- International Flavors & Fragrances Inc., Global Health and Nutrition Science, Danisco Sweeteners Oy, Kantvik, Finland
Optimal gut motility is central to bowel function and gut health. The link between the gut dysmotility related disorders and dysfunctional-intestinal barriers has led to a hypothesis that certain probiotics could help in normalizing gut motility and maintain gut health. This review investigates the roles of Bifidobacterium animalis subsp. lactis HN019 (B. lactis HN019™) on gut health, and its mechanisms of action in various pre-clinical and clinical studies. Research supports the hypothesis that B. lactis HN019™ has a beneficial role in maintaining intestinal barrier function during gastrointestinal infections by competing and excluding potential pathogens via different mechanisms; maintaining normal tight junction function in vitro; and regulating host immune defense toward pathogens in both in vitro and human studies. This has been observed to lead to reduced incidence of diarrhea. Interestingly, B. lactis HN019™ also supports normal physiological function in immunosenescent elderly and competes and excludes potential pathogens. Furthermore, B. lactis HN019™ reduced intestinal transit time and increased bowel movement frequency in functional constipation, potentially by modulating gut–brain–microbiota axis, mainly via serotonin signaling pathway, through short chain fatty acids derived from microbial fermentation. B. lactis HN019™ is thus a probiotic that can contribute to relieving gut dysmotility related disorders.
Introduction
Bowel function plays a central role in gut health and overall well-being. A healthy gut involves many factors, including intact epithelial barrier function, homeostatic intestinal microbiota, optimal functioning digestive organs (stomach, liver/gallbladder, pancreas), and definitely optimal gut motility. The interactions of those systems are in homeostasis in healthy subjects with normal bowel function and balanced immune function. However, this can be perturbed by antibiotic usage, unbalanced diet, and other life-style factors, infections, and other disease conditions. This may lead to changes in bowel habits and stool consistency, diarrhea, constipation, or a spectrum of both them, such as manifested in different subtypes of irritable bowel syndrome (IBS) (1–3).
The human gastrointestinal tract (GIT) represents an extremely complex ecosystem, comprising of interactions within the digestive system as well as cross-functioning with various immune cells, endocrine cells, and nerve cells, and organ systems beyond the GIT (4–6). Evidence also showed that the interactions between GIT and other organs often involve the residential intestinal microbiota, which is a dense community (estimated to 4 × 1013 microbial cells), contributing to various metabolic functions, and immunological defenses in the maintenance of normal health (7–9). Therefore, gut health has traditionally been one of the most important targets for the majority of probiotic applications, and central for connections to other health benefits via the intestinal microbiota.
Probiotics are defined as live microorganisms that, when administered in adequate amounts, confer a health benefit on the host (10). Studies have shown that probiotics are beneficial for supporting the human body's natural functions in both health and disease; either by interacting with the host directly or indirectly by optimizing the composition and/or activity of the intestinal microbiota. Although common probiotic benefits have been proposed, the core benefits are strain-specific (10).
Bifidobacterium animalis subsp. lactis HN019™ (B. lactis HN019™) is a strain originally isolated from commercial yogurt, and commercialized as an ingredient for dietary supplements, fermented and non-fermented foods, and beverages for decades (11). Its complete genome sequence was published in 2018 (12), allowing for stringent control of product quality, safety, purity, and consistency by strain identity confirmation at industrial scale. B. lactis HN019™ is available in various finished formats, granting it profound application potential in food and beverage, dietary supplement, and pharmaceutical industries.
The objective of this review is to discuss the properties of B. lactis HN019™ in the context of both preclinical and clinical studies on the following aspects of gut health; survival through the gut, modulation of intestinal microbiota, maintain intestinal barrier functions during gastrointestinal infections, regulate gut motility, and improve symptoms in constipation, assist digestion, and utilization of macronutrients, and their plausible mechanisms of action. Further, the safety of the strain will be discussed. Although the same strain has been investigated for other health benefits (13), these will not be discussed here.
Safety
Bifidobacterium animalis subsp. lactis has been documented to be present in human food since 1980 but most likely has been consumed before that. The species is listed in Inventory of Microbial Food Cultures with Safety Demonstration in Fermented Food Products (14). The European Food Safety Authority (EFSA) has included the subspecies in the Qualified Presumption of Safety list (15). In China, B. lactis HN019™ has been specifically permitted for use in infant and toddler (>1 year) food since 2011 (16), and it was accepted to be Generally Recognized as Safe (GRAS, GRN445) in US in 2012 (17).
By December 2020, there were 42 studies/clinical trials published for 27 investigated cohorts, including both healthy and compromised subjects in all age groups from newborns to elderly (Table 1). The investigational products contain B. lactis HN019™ as a single strain or combined with other probiotics and/or prebiotics. The daily dosage of B. lactis HN019™ in the products ranged from 107 to 1011 colony forming units (CFU) per day and consumption lasted for 7 days to 2 years. None of these trials have reported any safety concerns related to B. lactis HN019™ consumption, and it may be concluded that infants, children, adults, and seniors can safely consume B. lactis HN019™ at doses up to 3 × 1011 CFU/day.
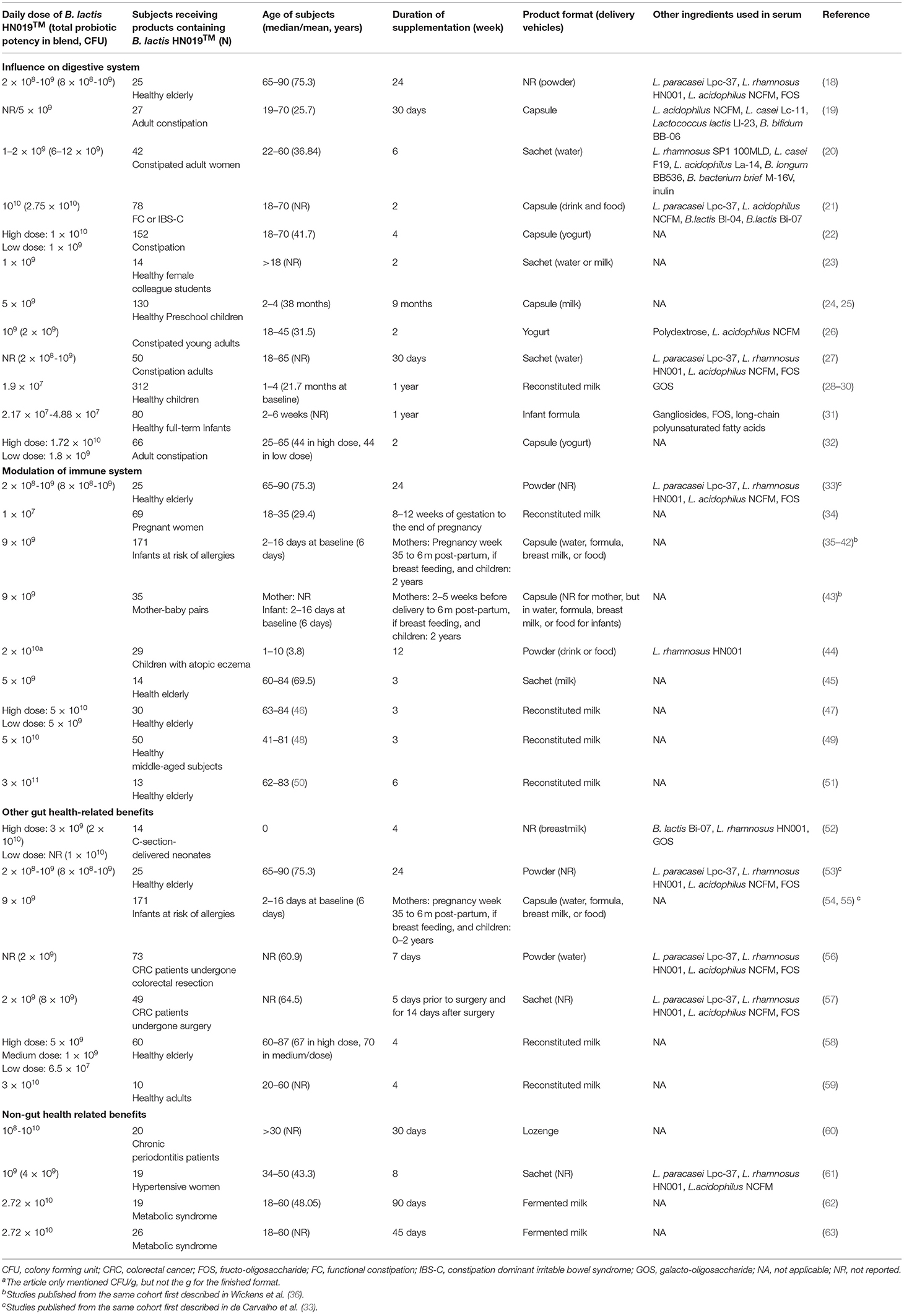
Table 1. Use of B. lactis HN019™, including probiotic blends, in human clinical trials published until December 2020.
To date, most of the published human studies for B. lactis HN019™ focused on gut health of which six studies contained B. lactis HN019™ as a single strain product in at least one arm (Table 2).
