Deciphering the potential role of Maca compounds prescription influencing gut microbiota in the management of exercise-induced fatigue by integrative genomic analysis
- 1State Key Laboratory of Food Science and Technology, Jiangnan University, Wuxi, Jiangsu, China
- 2Collaborative Innovation Center of Food Safety and Quality Control in Jiangsu Province, Jiangnan University, Wuxi, Jiangsu, China
- 3School of Food Science and Technology, Jiangnan University, Wuxi, Jiangsu, China
- 4Air Force Medical Center, Beijing, China
A growing number of nutraceuticals and cosmeceuticals have been utilized for millennia as anti-fatigue supplements in folk medicine. However, the anti-fatigue mechanism underlying is still far from being clearly explained. The aim of the study is to explore the underlying mechanism of the Maca compound preparation (MCP), a prescription for management of exercise-induced fatigue. In this study, mice weight-loaded swimming test was used to evaluate the anti-fatigue effect of MCP. MCP significantly improved the forelimb grip strength and Rota-rod test in behavioral tests via regulating energy metabolism. 16S rDNA sequencing results showed MCP can regulate the intestinal flora at the genus level by increasing several beneficial bacteria (i.e., Lactobacillus, Akkermansia and etc.), and decreasing the harmful bacteria (i.e., Candidatus_Planktophila and Candidatus_Arthromitus), where notable high relevance was observed between the fatigue-related biomarkers and fecal microbiota. The results of microbial function analysis suggested that MCP might improve exercise-induced fatigue by enhancing energy metabolism, carbohydrate and lipid metabolism and metabolism of terpenoids and polyketides and breakdown of amino acid metabolism. In addition, and H2O2-induced oxidative stress model on C2C12 cells was employed to further validate the regulation of MCP on energy metabolisms. MCP pre-treatment significantly reduced intracellular ROS accumulation, and increased glycogen content, ATP generation capacity and mitochondrial membrane potential of skeletal muscle cells, as well as conferred anti-cell necrosis ability. In conclusion, MCP plays a key role in regulating fatigue occurrence in exercising and gut microbiota balance, which may be of particular importance in the case of manual workers or sub-healthy populations.
Introduction
Fatigue is a systemic process in the decline of human physiology and psychology, and most of the homeopathic supplements are holistic treatments. A growing number of edible natural plants have become used in dietary supplements for relieving fatigue-related symptoms (1, 2), where the compound prescriptions has become one of the most commonly used forms for exercise fatigue (3). Maca compound preparation (MCP) has been administered as an anti-fatigue agent in folk medicine in China for a long time according to The Medical Classic of the Yellow Emperor and Compendium of Materia Medica. In this formula, Maca (Lepidium meyenii Walp.), the monarch drug, is an edible medicine and a new resource food and its anti-fatigue effect has been investigated in our previous study (4). Macamides are the major constituents in Maca and their anti-fatigue activity is mainly credited to their antioxidant and anti-inflammatory properties (5). The eight edible and medicinal plants in MCP (Table 1) have been used to treat fatigue or weakness, which can also inhibit oxidative stress or inflammatory injury induced by exhausting physical exercise.
With the in-depth study of intestinal flora, gut microbiota derived metabolites have been confirmed closely related to the progression of bidirectional communication pathways linking to host (6, 7). Therefore, the overall treatment targeting on both energy metabolism and intestinal bacteria may be a worthwhile therapeutic strategy to fatigue management.
In our previous study, the active components of MCP and anti-fatigue potential have been predicted by network pharmacology exploration (8). However, the effect of MCP on anti-fatigue has not been fully explained and validated, and meanwhile, the association between fatigue-related effects and microbial function has not been established yet. In this study, the weight-loaded forced swimming test (WFST) was performed to verify its anti-fatigue effect, and the underlying mechanisms were systematically explored in exercise-induced fatigue mice. To further explore probable associations between its anti-fatigue effects and gut prebiotic capacity, we tried to measure some preliminary link between physical fatigue and gut microbiota in genus-level. The PICRUSt2 algorithm and MicroCyc databases (9, 10) were employed to investigate the metabolic functions of gut microbes and how MCP regulated gut microbiota and metabolic processes in the fatigue. Based on Kyoto Encyclopedia of Genes and Genomes pathway (KEEG) pathways analysis, metabolism pathways at level 1 are critically involved in host-microbial interactions, where energy metabolisms were significantly promoted by MCP. Finally, a typical H2O2-induced C2C12 cell injury model was applied to mimic skeletal muscle oxidative injury to investigate the protective effects of antioxidant pretreatment and validated the regulations of the intracellular energy metabolisms by MCP in vitro.
Materials and methods
Preparation of Maca compound preparation
Lepidium meyenii Walp. was planted on the Tibet Plateau with an altitude over 3,000 meters. It was harvested in December, and the other 7 plants in MCP were harvested in Shandong Province, China, and were positively identified as the species by Guoliang Ding (Registered Traditional Chinese Medicine Practitioner, R.TCM.P., China).
The main medicinal parts of plants in MCP (Table 1) were cut into thin sections (3–5 mm). After soaking for half an hour, MCP was extracted in boiling water (w/v, 1:8) twice for 1 h at atmospheric pressure. After filtration, the hot water extracts were combined and cooled to room temperature prior to centrifuge at 3,000 rpm for 10 min, further removing the suspended residue in the filtrate. MCP is of high nutritional value, containing 34.78 ± 2.43 mg/ml of total polysaccharides, 0.157 ± 0.018 mg/ml of flavonoids and 1845.27 ± 10.92 mg/ml of total amino acids according to the published paper which used the same protocol (8). The MCP extract was lyophilized at −70°C using a freeze dryer. All lyophilized extracts powder was sealed in sterile sampling bags and stored at −80°C for further experiments.
Anti-fatigue effect of Maca compound preparation in vivo
Experimental animals
All experimental animal procedures were approved by the Ethics Committee of Experimental Animal Center of Jiangnan University (JN.No 20200710i0720915) and the whole project team was strongly committed to comply with the ethical policy. The experiments were performed when Institute of Cancer Research (ICR) mice (18–22 g, male) had adapted to the experimental environment for a week. Animals were randomly divided into 6 different groups (n = 10): control with vehicle treatment (Con); swimming exercise with vehicle treatment (Ex); swimming exercise with MCP (Ex + MCP) or caffeine (purity ≥ 99.8%, Pos, 10 mg/kg bw.) every day for 30 days. The vehicle group received the same volume of sterile water and the 1/10th of the recommended dosage for humans of MCP (12 g/day) received by the mice was based on the “mouse equivalent dose” (8, 18). Thus, MCP was orally administered at 1.0, 2.0, or 4.0 g/kg bw., respectively (the calculated process described in the Appendix), which was reconstituted in sterile water. The low, moderate, and high dose levels of MCP were labeled as MCP-L, MCP-M, MCP-H (Figure 1A).
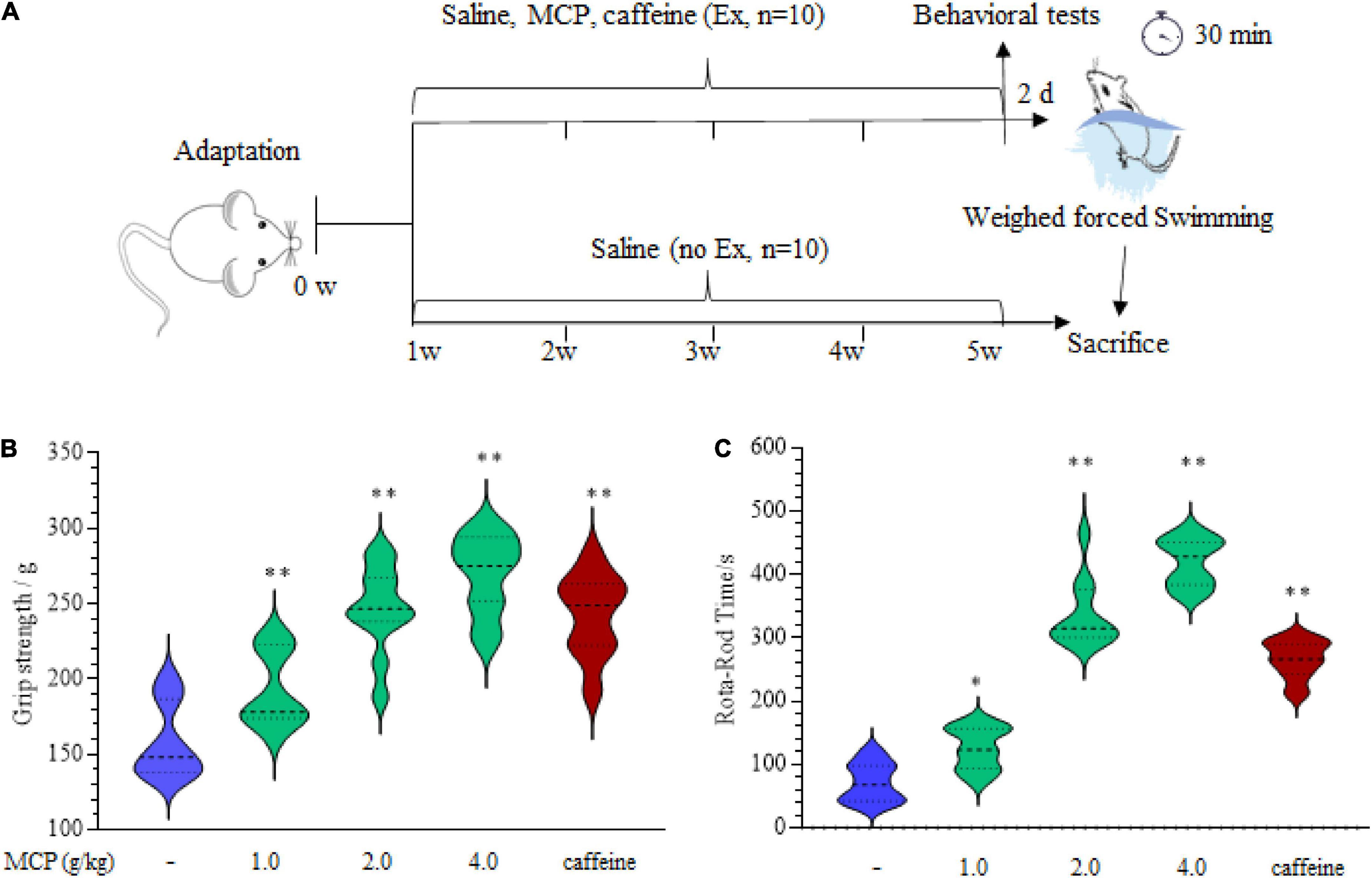
Figure 1. Effects of Maca compound preparation (MCP) on the mice physical fitness measurement tests. (A) Scheme of animal experiments of the weighed-forced swimming test (WFST, 5% bodyweight). (B) The forelimb grip strength of mice examined by grip-strength test. (C) The motor coordination of mice in Rota-rod test. *p < 0.05, **p < 0.01 vs. control group (saline).
