gao Y, Tu Y. Mechanisms of body weight reduction by black tea polyphenols. Molecules. (2016) 21:1659. doi: 10.3390/molecules21121659
17. Manach C, Mazur A, Scalbert A. Polyphenols and prevention of cardiovascular diseases. Curr Opin Lipidol. (2005) 16:77–84. doi: 10.1097/00041433-200502000-00013
18. Hodgson JM, Croft KD. Tea flavonoids and cardiovascular health. Mol Aspects Med. (2010) 31:495–502. doi: 10.1016/j.mam.2010.09.004
19. Cheng YC, Sheen JM, Hu WL, Hung YC. Polyphenols and oxidative stress in atherosclerosis-related ischemic heart disease and stroke. Oxid Med Cell Longev. (2017) 2017:8526438. doi: 10.1155/2017/8526438
20. Giglio RV, Patti AM, Cicero AFG, Luppi G, Rizzo M, Toth PP, et al. Polyphenols: potential use in the prevention and treatment of cardiovascular diseases. Curr Pharm Des. (2018) 24:239–58. doi: 10.2174/1381612824666180130112652
21. Tang GY, Meng X, Gan RY, Zhao CN, Liu Q, Feng YB, et al. Health functions and related molecular mechanisms of tea components: an update review. Int J Mol Sci. (2019) 20:6196. doi: 10.3390/ijms20246196
22. Laraia BA, Leak TM, Tester JM, Leung CW. Biobehavioral factors that shape nutritional in low-income populations: a narrative review. Am J Prev Med. (2017) 52:S118–26. doi: 10.1016/j.amepre.2016.08.003
23. Jones NRV, Conklin AI, Suhrcke M, Monsivais P. The growing price gap between more and less healthy foods: analysis of a novel longitudinal UK dataset. PLoS ONE. (2014) 9:e109343. doi: 10.1371/journal.pone.0109343
24. Seguin R, Connor L, Nelson M, LaCroix A, Eldridge G. Understanding barriers and facilitators to healthy eating and active living in rural communities. J Nutr Metab. (2014) 2014:146502. doi: 10.1155/2014/146502
25. Fard NA, Morales GF, Mejova Y, Schifanella R. On the interplay between educational attainment and nutrition: a spatially-aware perspective. EPJ Data Sci. (2021) 10:18. doi: 10.1140/epjds/s13688-021-00273-y
26. Zhu F, Du B, Zheng L, Li J. Advance on the bioactivity and potential applications of dietary fibre from grape pomace. Food Chem. (2015) 186:207–12. doi: 10.1016/j.foodchem.2014.07.057
27. Ribeiro LF, Ribani RH, Francisco TMG, Soares AA, Pontarolo R, Haminiuk CWI. Profile of bioactive compounds from grape pomace (Vitis vinifera and Vitis labrusca) by spectrophotometric, chromatographic and spectral analyses. J Chromatogr B Analyt Technol Biomed Life Sci. (2015) 1007:72–80. doi: 10.1016/j.jchromb.2015.11.005
28. Rasines-Perea Z, Teissedre PL. Grape polyphenols' effects in human cardiovascular diseases and diabetes. Molecules. (2017) 22:68. doi: 10.3390/molecules22010068
29. Talhaoui N, Gómez-Caravaca AM, León L, De la Rosa R, Fernández-Gutiérrez A, Segura-Carretero A. From olive fruits to olive oil: phenolic compound transfer in six different olive cultivars grown under the same agronomical conditions. Int J Mol Sci. (2016) 17:337. doi: 10.3390/ijms17030337
30. Dal S, Sigrist S. The protective effect of antioxidants consumption on diabetes and vascular complications. Diseases. (2016) 4:24. doi: 10.3390/diseases4030024
31. Lamien-Meda A, Lamien CE, Compaoré MMY, Meda RNT, Kiendrebeogo M, Zeba B, et al. Polyphenol content and antioxidant activity of fourteen wild edible fruits from Burkina Faso. Molecules. (2008) 13:581–94. doi: 10.3390/molecules13030581
32. Shan S, Huang X, Shah MH, Abbasi AM. Evaluation of polyphenolics content and antioxidant activity in edible wild fruits. Biomed Res Int. (2019) 2019:1381989. doi: 10.1155/2019/1381989