Survival Through the Gut
Although not stipulated in the definition, it is often assumed that probiotic strains should be able to survive passage through the digestive system, transiently colonize in the GIT, and potentially modulate host factors, such as immune responses, digestion, or the intestinal microbiota composition and/or activity. These probiotic attributes may be observed more pronounced in subjects with suboptimal physiological status but are often not observed in the healthy subjects. These functional characterizations can be investigated in various in vitro and/or animal models, while ultimately, health efficacy can only be confirmed by human clinical studies (64). Although adhesion is not a pre-requisite for a strain to elicit probiotic properties, interaction with intestinal epithelial cells (IECs) and intestinal mucosa is considered important for colonization and modulation of host factors. The excellent adhesion property of B. lactis HN019™ to IEC lines, such as HT29, Caco-2, and HT29-MTX, were documented in vitro in comparison with two other probiotic lactobacilli strains and a negative control (non-probiotic Lactobacillus bulgaricus) (65).
B. lactis HN019™ demonstrates high tolerance to low pH and varying resistance to bile salts in vitro, which are two important markers for assessing survival during intestinal passage (66). So far, five human clinical studies from four cohorts investigated the survival and transient colonization of B. lactis HN019™ in interventions, of which only Gopal et al. (59) identified B. lactis HN019™ at strain level (Supplementary Table 1) (24, 25, 35, 58, 59). Regardless of the variations caused by different quantitative techniques, given the observations that B. lactis/bifidobacteria was/were not detected in the control group (24) or counts decreased after the cessation of the supplementation (35, 58), it is highly likely that B. lactis/bifidobacteria detected in the probiotic group in these studies was B. lactis HN019™. These studies suggest that B. lactis HN019™ could survive and transiently persist in intestinal transit in both short-term (2–4 weeks) and long-term (>6 months) dietary interventions in almost all age groups, including infants, toddlers, pre-school children, adults, and elderly. This, notwithstanding a high inter-individual variability (0.1–68.8%) of B. lactis HN019™ colonies quantified by strain-specific probes from total fecal bifidobacteria was reported in healthy adults (59). Fecal recovery has also been assessed with products containing B. lactis HN019™ in combination with other active components, such as prebiotics where increases in B. lactis or B. lactis HN019™ were observed (31, 34). However, Horvath et al. (67) did not observe such increases with a multi-strain probiotic containing B. lactis HN019™ (67).
Modulation of Intestinal Microbiota
Bifidobacterium and Lactobacillus sensu lato have long been used in processing and preserving food and are considered as beneficial (68). Although they are not the most abundant, they are relatively stable in the adult intestinal microbiota, maintaining essential metabolic functions, such as fermentation of undigested carbohydrates into short-chain fatty acids (SCFAs), lipid metabolism, and vitamin synthesis throughout the entire lifespan (69, 70). This balance could be breached during aging, where the gut microbiota become less diverse and total bifidobacterial counts decrease (71). Therefore, sustaining these two genera at a stable level may be beneficial for maintaining a balanced healthy gut microbiota, particularly for elderly. B. lactis HN019™ can become a significant component of the normal fecal bifidobacterial population, and can increase total Lactobacillus sensu lato and Bifidobacterium spp. counts in feces, with daily consumption of 6.5 × 107 CFU to 3 × 1010 CFU (58, 59). There were no significant differences between the responses of the different dose groups, indicating that even the lowest dose (6.5 × 107 CFU/day) was able to confer desired changes with regards to these two beneficial bacterial groups in the intestinal microbiota (58). Furthermore, in these two studies fecal bifidobacterial and Lactobacilli counts decreased to baseline levels after cessation of the supplementation, remaining at 108-109 CFU/g for bifidobacteria and 107-108 CFU/g for lactobacilli. Interestingly, Ahmed et al. (58) reported significant increases in enterococci in the placebo group during the intervention with healthy elderly subjects. Although enterococci are normal members of the colonic microbiota, they are also opportunistic pathogens and may increase during aging (72). Moreover, in the same study, enterococci were reduced significantly in the same group during B. lactis HN019™ consumption, indicating that B. lactis HN019™ can reduce levels of fecal enterococci in elderly (58).
B. lactis HN019™ was shown to support maintaining the healthy/normal intestinal microbiota against aging process and by competing and excluding harmful pathogens, both at taxonomical and functional levels (25, 58, 59). In addition, further evidence for intestinal microbiota support can be found from combination products containing B. lactis HN019™. For example, both B. lactis HN019™ (109 CFU/day) alone or with polydextrose (6.25 g/day) for 2 weeks increased the proportion of fecal Bacteroides in healthy young Japanese females, while the synbiotic format had better synergistic outcomes than probiotic alone, in terms of greater proportion of this genus among the entire microbial community (23). In a recent pilot study in Chinese neonates, after 28 days supplementation with a B. lactis HN019™ synbiotic since birth, the complexity and similarity of gut microbiota in cesarean-born neonates across time and across individuals were similar to those in the vaginally-born infants (52). Furthermore, both Bifidobacterium and Lactobacillus increased in their abundances after the third day of the intervention.
These findings from B. lactis HN019™ alone or in combination with other ingredients indicate that the above-mentioned modulations of the intestinal microbiota are ubiquitous, across age and geography.
Maintain Intestinal Barrier Functions During Gastrointestinal Infections
Gastrointestinal infections often lead to diarrhea in humans, which was estimated as a leading cause of death among all ages across the world (73). Rotavirus, Shigella spp., and Salmonella spp. are the top three etiologies for diarrhea mortality, whereas the first two are typical for children under the age of five (73). The leading risk factors for diarrhea were unchanged from 2005 to 2015, which are poor hygiene in water supply and food chain, where bacterial pathogens are food poisoning organisms (73).
The gut forms the border between the inside (body) and the outside (lumen), an intestinal barrier is therefore indispensable and includes both a physical and an immunological barrier, preventing pathogenic bacteria and other harmful substances from entering the body while at the same time allowing nutrients and water to be absorbed. Intestinal barrier integrity is a prerequisite for homeostasis of mucosal function, which is balanced to maximize absorptive capacity, while maintaining efficient defensive reactions against chemical and microbial challenges.
B. lactis HN019™ Maintains Epithelium Integrity
The inner surface of the intestine consists of a layer of cells (epithelium), which are covered by a mucus layer (a viscoelastic layer consisting mainly of protein-linked carbohydrates) which plays a key role in the barrier effect mechanism.
Tight junctions (TJ) are cell–cell junctional complexes that form a continuous intercellular barrier between epithelial cells, that the major components are claudin and occludin proteins (74). These structures control and maintain balanced intestinal permeability. Increased permeability is associated with disease conditions, which are often characterized with intestinal mucosal inflammation (74). Therefore, a proper regulation of the function of TJ is important in health maintenance and disease prevention. Putaala et al. (75) showed that cell-free supernatant (CFS) of B. lactis HN019™ may increase the strength of TJ, measured as trans-epithelial electrical resistance (TEER), although not to an extent that is statistically significant, while CFS of enterohemorrhagic Escherichia coli (EHEC) reduced the TEER significantly (75).
Cyclooxygenases (COX) play a role in normal physiological function as well as fight against pathogenic bacteria. There are two isoforms of COX in humans: COX-1, contributing to the maintenance of the physiological functions, whereas COX-2 as an inducible enzyme mediates pathogenic inflammatory responses (75). In Putaala et al. (75), CFS of both B. lactis HN019™ and EHEC exhibited significant increase in the ratio of COX-2 to COX-1, but the underlying mechanisms are different. EHEC CFS induced increase of COX-2 and decrease of COX-1, whereas B. lactis HN019™ CFS induced a slightly increase of COX-2 and retained level of COX-1, confirming the immunostimulatory properties of B. lactis HN019™, which lead to the activation and maintenance of normal physiological function in elderly under immunosenescence, but not pathological inflammation (47, 76).
B. lactis HN019™ Shows Competitive Advantages Against Entero-Pathogens
Pre-treatment of EHEC with CFS of B. lactis HN019™ reduced the culturable E. coli numbers along with their invasive ability and cell association characteristics of the pathogenic strain (65). Both B. lactis HN019™ and Salmonella typhimurium adhere effectively to INT-407 cells, and the adhesive capacity of B. lactis HN019™ was similar to S. typhimurium. Nevertheless, the adhesion values obtained in co-treated assays showed that B. lactis HN019™ could significantly decrease the number of attached S. typhimurium while the number of attached B. lactis HN019™ was not affected by S. typhimurium (77).
B. lactis HN019™ Regulates Host Immune Defense Toward Pathogens
B. lactis HN019™ showed potential to protect enterocytes from inflammatory responses induced by S. typhimurium and lipopolysaccharide (LPS) in vitro. A robust interleukin (IL)-8 expression was induced when INT-407 cells were exposed to S. typhimurium. However, live and heat-killed B. lactis HN019™ not only functionally modulated the epithelium by inhibiting the constitutive mRNA level of IL-8 and attenuating S. typhimurium-induced IL-8 gene expression, but also protect the INT-407 cells from IL-8 protein production activated by LPS. Similar results were discovered on tumor necrosis factor (TNF)-α and IL-1β gene expression. TNF-α expression was up-regulated by live B. lactis HN019™, but the expression levels were much lower than with S. typhimurium (77).