Behavioral test
Grip strength test
The forelimb grip strength was assessed by a grip strength meter (YLS-13A Jinan Yiyan Technology Development Co., Ltd., Jinan, China) after oral gavage for 4 weeks. Each mouse was lifted by tail, so its forepaws could grip the wire of the strength meter, and then gently pulled back with tail parallel to table until the mouse lost its grip on the wire. Three tests were performed in succession on each mouse, and scores were averaged for statistical analysis.
Rota-rod test
Mice from each group were placed on an accelerating rota-rod cylinder (ZB-200, Chengdu Taimeng Science Technology Co., Ltd.). The rota-rod was accelerated from 5 to 15 rpm in 5 min. Each mouse remained on revolving rod for the training period. In the formal test, mice were placed on the rota-rod at speed of 15 rpm, until they were exhausted and dropped from the rod. The time of duration was recorded to evaluate motor coordination.
Weight-loaded forced swimming test
Weight-loaded forced swimming test (WFST) was carried out after orally administration on the last day (19, 20). The mice were placed in a tank (height: 65 cm; diameter: 40 cm) of room temperature water (25 ± 2°C) with a depth of 25 cm. The mice were loaded with a lead block (5% of body weight) attached to tails. After the WFST for 30 min, mice were sacrificed for the fatigue-related biochemical indexes measurement.
Biochemical indexes measurement
The blood was detected for blood sugar by Roche blood sugar meter (ACCU-CHEK Performa) and centrifugated at 3,500 g for 10 min at room temperature for measurement of blood lactic acid (BLA), blood urea nitrogen (BUN), lactate dehydrogenase activity (LDH) according to the instructions of the assay kit (Jiancheng Biotechnology Co., Nanjing, China). The left hind leg thigh muscle of mice was collected to measure the levels of reactive oxygen species (ROS), adenosine-triphosphate (ATP), nicotinamide adenine dinucleotide (reduce) [NAD(H)] and glycogen, which were detected (Fankew, Shanghai FANKEL Industrial Co., Ltd., Shanghai, China).
Hematoxylin and eosin staining
For histological examination, the muscle tissue was fixed in 4% (v/v) paraformaldehyde/PBS and embedded in paraffin, then stained the tissue with H&E. Finally, images were acquired from light microscopy (Olympus, Tokyo, Japan) (×200).
16S rRNA gene and bioinformatics analysis
The 5 mice were selected from Ex and MCP-M groups at random, and DNA from feces was extracted by using a Genomic DNA Kit (Omega Bio-tek, Inc., Norcross, GA, USA). The 16S rRNA genes (V3-V4 regions) were amplified from the whole genome via the primer pair (341 F, 5′- CCTAYGGGRBGCASCAG-3′; 806R, 5′-GGACTACHVGGGTWTCTAAT-3′). All the amplicons were purified, quantified, and sequenced on an Illumina novaseq platform (San Diego, CA, USA). The barcode and connector sequence were removed. FLASH (v1.2.8) was used to stitch the double-ended sequences, and Vsearch (v2.3.4) was used to filter out the unqualified sequences (8). Finally, the sequence with 97% similarity was classified as an OTU. 16S rRNA gene reads were down-sampled to a read depth of 44,367 reads/sample and reads mapped to 16S OTUs (1,635 reads), to ensure sample compatibility regardless of sampling depth (21). Gene functions were predicted by PICRUSt.2 and analyzed by KEGG pathway enrichment analysis.1
Effect of Maca compound preparation on cellular metabolism in C2C12 cells
Cell culture and differentiation
The C2C12 (mouse skeletal muscle) cells were purchased from (Hunan Fenghui Biotechnology Co., Ltd., Changsha, China). The cells were maintained in growth medium [Dulbecco’s modified Eagle medium (DMEM) supplemented, 10% (v/v) fetal bovine serum (FBS), 1% (v/v) Penicillin-Streptomycin for cell culture (Gibico)] in a 25 cm2 culture flask. The cells were cultivated at 37°C under a humidified atmosphere of 5% (v/v) CO2. To induce cell differentiation, 70% confluent cultures were switched to DMEM containing 2% horse serum (HS) and 10 μg/ml of insulin for 3 days with medium changes every other day.
H2O2-induced oxidative stress on C2C12 cells
When the C2C12 cells were grown to approximately 70–80% confluence in 96-well flat-bottomed plates (n = 5), they were replenished MCP (re-dissolved in basal medium, free of FBS), and incubated for a further 24 h. According to a previous study (4), cells were pre-treated with 0.48 mmol/L H2O2 for 6 h to inflict oxidative stress before harvest. The cell viability of reagents on C2C12 cells was, respectively, assessed using Cell Counting Kit-8 (CCK-8, Beyotime) assays. After incubating for 1h, the absorbance of each well was measured at 450 nm with a microplate reader (BioTek Instruments, Winooski, VT, USA).
Measurement of reactive oxygen species level in C2C12 cells
The levels of ROS in C2C12 cells were determined using fluorescent probe (DCFH-DA, Beyotime, China). For flow cytometry analysis, C2C12 cells were seeded in 6-well plates at a density of 5 × 105 cells per well (treatment of cells was shown in 2.2.2). Both suspended and adherent cells were collected and washed with PBS twice (for washing out the trypsin). The cells were stained with DCFH-DA and incubated at dark ambient for 20 min. After re-suspending in PBS, they were analyzed by flow cytometry (FACSAriaII, Becton, Dickinson and Company, Franklin Lakes, NJ, USA) according to the manufacturer’s protocol. Analysis of flow cytometry data was performed with FlowJo software (v10.8.1).
Measurement of energy metabolism level in C2C12 cells
To evaluate the effect of the compounds on energy metabolism during muscle growth, the anthrone reagent was employed in the estimation of glycogen content by use of glycogen assay kit (Solarbio Science & Technology Co. Ltd., Beijing, China). C2C12 cells were seeded in 6-wells plates, and 5 million cells would be harvested in 2 days. The glycogen content in cells was measured with an anthrone color reagent from an alkaline digest in concentrated sulfuric acid and calculated in the number of 104 cells.
ATP levels in C2C12 cells were measured by an enhanced ATP assay kit (Beyotime Inc., Shanghai, China) as described in manufacturer’s instructions. Luminescence in the supernatant from each sample was measured in a Synergy Mx multifunctional Microplate Reader (Gene Company Ltd., Hongkong, China). Data were normalized to the control as 100%.
Calcein-AM/PI double staining of C2C12 cells
The Calcein-AM/PI Double Stain Kit (Dojindo Laboratories, Kumamoto, Japan) was used to assess the live/dead staining assay. In brief, the C2C12 cells were seeded onto glass coverslips in 96-well cell culture plates. Cells were treated as shown in 2.2.2. After co-incubation with agents (for 24 h) and H2O2 (for 6 h), cells were washed twice with 1 × Assay Buffer, then loaded with Calcein-AM and Propidium Iodide (PI) at 37°C for 20 min, washed twice in staining buffer, and fluorescence were measured by inverted fluorescence microscope (Carl Zeiss, Jena, Germany). The experiments were performed on C2C12 cells from 3 different visions at random.
Measurement of mitochondrial membrane potential (MMP, ΔΨm)
Changes in ΔΨm of C2C12 cells were measured by using a mitochondrion-specific cationic dye JC-1 according to manufacturer’s instructions (Beyotime) (22). Cells were incubated with JC-1 working solution in the dark for 20 min, which was measured by high-resolution confocal laser microscope (LSM880, Carl Zeiss, Germany). Red-fluorescent emissions were formed by J-aggregates at high membrane potential (excitation 585 nm, emission 590 nm), whereas green-fluorescent monomers existed at low potential (excitation 514 nm, emission 529 nm). Mitochondrial depolarization manifests by a decrease in the ratio of the red and green fluorescence.
Network pharmacology and molecular docking analysis
Swiss Target Prediction2 was used to predict all the targets of candidates, which were imported into Cytoscape 3.8.0 to construct a drug-target network. Molecular docking was carried out online by using SwissDock.3 Crystal structures of the four validated targets in PDB format were downloaded from AlphaFold Protein Structure Database4 and uploaded with candidate ligands in MOL2 format. The lowest Gibbs free energy (△G) and fullfitness of each interaction were calculated in silico.