33. Pandey KB, Rizvi SI. Plant polyphenols as dietary antioxidants in human health and disease. Oxid Med Cell Longev. (2009) 2:270–8. doi: 10.4161/oxim.2.5.9498
34. Kumar V, Sharma A, Kohli SK, Bali S, Sharma M, Kumar R, et al. Differential distribution of polyphenols in plants using multivariate techniques. Biotechnol Res Innov. (2019) 3:1–21. doi: 10.1016/j.biori.2019.03.001
35. Serreli G, Deiana M. Biological relevance of extra virgin olive oil polyphenols metabolites. Antioxidants. (2018) 7:170. doi: 10.3390/antiox7120170
36. Lima GPP, Vianello F, Corrêa CR, Campos RAS, Borguini MG. Polyphenols in fruits and vegetables and its effect on human health. Food Nutr Sci. (2014) 5:1065–82. doi: 10.4236/fns.2014.511117
37. Xu DP, Li Y, Meng X, Zhou T, Zhou Y, Zheng J, et al. Natural antioxidants in foods and medicinal plants: Extraction, assessment and resources. Int J Mol Sci. (2017) 18:96. doi: 10.3390/ijms18010096
38. Wei Z, Luo J, Huang Y, Guo W, Zhang Y, Guan H, et al. Profile of polyphenol compounds of five muscadine grapes cultivated in the United States and in newly adapted locations in China. Int J Mol Sci. (2017) 18:631. doi: 10.3390/ijms18030631
39. Liu C, Guo Y, Sun L, Lai X, Li Q, Zhang W, et al. Six types of tea reduce high-fat diet-induced fat accumulation in mice by increasing lipid metabolism and suppressing inflammation. Food Funct. (2019) 10:2061–74. doi: 10.1039/C8FO02334D
40. Chuang CC, Shen W, Chen H, Xie G, Jia W, Chung S, et al. Differential effects of grape powder and its extract on glucose tolerance and chronic inflammation in high-fat-fed obese mice. J Agric Food Chem. (2012) 60:12458–68. doi: 10.1021/jf3028107
41. Pascual-Serrano A, Arola-Arnal A, Suárez-García S, Bravo FI, Suárez M, Arola L, et al. Grape seed proanthocyanidin supplementation reduces adipocyte size and increases adipocyte number in obese rats. Int J Obes. (2017) 41:1246–55. doi: 10.1038/ijo.2017.90
42. Elmhdwi MF, Elaali NMS, Mohamed NN, Muktar MA, Buzgeia NB. Anti-diabetic activity of methanolic extract of grape seeds in alloxan induced diabetic rats. Agr Res Tech. (2017) 6:555699. doi: 10.19080/ARTOAJ.2017.06.555699
43. Ballard CR, Santos EF, Dubois MJ, Pilon G, Cazarin CBB, Maróstica Junior MR, et al. Two polyphenol-rich Brazilian fruit extracts protect from diet-induced obesity and hepatic steatosis in mice. Food Funct. (2020) 11:8800–10. doi: 10.1039/D0FO01912G
44. Ebaid H, Bashandy SAE, Alhazza IM, Hassan I, Al-Tamimi J. Efficacy of a methanolic extract of Adansonia digitata leaf in alleviating hyperglycemia, hyperlipidemia, and oxidative stress of diabetic rats. Biomed Res Int. (2019) 2019:2835152. doi: 10.1155/2019/2835152
45. Vazquez A, Sanchez-Rodriguez E, Vargas F, Montoro-Molina S, Romero M, Espejo-Calvo JA, et al. Cardioprotective effect of a virgin olive oil enriched with bioactive compounds in spontaneously hypertensive rats. Nutrients. (2019) 11:1728. doi: 10.3390/nu11081728
46. Jurado-Ruiz E, Álvarez-Amor L, Varela LM, Bern á G, Parra-Camacho MS, Oliveira-Lopez MJ, et al. Extra virgin olive oil diet intervention improves insulin resistance and islet performance in diet-induced diabetes in mice. Sci Rep. (2019) 9:11311. doi: 10.1038/s41598-019-47904-z
47. Weisberg SP, Leibel R, Tortoriello DV. Dietary curcumin significantly improves obesity- associated inflammation and diabetes in mouse models of diabesity. Endocrinology. (2008) 149:3549–58. doi: 10.1210/en.2008-0262
48. Seo KI, Lee J, Choi RY, Lee HI, Lee JH, Jeong YK, et al. Anti-obesity and anti-insulin resistance effects of tomato vinegar beverage in diet-induced obese mice. Food Funct. (2014) 5:1579–86. doi: 10.1039/c4fo00135d