Both S. typhimurium and EHEC are important causes of food poisoning under poor hygienic conditions, whereas E. coli and rotavirus are common causes of diarrhea in infants and young animals. The latter two infectious agents also commonly cause diarrhea in piglets during weaning, making piglets an ideal model for studying this type of gastrointestinal infections. The administration of B. lactis HN019™ to weaning piglets resulted in a significantly lower incidence of diarrhea during the first 2 days after weaning. The fecal levels of E. coli and rotavirus were also lower in the treatment group, which exhibited a significantly higher titer of specific antibodies in the feces (78). In addition, in mice models, B. lactis HN019™ was found to protect the animals against S. typhimurium and EHEC with lower rate of morbidity (79, 80). The probiotic-fed mice also showed significantly higher numbers of phagocytic active leukocytes (80). These findings indicated a mechanism of enhanced immune-mediated protection by B. lactis HN019™ against gastrointestinal infections. Moreover, in a large randomized trial in India, B. lactis HN019™ showed efficacy in reducing the incidence of diarrhea and fever during the rainy season (months August and September), when incidence of diarrhea is the highest (24). Fecal immunoglobulin (Ig)A and serum inflammatory marker IL-8 were also significantly decreased in the B. lactis HN019™ arm compared to placebo (24). Similar observations were made with B. lactis HN019™ in combination with galacto-oligosaccharides (GOS); reducing the incidence of dysentery and showing a trend (p = 0.08) for reduced incidence of diarrhea (28).
Regulate Gut Motility and Improve Symptoms in Constipation
Constipation without identifiable organic causes, may refer to slow-transit constipation (STC) or obstruction constipation, which are related to poor lifestyles, such as high fat/protein diet and lack of fiber and liquid intake and exercise. The typical clinical symptom of STC is the prolonged gut transit time (GTT), which may be resulted from the dysfunction of colonic smooth muscles (81).
B. lactis HN019™ showed prokinetic effects on subjects suffered functional constipation (FC). For example, daily intake of B. lactis HN019™ at both high (1.72 × 1010 CFU) and low (1.8 × 109 CFU) doses for 2 weeks decreased colonic transit time (CTT) in 100 constipated adults, with no change observed in the placebo group (32). This finding of shorten CTT was not observed in Ibarra et al. (22), who investigated the effects of B. lactis HN019™ using a similar intervention regime but longer duration (4 weeks) in 228 constipated adults diagnosed with the same ROME III criteria as in Waller et al. (32). However, a sub-group of subjects (N = 65) with bowel movement frequency (BMF) of less than three bowel movements per week (i.e., those who were constipated) benefitted from the consumption of the strain (22). After 4 weeks, daily doses of 1010 CFU (high does) and 109 CFU (low dose) increased 2 and 1.7 bowel movements/week, respectively. The stool consistency outcomes indicated that the participants in Ibarra et al. (22) were mild-constipated subjects (Bristol Stool Scale: 3.3) without changing their level of constipation after the intervention. However, Aoe et al. (23) did not observe an effect of B. lactis HN019™ alone on BMF in constipated women after 2-week supplementation. The study population was, however, under-powered; with only seven subjects per treatment group (23). In the same study, Aoe et al. (23) explored the outcomes on stool characteristics in a synbiotic intervention arm, where polydextrose (6.25 g/day) and B. lactis HN019™ (109 CFU/day) were combined. This synbiotic showed increased stool amounts which positively correlated with the relative abundance of Bacteroides. It is important to note that PDX has been reported to positively influence stool consistency and bowel function (82). Other studies with B. lactis HN019™ in combination with other probiotics or prebiotics observed improvements after intervention in bowel functions, such as shortened CTT (26), increased stool frequency and consistency (18, 27), reduced flatulence symptoms (21). However, although Botelho et al. observed a change in fecal microbiota composition, the study failed to observe a positive effect on bowel function as compared to the placebo group (19) (Table 1).
These observations on bowel habits led to the hypothesis that B. lactis HN019™ affects colonic motility patterns, which most probably via direct interactions with host factors rather than alteration of water and electrolyte secretion, even though improvements for stool amount were observed in combination with polydextrose, which is a soluble fiber. This hypothesis was investigated in an ex vivo model, where B. lactis HN019™ extract markedly increased the contractile amplitude of synchronous contractions spanning the proximal colon to the rectum. Interestingly, this effect occurred post-treatment (83). Even though the exact components of B. lactis HN019™ extract are not clear, several potential mechanistic pathways including potential active molecules will be reviewed below (Figure 1).
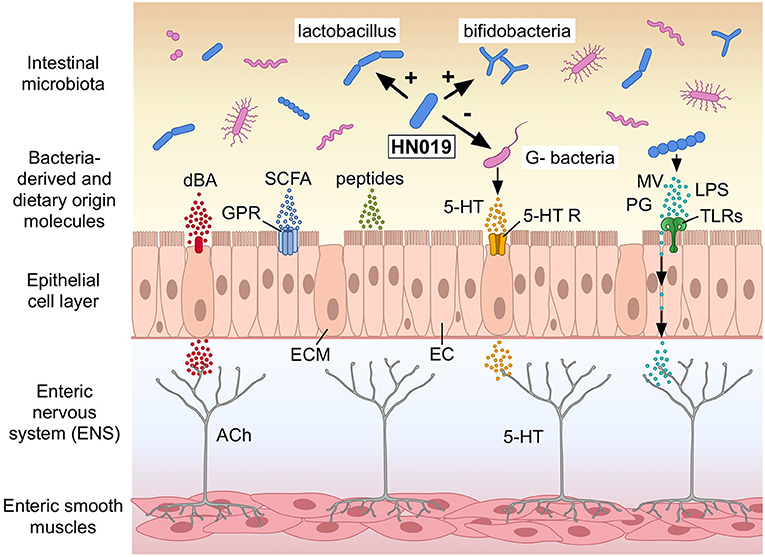
Figure 1. Potential mechanisms how Bifidobacterium lactis HN019™ (HN019) may modulate colonic motility in humans. The human intestinal epithelial cell layer comprises, among others, of epithelial cells (EC) and enterochromaffin cells (ECM) lining the gut wall. B. lactis HN019™ may stimulate Lactobacilli and Bifidobacteria and inhibit certain Gram-negative bacteria (G− bacteria). Bacteria-derived and neurotransmitters or modulators of dietary origin, such as deconjugated bile acids (dBA), short chain fatty acids (SCFA), and serotonin (5-HT) could interact with their host receptors expressed in the epithelial cell layer; G protein-coupled receptors (GPR); and 5-HT receptors (5-HT R). Microvesicles (MV), peptidoglycan (PG), and lipopolysaccharide (LPS) from different bacteria interact with Toll-like receptors (TLRs). These components may also cross the epithelial layer and like the receptors signal afferent neurons in the enteric nervous system (ENS) with among others acetylcholine (ACh) to regulate colonic motility. Modified after Dalziel et al. (2021) (with permission). ©Pinja Kettunen/SciArt and IFF, with permission.
Modulate Microbiota–Fermentation–Gut–Brain Signaling
The primary pathophysiological mechanism for constipation is gut dysmotility, presenting dysregulated or deficient colonic propulsive motor patterns (6, 84). So far, molecules reported to impact on gut motility are SCFAs, formylated peptides, and serotonin (5-hydroxytryptamine, 5-HT), which might be derived from bacterial surface structures or bacterial fermentation of nutrients (6, 85). Short-chain fatty acid concentrations can directly influence motility through the G protein-coupled receptor (GPR) 41 and GPR43, where the underlying mechanisms have been mostly addressed in animal studies (86–88). For example, intra-luminal administration of a blend of acetate, propionate and butyrate in rat was shown to lead to an increased 5-HT concentration and subsequently decreased CTT (86). B. lactis HN019™ has been shown to produce lactate and acetate, where acetate was more selective potent agonist for GPR43 (48, 89). However, there are a few studies confirming the effects of SCFAs on motility in humans (90, 91).
In addition, B. lactis HN019™ may also influence gut motility via gut commensals, which are known to be involved in serotonin biosynthesis and bile acid metabolism (92, 93). Bile salts are known to stimulate colonic contractility and transit in humans (94–96), where probiotic strains may deconjugate bile salts, leading to the formation of secondary bile salts with laxative effects (6). However, to date, no data is available on the ability of B. lactis HN019™ to deconjugate bile salts.
Improve Gastrointestinal Symptoms in Constipation
Except bowel habits, B. lactis HN019™ has been reported to reduce the frequency of functional gastrointestinal symptoms in adults, including vomiting, regurgitation, abdominal pain, nausea, gurgling, constipation, diarrhea, and flatulence (32). Most symptoms improved in the high dose (eight of nine symptoms) and low dose (seven of nine symptoms) groups, respectively, while only two of nine symptoms showed a statistically significant improvement with placebo. In Ibarra et al. (22), when comparing the measured gastrointestinal symptoms between subjects taken B. lactis HN019™ vs. placebo, only a reduction observed in the degree of straining in the sub-group of subjects (BMF<3). Improvements of gastrointestinal symptoms were also observed in studies with B. lactis HN019™ in combination with other probiotics or prebiotics (21, 27) (Table 1).
