- 1College of Pharmacy, Seoul National University, Seoul, South Korea
- 2Research Institute of Pharmaceutical Sciences, Seoul National University, Seoul, South Korea
- 3Department of Statistics, Sungshin Women’s University, Seoul, South Korea
- 4Department of Statistics, Seoul National University, Seoul, South Korea
- 5Plant Genomics and Breeding Institute, Seoul National University, Seoul, South Korea
Background: Quantitative evidence of the metabolic and cardiovascular effects of apples (Malus domestica) is lacking in interventional studies. This study aimed to summarize the available evidence of the beneficial effects of apples and apple-derived products (ADPs) on metabolic and cardiovascular markers.
Methods: Peer-reviewed randomized controlled trials (RCTs) were identified from four databases on May 3, 2021 and regularly updated until the end of May 2021. Demographic characteristics, intervention types, and evaluation parameters were extracted. A meta-analysis on the mean difference of change scores was conducted on commonly presented outcomes in the RCTs.
Results: The metabolic and cardiovascular effects of diverse regimens, including whole apple, apple extract, and apple juice, were examined in 18 eligible RCTs. Nine common evaluation outcomes were eventually introduced to the meta-analysis, including total cholesterol (TC), low-density lipoprotein (LDL), high-density lipoprotein (HDL), triglyceride, glucose, insulin, C-reactive protein, and systolic/diastolic blood pressures. The levels of TC (−2.69 mg/dL; 95% CI: −5.43, 0.04 mg/dL) and LDL (−2.80 mg/dL; 95% CI: −5.78, 0.17 mg/dL) showed a non-significant decreasing tendency after at least a week of apple consumption. Further subgroup analysis, particularly, a comparison with placebo as a control, showed a significant reduction in TC and LDL levels. When stratified by the baseline level, subjects with high TC and LDL level were shown to have more benefits from the apple intake. Intriguingly, apple and ADPs significantly reduced HDL levels to a small extent (−1.04 mg/dL; 95% CI: −1.79, −0.29 mg/dL). The other markers were mostly unaffected by the intervention.
Conclusion: Our investigation revealed that apples could improve blood cholesterol levels.
Systematic Review Registration: [https://www.crd.york.ac.uk/prospero/], identifier [CRD42020215977].
Introduction
The apple (Malus domestica) is one of the most widely consumed fruits and accounts for 12.5% of all fruits worldwide (1). Approximately 26.9 million small fresh apples are consumed daily in the United States (2) and approximately 22 kg of apples is consumed per capita annually in the total European diet (3). The functional properties of apples on metabolic syndromes have steadily gained attention, particularly because they are a rich source of functional phytochemicals such as flavonols, phloretin, and procyanidin oligomers (4–7). More specifically, apples and apple-derived products (ADPs) exert anti-obesity activities and reduce the risk of cardiovascular diseases (6–8). As a result, several systematic reviews have been conducted on the evidence-based outcomes of the biological effects of apple intake on metabolic and cardiovascular markers and complications. For example, a meta-analysis of prospective cohort studies on type 2 diabetes reported on the beneficial effect of apples on reducing the risk of disease (9). Notably, two systematic reviews evaluated the alteration of metabolic parameters following apple (10) or apple vinegar intake (11). Nevertheless, these studies were either limited to observational studies or lacked statistical power due to the small sample size.
This study aimed to systematically analyze randomized controlled trials (RCTs) that investigated the clinical functions of apples and ADPs through oral intake and evaluate their effects on the improvement of metabolic and cardiovascular markers compared to placebo or any alternative diet. There was no limitation regarding the processing method of ADPs, and all human subjects were considered eligible regardless of age and basal condition. Finally, we deduced the effect of apple and ADP intake on the nine most commonly reported markers by conducting a meta-analysis with exhaustive subgroup analyses.
Methods
Search Strategy
The study was registered at the PROSPERO International Prospective Register of Systematic Reviews (CRD42020215977) and followed the Preferred Reporting Items for Systematic Reviews and Meta-Analyses guidelines (Supplementary Table 1) (12). The databases PubMed, Embase, CENTRAL, and ClinicalTrials.gov were systematically searched with terms related to “apple” and metabolic and cardiovascular biomarkers such as “lipid profile,” “glucose,” “insulin,” and “C-reactive protein.” The full search terms are listed in Supplementary Table 2. There were no limitations in terms of the search period and language of the publications. Articles were preliminarily searched on February 13, 2020 and were first collected on May 3, 2021. The list was regularly updated by searching for RCTs published between the initial search date and the end of May 2021. Duplicates were automatically removed in Endnote X9, followed by manual inspection by two reviewers (SJK and NA) in a blinded manner.