Data analysis
GraphPad Prism 9.0 was used for analysis. Results were expressed as mean ± standard deviation. One-way ANOVA with Dennett’s multiple comparisons test was used for comparison between the three groups, *p < 0.05, **p < 0.01 were statistically significant among the groups. The correlation among these indicators was conducted by Pearson correlation analysis using R software version 4.1.0.
Results and discussion
Effect of Maca compound preparation on the mice physical fitness
The imbalance of metabolic utilization in peripheral muscle may be both the cause and result of exercise-induced fatigue (23). To validate the effect of MCP on the physical fitness, the grip-strength test and Rota-rod test were carried out after administration of MCP for 4 weeks, as shown in Figure 1A. There is a significant improvement in the forelimb grip strength (Figure 1B), demonstrating MCP enhanced mice muscle strength force production. Meanwhile, the difference seemed to be implicitly stated in Rota-rod exercising (Figure 1C), since motor abilities on accelerating Rota-rod increased significantly in MCP mice. These results revealed improved locomotor capacity in mice treated with MCP. However, to determine whether MCP acted as an antagonist on fatigue/tiredness produced by exercise, we employed the WFST model to investigate the effects of MCP on muscle fatigue status in vivo.
Effect of Maca compound preparation on mice energetic metabolism
To evaluate the energy metabolisms during fatigue, the contents of blood sugar, BLA, BUN, LDH in serum and NAD(H) in muscle were investigated in exercise mice. The occurrence of fatigue is accelerated by the decrease of blood sugar and accumulation of metabolites such as BLA, BUN and LDH, however, this situation is effectively reversed by the treatment of MCP as shown in Figures 2A–D. In addition to these metabolites, NAD(H) plays key role in the process of glycolysis process and cellular respiration, which is also an integral determining factor in fatigue (24). The levels of NAD(H) were decreased by approximately half in mice muscle after swimming, which were significantly increased by MCP-treatment compared with those of Ex group (Figures 2E,F). MCP increased energy materials in muscle, which could improve athletic capacity and enhance exercising endurance, in line with the observed results of exercise performance.
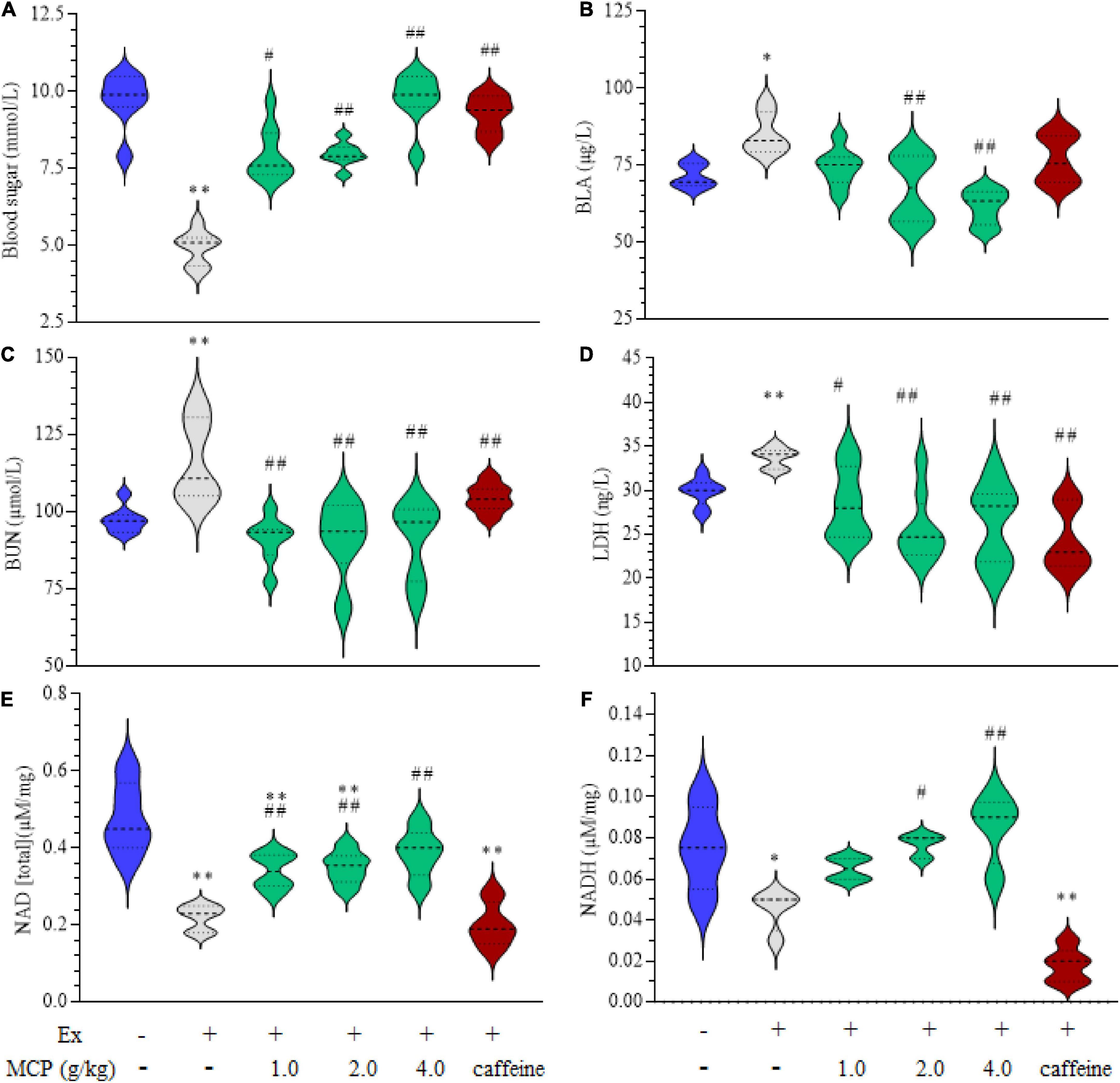
Figure 2. Effects of Maca compound preparation (MCP) on energetic metabolism of exercise mice. (A) Blood sugar. (B) Blood lactic acid (BLA). (C) Blood urea nitrogen (BUN). (D) Lactate dehydrogenase activity (LDH) in the serum. (E,F) Nicotinamide adenine dinucleotide (reduced) [NAD(H)] in the muscle. *p < 0.05, **p < 0.01 vs control (Con); #p < 0.05, ##p < 0.01 vs exercise (Ex) group.
Effect of Maca compound preparation on mice exercise-induced oxidative stress and muscle injuries
The above results of changes in plasma and muscle metabolites suggested abnormal energy metabolism of exercise mice during fatigue loading. To further explore the role of MCP in resistance to acute exercise-induced peripheral fatigue, we focused on the site of muscle in vivo. In WFST model, the ROS levels in the left hind thigh mice muscle were significantly down-regulated by MCP supplementation compared to the Ex group (p < 0.05) in Figure 3A, which is parallel to the outcomes of the in vitro tests. In addition, MCP concentration-dependently enhanced the muscle glycogen contents (Figure 3B) and ATP level (Figure 3C), promoting energy metabolism in muscle during swimming. To intuitively observe the protective effect of MCP, H&E staining were carried out on the hind leg thigh muscle (Figure 3D). Representative images showing H&E staining on mice muscle. Like the in vitro studies, the intense exercise led to muscle cells swelling, necrosis or degradation with abundant cracks. Pre-treatment of MCP at moderate or high dose exhibited pronounced cytoprotective effects, and protected muscle issues from disorganization and loss of tissue structure. Based on effects of the mice physical fitness, an obvious enhancement was observed on MCP at moderate dose compared with the low dose, which was larger than that between the moderate and high doses. Thus, the moderate dose (2.0 g/kg), which was equivalent to the recommended dose in humans, was selected for subsequent experiments.