Assist Digestion and Utilization of Macronutrients
Some of the constipation related symptoms are associated with inadequate digestion of fibrous components from the diet. Plant-based diets, rich in fermentable residues, could be a solution to constipation but may be accompanied with complaints of gas produced during endogenous microbial fermentation. Aiding their digestion may thus help reduce these symptoms. In vitro, B. lactis HN019™ was shown to utilize commercial oligosaccharides: FOS, GOS, and xylo-oligosaccharide (XOS) (46, 50). Further, the analysis of complete genomes from several commercial B. lactis strains, including B. lactis HN019™ supports this, as well as the identification of several putative carbohydrate-modifying enzymes in the genome of B. lactis HN019™ for a wide range of complex carbohydrates (97, 98). This is supported by the utilization of oligosaccharides by B. lactis HN019™ seems best for mono- and di-saccharides, such as stachyose, raffinose, but not able to utilize oligosaccharides with a degree of polymerization of more than 7 (99, 100). Such oligosaccharide utilization may contribute to a reduced fermentation by the endogenous microbiota and improve tolerance to such fibers. Bifidobacterium spp. are usually non-gas producers, since in general, they metabolize monosaccharides via the fructose-6-phosphate pathway eventually to SCFAs without gas as a by-product (98, 101). Therefore, endogenous and consumed bifidobacteria, including B. lactis HN019™, could divert the fermentation in the colon toward non-gaseous end-products. This property of B. lactis HN019™ may support tolerance for the fermentation of oligosaccharides in vivo, which is in line with clinical observations of B. lactis HN019™ on reduced flatulence (21, 32). In addition, lactate and acetate are produced during B. lactis HN019™ fermentation, indicating potential roles in digestion of dietary components without differentiation of the origin; carbohydrate or protein (89, 102).
Weak indications were observed for the involvement of B. lactis HN019™ in digestion of specific dietary component(s) in human (44). In this study, a combination of L. rhamnosus HN001 and B. lactis HN019™ was examined on established atopic dermatitis (AD) in children SCORAD (SCORing Atopic Dermatitis), a measure of the extent and severity of AD, was assessed at baseline, 2 and 12 weeks after starting treatment and 4 weeks after treatment was discontinued. The supplement alleviated AD symptoms, but only in a sub-group of food-sensitized children, whereas no effect on children sensitized to environmental allergens, suggesting the beneficial effect of the probiotic may only relate to local effects on GIT, mainly toward food challenges (44). However, since the results came from sub-group analysis in children only tested for several common food allergens, the results should be interpreted with caution, and more confirmatory human studies should be carried out in larger population.
Gut microbial bile salt hydrolase (BSH) enzymes promote deconjugation, dehydrogenation, and dihydroxylation of primary bile acids, increasing the chemical diversity of bile acids, which can in turn have an impact on host lipid metabolism (93). Unconjugated bile acids are less efficient emulsifiers of dietary fats and may positively influence blood lipid profiles. Even though no data showed B. lactis HN019™ could deconjugate bile salts, as discussed earlier, B. lactis HN019™ may have a role in bile acid metabolism via affecting gut commensals, which in turn can change the amount of fat that the body is able to absorb. In addition, several studies have shown the potential of B. lactis HN019™ alone or in combination with other probiotics in improving blood lipid profiles (61, 63).
Discussion
Different aspects of digestive health are the most common health benefits addressed by probiotics (103). The above review has shown that also B. lactis HN019™ has several health benefits in this area, in particular in the area of bowel function and intestinal motility. Bifidobacterium animalis subsp. lactis is one of the most common lactic acid producing probiotics in North-America and Europe (104). Its superiority in the dietary supplement and dairy industry has yielded several well-known commercial strains, such as B. lactis DN-173 010/B. lactis CNCM I-2494 (Danone), BB-12 (Chr. Hansen), and the here discussed B. lactis HN019™ (International Flavors & Fragrances).
B. lactis DN-173010/B. lactis CNCM I-2494 in fermented milk at a dosage of 1.25 × 1010 CFU two to three times/day, has shown beneficial effects on acceleration of gastrointestinal transit in both a healthy and constipation dominant irritable bowel syndrome (IBS-C) population after 10 days to 4 weeks intervention (105–107). Moreover, this probiotic fermented milk (PFM) has been shown to improve general gastrointestinal well-being, reduce symptomatology including abdominal distention and discomfort in individuals from the general population with minor digestive symptoms but not functional gastrointestinal disorders (107–109), as well as in IBS-C population (106). These studies were all performed with fermented milk which also contained the classic yogurt cultures: S. thermophilus and L. bulgaricus. Noteworthy, both Guyonnet et al. (107) and Marteau et al. (109) recruited healthy women without any diagnosis of digestive disease, including FBD, such as IBS with normal BMF (3–21/week). This hypothesis was further confirmed, in rat model, where B. lactis CNCM I-2494 reduced stress-induced visceral hypersensitivity by restoring intestinal barrier function (110), and in a proof-of-concept study where healthy women consumed this PFM and modulation of brain activities related to mood was reported (111).
B. lactis BB-12 is another well-documented probiotic strain that has been investigated for its digestive health benefits (112). In several studies the strain has been evaluated for the effects it has on BMF. In a large study testing two doses; 109 and 1010 CFU/day for four weeks, a beneficial effect was observed especially in the lower tested dose (113). Similarly, a fermented oat product with B. lactis BB-12 was observed to normalize bowel function in elderly. In combination with Lacticaseibacillus paracasei CRL-341 softer stools were observed (114). This could suggest a potential mechanism of action. A recent study was, however, not able to replicate the effect on intestinal transit time (115); most likely because of the small number of subjects and that subjects were not selected to have long transit times or be constipated. Consumption of yogurt or a capsule with the strain was not found to change the intestinal microbiota, this is not unusual; the resilience of a healthy colonic microbiota is likely to resist change from one (probiotic) strain (116). However, consumption of the probiotic yogurt did lead to fecal recovery of the strain (115).
In summary, all three B. lactis strains discussed above do seem to have quite similar effects on bowel movements. This is confirmed by a recent meta-analysis which concluded that B. lactis may be more effective in increasing stool frequency and in improving stool consistency (117). It is therefore tempting to speculate that this relates to the high genome similarity and almost complete synteny of the genomes between various strains within the subspecies B. lactis (97, 118). The small genetic difference between strains of B. lactis could be investigated further and correlated with phenotypic traits to help understand mechanisms of action.
As manifested by the current research, serotonin signaling pathway seems to be a major effector pathway underlining the efficacy of B. lactis HN019™ on gut motility, where SCFAs derived from gut microbial fermentation seem to be promising candidate effector molecules. The contribution from other bacterial derived molecules, such as formylated peptides acting on FPR1 cannot be ruled out with the current knowledge. Although probiotics in healthy subject generally cause no or minor changes in the gut microbiota (113); it nevertheless seems to be involved in the complex interactions of B. lactis HN019™ with host metabolisms and immune defenses, including endocrine cells, immune cytokines, and neurotransmitters, which are yet to be fully understood. Therefore, future investigations on the exact role of B. lactis HN019™ on gut motility should focus on identifying key molecules and signaling pathways in the gut–brain–microbiota interactions in humans, which could enhance our understanding. To this end, focus of microbiota analysis should shift or expand to the analysis of the colonic mucosal microbiota and metabolomics of the intestinal microbiota (119). Recent scientific advances suggest great potential for bacterial-derived microvesicles (MVs) on gut health. Microvesicles from L. reuteri DSM 17938 were shown in an ex vivo mouse colon to recapitulate the effects of the whole bacteria on increasing colonic propagating contraction frequency (120). Application of L. rhamnosus JB-1 and its MVs separately to the apical side of the gut epithelium, could reduce the amplitude of propagating neural contractions in ex vivo mouse colon via interactions with ENS (121). These findings suggest a role for MVs from probiotics on signaling and their significance in the mechanisms of action within the gut–brain–microbiota axis, would therefore be potential mode of action to be investigated in the future for a better understanding of mechanisms of B. lactis HN019™.
In conclusion, B. lactis HN019™ has been observed to have benefits on different aspects of digestive health; not unlike some other commercial probiotic B. lactis strains. What is needed, however, is a better understanding of the mechanisms of action on how B. lactis HN019™ and probiotics in general function (122). Further, clinical trials should be better designed; taking into account the heterogeneity across different populations (123, 124) with sufficiently sized studies and clearly defined endpoints.
Author Contributions
JC, AL, and AO: conceptualization, review, and editing. JC and AO: original draft preparation. All authors have read and agreed to the published version of the manuscript.
Conflict of Interest
At the time of writing, the authors were employed by International Flavors & Fragrances Inc. (IFF). IFF manufactures and markets Bifidobacterium animalis subsp. lactis HN019.
Publisher's Note
All claims expressed in this article are solely those of the authors and do not necessarily represent those of their affiliated organizations, or those of the publisher, the editors and the reviewers. Any product that may be evaluated in this article, or claim that may be made by its manufacturer, is not guaranteed or endorsed by the publisher.
Acknowledgments
The authors would like to acknowledge Dr. Johanna Maukonen for constructive criticisms.