Study Selection
We collected peer-reviewed RCTs that investigated the effects of oral intake of apple or ADPs on metabolic and cardiovascular markers. The markers included circulating lipids and lipoproteins, C-reactive protein (CRP), diabetic indicators, and blood pressure. We considered an RCT to be eligible for meta-analysis only if it provided quantitatively synthesizable data for calculating the mean difference of change score between the apple and control groups.
A study was deemed ineligible for inclusion if it was (did) (i) not designed as an RCT; (ii) unrelated to apple intake; (iii) not able to provide relevant data, such as the study employed significant apple intake as a control treatment or a comparison among different types of apple species; (iv) investigate the acute effect of apple intake (less than a week of treatment); or (v) review, conference, abstract, or any other secondary scientific reports. At least three reviewers (SJK, NA, and CWJ) independently evaluated the eligibility of each study in a blinded manner by screening the titles and abstracts. Disagreements were resolved by a third party through a careful discussion. Additional screening of reference reviews was performed to improve the coverage. We employed Rayyan QCRI, an open-source repository, to facilitate the screening of abstracts and titles (13).
Data Extraction
All data extraction procedures were performed by at least two reviewers for each RCT, and consensus was reached via discussion when conflicts arose. We first collected demographic information, including cohort allocation (country), basal disease condition, sex, and sample size. In terms of experimental details, study design (crossover vs. parallel), dosage, type of product, evaluation system, and adverse effects were recorded if applicable. Additionally, we conducted an in-house quality control, where another reviewer randomly checked the extracted information without any prior discussion.
Meta-Analysis
A meta-analysis was conducted using Review Manager 5.4 (Nordic Cochrane Center, The Cochrane Collaboration version 5.4). Common outcome parameters across the included studies were screened. An outcome was considered suitable for meta-analysis when more than five studies reported the outcome by either a change score or both baseline and post-intervention values with a treatment duration of at least 1 week. Therefore, we focused on the changes in total cholesterol (TC), high-density lipoprotein (HDL), low-density lipoprotein (LDL), triglyceride (TG), glucose, insulin, CRP, systolic blood pressure (SBP), and diastolic blood pressure (DBP). Before data integration, the units of TC, HDL, LDL, and TG data were converted to milligram per deciliter (mg/dL) by multiplying mmol/L by 38.67 for TC, HDL, and LDL, and by multiplying mmol/L by 88.57 for TG. In addition, the unit for glucose was converted from mmol/L to mg/dL by multiplying by 18.02, while the unit for insulin was converted to μU/mL. From individual studies, we extracted information on the change scores from baseline to post-intervention and considered their differences from the treatment and control groups. The standard error (SE) values of the differences were also determined. If a study did not provide the necessary statistics, we manually calculated them, as explained. The difference in the change scores was easily determined by subtracting the mean values of the treatment and control groups. However, the calculation of SE differed depending on the type of information (change in score vs. mean/standard deviation) and the type of experiment (parallel vs. crossover). To provide a more explicit explanation, we introduce the notations in Table 1. The calculation formulas used to derive the SE for four different cases are summarized in Table 2. For example, ρX1,X2 is the correlation coefficient between the two states X1 and X2. Notably, the SE of a crossover study was derived with an additional assumption of X2 = Y2, which stems from the assumption that a washout period eliminates the effect of intake in an individual. All statistics, except for the correlation coefficient(s), were obtained from a study depending on the type of given information and experiment. We set unknown correlation coefficients as 0.5, which is in line with previous literature (14). To check for potential bias from the imputed correlation, sensitivity analysis was conducted by plugging in 0.2 and 0.8 as the correlation coefficients. For a study with multiple comparison groups, the most relevant intervention and control groups were chosen (e.g., whole apple vs. placebo, instead of whole apple vs. vitamin juice). In addition, if a study reported measurements at multiple time points, then the time point most approximate to other studies was used (e.g., 4 weeks of intervention). Studies that reported only percentage changes as results and studies with results that could not be used due to missing data were excluded from the meta-analysis. Once all information was collected from the studies, we analyzed the input values using a generic inverse variance outcome and displayed the data as mean difference (MD) with a 95% confidence interval (CI) for measuring the effect for all the outcomes, except CRP, for which the standardized mean difference (SMD) of change scores was applied because there was high heterogeneity regarding the magnitude of blood levels. Data transformation from MD and SE of MD to SMD and SE of SMD was performed according to the type of experiment (parallel vs. crossover) for each study, following Cochrane guidelines (15, 16). We used a random-effects model because it incorporates both within-study and between-study heterogeneity. The effects of apples and ADPs were visualized using forest plots. Cochran’s Q test and I2 statistics were used to inspect heterogeneity across the studies. Multiple subgroup analyses were performed based on the participants’ basal condition (healthy subjects and subjects with metabolic and cardiovascular risk), treatment intervention type (whole apple, apple polyphenol, apple pectin, and apple vinegar), control intervention type (placebo and other materials), baseline level of the marker (low and high), sample type (serum and plasma), and design of the study (crossover and parallel). Placebo here indicates control diet treated with either true placebo that is undistinguishable to apple intervention or no intervention, without any specific functional compounds. Furthermore, a leave-one-out sensitivity analysis was performed to examine whether the overall effect depended heavily on a specific study. Statistical significance was set at p < 0.05.