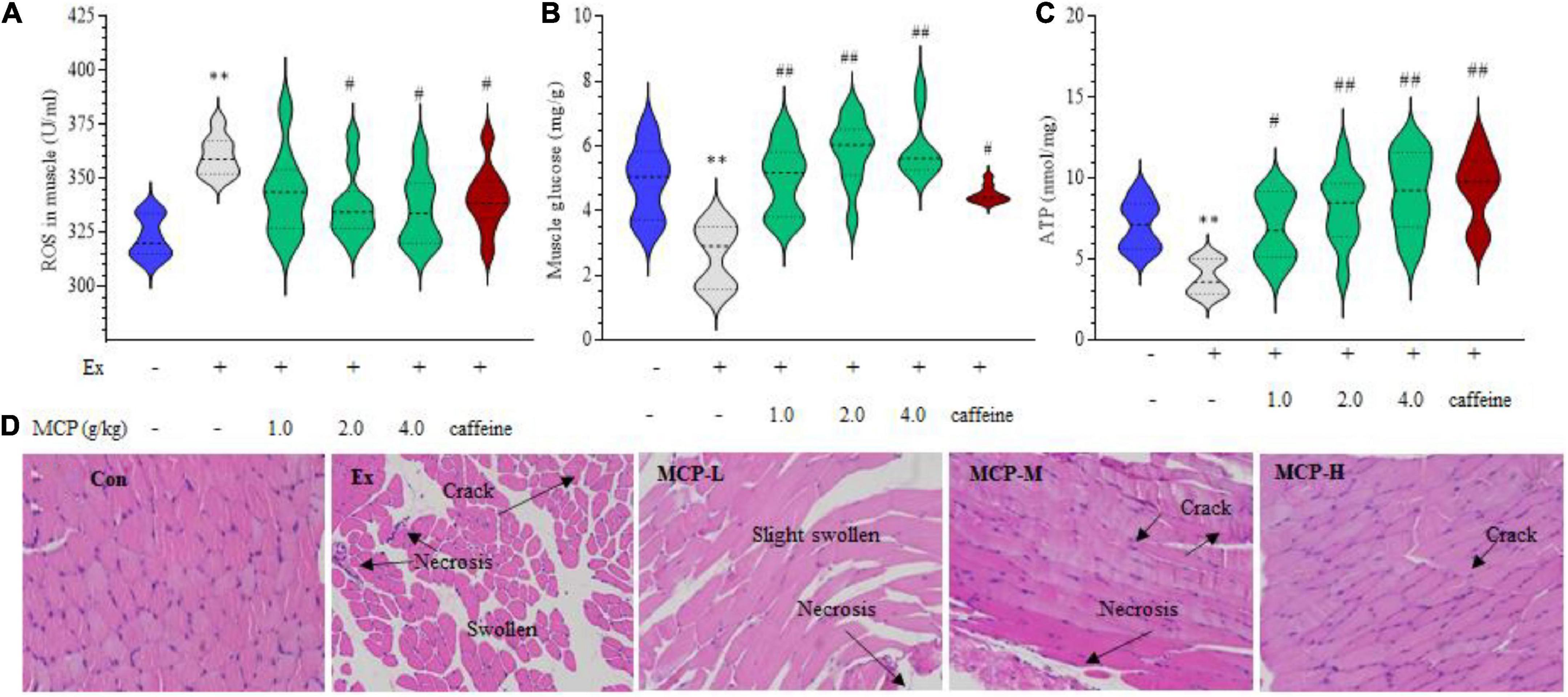
Figure 3. Maca compound preparation (MCP) protect muscle damage from oxidative stress in exercising mice. (A) Reactive oxygen species (ROS) accumulation. (B) Glycogen contents. (C) Adenosine triphosphate (ATP) generation capacity. The content of ATP was calculated as a value relative to that in Con group, which was set as 100%. **p < 0.01 vs control (Con) group; #p < 0.05, ##p < 0.01 vs exercise (Ex) group. (D) Hematoxylin and Eosin (H&E) stain of skeletal muscle. MCP-L, MCP-M, MCP-H represent MCP of low, moderate, and high dose levels.
Effect of Maca compound preparation on mice gut microbiota and correlation to the biochemical parameters
According to the result of 16s RNA, the relative genus-level gut flora abundance was significantly different between Ex and Ex + MCP groups (Supplementary Table 1). MCP can improve resistance to fatigue by regulating the intestinal flora by increasing several primary beneficial bacteria (i.e., Lactobacillus, Akkermansia and etc.), and decreasing harmful bacteria (i.e., Candidatus_Planktophila and Candidatus_Arthromitus; Figure 4A). Notably, the relative genus-level gut flora abundance of Lactobacillus is increased over 2.5 folds (51.4 vs 19.8%, MCP vs Ex group), and that of Candidatus_Planktophila is decreased about 16 folds (2.2 vs 36.9%). Fatigue-related biochemical changes, as well as gut microbiota changes have been found their contributions to exercise mice performance (19, 25). In particular, fatigue generation is proved as one of the consequences of gastrointestinal imbalance, which may be associated with host metabolism and intestinal microecology (26). To further evaluate the correlations among oxidative stress and energy metabolism in serum and muscle, Pearson correlation is performed to analyze the correlations (Figure 4B). According to Pearson correlation analysis, high relevance was observed between fatigue-related biomarkers (BLD, BUN, LDH and ROS) and energy-supplying substances (blood sugar, MG and ATP), which were strongly correlated to fecal microbiota at the genus level as well (Figure 4C). Accumulating convincing evidence has shown that the gut flora plays key role in resistance to fatigue in clinic (25). According to matrix plots, the exercise performance is positively related to the relative abundance of Lactobacillus, blautia, Clostridia_UCG-014 ant etc. (r ≥ 0.5, p < 0.01), whereas negatively related to that of harmful bacteria (i.e., Candidatus_Planktophila and Candidatus_Arthromitus) (r ≤ −0.5, p < 0.01). The absolute values of Pearson correlation lower than 0.5 were hidden, which were accumulated and displayed in two-side. According to the cumulative value in Figure 4C, BLD is one of the most relative biomarkers indicating exercise-induced fatigue, indicating that BLD might play a key role in exercise physiological, performance, and energy utilization via microbe-host interactions (27). Meanwhile, Lactobacillus and Candidatus_Planktophila may be the most relative beneficial/harmful bacteria.
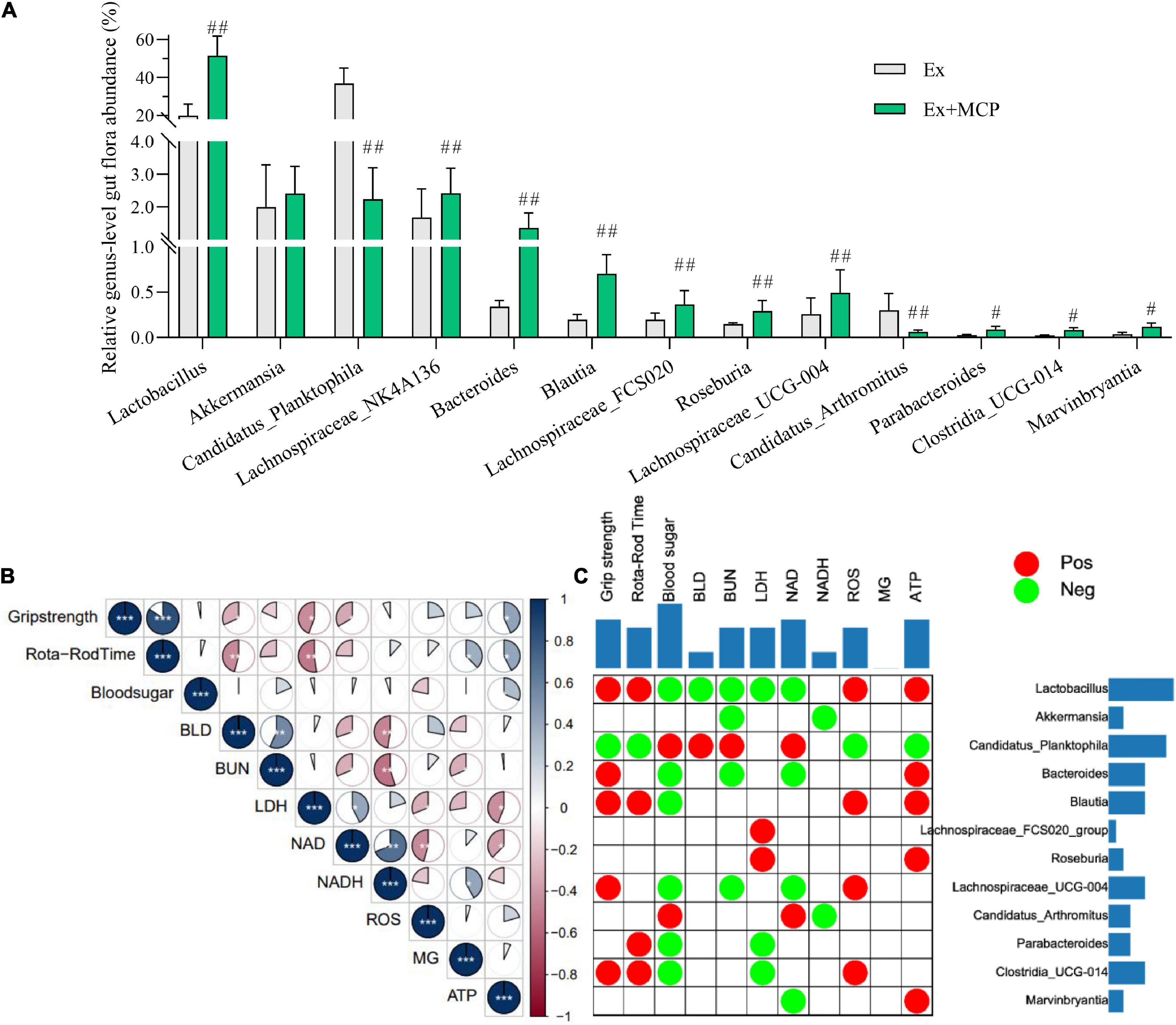
Figure 4. Mice gut microbiota and their correlation to the fatigue-related biochemical parameters of exercising mice. (A) Relative genus-level gut flora abundance; (B) Pearson correlation analysis among fatigue-related indicators; (C) Pearson correlation analysis between fatigue-related indicators and relative genus-level gut flora abundance (red plots represent r ≥ 0.5, and green represent r ≤ −0.5; the two-side blue bar represents the number of positive/negative plots in each row or column, reflecting the importance of the gut microbiota (row) or biochemical parameter (column) in the correlation grid). #p < 0.05, ##p < 0.01 vs exercise (Ex) group.