49. Oliveira PR, Costa CA, Bem GF, Cordeiro VSC, Santos IB, Carvalho LCRM, et al. Euterpe oleracea Mart.–Derived polyphenols protect mice from diet-induced obesity and fatty liver by regulating hepatic lipogenesis and cholesterol excretion. PLoS ONE. (2015) 10:e0143721. doi: 10.1371/journal.pone.0143721
50. Panchal SK, Poudyal H, Waanders J, Brown L. Coffee extract attenuates changes in cardiovascular and hepatic structure and function without decreasing obesity in high-carbohydrate, high-fat diet-fed male rats. J Nutr. (2012) 142:690–7. doi: 10.3945/jn.111.153577
51. Boqué N, Iglesia R, Garza AL, Milagro FI, Olivares M, Bañuelos O, et al. Prevention of diet-induced obesity by apple polyphenols in Wister rats through regulation of adipocyte gene expression and DNA methylation patterns. Mol Nutr Food Res. (2013) 57:1473–8. doi: 10.1002/mnfr.201200686
52. Azman KF, Amom Z, Azlan A, Esa NM, Ali RM, Shah ZM, et al. Antiobesity effect of Tamarindus indica L. pulp aqueous extract in high-fat diet-induced obese rats. J Nat Med. (2011) 66:333–42. doi: 10.1007/s11418-011-0597-8
53. Aranaz P, Navarro-Herrera D, Romo-Hualde A, Zabala M, López-Yoldi M, González-Ferrero C, et al. Broccoli extract improves high fat diet-induced obesity, steatosis and glucose intolerance in Wistar rats. J Funct Foods. (2019) 59:319–28. doi: 10.1016/j.jff.2019.05.054
54. Lambert JD, Sang S, Yang CS. Possible controversy over dietary polyphenols: benefits vs risks. Chem Res Toxicol. (2007) 20:583–5. doi: 10.1021/tx7000515
55. Mennen LI, Walker R, Bennetau-Pelissero C, Scalbert A. Risks and safety of polyphenols consumption. Am J Clin Nutr. (2005) 81:326S−9S. doi: 10.1093/ajcn/81.1.326S
56. Ofosu FK, Daliri EBM, Elahi F, Chelliah R, Lee BH, Oh DH. New insights on the use of polyphenols as natural preservatives and their emerging safety concerns. Front Sustain Food Syst. (2020) 4:525810. doi: 10.3389/fsufs.2020.525810
57. Wang S, Moustaid-Moussa N, Chen L, Mo H, Shastri A, Su R, et al. Novel insights of dietary polyphenols and obesity. J Nutr Biochem. (2014) 25:1–18. doi: 10.1016/j.jnutbio.2013.09.001
58. Zunino SJ, Peerson JM, Freytag TL, Breksa AP, Bonnel EL, Woodhouse LR, et al. Dietary grape powder increases IL-1β and IL-6 production by lipopolysaccharide- activated monocytes and reduces plasma concentrations of large LDL and large LDL-cholesterol particles in obese humans. Br J Nutr. (2014) 112:369–80. doi: 10.1017/S0007114514000890
59. Chew B, Mathison B, Kimble L, McKay D, Kaspar K, Khoo C, et al. Chronic consumption of a low calorie, high polyphenol cranberry beverage attenuates inflammation and improves glucoregulation and HDL cholesterol in healthy overweight humans: a ranamized controlled trial. Eur J Nutr. (2019) 58:1223–35. doi: 10.1007/s00394-018-1643-z
60. Álvarez-Pérez J, Sánchez-Villegas A, Díaz-Benítez EM, Ruano-Rodríguez C, Corella D, Martínez-González AM, et al. Influence of a Mediterranean dietary pattern on body fat distribution: results of the PREDIMED–Canarias intervention randomized trial. J Am Coll Nutr. (2016) 35:568–80. doi: 10.1080/07315724.2015.1102102
61. Lum T, Connolly M, Marx A, Beidler J, Hooshmand S, Kern M, et al. Effects of fresh watermelon consumption on the acute satiety response and cardiometabolic risk factors in overweight and obese adults. Nutrients. (2019) 11:595. doi: 10.3390/nu11030595
62. Balsan G, Pellanda LC, Sausen G, Galarraga T, Zaffari D, Pontin B, et al. Effect of yerba mate and green tea on paraoxonase and leptin levels in patients affected by overweight or obesity and dyslipidemia: a randomized clinical trial. Nutr J. (2019) 18:5. doi: 10.1186/s12937-018-0426-y