Supplementary Material
The Supplementary Material for this article can be found online at: https://www.frontiersin.org/articles/10.3389/fnut.2021.790561/full#supplementary-material
References
1. Lei YM, Nair L, Alegre ML. The interplay between the intestinal microbiota and the immune system. Clin Res Hepatol Gastroenterol. (2015) 39:9–19. doi: 10.1016/j.clinre.2014.10.008
2. Jalanka J, Spiller R. Role of microbiota in the pathogenesis of functional disorders of the lower GI tract: work in progress. Neurogastroenterol Motil. (2017) 29:1–5. doi: 10.1111/nmo.13194
3. Plaza-Diaz J, Ruiz-Ojeda FJ, Gil-Campos M, Gil A. Mechanisms of action of probiotics. Adv Nutr. (2019) 10(Suppl_1):S49–66. doi: 10.1093/advances/nmy063
4. Kechagia M, Basoulis D, Konstantopoulou S, Dimitriadi D, Gyftopoulou K, Skarmoutsou N, et al. Health benefits of probiotics: a review. ISRN Nutr. (2013) 2013:481651. doi: 10.5402/2013/481651
5. de Moreno de LeBlanc A, LeBlanc JG. Effect of probiotic administration on the intestinal microbiota, current knowledge and potential applications. World J Gastroenterol. (2014) 20:16518–28. doi: 10.3748/wjg.v20.i44.16518
6. Dimidi E, Christodoulides S, Scott SM, Whelan K. Mechanisms of action of probiotics and the gastrointestinal microbiota on gut motility and constipation. Adv. Nutr. (2017) 8:484–94. doi: 10.3945/an.116.014407
7. Vighi G, Marcucci F, Sensi L, Di Cara G, Frati F. Allergy and the gastrointestinal system. Clin Exp Immunol. (2008) 153(Suppl 1):3–6. doi: 10.1111/j.1365-2249.2008.03713.x
8. Sender R, Fuchs S, Milo R. Revised estimates for the number of human and bacteria cells in the body. PLoS Biol. (2016) 14:e1002533. doi: 10.1371/journal.pbio.1002533
9. Rowland I, Gibson G, Heinken A, Scott K, Swann J, Thiele I, et al. Gut microbiota functions: metabolism of nutrients and other food components. Eur J Nutr. (2018) 57:1–24. doi: 10.1007/s00394-017-1445-8
10. Hill C, Guarner F, Reid G, Gibson GR, Merenstein DJ, Pot B, et al. Expert consensus document. The International Scientific Association for Probiotics and Prebiotics consensus statement on the scope and appropriate use of the term probiotic. Nat Rev Gastroenterol Hepatol. (2014) 11:506–14. doi: 10.1038/nrgastro.2014.66
11. Sanders ME. Summary of probiotic activities of Bifidobacterium lactis HN019. J Clin Gastroenterol. (2006) 40:776–83. doi: 10.1097/01.mcg.0000225576.73385.f0
12. Morovic W, Roos P, Zabel B, Hidalgo-Cantabrana C, Kiefer A, Barrangou R. Transcriptional and functional analysis of Bifidobacterium animalis subsp. lactis exposure to tetracycline. Appl Environ Microbiol. (2018) 84:e01999-18. doi: 10.1128/AEM.01999-18
13. Miller LE, Lehtoranta L, Lehtinen MJ. Short-term probiotic supplementation enhances cellular immune function in healthy elderly: systematic review and meta-analysis of controlled studies. Nutr Res. (2019) 64:1–8. doi: 10.1016/j.nutres.2018.12.011
14. Bourdichon F, Alper I, Bibiloni R, Dubois A, Laulund S, Miks M, et al. Inventory of microbial food cultures with safety demonstration in fermented food products. Bull Int Dairy Federat. (2018) 495:5–71.
15. Ricci A, Allende A, Bolton D, Chemaly M, Davies R, Girones R, et al. Scientific opinion on the update of the list of QPS-recommended biological agents intentionally added to food or feed as notified to EFSA. EFSA J. (2017) 15:e04664. doi: 10.2903/j.efsa.2017.4664
16. National Health Commission of the People's Republic of China. Food Safety Standards, Risk Surveillance and Assessment. (2011). Available online at: http://www.nhc.gov.cn/sps/s7891/201111/a10fe4a0b1dd477c9884649220368cc2.shtml (accessed November 12, 2021).
18. Valentini Neto J, Chella TP, Rudnik DP, Ribeiro SML. Effects of synbiotic supplementation on gut functioning and systemic inflammation of community-dwelling elders - secondary analyses from a randomized clinical trial. Arq Gastroenterol. (2020) 57:24–30. doi: 10.1590/s0004-2803.202000000-06
19. Botelho PB, Ferreira MVR, Araujo AM, Mendes MM, Nakano EY. Effect of multispecies probiotic on gut microbiota composition in individuals with intestinal constipation: a double-blind, placebo-controlled randomized trial. Nutrition. (2020) 78:110890. doi: 10.1016/j.nut.2020.110890
20. Maria Ferreira Pinheiro J, Nazaré Batista M, de Lima e Silva C, Nunes de Lima Barroso F, Torres de Paiva G, de Sousa Rebouças A, et al. Efeito de um mix de probióticos na constipação funcional de mulheres adultas: um estudo randomizado duplo-cego. O Mun Saúd. (2019) 43:622–39. doi: 10.15343/0104-7809.20194303622639
21. Airaksinen K, Yeung N, Lyra A, Lahtinen SJ, Huttunen T, Shanahan F, et al. The effect of a probiotic blend on gastrointestinal symptoms in constipated patients: a double blind, randomised, placebo controlled 2-week trial. Benef Microbes. (2019) 10:617–27. doi: 10.3920/BM2018.0163
22. Ibarra A, Latreille-Barbier M, Donazzolo Y, Pelletier X, Ouwehand AC. Effects of 28-day Bifidobacterium animalis subsp. lactis HN019 supplementation on colonic transit time and gastrointestinal symptoms in adults with functional constipation: a double-blind, randomized, placebo-controlled, and dose-ranging trial. Gut Microbes. (2018) 9:236–51. doi: 10.1080/19490976.2017.1412908
23. Aoe S, Nakazawa Y, Ohmisya S. Combined effect of a probiotic (Bifidobacterium) and a prebiotic (polydextrose) on the fecal microbiota and stool parameters of healthy young women. Integr Mol Med. (2017) 4:5. doi: 10.15761/imm.1000306
24. Hemalatha R, Ouwehand AC, Forssten SD, Geddan JB, Mamidi RS, Bhaskar V, et al. A community-based randomized double blind controlled trial of Lactobacillus paracasei and Bifidobacterium lactis on reducing risk for diarrhea and fever in preschool children in an urban slum in India. Eur J Food Res Rev. (2014) 4:325–41. doi: 10.9734/EJNFS/2014/8280
25. Hemalatha R, Ouwehand AC, Saarinen MT, Prasad UV, Swetha K, Bhaskar V. Effect of probiotic supplementation on total Lactobacilli, bifidobacteria and short chain fatty acids in 2-5-year-old children. Microb Ecol Health Dis. (2017) 28:1298340. doi: 10.1080/16512235.2017.1298340
26. Magro DO, de Oliveira LM, Bernasconi I, Ruela Mde S, Credidio L, Barcelos IK, et al. Effect of yogurt containing polydextrose, Lactobacillus acidophilus NCFM and Bifidobacterium lactis HN019: a randomized, double-blind, controlled study in chronic constipation. Nutr J. (2014) 13:75. doi: 10.1186/1475-2891-13-75
27. Waitzberg DL, Logullo LC, Bittencourt AF, Torrinhas RS, Shiroma GM, Paulino NP, et al. Effect of synbiotic in constipated adult women - a randomized, double-blind, placebo-controlled study of clinical response. Clin Nutr. (2013) 32:27–33. doi: 10.1016/j.clnu.2012.08.010
28. Sazawal S, Dhingra U, Hiremath G, Sarkar A, Dhingra P, Dutta A, et al. Prebiotic and probiotic fortified milk in prevention of morbidities among children: community-based, randomized, double-blind, controlled trial. PLoS ONE. (2010) 5:e12164. doi: 10.1371/journal.pone.0012164
29. Sazawal S, Dhingra U, Sarkar A, Dhingra P, Deb S, Marwah D, et al. Efficacy of milk fortified with a probiotic Bifidobacterium lactis (DR-10) and prebiotic galacto-oligosaccharides in prevention of morbidity and on nutritional status. Asia Pac J Clin Nutr. (2004) 13(Suppl):S28.