Effect of Maca compound preparation on mice gut microbial functions
The host-microbial and microbe-microbial interactions are often governed by the complex exchange of metabolites, which are highly associated with the metabolic capacity of hosts in health and disease (28). In Figure 5A, networks of microbe-microbe interactions revealed the regulation of MCP among microbiomes (p < 0.01), where Lactobacillus suggested that it might occupy the dominant position due to the biggest circle size. To further investigate how MCP regulates gut microbiota and metabolic processes in the fatigue, the PICRUSt2 algorithm and MicroCyc databases were employed to analyze the metabolic functions of gut microbes. By comparing with the microbial genome sequences in the database, the key pathways (L1) involved in the breakdown of MCP by microbes were most related to metabolic pathways, and significantly distinguished from Ex in the environmental information processing, human diseases pathways and metabolism (Figure 5B). In addition, the gut microbial functions were analyzed and principal component (PCA) analysis revealed striking differences between the two groups based on KEGG orthology (L2; Figure 5C). Compared with the Ex group, MCP significantly regulated 19 pathways at levels 2 (Figure 5D) and the changes in the mice individual level were shown in Figure 5E. MCP could enhance the cellular processes of cell motility. Six human diseases were downregulated by MCP, involving in substance dependence, infectious disease, immune disease, drug resistance, cardiovascular disease and cancer. Additionally, treatment with MCP significantly reduced amino acid metabolism, and global and overview maps, while MCP significantly enhanced energy metabolism, carbohydrate, lipid metabolism, metabolism of terpenoids and polyketides compared with the Ex group. MCP might be critically involved in tissue development and tissue homeostasis in aging, circulatory system, digestive system, excretory system, immune system, and nervous system. Furthermore, MCP significantly downregulated the pathways of human cytomegalovirus infection, atrazine degradation, renin angiotensin system and etc. at level 3 (Supplementary Figure 1). Thus, the nutritional modulation of gut microbiota by MCP and its interplay between intestinal microbiota and host metabolism may provide a promising insight into fatigue process and a promising avenue for some metabolic dysfunctions (29).
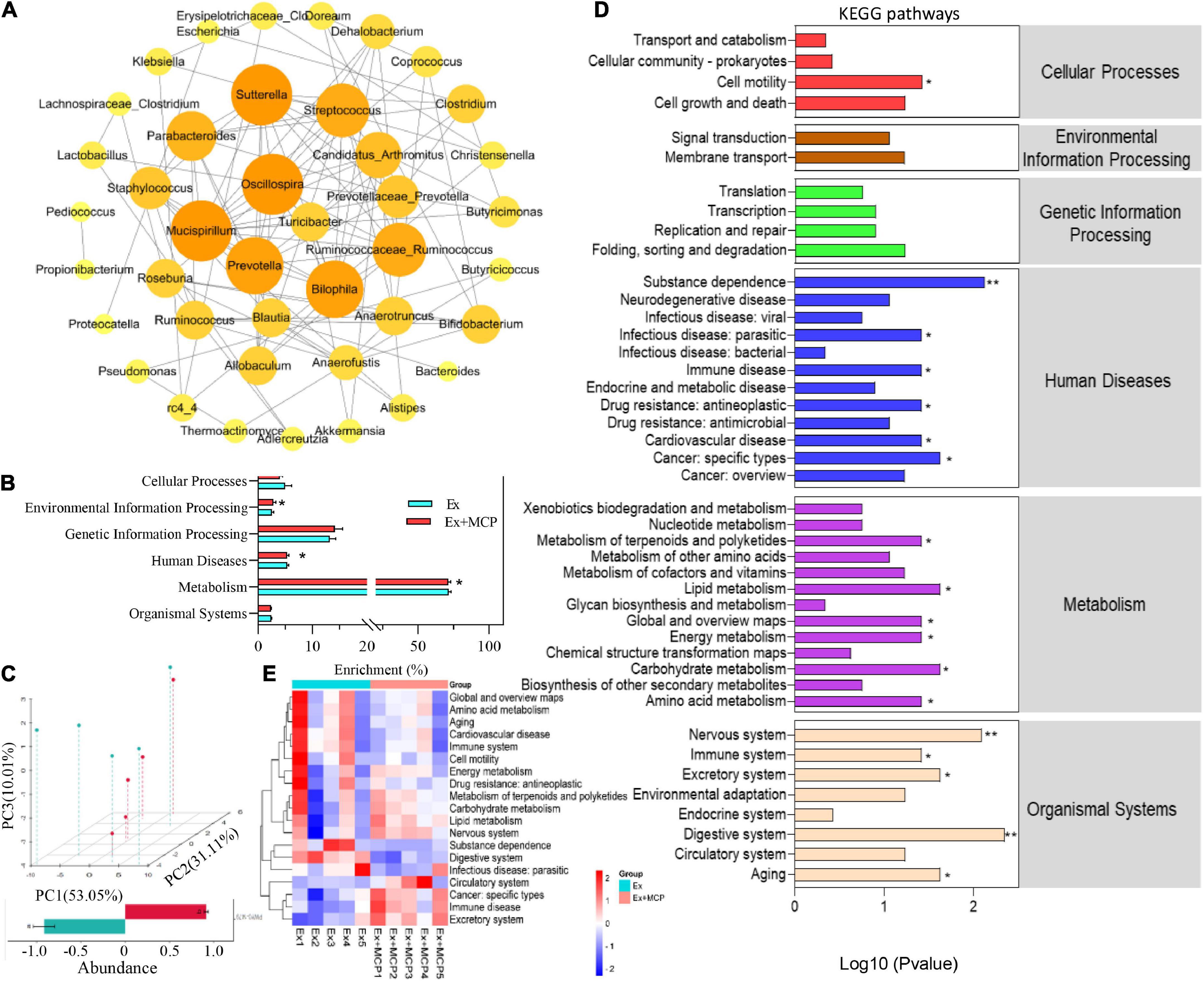
Figure 5. Effect of Maca compound preparation (MCP) on the mice gut microbial functions. (A) Correlation networks of microbe-microbe interactions regulated by MCP. Here, the lines between the circles represents a significant correlation (p-value < 0.05), the red represents a positive correlation, and blue represents a negative correlation. (B) Kyoto Encyclopedia of Genes and Genomes pathway (KEGG) of microbial (level 1). (C) Principal component (PCA) analysis of microbial genus levels and functional differences between Ex and Ex + MCP groups; (D) Heatmap of KEGG (level 2) pathways (adj. p-value < 0.05). (E) KEGG (level 2) pathways of the mice gut microbial gene functions related to cellular processes, environmental information processing, genetic information processing, human diseases, metabolism, and organismal systems. **p < 0.01.
Effect of Maca compound preparation on oxidative stress and energy metabolism in vitro
Based on the above results, in vitro experiment was carried out to evaluate energy metabolism regulation of MCP in fatigue. According to the free radical theory, the accumulation of oxidative damage is a causal factor for muscle fatigue (30). Thus, H2O2 exposure was widely used for inducing C2C12 cell injuries to mimic oxidative damage in muscle fatigue (31, 32). Commonly, cell viability is a comprehensive index used to evaluate proliferation and cell death, indicating the potential of MCP to resist fatigue. In contrast, MCP (0.1–1.0 mg/ml) showed a marked potential for protecting skeletal muscle cells from oxidative stress injuries (Figure 6A). Oxidative stress and energy metabolism play indispensable roles in the in-tissue homeostasis and fatigue-related symptoms. The fluorescence intensity represented the amount of intracellular ROS production in C2C12 cells, which were detected by using DCFH-DA as fluorescent probe and analyzed by flow cytometry. H2O2 treatment increased ROS levels in cells, whereas MCP pre-incubation reduced H2O2-induced ROS generation (p < 0.01), suggesting that resistance to H2O2 injury was improved by MCP (Figure 6B). meanwhile, MCP concentration-dependently enhanced the intracellular glycogen and ATP contents, promoting energy metabolism in C2C12 cells. Under oxidative stress damage, the glycogen contents in Mod decreased significantly (**p < 0.01) by nearly 30%. The MCP groups showed higher contents of C2C12 glycogen and ATP levels than those of Mod groups, indicating its anti-fatigue potential on H2O2 mediated-oxidative stress on cells. Moreover, it was worth noting that MCP concentration-dependently enhanced the glycogen content (R2 = 0.9983), and ATP generation ability (R2 = 0.9917) in vitro (Figures 6C,D). MCP significantly conferred anti-cell necrosis ability and attenuated exercise-induced damage, as evidenced by an increase of Live/Dead assay and skeletal muscle morphology (Figure 6E). Compared with Con, the H2O2 shrunk the cells, deformed their shapes, and generated large quantities of cell debris, while MCP significantly alleviated H2O2-induced oxidative damage both in viability or cell morphology. As shown in Figure 6F, H2O2 injury impaired ΔΨm, while MCP treatment improved the stability of ΔΨm and morphology, indicating protective effects on mitochondrial functions. Thus, the in vitro experiments further demonstrated a substantial effect of MCP on mitochondrial function and energy metabolism.