63. Herranz-López M, Olivares-Vicente M, Boix-Castejón M, Caturla N, Roche E, Micol V. Differential effects of a combination of Hibiscus sabdariffa and Lippia citriodora polyphenols in overweight/obese subjects: a randomized controlled trial. Sci Rep. (2019) 9:2999. doi: 10.1038/s41598-019-39159-5
64. Azzini E, Venneria E, Ciarapica D, Foddai MS, Intorre F, Zaccaria M, et al. Effect of red orange juice consumption on body composition and nutritional status in overweight/obese female: a pilot study. Oxid Med Cell Longev. (2017) 2017:1672567. doi: 10.1155/2017/1672567
65. Zunino SJ, Parelman MA, Freytag TL, Stephensen CB, Kelley DS, Mackey BE, et al. Effects of dietary strawberry powder on blood lipids and inflammatory markers in obese human subjects. Br J Nutr. (2012) 108:900–9. doi: 10.1017/S0007114511006027
66. Roussel AM, Hininger I, Benaraba R, Ziegenfuss TN, Anderson RA. Antioxidant effects of a cinnamon extract in people with impaired fasting glucose that are overweight or obese. J Am Coll Nutr. (2009) 28:16–21. doi: 10.1080/07315724.2009.10719756
67. Leverrier A, Daguet D, Calame W, Dhoye P, Kodimule SP. Helianthus annuus seed extract affects weight and body composition of healthy obese adults during 12 weeks of consumption: a randomized, double-blind, placebo-controlled pilot study. Nutrients. (2019) 11:1080. doi: 10.3390/nu11051080
68. Saini RK, Shetty N, Giridhar P. GC-FID/MS Analysis of fatty acids in Indian cultivars of Moringa oleifera: potential sources of PUFA. J Am Oil Chem Soc. (2014) 91:1029–34. doi: 10.1007/s11746-014-2439-9
69. Saini RK, Shang XM, Ko EY, Choi JH, Kim D, Keum YS. Characterization of nutritionally important phytoconstituents in minimally processed ready-to-eat baby-leaf vegetables using HPLC-DAD and GC-MS. J Food Meas Charact. (2016) 10:341–9. doi: 10.1007/s11694-016-9312-5
70. Kim DE, Shang X, Assefa AD, Keum YS, Saini RK. Metabolite profiling of green, green/red, and red lettuce cultivars: variation in health beneficial compounds and antioxidant potential. Food Res Int. (2018) 105:361–70. doi: 10.1016/j.foodres.2017.11.028
71. Hernández-Martínez M, Gallardo-Velázquez T, Osorio-Revilla G, Castañeda-Pérez E, Uribe-Hernández K. Characterization of Mexican fishes according to fatty acid profile and fat nutritional indices. Int J Food Prop. (2016) 19:1401–12. doi: 10.1080/10942912.2015.1079787
72. Sharafi Y, Majidi MM, Goli SAH, Rashidi F. Oil content and fatty acids composition in Brassica species. Int J Food Prop. (2015) 18:2145–54. doi: 10.1080/10942912.2014.968284
73. Pereira H, Barreira L, Figueiredo F, Custódio L, Vizetto-Duarte C, Polo C, et al. Polyunsaturated fatty acids of marine macroalgae: potential for nutritional and pharmaceutical applications. Mar Drugs. (2012) 10:1920–35. doi: 10.3390/md10091920
74. Ramos Filho MM, Ramos MIL, Hiane PA, Souza EMT. Nutritional value of seven freshwater fish species from the Brazilian Pantanal. J Am Oil Chem Soc. (2010) 87:1461–7. doi: 10.1007/s11746-010-1639-1
75. Halinski LP, Topolewska A, Rynkowska A, Mika A, Urasinska M, Czerski M, et al. Impact of plant domestication on selected nutrient and anti-nutrient compounds in Solanaceae with edible leaves (Solanum spp.). Genet Rosour Crop Evol. (2019) 66:89–103. doi: 10.1007/s10722-018-0699-1
76. Ljubojevic D, Trbovic D, Lujic J, Bjelic-Cabrilo O, Kostic D, Novaov N, et al. Fatty acid composition of fishes from inland waters. Bulg J Agric Sci. (2013) 19:62–71.