30. Prasad J, Sazawal S, Dhingra U, Gopal PK. Detection of viable Bifidobacterium lactis HN019 (DR10™) in stools of children during a synbiotic dietary intervention trial. Int Dairy J. (2013) 30:64–7. doi: 10.1016/j.idairyj.2012.12.001
31. Oswari H, Prayitno L, Dwipoerwantoro PG, Firmansyah A, Makrides M, Lawley B, et al. Comparison of stool microbiota compositions, stool alpha1-antitrypsin and calprotectin concentrations, and diarrhoeal morbidity of Indonesian infants fed breast milk or probiotic/prebiotic-supplemented formula. J Paediatr Child Health. (2013) 49:1032–9. doi: 10.1111/jpc.12307
32. Waller PA, Gopal PK, Leyer GJ, Ouwehand AC, Reifer C, Stewart ME, et al. Dose-response effect of Bifidobacterium lactis HN019 on whole gut transit time and functional gastrointestinal symptoms in adults. Scand J Gastroenterol. (2011) 46:1057–64. doi: 10.3109/00365521.2011.584895
33. de Carvalho CMRG, Luzia LA, Rondó PH, Ribeiro SML. Associations between vitamin D, systemic inflammation and synbiotic supplementation: secondary study from a randomized clinical trial. Nutrire. (2019) 45:4. doi: 10.1186/s41110-019-0104-7
34. Wibowo N, Bardosono S, Irwinda R. Effects of Bifidobacterium animalis lactis HN019 (DR10TM), inulin, and micronutrient fortified milk on faecal DR10TM, immune markers, and maternal micronutrients among Indonesian pregnant women. Asia Pac J Clin Nutr. (2016) 25(Suppl 1):S102–10. doi: 10.6133/apjcn.122016.s2
35. Murphy R, Morgan XC, Wang XY, Wickens K, Purdie G, Fitzharris P, et al. Eczema-protective probiotic alters infant gut microbiome functional capacity but not composition: sub-sample analysis from a RCT. Benef Microbes. (2019) 10:5–17. doi: 10.3920/BM2017.0191
36. Wickens K, Black PN, Stanley TV, Mitchell E, Fitzharris P, Tannock GW, et al. A differential effect of 2 probiotics in the prevention of eczema and atopy: a double-blind, randomized, placebo-controlled trial. J Allergy Clin Immunol. (2008) 122:788–94. doi: 10.1016/j.jaci.2008.07.011
37. Dekker JW, Wickens K, Black PN, Stanley TV, Mitchell EA, Fitzharris P, et al. Safety aspects of probiotic bacterial strains Lactobacillus rhamnosus HN001 and Bifidobacterium animalis subsp. lactis HN019 in human infants aged 0–2 years. Int Dairy J. (2009) 19:149–54. doi: 10.1016/j.idairyj.2008.10.004
38. Wickens K, Black P, Stanley TV, Mitchell E, Barthow C, Fitzharris P, et al. A protective effect of Lactobacillus rhamnosus HN001 against eczema in the first 2 years of life persists to age 4 years. Clin Exp Allergy. (2012) 42:1071–9. doi: 10.1111/j.1365-2222.2012.03975.x
39. Wickens K, Stanley TV, Mitchell EA, Barthow C, Fitzharris P, Purdie G, et al. Early supplementation with Lactobacillus rhamnosus HN001 reduces eczema prevalence to 6 years: does it also reduce atopic sensitization? Clin Exp Allergy. (2013) 43:1048–57. doi: 10.1111/cea.12154
40. Morgan AR, Han DY, Wickens K, Barthow C, Mitchell EA, Stanley TV, et al. Differential modification of genetic susceptibility to childhood eczema by two probiotics. Clin Exp Allergy. (2014) 44:1255–65. doi: 10.1111/cea.12394
41. Marlow G, Han DY, Wickens K, Stanley T, Crane J, Mitchell EA, et al. Differential effects of two probiotics on the risks of eczema and atopy associated with single nucleotide polymorphisms to Toll-like receptors. Pediatr Allergy Immunol. (2015) 26:262–71. doi: 10.1111/pai.12371
42. Wickens K, Barthow C, Mitchell EA, Kang J, van Zyl N, Purdie G, et al. Effects of Lactobacillus rhamnosus HN001 in early life on the cumulative prevalence of allergic disease to 11 years. Pediatr Allergy Immunol. (2018) 29:808–14. doi: 10.1111/pai.12982
43. Prescott SL, Wickens K, Westcott L, Jung W, Currie H, Black PN, et al. Supplementation with Lactobacillus rhamnosus or Bifidobacterium lactis probiotics in pregnancy increases cord blood interferon-gamma and breast milk transforming growth factor-beta and immunoglobin A detection. Clin Exp Allergy. (2008) 38:1606–14. doi: 10.1111/j.1365-2222.2008.03061.x
44. Sistek D, Kelly R, Wickens K, Stanley T, Fitzharris P, Crane J. Is the effect of probiotics on atopic dermatitis confined to food sensitized children? Clin Exp Allergy. (2006) 36:629–33. doi: 10.1111/j.1365-2222.2006.02485.x
45. Gill HS, Rutherfurd KJ, Cross ML. Dietary probiotic supplementation enhances natural killer cell activity in the elderly: an investigation of age-related immunological changes. J Clin Immunol. (2001) 21:264–71. doi: 10.1023/a:1010979225018
46. Gopal PK, Sullivan PA, Smart JB. Utilisation of galacto-oligosaccharides as selective substrates for growth by lactic acid bacteria including Bifidobacterium lactis DR10 and Lactobacillus rhamnosus DR20. Int Dairy J. (2001) 11:19–25. doi: 10.1016/S0958-6946(01)00026-7
47. Gill HS, Rutherfurd KJ, Cross ML, Gopal PK. Enhancement of immunity in the elderly by dietary supplementation with the probiotic Bifidobacterium lactis HN019. Am J Clin Nutr. (2001) 74:833–9. doi: 10.1093/ajcn/74.6.833
48. Le Poul E, Loison C, Struyf S, Springael JY, Lannoy V, Decobecq ME, et al. Functional characterization of human receptors for short chain fatty acids and their role in polymorphonuclear cell activation. J Biol Chem. (2003) 278:25481–9. doi: 10.1074/jbc.M301403200
49. Chiang BL, Sheih YH, Wang LH, Liao CK, Gill HS. Enhancing immunity by dietary consumption of a probiotic lactic acid bacterium (Bifidobacterium lactis HN019): optimization and definition of cellular immune responses. Eur J Clin Nutr. (2000) 54:849–55. doi: 10.1038/sj.ejcn.1601093
50. Mäkeläinen H, Saarinen M, Stowell J, Rautonen N, Ouwehand AC. Xylo-oligosaccharides and lactitol promote the growth of Bifidobacterium lactis and Lactobacillus species in pure cultures. Benef Microbes. (2010) 1:139–48. doi: 10.3920/BM2009.0029
51. Arunachalam K, Gill HS, Chandra RK. Enhancement of natural immune function by dietary consumption of Bifidobacterium lactis (HN019). Eur J Clin Nutr. (2000) 54:263–7. doi: 10.1038/sj.ejcn.1600938
52. Yang W, Tian L, Luo J, Yu J. Ongoing supplementation of probiotics to cesarean-born neonates during the first month of life may impact the gut microbial. Am J Perinatol. (2020). 38:1181–91. doi: 10.1055/s-0040-1710559
53. Louzada ER, Ribeiro SML. Synbiotic supplementation, systemic inflammation, and symptoms of brain disorders in elders: a secondary study from a randomized clinical trial. Nutr Neurosci. (2020) 23:93–100. doi: 10.1080/1028415X.2018.1477349
54. Slykerman RF, Kang J, Van Zyl N, Barthow C, Wickens K, Stanley T, et al. Effect of early probiotic supplementation on childhood cognition, behaviour and mood a randomised, placebo-controlled trial. Acta Paediatr. (2018) 107:2172–8. doi: 10.1111/apa.14590
55. Slykerman RF, Coomarasamy C, Wickens K, Thompson JMD, Stanley TV, Barthow C, et al. Exposure to antibiotics in the first 24 months of life and neurocognitive outcomes at 11 years of age. Psychopharmacology (Berl). (2019) 236:1573–82. doi: 10.1007/s00213-019-05216-0
56. Polakowski CB, Kato M, Preti VB, Schieferdecker MEM, Ligocki Campos AC. Impact of the preoperative use of synbiotics in colorectal cancer patients: a prospective, randomized, double-blind, placebo-controlled study. Nutrition. (2019) 58:40–6. doi: 10.1016/j.nut.2018.06.004
57. Flesch AT, Tonial ST, Contu PC, Damin DC. Perioperative synbiotics administration decreases postoperative infections in patients with colorectal cancer: a randomized, double-blind clinical trial. Rev Col Bras Cir. (2017) 44:567–73. doi: 10.1590/0100-69912017006004
58. Ahmed M, Prasad J, Gill H, Stevenson L, Gopal P. Impact of consumption of different levels of Bifidobacterium lactis HN019 on the intestinal microflora of elderly human subjects. J Nutr Health Aging. (2007) 11:26–31.