Table 2. Calculation of standard error based on the type of information (change score vs. mean/standard deviation) and the type of experiments (parallel vs. crossover).
Publication Bias
Publication bias was evaluated for every marker using funnel plot visualization. Egger’s regression test and Begg’s rank test (not-continuity corrected) were conducted to determine the statistical values. A p-value of less than 0.1 indicated the existence of publication bias. Publication bias assessment was not performed for insulin and CRP because of the small number of studies (less than 10).
Quality Assessment
The risk of bias in individual studies was evaluated using the latest Cochrane Collaboration tool (RoB 2) for randomized trials (17). The tool introduces five distinct domains to assess different sources of bias, with each domain having multiple signaling questions. Individual domains were independently evaluated based on the answers to their signaling questions and categorized as either “low risk of bias,” “some concerns,” or “high risk of bias.” Multiple reviewers assessed the methodological qualities of all the included studies, and a consensus was reached via discussion of the conflicting judgments.
Quality of Evidence
The quality of evidence of meta-analysis outcomes was rated using the Grading of Recommendations Assessment, Development, and Evaluation (GRADE) (18). Since the current meta-analysis was conducted on RCTs, all evidences began as high-quality and gradually downgraded following the number of drawbacks, including risk of bias, inconsistency, indirectness, imprecision, and publication bias.
Results
Search Results
After performing systematic searches on the four databases, we retrieved 1,008 studies in total. Duplication was checked after all studies were imported into Endnote X9, and 150 duplicates were removed. Thereafter, 858 studies were screened for titles and abstracts. After careful evaluation, 799 studies were excluded owing to ineligibility. Finally, 59 studies were gathered for full-text screening. Eighteen studies, including a paper published after the first literature searched (19), were deemed eligible for data extraction. Figure 1 shows the flowchart of the study selection process.
Study Design and Participants
Among the included RCTs, 11 employed a parallel design (19–29), whereas the others employed a crossover study design (1, 30–35). The overall intervention period ranged from 1 to 12 weeks. Apples were administered in various forms such as in a drink (liquid supplement, clear juice, or puree), whole (fresh or dried), powder, extract, vinegar, and pomace. The apple extract was the most frequently tested ADP, particularly reinforced in polyphenolic compounds and apple pectin. Two other common forms of administration were unprocessed whole apple and apple juice. Whole-apple intake ranged from one to three apples per day. Cohort allocation was mainly located in Europe and North America.
Total Cholesterol, High-Density Lipoprotein, Low-Density Lipoprotein, and Triglyceride
Blood lipid profiles, including TC, HDL, LDL, and TG, were the most frequently reported metabolic markers following apple or ADP intake. Among the included RCTs, 17 (n = 793) provided applicable data for meta-analysis (1, 19–27, 29–35). Among them, 15 (n = 670) reported four lipid profile parameters; one study reported HDL, LDL, and TG (32); and the remaining one reported HDL and TG as the change scores (20). Regarding study design, 10 studies used a parallel design (19–27, 29), while the other seven employed a crossover design (1, 30–35). Regarding the type of intervention, apple polyphenols (n = 9) (21, 23, 25, 29–31, 33–35) were the most frequently used, followed by whole apple (n = 6) (1, 19, 20, 22, 27, 32), and apple pectin (n = 2) (24, 26). The treatment dosage varied depending on the type of formulation used. Serum and plasma were the two types of samples collected for measuring lipid levels in all trials. Eleven studies used placebo diets for control intervention (1, 19, 21, 23–27, 29, 33, 35), while the other studies used another type of intervention or a habitual diet, which might possess a different extension of the effect. Most of the included trials were conducted on a population with cardiovascular disease (CVD) risk factors (n = 13) such as dyslipidemia, high blood pressure, and obesity (1, 19, 21–25, 27, 29, 31, 33–35). The intervention period ranged from 1 to 12 weeks, with 4 weeks being the most common. The data on the study characteristics and evaluation parameters are presented in Table 3.