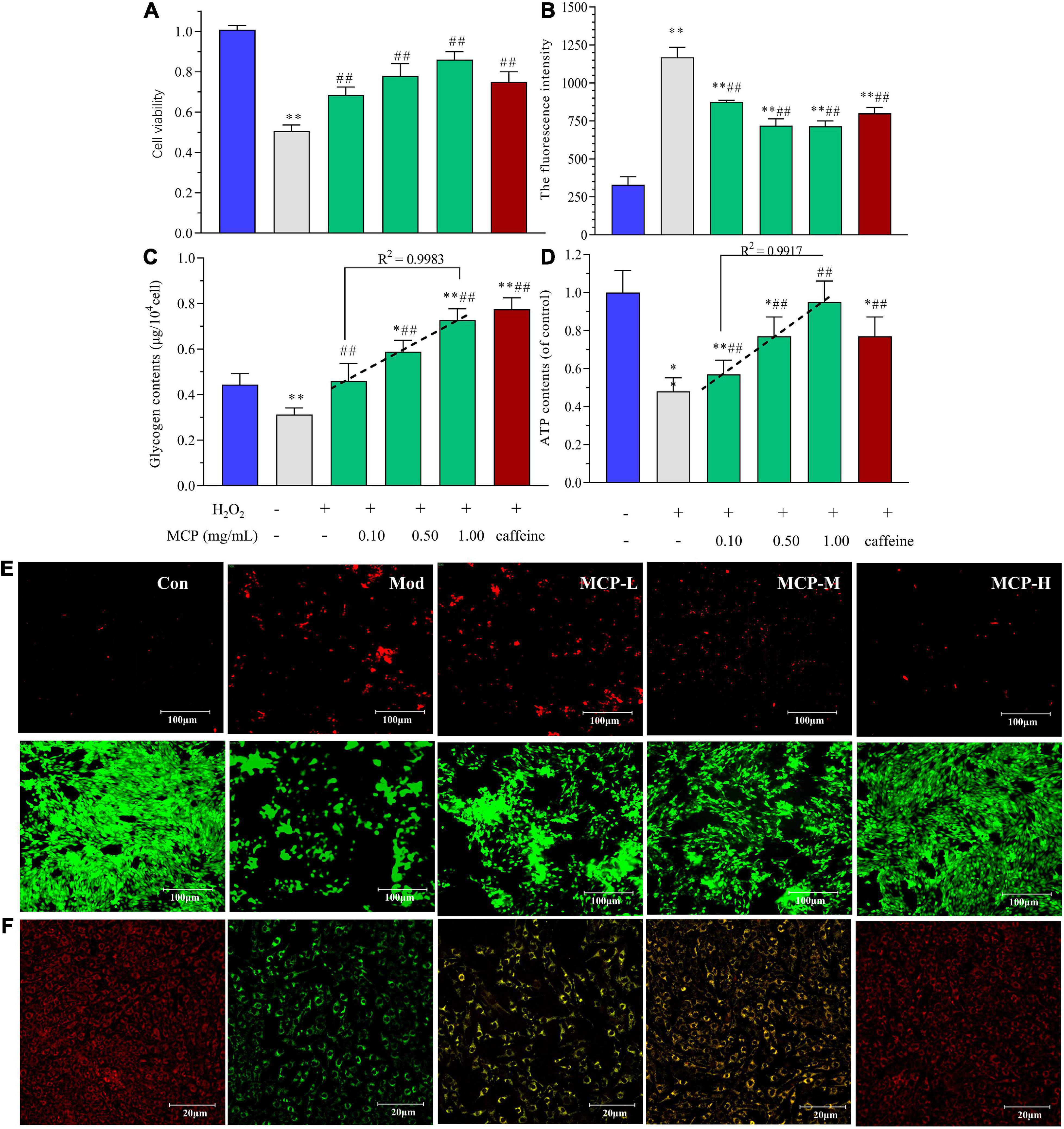
Figure 6. Effects of Maca compound preparation (MCP) on H2O2-induced C2C12 cells. (A) Cell viability of MCP on oxidative stress-induced C2C12 cells. (B) ROS accumulation analyzed by flow cytometry. (C) Glycogen contents. (D) ATP generation capacity. The content of ATP was calculated as a value relative to that in con group, which was set as 100%. *p < 0.05, **p < 0.01 vs control (Con); #p < 0.05, ##p < 0.01 vs model (Mod). (E) The Live (green)/Dead (red) assay of C2C12 treated with H2O2-induced oxidative stress. (F) Mitochondrial membrane potential and morphology. MCP-L, MCP-M, MCP-H represent MCP of low, moderate, and high dose levels.
Exploration the potential metabolic pathways of Maca compound preparation
Mitochondrial respiratory metabolism is the major source of cellular glycogen and ATP, and is associated with the proper maintenance of cellular metabolism as a whole (33). To further explore the key metabolic pathways of MCP, a joint pathway analysis was performed based on network pharmacology and metabolic pathway analyses on MetaboAnalyst platform. Eight anti-fatigue intestine-specific expressed targets (ABCG2, PDE9A, SLC6A4, CHRNA7, HNF4A and MAOA) of MCP and six metabolites (BLA, blood sugar, glycogen, ATP, NAD and NADH) were plugged into MetScape to build compound-reaction-enzyme-gene networks by matching with the potential targets obtained from MetScape (Figure 7A). The intestine-specific expression of phosphodiesterase 9A (PDE9A) was identified as the key target. Therefore, we believe that PDE9A may have a crucial effect on the efficacy of MCP for fatigue.
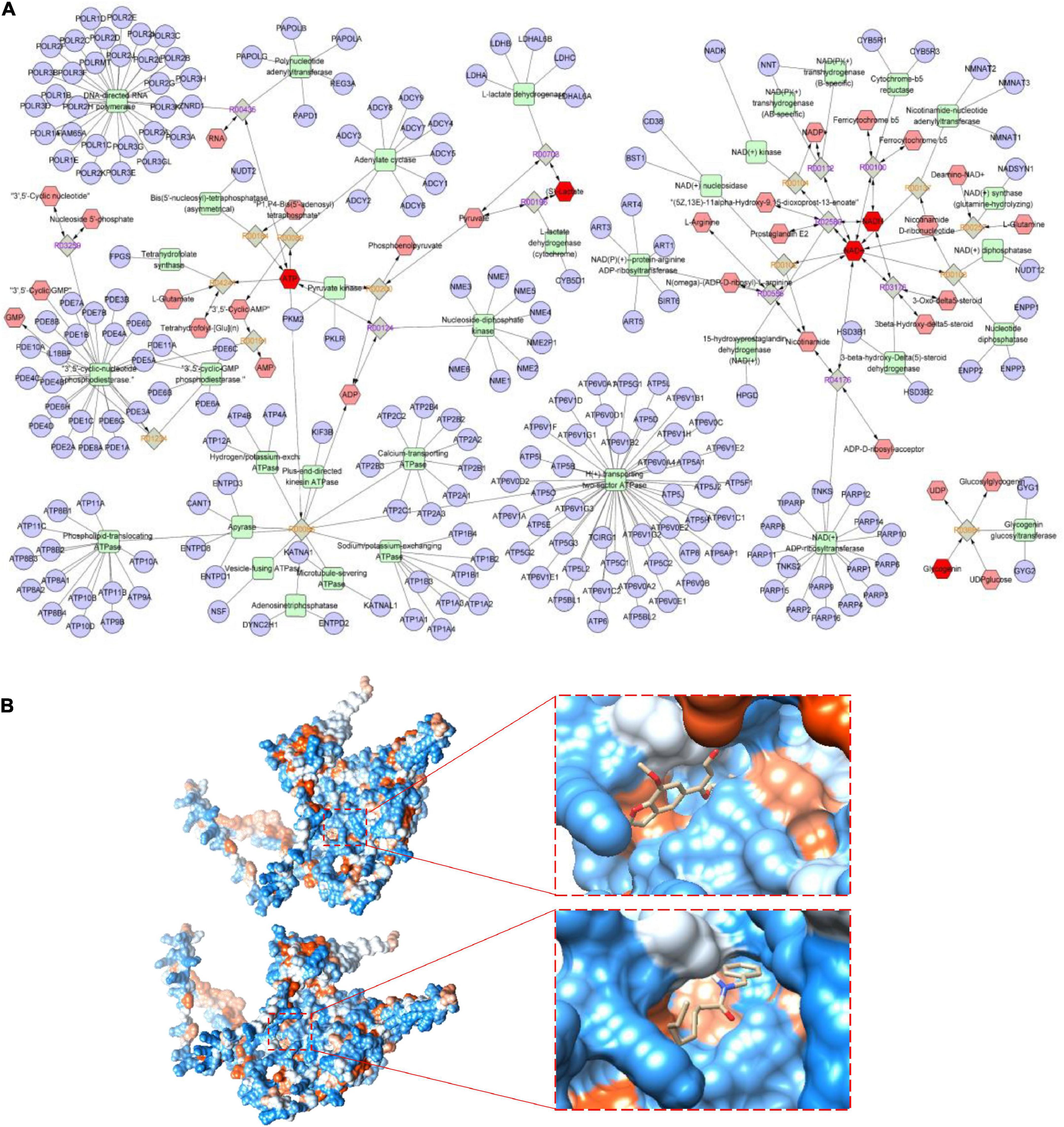
Figure 7. Metabolic pathways and molecular docking. (A) Potential metabolic network regulated by Maca compound preparation (MCP) in exercising mice. The purple circular nodes represent genes, the green square nodes represent metabolism-related enzymes, and the red hexagon nodes represent fatigue-related metabolites, involving blood lactic acid (BLA), blood sugar, glycogen, adenosine triphosphate (ATP), and nicotinamide adenine dinucleotide (reduce) [NAD(H)] determined in exercising mice. (B) Molecular docking of cnidilin (△G = −6.36 kcal/mol) and obacunone (△G = −6.11 kcal/mol) to the key protein of the intestine-specific expression of phosphodiesterase 9A (PDE9A).
Several bioactive substances might play critical roles in MCP. Based on previous network pharmacology analysis (8), N-benzyl-octanamide (from Maca), vitamin E (from Amomum villosum Lour), cnidilin and phyllanthin (from Angelica sinensis), obacunone (from Citrus reticulata Blanco), are the active ingredients targeted on PDE9A. Figure 7B showed the visualization of the most energetically favorable binding of the six ligands into the key protein of PDE9A. As the most favorable interaction, cnidilin and obacunone showed the lowest Gibbs free energy (△G = −6.36 and −6.11 kcal/mol) and for PDE9A, suggesting that suggesting that dietary influences by MCP are most probably mediated in part by the gut microbes.