59. Gopal PK, Prasad J, Gill HS. Effects of the consumption of Bifidobacterium lactis HN019 (DR10 TM) and galacto-oligosaccharides on the microflora of the gastrointestinal tract in human subjects. Nutr Res. (2003) 23:1313–28. doi: 10.1016/S0271-5317(03)00134-9
60. Invernici MM, Salvador SL, Silva PHF, Soares MSM, Casarin R, Palioto DB, et al. Effects of Bifidobacterium probiotic on the treatment of chronic periodontitis: a randomized clinical trial. J Clin Periodontol. (2018) 45:1198–210. doi: 10.1111/jcpe.12995
61. Romao da Silva LF, de Oliveira Y, de Souza EL, de Luna Freire MO, Braga VA, Magnani M, et al. Effects of probiotic therapy on cardio-metabolic parameters and autonomic modulation in hypertensive women: a randomized, triple-blind, placebo-controlled trial. Food Funct. (2020) 11:7152–63. doi: 10.1039/d0fo01661f
62. Bernini LJ, Simao ANC, de Souza CHB, Alfieri DF, Segura LG, Costa GN, et al. Effect of Bifidobacterium lactis HN019 on inflammatory markers and oxidative stress in subjects with and without the metabolic syndrome. Br J Nutr. (2018) 120:645–52. doi: 10.1017/S0007114518001861
63. Bernini LJ, Simao AN, Alfieri DF, Lozovoy MA, Mari NL, de Souza CH, et al. Beneficial effects of Bifidobacterium lactis on lipid profile and cytokines in patients with metabolic syndrome: a randomized trial. Effects of probiotics on metabolic syndrome. Nutrition. (2016) 32:716–9. doi: 10.1016/j.nut.2015.11.001
64. Forssten SD, Laitila A, Maukonen J, Ouwehand AC. Probiotic triangle of success; strain production, clinical studies and product development. FEMS Microbiol Lett. (2020) 367:fnaa167. doi: 10.1093/femsle/fnaa167
65. Gopal PK, Prasad J, Smart J, Gill HS. In vitro adherence properties of Lactobacillus rhamnosus DR20 and Bifidobacterium lactis DR10 strains and their antagonistic activity against an enterotoxigenic Escherichia coli. Int J Food Microbiol. (2001) 67:207–16. doi: 10.1016/s0168-1605(01)00440-8
66. Prasad J, Gill H, Smart J, Gopal PK. Selection and characterisation of Lactobacillus and Bifidobacterium strains for use as probiotics. Int Dairy J. (1998) 8:993–1002. doi: 10.1016/S0958-6946(99)00024-2
67. Horvath A, Leber B, Feldbacher N, Steinwender M, Komarova I, Rainer F, et al. The effects of a multispecies synbiotic on microbiome-related side effects of long-term proton pump inhibitor use: a pilot study. Sci Rep. (2020) 10:2723. doi: 10.1038/s41598-020-59550-x
68. Ayivi RD, Gyawali R, Krastanov A, Aljaloud SO, Worku M, Tahergorabi R, et al. Lactic acid bacteria: food safety and human health applications. Dairy. (2020) 1:202–32. doi: 10.3390/dairy1030015
69. Gerritsen J, Smidt H, Rijkers GT, de Vos WM. Intestinal microbiota in human health and disease: the impact of probiotics. Genes Nutr. (2011) 6:209–40. doi: 10.1007/s12263-011-0229-7
70. Rinninella E, Raoul P, Cintoni M, Franceschi F, Miggiano GAD, Gasbarrini A, et al. What is the healthy gut microbiota composition? A changing ecosystem across age, environment, diet, and diseases. Microorganisms. (2019). 7:14. doi: 10.3390/microorganisms7010014
71. Dinan TG, Cryan JF. Gut instincts: microbiota as a key regulator of brain development, ageing and neurodegeneration. J Physiol. (2017) 595:489–503. doi: 10.1113/JP273106
72. Biagi E, Nylund L, Candela M, Ostan R, Bucci L, Pini E, et al. Through ageing, and beyond: gut microbiota and inflammatory status in seniors and centenarians. PLoS ONE. (2010) 5:e10667. doi: 10.1371/journal.pone.0010667
73. Troeger C, Forouzanfar M, Rao PC, Khalil I, Brown A, Reiner RC Jr. Estimates of global, regional, and national morbidity, mortality, and aetiologies of diarrhoeal diseases: a systematic analysis for the Global Burden of Disease Study 2015. Lancet Infect Dis. (2017) 17:909–48. doi: 10.1016/S1473-3099(17)30276-1
74. Bischoff SC, Barbara G, Buurman W, Ockhuizen T, Schulzke JD, Serino M, et al. Intestinal permeability–a new target for disease prevention and therapy. BMC Gastroenterol. (2014) 14:189. doi: 10.1186/s12876-014-0189-7
75. Putaala H, Salusjarvi T, Nordstrom M, Saarinen M, Ouwehand AC, Bech Hansen E, et al. Effect of four probiotic strains and Escherichia coli O157:H7 on tight junction integrity and cyclo-oxygenase expression. Res Microbiol. (2008) 159:692–8. doi: 10.1016/j.resmic.2008.08.002
76. Zhou JS, Gill HS. Immunostimulatory probiotic Lactobacillus rhamnosus HN001 and Bifidobacterium lactis HN019 do not induce pathological inflammation in mouse model of experimental autoimmune thyroiditis. Int J Food Microbiol. (2005) 103:97–104. doi: 10.1016/j.ijfoodmicro.2004.11.031
77. Liu C, Zhang ZY, Dong K, Guo XK. Adhesion and immunomodulatory effects of Bifidobacterium lactis HN019 on intestinal epithelial cells INT-407. World J Gastroenterol. (2010) 16:2283–90. doi: 10.3748/wjg.v16.i18.2283
78. Shu Q, Qu F, Gill HS. Probiotic treatment using Bifidobacterium lactis HN019 reduces weanling diarrhea associated with rotavirus and Escherichia coli infection in a piglet model. J Pediatr Gastroenterol Nutr. (2001) 33:171–7. doi: 10.1097/00005176-200108000-00014
79. Shu Q, Lin H, Rutherfurd KJ, Fenwick SG, Prasad J, Gopal PK, et al. Dietary Bifidobacterium lactis (HN019) enhances resistance to oral Salmonella typhimurium infection in mice. Microbiol Immunol. (2000) 44:213–22. doi: 10.1111/j.1348-0421.2000.tb02486.x
80. Shu Q, Gill HS. A dietary probiotic (Bifidobacterium lactis HN019) reduces the severity of Escherichia coli O157:H7 infection in mice. Med Microbiol Immunol. (2001) 189:147–52. doi: 10.1007/s430-001-8021-9
81. Dimidi E, Christodoulides S, Fragkos KC, Scott SM, Whelan K. The effect of probiotics on functional constipation in adults: a systematic review and meta-analysis of randomized controlled trials. Am J Clin Nutr. (2014) 100:1075–84. doi: 10.3945/ajcn.114.089151
82. Hengst C, Ptok S, Roessler A, Fechner A, Jahreis G. Effects of polydextrose supplementation on different faecal parameters in healthy volunteers. Int J Food Sci Nutr. (2009) 60(Suppl 5):96–105. doi: 10.1080/09637480802526760
83. Dalziel JE, Anderson RC, Peters JS, Lynch AT, Spencer NJ, Dekker J, et al. Promotility action of the probiotic Bifidobacterium lactis HN019 extract compared with prucalopride in isolated rat large intestine. Front Neurosci. (2017) 11:20. doi: 10.3389/fnins.2017.00020
84. Spiller R. Recent advances in understanding the role of serotonin in gastrointestinal motility in functional bowel disorders: alterations in 5-HT signalling and metabolism in human disease. Neurogastroenterol Motil. (2007) 19(Suppl 2):25–31. doi: 10.1111/j.1365-2982.2007.00965.x
85. Chandrasekharan B, Saeedi BJ, Alam A, Houser M, Srinivasan S, Tansey M, et al. Interactions between commensal bacteria and enteric neurons, via FPR1 induction of ROS, increase gastrointestinal motility in mice. Gastroenterology. (2019) 157:179.e2–92.e2. doi: 10.1053/j.gastro.2019.03.045
86. Fukumoto S, Tatewaki M, Yamada T, Fujimiya M, Mantyh C, Voss M, et al. Short-chain fatty acids stimulate colonic transit via intraluminal 5-HT release in rats. Am J Physiol Regul Integr Comp Physiol. (2003) 284:R1269–76. doi: 10.1152/ajpregu.00442.2002
87. Mitsui R, Ono S, Karaki S, Kuwahara A. Neural and non-neural mediation of propionate-induced contractile responses in the rat distal colon. Neurogastroenterol Motil. (2005) 17:585–94. doi: 10.1111/j.1365-2982.2005.00669.x
88. Tazoe H, Otomo Y, Kaji I, Tanaka R, Karaki S, Kuwahara A. Roles of short-chain fatty acids receptors, GPR41 and GPR43 on colonic functions. J Physiol Pharmacol. (2008) 59:251–62.
89. Henrique-Bana F, Macedo F Jr, Oliveira L, Takihara A, Miglioranza L, Spinosa W, et al. Bifidobacterium lactis HN019: survival, acid production and impact on sensory acceptance of fermented milk. Int Food Res J. (2019). 26:695–703.
90. Kamath PS, Phillips SF, Zinsmeister AR. Short-chain fatty acids stimulate ileal motility in humans. Gastroenterology. (1988) 95:1496–502. doi: 10.1016/s0016-5085(88)80068-4
91. Coremans G, Tack J, Vantrappen G, Janssens J, Annese V. Is the irritable bowel really irritable? Ital J Gastroenterol. (1991) 23(8 Suppl 1):39–40.