77. Peltomaa E, Johnson MD, Taipale SJ. Marine cryptophytes are great sources of EPA and DHA. Mar Drugs. (2017) 16:3. doi: 10.3390/md16010003
78. Saini RK, Keum YS. Omega-3 and omega-6 polyunsaturated fatty acids: dietary sources, metabolism, and significance–a review. Life Sci. (2018) 203:255–67. doi: 10.1016/j.lfs.2018.04.049
79. Shahidi F, Ambigaipalan P. Omega-3 polyunsaturated fatty acids and their health benefits. Annu Rev Food Sci Technol. (2018) 9:345–81. doi: 10.1146/annurev-food-111317-095850
80. Burns-Whitmore B, Froyen E, Heskey C, Parker T, Pablo GS. Alpha-linolenic and linoleic fatty acids in the vegan diet: do they require dietary reference intake/adequate intake special consideration? Nutrients. (2019) 11:2365. doi: 10.3390/nu11102365
81. Bazinet RP, Layé S. Polyunsaturated fatty acids and their metabolites in brain function and disease. Nat Rev Neurosci. (2014) 15:771–85. doi: 10.1038/nrn3820
82. Long EK, Picklo MJ Sr. Trans-4-hydroxy-2-hexenal, a product of n-3 fatty acid peroxidation: make some room HNE. Free Radic Biol Med. (2010) 49:1–8. doi: 10.1016/j.freeradbiomed.2010.03.015
83. Tanaka R, Shigeta K, Sugiura Y, Hatate H, Matsushita T. Accumulation of hydroxyl lipids and 4-hydroxy-2-hexenal in live fish infected with fish diseases. Lipids. (2014) 49:385–96. doi: 10.1007/s11745-013-3875-2
84. Tao L. Oxidation of polyunsaturated fatty acids and its impact on food quality and human health. Adv Food Technol Nutr Sci Open J. (2015) 1:135–42. doi: 10.17140/AFTNSOJ-1-123
85. Ward PA. Resolvins on the way to resolution. J Exp Med. (2015) 212:1142. doi: 10.1084/jem.2128insight4
86. Zhang J, Freund MA, Culler MD, Yang R, Chen PB, Park Y, et al. How to stabilize ω-3 polyunsaturated fatty acids (PUFAs) in an animal feeding study?–Effects of the temperature, oxygen level, and antioxidant on oxidative stability of ω-3 PUFAs in a mouse diet. J Agric Food Chem. (2020) 68:1146–53. doi: 10.1021/acs.jafc.9b08298
87. Halvorsen BL, Blomhoff R. Determination of lipid oxidation products in vegetable oils and marine omega-3 supplements. Food Nutr Res. (2011) 55:5792. doi: 10.3402/fnr.v55i0.5792
88. Bastías JM, Balladares P, Acuña S, Quevedo R, Muñoz O. Determining effect of different cooking methods on the nutritional composition of salmon (Salmo salar) and Chilean jack mackerel (Trachurus murphyi) fillets. PLoS ONE. (2017) 12:e0180993. doi: 10.1371/journal.pone.0180993
89. Leung KS, Galano JM, Durand T, Lee JCY. Profiling of omega-polyunsaturated fatty acids and their oxidized products in salmon after different cooking methods. Antioxidants. (2018) 7:96. doi: 10.3390/antiox7080096
90. Damanik M, Murkovic M. The stability of palm oils during heating in a rancimat. Eur Food Res Tech. (2018) 244:1293–9. doi: 10.1007/s00217-018-3044-1
91. Hassanien MMM, Abdel-Razek AG, Rudzinska M, Siger A, Ratusz K, Przybylski R. Phytochemical contents and oxidative stability of oils from non-traditional sources. Eur J Lipid Sci Tech. (2014) 116:1563–71. doi: 10.1002/ejlt.201300475
92. Shadyro O, Sosnovskaya A, Edimecheva I. Effect of biologically active substances on oxidative stability. J Food Sci Technol. (2020) 57:243–52. doi: 10.1007/s13197-019-04054-4
93. Pérez-Matute P, Pérez-Echarri N, Martínez JA, Marti A, Moreno-Aliaga MJ. Eicosapentaenoic acid actions on adiposity and insulin resistance in control and high-fat-fed rats: Role of apoptosis, adiponectin and tumour necrosis factor-α. Brit J Nutr. (2007) 97:389–98. doi: 10.1017/S0007114507207627
94. Hassanali Z, Ametaj BN, Field CJ, Proctor SD, Vine DF. Dietary supplementation of n-3 PUFA reduces weight gain and improves postprandial lipaemia and the associated inflammatory response in the obese JCR:LA-cp rat. Diabetes Obes Metab. (2010) 12:139–47. doi: 10.1111/j.1463-1326.2009.01130.x
95. Castro GS, Deminice R, Simões-Ambrosio LMC, Calder PC, Jordão AA, Vannucchi H. Dietary docosahexaenoic acid and eicosapentaenoic acid influence liver triacylglycerol and insulin resistance in rats fed a high-fructose diet. Mar Drugs. (2015) 13:1864–81. doi: 10.3390/md13041864
96. Shang T, Liu L, Zhou J, Zhang M, Hu Q, Fang M, et al. Protective effects of various ratios of DHA/EPA supplementation on high-fat diet-induced liver damage in mice. Lipids Health Dis. (2017) 16:65. doi: 10.1186/s12944-017-0461-2