Discussion
Fatigue were the most commonly reported systemic reactions, suggesting a link existed among fatigue-related parameters from central to peripheral (19). Intestinal dysbiosis can dysregulate inflammation of intestinal surrounding tissues as well as cognition and mood (34). In the formula, Maca could improve male sexual behavior so that it has been known as “South American ginseng” or “Plant Viagra” worldwide (5). Its extract could increase glucose uptake by inhibiting mitochondrial function (35). Natural herbal medicine with compatibility and multiple targets, is widely regarded as a new way for drugs discovery for anti-fatigue. It points out directions for future studies on the internationalization of Traditional Chinese Medicine (TCM) (36). Under complementarity principle, the prescription was expected to exert anti-fatigue activities on multiple targets with the compatibility detoxication-based TCM theory. Meanwhile, the combined extracts produced better synergistic effects than a single drug (37). Compared with conventional single-target drugs, TCM prescriptions usually contain several medicinal herbs, along with synergistic effects (multiple compounds – multiple targets – one pathway) (38, 39).
Increasing numbers of evidence suggested that gut microbiota-targeted therapy is a promising strategy to treat the chronic or metabolic disorders, which may behave as one of the mechanism for altering the pathogenesis TCM herbals (40). Currently, accumulated results have showed that Lactobacillus could significantly elevated the exercise performance in a dose-dependent manner and improved the fatigue-associated features correlated with better physiological adaptation (41). A recent study has revealed the impacts of gut microbiome on the host’s health, where Lactobacillus primarily modulated the overall microbial community structure (42). On the other hand, Candidatus Planktophila is an actinobacterium, which might represent pathogenic bacteria from freshwater bacterioplankton (43). Candidatus_Arthromitus was proved associated with depression (44). PDE9A is an intracellular cyclic guanosine monophosphate (cGMP) hydrolase, which has been exploited as one of the most promising therapeutics for treatment of metabolic diseases, such as diabetes and Alzheimer’s (45), as well as fatigue syndromes (46). In this study, the link between fatigue-related parameters and gut microbiota have been established by treatment of MCP, however, the underlying mechanisms should be further explored – the gut microbiota and the host interplay. Thus, further studies on host-microbe-drug-nutrient may probably need to answer the fundamental question of how MCP maintains intestinal microecological balance and delays generation of host fatigue (47).
In conclusion, it was demonstrated a medicinal and edible decoction – MCP, as a promising candidate to manage exercise-induced fatigue, which may be of particular importance in the case of manual workers or sub-healthy populations.
Data availability statement
The datasets presented in this study can be found in online repositories. The names of the repository/repositories and number(s) can be found below: NCBI [BioProject: PRJNA888212 for 16S rRNA sequencing].
Ethics statement
The animal study was reviewed and approved by the Ethics Committee of Experimental Animal Center of Jiangnan University (JN.No 20200710i0720915).
Author contributions
HZ: conceptualization and writing – original draft preparation. RW and HH: validation and formal analysis. HQ and PD: conceptualization. All authors contributed to the article and approved the submitted version.
Funding
This work was financially supported by the Youth Cultivation Program (21QNPY095).
Acknowledgments
We sincerely thank Guoliang Ding (Registered Traditional Chinese Medicine Practitioner, R.TCM.P., China) for the guidance of the theory of TCM.
Conflict of interest
The authors declare that the research was conducted in the absence of any commercial or financial relationships that could be construed as a potential conflict of interest.
Publisher’s note
All claims expressed in this article are solely those of the authors and do not necessarily represent those of their affiliated organizations, or those of the publisher, the editors and the reviewers. Any product that may be evaluated in this article, or claim that may be made by its manufacturer, is not guaranteed or endorsed by the publisher.
Supplementary material
The Supplementary Material for this article can be found online at: https://www.frontiersin.org/articles/10.3389/fnut.2022.1004174/full#supplementary-material
Footnotes
- ^ http://huttenhower.sph.harvard.edu/galaxy/
- ^ http://swisstargetprediction.ch/index.php
- ^ http://swissdock.ch/
- ^ https://alphafold.ebi.ac.uk/
References
1. Song J-H, Won S-K, Eom G-H, Lee D-S, Park B-J, Lee J-S, et al. Improvement effects of myelophil on symptoms of chronic fatigue syndrome in a reserpine-induced mouse model. Int J Mol Sci. (2021) 22:10199. doi: 10.3390/ijms221910199
2. Jin HM, Wei P. Anti-fatigue properties of Tartary buckwheat extracts in mice. Int J Mol Sci. (2011) 12:4770–80. doi: 10.3390/ijms12084770
3. Zhang JT, Zhang C, Liu AF, Ji Q, Ren LX, Ma CR, et al. Synthesis of icariin-Zinc and its protective effect on exercise fatigue and reproductive system related glands in male rats. Front Pharmacol. (2021) 12:611722. doi: 10.3389/fphar.2021.611722
4. Zhu H, Xu W, Wang N, Jiang W, Cheng Y, Guo Y, et al. Anti-fatigue effect of Lepidium meyenii Walp. (Maca) on preventing mitochondria-mediated muscle damage and oxidative stress in vivo and vitro. Food Funct. (2021) 12:3132–41. doi: 10.1039/d1fo00383f
5. Zhu HK, Hu B, Hua HY, Liu C, Cheng YL, Guo YH, et al. Macamides: a review of structures, isolation, therapeutics and prospects. Food Res Int. (2020) 138:11. doi: 10.1016/j.foodres.2020.109819
6. Wang LA, Gao MX, Kang GB, Huang H. The potential role of phytonutrients flavonoids influencing gut microbiota in the prophylaxis and treatment of inflammatory bowel disease. Front Nutr. (2021) 8:798038. doi: 10.3389/fnut.2021.798038
7. Li P, Li M, Song Y, Huang XC, Wu T, Xu ZZ, et al. Green banana flour contributes to gut microbiota recovery and improves colonic barrier integrity in mice following antibiotic perturbation. Front Nutr. (2022) 9:832848. doi: 10.3389/fnut.2022.832848
8. Zhu HK, Wang RY, Hua HY, Cheng YL, Guo YH, Qian H, et al. Network Pharmacology exploration reveals gut microbiota modulation as a common therapeutic mechanism for anti-fatigue effect treated with Maca compounds prescription. Nutrients. (2022) 14:1533. doi: 10.3390/nu14081533
9. Liu C, Hua H, Zhu H, Xu W, Guo Y, Yao W, et al. Study of the anti-fatigue properties of macamide, a key component in Maca water extract, through foodomics and gut microbial genomics. Food Biosci. (2022) 49:101876. doi: 10.1016/j.fbio.2022.101876
10. Le Boulch M, Dehais P, Combes S, Pascal G. The MACADAM database: a metabolic pathways database for microbial taxonomic groups for mining potential metabolic capacities of archaeal and bacterial taxonomic groups. Database. (2019) 2019:baz049. doi: 10.1093/database/baz049
11. Cui X, Wang S, Cao H, Guo H, Li Y, Xu F, et al. A review: the bioactivities and pharmacological applications of Polygonatum sibiricum polysaccharides. Molecules. (2018) 23:1170. doi: 10.3390/molecules23051170
12. Chen Z, Liu L, Gao C, Chen W, Vong CT, Yao P, et al. Astragali radix (Huangqi): a promising edible immunomodulatory herbal medicine. J Ethnopharmacol. (2020) 258:112895. doi: 10.1016/j.jep.2020.112895
13. Sadaf S, Bhatti HN, Iqbal Z, Shahid M. Chemical composition, antioxidant and antimicrobial activities of Citrus jambhiri Lush and Citrus reticulata Blanco essential oils. J Chem Soc Pak. (2009) 31:838–44.
14. Xie Q, Sun Y, Cao L, Chen L, Chen J, Cheng X, et al. Antifatigue and antihypoxia activities of oligosaccharides and polysaccharides from Codonopsis pilosulain mice. Food Funct. (2020) 11:6352–62. doi: 10.1039/d0fo00468e
15. Zhao P, Zhao C, Li X, Gao Q, Huang L, Xiao P, et al. The genus polygonatum: a review of ethnopharmacology, phytochemistry and pharmacology. J Ethnopharmacol. (2018) 214:274–91. doi: 10.1016/j.jep.2017.12.006
16. Ye X, Pi X, Zheng W, Cen Y, Ni J, Xu L, et al. The methanol extract of Polygonatum odoratum ameliorates colitis by improving intestinal short-chain fatty acids and gas production to regulate microbiota dysbiosis in Mice. Front Nutr. (2022) 9:899421. doi: 10.3389/fnut.2022.899421
17. Chen Z, Ni W, Yang C, Zhang T, Lu S, Zhao R, et al. Therapeutic effect of Amomum villosum on inflammatory bowel disease in rats. Front Pharmacol. (2018) 9:639. doi: 10.3389/fphar.2018.00639
18. Sharma V, McNeill JH. To scale or not to scale: the principles of dose extrapolation. Br J Pharmacol. (2009) 157:907–21. doi: 10.1111/j.1476-5381.2009.00267.x
19. Zhu H, Wang R, Hua H, Cheng Y, Guo Y, Qian H, et al. The macamide relieves fatigue by acting as inhibitor of inflammatory response in exercising mice: from central to peripheral. Eur J Pharmacol. (2022) 917:174758. doi: 10.1016/j.ejphar.2024.174758
20. Sunil C, Zheng X, Yang Z, Cui K, Su Y, Xu B. Antifatigue effects of Hechong (Tylorrhynchus heterochaetus) through modulation of Nrf2/ARE- mediated antioxidant signaling pathway. Food Chem Toxicol. (2021) 157:112589. doi: 10.1016/j.fct.2021.112589
21. Maifeld A, Bartolomaeus H, Löber U, Avery EG, Steckhan N, Markó L, et al. Fasting alters the gut microbiome reducing blood pressure and body weight in metabolic syndrome patients. Nat Commun. (2021) 12:1970. doi: 10.1038/s41467-021-22097-0
22. Shailaja VL, Christina VS, Mohanapriya CD, Sneha P, Sundaram RL, Magesh R, et al. A natural anticancer pigment, pheophytin a, from a seagrass acts as a high affinity human mitochondrial translocator protein (TSPO) ligand, in silico, to reduce mitochondrial membrane potential (Delta psi(mit)) in adenocarcinomic A549 cells. Phytomedicine. (2019) 61:152858. doi: 10.1016/j.phymed.2019.152858
23. Wan J-J, Qin Z, Wang P-Y, Sun Y, Liu X. Muscle fatigue: general understanding and treatment. Exp Mol Med. (2017) 49:e384.