92. Yano JM, Yu K, Donaldson GP, Shastri GG, Ann P, Ma L, et al. Indigenous bacteria from the gut microbiota regulate host serotonin biosynthesis. Cell. (2015) 161:264–76. doi: 10.1016/j.cell.2015.02.047
93. Enright EF, Griffin BT, Gahan CGM, Joyce SA. Microbiome-mediated bile acid modification: role in intestinal drug absorption and metabolism. Pharmacol Res. (2018) 133:170–86. doi: 10.1016/j.phrs.2018.04.009
94. Rao AS, Wong BS, Camilleri M, Odunsi-Shiyanbade ST, McKinzie S, Ryks M, et al. Chenodeoxycholate in females with irritable bowel syndrome-constipation: a pharmacodynamic and pharmacogenetic analysis. Gastroenterology. (2010) 139:1549–58, 1558.e1. doi: 10.1053/j.gastro.2010.07.052
95. Wong BS, Camilleri M, McKinzie S, Burton D, Graffner H, Zinsmeister AR. Effects of A3309, an ileal bile acid transporter inhibitor, on colonic transit and symptoms in females with functional constipation. Am J Gastroenterol. (2011) 106:2154–64. doi: 10.1038/ajg.2011.285
96. Wong BS, Camilleri M, Carlson P, McKinzie S, Busciglio I, Bondar O, et al. Increased bile acid biosynthesis is associated with irritable bowel syndrome with diarrhea. Clin Gastroenterol Hepatol. (2012) 10:1009.e3–15.e3. doi: 10.1016/j.cgh.2012.05.006
97. Barrangou R, Briczinski EP, Traeger LL, Loquasto JR, Richards M, Horvath P, et al. Comparison of the complete genome sequences of Bifidobacterium animalis subsp. lactis DSM 10140 and Bl-04. J Bacteriol. (2009) 191:4144–51. doi: 10.1128/JB.00155-09
98. Pokusaeva K, Fitzgerald GF, van Sinderen D. Carbohydrate metabolism in Bifidobacteria. Genes Nutr. (2011) 6:285–306. doi: 10.1007/s12263-010-0206-6
99. Sims IM, Ryan JL, Kim SH. In vitro fermentation of prebiotic oligosaccharides by Bifidobacterium lactis HN019 and Lactobacillus spp. Anaerobe. (2014) 25:11–7. doi: 10.1016/j.anaerobe.2013.11.001
100. Zartl B, Silberbauer K, Loeppert R, Viernstein H, Praznik W, Mueller M. Fermentation of non-digestible raffinose family oligosaccharides and galactomannans by probiotics. Food Funct. (2018) 9:1638–46. doi: 10.1039/c7fo01887h
101. Amann MM, Kullen MJ, Martini MC, Busta FF, Brady LJ. Consumption of exogenous bifidobacteria does not alter fecal bifidobacteria and breath hydrogen excretion in humans. J Nutr. (1998) 128:996–1002. doi: 10.1093/jn/128.6.996
102. Henrique-Bana FC, Wang X, Costa GN, Spinosa WA, Miglioranza LHS, Scorletti E, et al. In vitro effects of Bifidobacterium lactis-based synbiotics on human faecal bacteria. Food Res Int. (2020) 128:108776. doi: 10.1016/j.foodres.2019.108776
103. Dronkers TMG, Ouwehand AC, Rijkers GT. Data on global analysis of clinical trials with probiotics. Data Brief. (2020) 32:106269. doi: 10.1016/j.dib.2020.106269
104. Sharma M, Wasan A, Sharma RK. Recent developments in probiotics: an emphasis on Bifidobacterium. Food Biosci. (2021) 41:100993. doi: 10.1016/j.fbio.2021.100993
105. Marteau P, Cuillerier E, Meance S, Gerhardt MF, Myara A, Bouvier M, et al. Bifidobacterium animalis strain DN-173 010 shortens the colonic transit time in healthy women: a double-blind, randomized, controlled study. Aliment Pharmacol Ther. (2002) 16:587–93. doi: 10.1046/j.1365-2036.2002.01188.x
106. Agrawal A, Houghton LA, Morris J, Reilly B, Guyonnet D, Goupil Feuillerat N, et al. Clinical trial: the effects of a fermented milk product containing Bifidobacterium lactis DN-173 010 on abdominal distension and gastrointestinal transit in irritable bowel syndrome with constipation. Aliment Pharmacol Ther. (2009) 29:104–14. doi: 10.1111/j.1365-2036.2008.03853.x
107. Guyonnet D, Schlumberger A, Mhamdi L, Jakob S, Chassany O. Fermented milk containing Bifidobacterium lactis DN-173 010 improves gastrointestinal well-being and digestive symptoms in women reporting minor digestive symptoms: a randomised, double-blind, parallel, controlled study. Br J Nutr. (2009) 102:1654–62. doi: 10.1017/S0007114509990882
108. Guyonnet D, Woodcock A, Stefani B, Trevisan C, Hall C. Fermented milk containing Bifidobacterium lactis DN-173 010 improved self-reported digestive comfort amongst a general population of adults. A randomized, open-label, controlled, pilot study. J Dig Dis. (2009) 10:61–70. doi: 10.1111/j.1751-2980.2008.00366.x
109. Marteau P, Guyonnet D, Lafaye de Micheaux P, Gelu S. A randomized, double-blind, controlled study and pooled analysis of two identical trials of fermented milk containing probiotic Bifidobacterium lactis CNCM I-2494 in healthy women reporting minor digestive symptoms. Neurogastroenterol Motil. (2013) 25:331–e252. doi: 10.1111/nmo.12078
110. Agostini S, Goubern M, Tondereau V, Salvador-Cartier C, Bezirard V, Leveque M, et al. A marketed fermented dairy product containing Bifidobacterium lactis CNCM I-2494 suppresses gut hypersensitivity and colonic barrier disruption induced by acute stress in rats. Neurogastroenterol Motil. (2012) 24:376–e172. doi: 10.1111/j.1365-2982.2011.01865.x
111. Tillisch K, Labus J, Kilpatrick L, Jiang Z, Stains J, Ebrat B, et al. Consumption of fermented milk product with probiotic modulates brain activity. Gastroenterology. (2013). 144:1394–401 1401.e1–4. doi: 10.1053/j.gastro.2013.02.043
112. Dronkers TMG, Ouwehand AC, Rijkers GT. Global analysis of clinical trials with probiotics. Heliyon. (2020) 6:e04467. doi: 10.1016/j.heliyon.2020.e04467
113. Eskesen D, Jespersen L, Michelsen B, Whorwell PJ, Muller-Lissner S, Morberg CM. Effect of the probiotic strain Bifidobacterium animalis subsp. lactis, BB-12(R), on defecation frequency in healthy subjects with low defecation frequency and abdominal discomfort: a randomised, double-blind, placebo-controlled, parallel-group trial. Br J Nutr. (2015) 114:1638–46. doi: 10.1017/S0007114515003347
114. Larsen CN, Nielsen S, Kaestel P, Brockmann E, Bennedsen M, Christensen HR, et al. Dose-response study of probiotic bacteria Bifidobacterium animalis subsp lactis BB-12 and Lactobacillus paracasei subsp paracasei CRL-341 in healthy young adults. Eur J Clin Nutr. (2006) 60:1284–93. doi: 10.1038/sj.ejcn.1602450
115. Ba Z, Lee Y, Meng H, Kris-Etherton PM, Rogers CJ, Lewis ZT et al. Matrix effects on the delivery efficacy of Bifidobacterium animalis subsp. lactis BB-12 on fecal microbiota, gut transit time, and short-chain fatty acids in healthy young adults. mSphere. (2021) 6:e0008421. doi: 10.1128/mSphere.00084-21
116. Kristensen NB, Bryrup T, Allin KH, Nielsen T, Hansen TH, Pedersen O. Alterations in fecal microbiota composition by probiotic supplementation in healthy adults: a systematic review of randomized controlled trials. Genome Med. (2016) 8:52. doi: 10.1186/s13073-016-0300-5
117. Wen Y, Li J, Long Q, Yue CC, He B, Tang XG. The efficacy and safety of probiotics for patients with constipation-predominant irritable bowel syndrome: a systematic review and meta-analysis based on seventeen randomized controlled trials. Int J Surg. (2020) 79:111–9. doi: 10.1016/j.ijsu.2020.04.063
118. Chervaux C, Grimaldi C, Bolotin A, Quinquis B, Legrain-Raspaud S, van Hylckama Vlieg JE, et al. Genome sequence of the probiotic strain Bifidobacterium animalis subsp. lactis CNCM I-2494. J Bacteriol. (2011) 193:5560–1. doi: 10.1128/JB.05716-11
119. Kaminski M, Skonieczna-Zydecka K, Loniewski I, Koulaouzidis A, Marlicz W. Are probiotics useful in the treatment of chronic idiopathic constipation in adults? A review of existing systematic reviews, meta-analyses, and recommendations. Prz Gastroenterol. (2020) 15:103–18. doi: 10.5114/pg.2019.86747
120. West CL, Stanisz AM, Mao YK, Champagne-Jorgensen K, Bienenstock J, Kunze WA. Microvesicles from Lactobacillus reuteri (DSM-17938) completely reproduce modulation of gut motility by bacteria in mice. PLoS ONE. (2020) 15:e0225481. doi: 10.1371/journal.pone.0225481
121. Al-Nedawi K, Mian MF, Hossain N, Karimi K, Mao YK, Forsythe P, et al. Gut commensal microvesicles reproduce parent bacterial signals to host immune and enteric nervous systems. FASEB J. (2015) 29:684–95. doi: 10.1096/fj.14-259721
122. Kleerebezem M, Binda S, Bron PA, Gross G, Hill C, van Hylckama Vlieg JE, et al. Understanding mode of action can drive the translational pipeline towards more reliable health benefits for probiotics. Curr Opin Biotechnol. (2019) 56:55–60. doi: 10.1016/j.copbio.2018.09.007
123. Miller LE, Ibarra A, Ouwehand AC. Normative values for colonic transit time and patient assessment of constipation in adults with functional constipation: systematic review with meta-analysis. Clin Med Insights Gastroenterol. (2017) 11:1179552217729343. doi: 10.1177/1179552217729343
Keywords: bowel function, diarrhea, gut motility, Bifidobacterium animalis subsp. lactis HN019, intestinal barrier, probiotic, gut–brain
Citation: Cheng J, Laitila A and Ouwehand AC (2021) Bifidobacterium animalis subsp. lactis HN019 Effects on Gut Health: A Review. Front. Nutr. 8:790561. doi: 10.3389/fnut.2021.790561
Received: 06 October 2021; Accepted: 22 November 2021;
Published: 14 December 2021.
Edited by:
Anand Babu Perumal, Zhejiang University, ChinaReviewed by:
Tomás García-Cayuela, Monterrey Institute of Technology and Higher Education (ITESM), MexicoCarlos Gómez-Gallego, University of Eastern Finland, Finland
Zhihong Sun, Inner Mongolia Agricultural University, China
Copyright © 2021 Cheng, Laitila and Ouwehand. This is an open-access article distributed under the terms of the Creative Commons Attribution License (CC BY). The use, distribution or reproduction in other forums is permitted, provided the original author(s) and the copyright owner(s) are credited and that the original publication in this journal is cited, in accordance with accepted academic practice. No use, distribution or reproduction is permitted which does not comply with these terms.
*Correspondence: Jing Cheng, SmluZy5DaGVuZ0BpZmYuY29t