97. Huber J, Löffler M, Bilban M, Reimers M, Kadl A, Todoric J, et al. Prevention of high-fat diet-induced adipose tissue remodeling in obese diabetic mice by n-3 polyunsaturated fatty acids. Inter J Obes. (2007) 31:1004–13. doi: 10.1038/sj.ijo.0803511
98. Pauter AM, Fischer AW, Bengtsson T, Asadi A, Talamonti E, Jacobsson A. Synergistic effects of DHA and sucrose on body weight gain in PUFA-deficient Elovl2-/-mice. Nutrients. (2019) 11:852. doi: 10.3390/nu11040852
99. Soni N, Ross AB, Scheers N, Nookaew I, Gabrielsson BG, Sandberg AS. The omega-3 fatty acids EPA and DHA, as a part of a murine high-fat diet, reduced lipid accumulation in brown and white adipose tissues. Int J Mol Sci. (2019) 20:5895. doi: 10.3390/ijms20235895
100. Liu HQ, Qiu Y, Mu Y, Zhang XJ, Liu L, Hou XH, et al. A high ratio of dietary n-3/n-6 polyunsaturated fatty acids improves obesity-linked inflammation and insulin resistance through suppressing activation of TLR4 in SD rats. Nutr Res. (2013) 33:849–58. doi: 10.1016/j.nutres.2013.07.004
101. Demizieux L, Piscitelli F, Troy-Fioramonti S, Iannotti FA, Borrino S, Gresti J, et al. Early low-fat diet enriched with linolenic acid reduces liver endocannabinoid tone and improves late glycemic control after a high-fat diet challenge in mice. Diabetes. (2016) 65:1824–37. doi: 10.2337/db15-1279
102. Tyagi A, Kumar U, Reddy S, Santosh VS, Mohammed SB, Ehtesham NZ, et al. Attenuation of colonic inflammation by partial replacement of dietary linoleic acid with α-linolenic acid in a rat model of inflammatory bowel disease. BJN. (2012) 18:1612–22. doi: 10.1017/S0007114511007197
103. Mayer C, Côme M, Ulmann L, Zittelli GC, Faraloni C, Hassan N, et al. Preventive effects of the marine microalga Phaeodactylum triconutum, used as a food supplement, on risk factors associated with metabolic syndrome in Wistar rats. Nutrients. (2019) 11:1069. doi: 10.3390/nu11051069
104. Chewcharat A, Chewcharat P, Rutirapong A, Papatheodorou S. The effects of omega-3 fatty acids on diabetic nephropathy: a meta-analysis of randomized controlled trials. PLoS ONE. (2020) 15:e0228315. doi: 10.1371/journal.pone.0228315
105. Chen C, Yu X, Shao S. Effects of omega-3 fatty acid supplementation on glucose control and lipid levels in type 2 diabetes: a meta-analysis. PLoS ONE. (2015) 10:e0139565. doi: 10.1371/journal.pone.0139565
106. Du S, Jin J, Fang W, Su Q. Does fish oil have an anti-obesity effect in overweight/obese adults? A meta-analysis of randomized controlled trials. PLoS ONE. (2015) 10:e0142652. doi: 10.1371/journal.pone.0142652
107. Root M, Collier SR, Zwetsloot KA, West KL, McGinn MC. A randomized trial of fish oil omega-3 fatty acids on arterial health, inflammation, and metabolic syndrome in a young healthy population. Nutr J. (2013) 12:40. doi: 10.1186/1475-2891-12-40
108. Abdelhamid AS, Brown TJ, Brainard JS, Biswas P, Thorpe GC, Moore HJ, et al. Omega-3 fatty acids for the primary and secondary prevention of cardiovascular disease. Cochrane Database Syst Rev. (2018) 3:CD003177. doi: 10.1002/14651858.CD003177.pub4
109. Curfman G. Do omega-3 fatty acids benefit health? J Am Med Assoc. (2020) 324:2280–1. doi: 10.1001/jama.2020.22898
110. Weylandt K, Serini S, Chen YQ, Su HM, Lim K, Cittadini A, et al. Omega-3 polyunsaturated fatty acids: the way forward in times of mixed evidence. Biomed Res Int. (2015) 2015:43109. doi: 10.1155/2015/143109
111. Mani UV, Mani I, Biswas M, Kumar SN. An open-label study on the effect of flax seed powder (Linum usitatissimum) supplementation in the management of diabetes mellitus. J Diet Suppl. (2011) 8:257–65. doi: 10.3109/19390211.2011.593615
112. Zhao G, Etherton TD, Martin KR, West SG, Gillies PJ, Kris-Etherton PM. Dietary alpha-linolenic acid reduces inflammatory and lipid cardiovascular risk factors in hypercholesterolemic men and women. J Nutr. (2004) 134:2997–2004. doi: 10.1093/jn/134.11.2991
113. Maki KC, Van Elswyk ME, McCarthy D, Hess SP, Veith PE, Bell M, et al. Lipid responses to a dietary docosahexaenoic acid supplement in men and women with below average levels of high density lipoprotein cholesterol. J Am Coll Nutr. (2005) 24:189–99. doi: 10.1080/07315724.2005.10719465
114. Sanders TAB, Gleason K, Griffin B, Miller GJ. Influence of an algal triacylglycerol containing docosahexaenoic acid (22:6n-3) and docosapentaenoico acid (22:5n-6) on cardiovascular risk factors in healthy men and women. Brit J Nutr. (2006) 95:525–31. doi: 10.1079/BJN20051658