24. Mach J, Midgley AW, Dank S, Grant RS, Bentley DJ. The effect of antioxidant supplementation on fatigue during exercise: potential role for NAD(+)(H). Nutrients. (2010) 2:319–29. doi: 10.3390/nu2030319
25. Buigues C, Fernandez-Garrido J, Pruimboom L, Hoogland AJ, Navarro-Martinez R, Martinez-Martinez M, et al. Effect of a prebiotic formulation on frailty syndrome: a randomized, double-blind clinical trial. Int J Mol Sci. (2016) 17:932. doi: 10.3390/ijms17060932
26. Zhou SS, Zhou J, Xu JD, Shen H, Kong M, Yip KM, et al. Ginseng ameliorates exercise-induced fatigue potentially by regulating the gut microbiota. Food Funct. (2021) 12:3954–64. doi: 10.1039/d0fo03384g
27. Huang W-C, Chen Y-H, Chuang H-L, Chiu C-C, Huang C-C. Investigation of the effects of microbiota on exercise physiological adaption, performance, and energy utilization using a gnotobiotic animal model. Front Microbiol. (2019) 10:1906. doi: 10.3389/fmicb.2019.01906
28. Shock T, Badang L, Ferguson B, Martinez-Guryn K. The interplay between diet, gut microbes, and host epigenetics in health and disease. J Nutr Biochem. (2021) 95:108631. doi: 10.1016/j.jnutbio.2021.108631
29. Ojeda P, Bobe A, Dolan K, Leone V, Martinez K. Nutritional modulation of gut microbiota – the impact on metabolic disease pathophysiology. J Nutr Biochem. (2016) 28:191–200. doi: 10.1016/j.jnutbio.2015.08.013
30. Kerasioti E, Stagos D, Priftis A, Aivazidis S, Tsatsakis AM, Hayes AW, et al. Antioxidant effects of whey protein on muscle C2C12 cells. Food Chem. (2014) 155:271–8. doi: 10.1016/j.foodchem.2014.01.066
31. Oh J, Han Y, Kim J, Park C, Oh D, Yun H, et al. Anti-fatigue activity of a mixture of Stauntonia hexaphylla (Thunb.) decaisne and Vaccinium bracteatum Thunb. Fruit extract. Prev Nutr Food Sci. (2020) 25:380–8. doi: 10.3746/pnf.2020.25.4.380
32. Seo DY, Heo J-W, No M-H, Yoo S-Z, Ko JR, Park D-H, et al. Exercise training protects against atorvastatin-induced skeletal muscle dysfunction and mitochondrial dysfunction in the skeletal muscle of rats. J Clin Med. (2020) 9:2292. doi: 10.3390/jcm9072292
33. Schroder F, Lisso J, Obata T, Erban A, Maximova E, Giavalisco P, et al. Consequences of induced brassinosteroid deficiency in Arabidopsis leaves. BMC Plant Biol. (2014) 14:309. doi: 10.1186/s12870-014-0309-0
34. Nagy-Szakal D, Williams BL, Mishra N, Che X, Lee B, Bateman L, et al. Fecal metagenomic profiles in subgroups of patients with myalgic encephalomyelitis/chronic fatigue syndrome. Microbiome. (2017) 5:44. doi: 10.1186/s40168-017-0261-y
35. Chen HT, Han Y, Jahan I, Wu SY, Clark BC, Wiseman JS. Extracts of maca (Lepidium meyenii) root induce increased glucose uptake by inhibiting mitochondrial function in an adipocyte cell line. J Herb Med. (2019) 17-18:100282. doi: 10.1016/j.hermed.2019.100282
36. Wang YL, Shi XM, Li L, Efferth T, Shang D. The impact of artificial intelligence on traditional Chinese medicine. Am J Chin Med. (2021) 49:1297–314. doi: 10.1142/s0192415x21500622
37. Zhang Y, Zhou FX, Ge FH. Effects of combined extracts of Lepidium meyenii and Allium tuberosum Rottl. On erectile dysfunction. BMC Complem Altern Med. (2019) 19:135. doi: 10.1186/s12906-019-2542-4
38. Campbell MS, Carlini NA, Fleenor BS. Influence of curcumin on performance and post-exercise recovery. Crit Rev Food Sci Nutr. (2020) 61:1152–62. doi: 10.1080/10408398.2020.1754754
39. Han C, Li F, Liu Y, Ma J, Yu X, Wu X, et al. Modified Si-Ni-San decoction ameliorates central fatigue by improving mitochondrial biogenesis in the rat hippocampus. Evid Based Complement Alternat Med. (2018) 2018:9452127. doi: 10.1155/2018/9452127
40. Ji W, Jiang T, Sun Z, Teng F, Ma C, Huang S, et al. The enhanced pharmacological effects of modified traditional Chinese medicine in attenuation of atherosclerosis is driven by modulation of gut microbiota. Front Pharmacol. (2020) 11:546589. doi: 10.3389/fphar.2020.546589
41. Huang WC, Lee MC, Lee CC, Ng KS, Hsu YJ, Tsai TY, et al. Effect of Lactobacillus plantarum TWK10 on exercise physiological adaptation, performance, and body composition in healthy humans. Nutrients. (2019) 11:2836. doi: 10.3390/nu11112836
42. Ravichandra V, Martoni CJ, Kavanagh K, Eri R. Lactobacillus acidophilus DDS-1 modulates the gut microbial co-occurrence networks in aging mice. Nutrients. (2022) 14:977. doi: 10.3390/nu14050977
43. Jezbera J, Sharma AK, Brandt U, Doolittle WF, Hahn MW. “Candidatus planktophila limnetica”, an actinobacterium representing one of the most numerically important taxa in freshwater bacterioplankton. Int J Syst Evol Microbiol. (2009) 59:2864–9. doi: 10.1099/ijs.0.010199-0
44. Yu M, Jia H, Zhou C, Yang Y, Zhao Y, Yang M, et al. Variations in gut microbiota and fecal metabolic phenotype associated with depression by 16S rRNA gene sequencing and LC/MS-based metabolomics. J Pharm Biomed Anal. (2017) 138:231–9. doi: 10.1016/j.jpba.2017.02.008
45. Shao YX, Huang M, Cuo WJ, Feng LJ, Wu YN, Cai YH, et al. Discovery of a phosphodiesterase 9A inhibitor as a potential hypoglycemic agent. J Med Chem. (2014) 57:10304–13. doi: 10.1021/jm500836h
46. Zhu Y, Zhang J, Wang J, Yang Z, Ge Y, Zhou T. Effects of paeoniflorin on the content, synthesis and hydrolase of cGMP in the hippocampus of rats with blood deficiency and liver depression syndrome. China J Tradit Chin Med Pharm. (2015) 30:818–20.
Appendix
According to the conversion ratio between mice and humans, the daily MCP dose (2.0 g/kg) for mice can be calculated as the following formula: conversion ratio of surface area between mice and humans (10) × a daily dose of an adult (12 g)/average weight of adults (60 kg), that is: 10 × 12 (g)/60 (kg) = 2.0 g/kg.
Keywords: anti-fatigue, gut microbiota, microbial function, oxidative stress, host metabolism
Citation: Zhu H, Wang R, Hua H, Qian H and Du P (2022) Deciphering the potential role of Maca compounds prescription influencing gut microbiota in the management of exercise-induced fatigue by integrative genomic analysis. Front. Nutr. 9:1004174. doi: 10.3389/fnut.2022.1004174
Received: 27 July 2022; Accepted: 27 September 2022;
Published: 12 October 2022.
Edited by:
Dalia El Khoury, University of Guelph, CanadaReviewed by:
Kun Ye, ETH Zürich, SwitzerlandFrancesca Ciani, University of Naples Federico II, Italy
Copyright © 2022 Zhu, Wang, Hua, Qian and Du. This is an open-access article distributed under the terms of the Creative Commons Attribution License (CC BY). The use, distribution or reproduction in other forums is permitted, provided the original author(s) and the copyright owner(s) are credited and that the original publication in this journal is cited, in accordance with accepted academic practice. No use, distribution or reproduction is permitted which does not comply with these terms.
*Correspondence: He Qian, amtf168168126.com; Peng Du, dupengaf@163.com