115. Satoh N, Shimatsu A, Kotani K, Sakane N, Yamada K, Suganami T, et al. Purified eicosapentaenoic acid reduces small dense LDL, remnant lipoprotein particles, and C-reactive protein in metabolic syndrome. Diabetes Care. (2007) 30:144–6. doi: 10.2337/dc06-1179
116. Yokoyama M, Origasa H, Matsuzaki M, Matsuzawa Y, Saito Y, Ishikawa Y, et al. Effects of eicosapentaenoic acid on major coronary events in hypercholesterolaemic patients (JELIS): a randomised open-label, blinded endpoint analysis. Lancet. (2007) 369:1090–8. doi: 10.1016/S0140-6736(07)60527-3
117. Tanaka K, Ishikawa Y, Yokoyama M, Origasa H, Matsuzaki M, Saito Y, et al. Reduction in the recurrence of stroke by eicosapentaenoic acid for hypercholesterolemic patients: subanalysis of the JELIS trial. Stroke. (2008) 39:2052–8. doi: 10.1161/STROKEAHA.107.509455
118. Haghiac M, Yang XH, Presley L, Smith S, Dettelback S, Minium J, et al. Dietary omega-3 fatty acid supplementation reduces inflammation in obese pregnant women: a randomized double-blind controlled clinical trial. PLoS ONE. (2015) 10:e0137309. doi: 10.1371/journal.pone.0137309
119. Elulu MS, Khaza'ai H, Patimah I, Rahmat A, Abed Y. Effect of long chain omega-3 polyunsaturated fatty acids on inflammation and metabolic markers in hypertensive and/or diabetic obese adults: a randomized controlled trial. Food Nutr Res. (2016) 60:29268. doi: 10.3402/fnr.v60.29268
120. Karnik S, Kanekar A. Childhood obesity: a global public health crisis. Int J Prev Med. (2012) 3:1–7. doi: 10.1201/b18227-3
121. Boateng GO, Adams EA, Boateng MO, Luginaah IN, Taabazuing MM. Obesity and the burden of health risks among the elderly in Ghana: a population study. PLoS ONE. (2017) 12:e0186947. doi: 10.1371/journal.pone.0186947
122. Calder PC. Omega-3 fatty acids and inflammatory processes: from molecules to man. Biochem Soc Trans. (2017) 45:1105–15. doi: 10.1042/BST20160474
123. Bocellino M, D'Angelo S. Anti-obesity effects of polyphenol intake: current status and future possibilities. Int J Mol Sci. (2020) 21:5642. doi: 10.3390/ijms21165642
124. Duvall MG, Levy BD. DHA- and EPA-derived resolvins, protectins, and maresins in airway inflammation. Eur J Pharmacol. (2016) 785:144–55. doi: 10.1016/j.ejphar.2015.11.001
125. Kwon Y. Immuno-resolving ability of resolvins, protectins, and maresins derived fromomega-3 fatty acids in metabolic syndrome. Mol Nutr Food Res. (2020) 64:e1900824. doi: 10.1002/mnfr.201900824
126. Kawabata K, Yoshioka Y, Terao J. Role of intestinal microbiota in the bioavailability and physiological functions of dietary polyphenols. Molecules. (2019) 24:370. doi: 10.3390/molecules24020370
127. Yahfoufi N, Alsadi N, Jambi M, Matar C. The immunomodulatory and anti- inflammatory role of polyphenols. Nutrients. (2018) 10:1618. doi: 10.3390/nu10111618
128. Van Meer F, Charbonnier L, Smeets PM. Food decision-making: effects of weight status and age. Curr Diab Rep. (2016) 16:84. doi: 10.1007/s11892-016-0773-z
129. Beslay M, Srour B, Méjean C, Allès B, Fiolet T, Debras C, et al. Ultra–processed food intake in association with BMI change and risk of overweight and obesity: a prospective analysis of the French NutriNet–Santé cohort. PLoS Med. (2020) 17:e1003256. doi: 10.1371/journal.pmed.1003256
130. Kumoro AC, Alhanif M, Wardhani DH. A critical review on tropical fruits seeds as prospective sources of nutritional and bioactive compounds for functional foods development: a case of Indonesian exotic fruits. Int J Food Sci. (2020) 2020:4051475. doi: 10.1155/2020/4051475
131. Torres-León C, Ramírez-Guzman N, Londoño-Hernandez L, Martinez-Medina GA, Díaz-Herrera R, Navarro-Macias V, et al. Food waste and byproducts: an opportunity to minimize malnutrition and hunger in development countries. Front Sustain Food Syst. (2018) 2:52. doi: 10.3389/fsufs.2018.00052
132. Guasch-Ferré M, Hu FB. Are fruit juices just as unhealthy as sugar-sweetened beverages? J Am Med Assoc Netw Open. (2019) 2:e193109. doi: 10.1001/jamanetworkopen.2019.3109
133. World Health Organization (2021). Global Health Observatory (GHO). Available online at: http://www.who.int/gho/ncd/risk_factors/overweight_obesity/obesity_adults/en/ (accessed August 20, 2021).
134. Hosomi R, Yoshida M, Fukunaga K. Seafood consumption and components for health. Glob J Health Sci. (2012) 4:72–86. doi: 10.5539/gjhs.v4n3p72
135. Rejman K, Górska-Warsewicz H, Kaczorowska J, Laskowski W. Nutritional significance of fruit and fruit products in the average polish diet. Nutrients. (2021) 13:2079. doi: 10.3390/nu13062079
136. Melse-Boonstra A. Bioavailability of micronutrients from nutrient-dense whole foods: zooming in on dairy, vegetable, and fruits. Front Nutr. (2020) 7:101. doi: 10.3389/fnut.2020.00101
137. Balami S, Sharma A, Karn R. Significance of nutritional value of fish for human health. Malays J Halal Res. (2019) 2:32–4. doi: 10.2478/mjhr-2019-0012
138. Kearney J. Food consumption trends and drives. Phil Trans R Soc B Biol Sci. (2010) 365:2793–807. doi: 10.1098/rstb.2010.0149
139. Sproesser G, Ruby MB, Arbit N, Akotia CS, Alvarenga MS, Bhangaokar R, et al. Understanding traditional and modern eating: the TEP10 framework. BMC Public Health. (2019) 19:1606. doi: 10.1186/s12889-019-7844-4
140. Zhang P, Wang R, Gao C, Jiang L, Lv X, Song Y, et al. Prevalence of central obesity among adults with normal BMI and its association with metabolic diseases in Northeast China. PLoS ONE. (2016) 11:e0160402. doi: 10.1371/journal.pone.0160402
141. Saklayen MG. The global epidemic of the metabolic syndrome. Curr Hypertens Rep. (2018) 20:12. doi: 10.1007/s11906-018-0812-z
142. Motevalli M, Drenowartz C, Tanous DR, Khan NA. Management of childhood obesity–time to shift from generalized to personalized intervention strategies. Nutrients. (2021) 13:1200. doi: 10.3390/nu13041200
143. Popkin BM, Adair LS, Ng SW. Now and then: The global nutrition transition: the pandemic of obesity in developing countries. Nutr Rev. (2012) 70:3–21. doi: 10.1111/j.1753-4887.2011.00456.x
Keywords: vegetable foodstuffs, fish foodstuff, metabolic diseases, α-linolenic acid, eicosapentaenoic acid, docosahexaenoic acid
Citation: Siroma TK, Machate DJ, Zorgetto-Pinheiro VA, Figueiredo PS, Marcelino G, Hiane PA, Bogo D, Pott A, Cury ERJ, Guimarães RdCA, Vilela MLB, Ferreira RdS and Nascimento VAd (2022) Polyphenols and ω-3 PUFAs: Beneficial Outcomes to Obesity and Its Related Metabolic Diseases. Front. Nutr. 8:781622. doi: 10.3389/fnut.2021.781622
Received: 18 October 2021; Accepted: 29 November 2021;
Published: 17 January 2022.
Edited by:
Zhaojun Wei, Hefei University of Technology, ChinaReviewed by:
Xiujie Jiang, Heilongjiang Bayi Agricultural University, ChinaRuijie Liu, Jiangnan University, China
Copyright © 2022 Siroma, Machate, Zorgetto-Pinheiro, Figueiredo, Marcelino, Hiane, Bogo, Pott, Cury, Guimarães, Vilela, Ferreira and Nascimento. This is an open-access article distributed under the terms of the Creative Commons Attribution License (CC BY). The use, distribution or reproduction in other forums is permitted, provided the original author(s) and the copyright owner(s) are credited and that the original publication in this journal is cited, in accordance with accepted academic practice. No use, distribution or reproduction is permitted which does not comply with these terms.
*Correspondence: Valter Aragão do Nascimento, YXJhZ2FvNjBAaG90bWFpbC5jb20=
†These authors have contributed equally to this work and share first authorship