Overall, there was no statistically significant change in TC levels following apple and ADPs consumption in terms of blood concentration compared to those in the control group (MD: −2.33, 95% CI: −4.69, 0.03 mg/dL; I2 = 0%). However, a high tendency of reduction (p = 0.05) was observed (Figure 2). Notably, a significant reduction in TC levels was observed in the pooled analysis of 11 placebo-controlled trials (MD: −4.96, 95% CI: −8.83, −1.1 mg/dL; I2 = 0%). In addition, subgroup analysis of the study with a high baseline TC level also showed a significant reduction (MD: −3.26, 95% CI: −6.33, −0.19 mg/dL; I2 = 19%). In terms of administration forms, apple polyphenol was found to be likely to lower TC, while apple pectin (n = 2) significantly lowered TC in the treatment intervention subgroup analysis. Moreover, a significant reduction was observed when the sample type was confined to plasma (MD: −6.2, 95% CI: −11.29, −1.1, and I2 = 0%). All the outcomes of subgroup analysis and heterogeneity for TC, LDL, HDL, and TG are presented in Table 4.
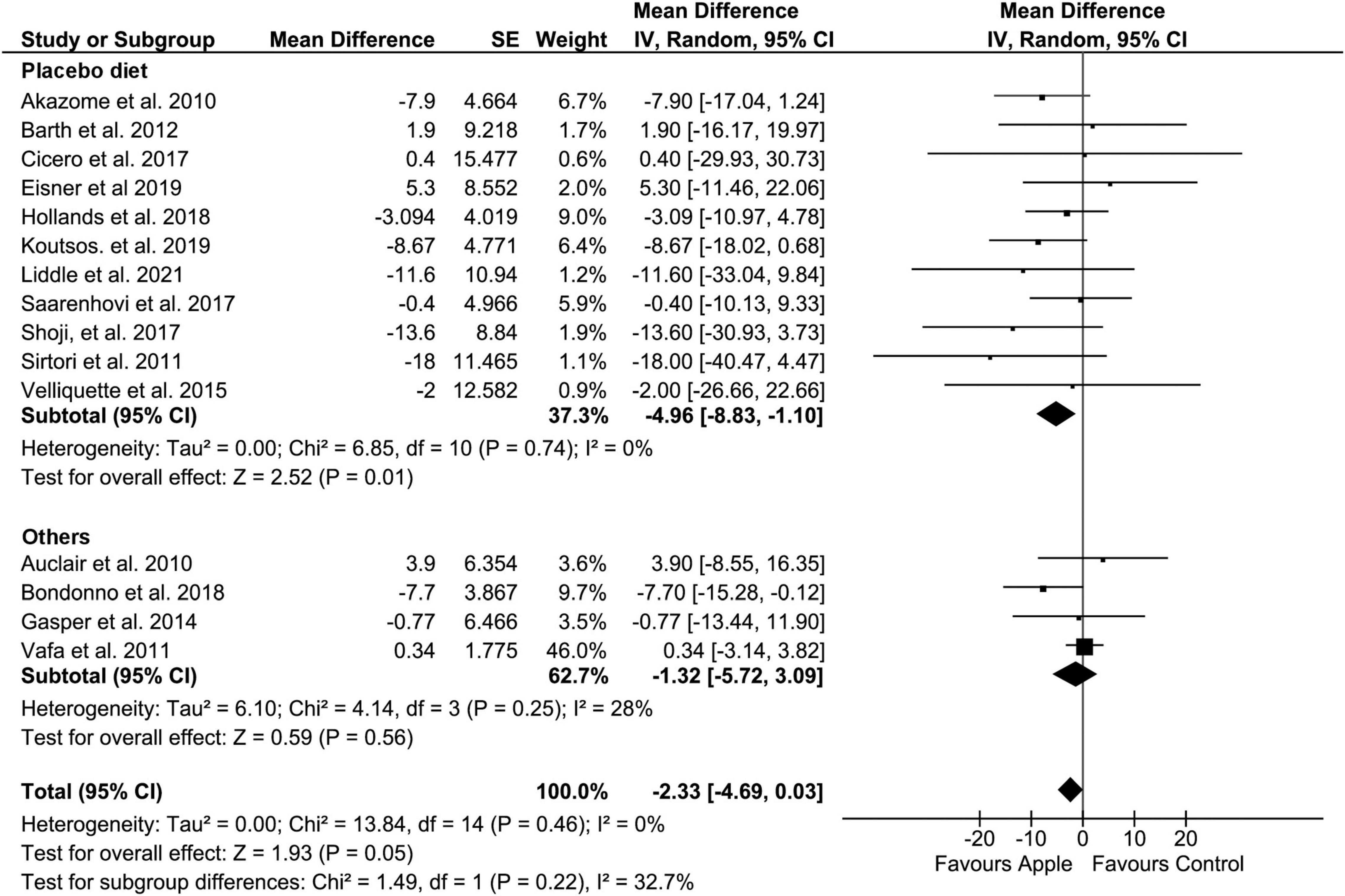
Figure 2. Meta-analysis results of total cholesterol. Forest plot is stratified by placebo diet or other alternative diets.
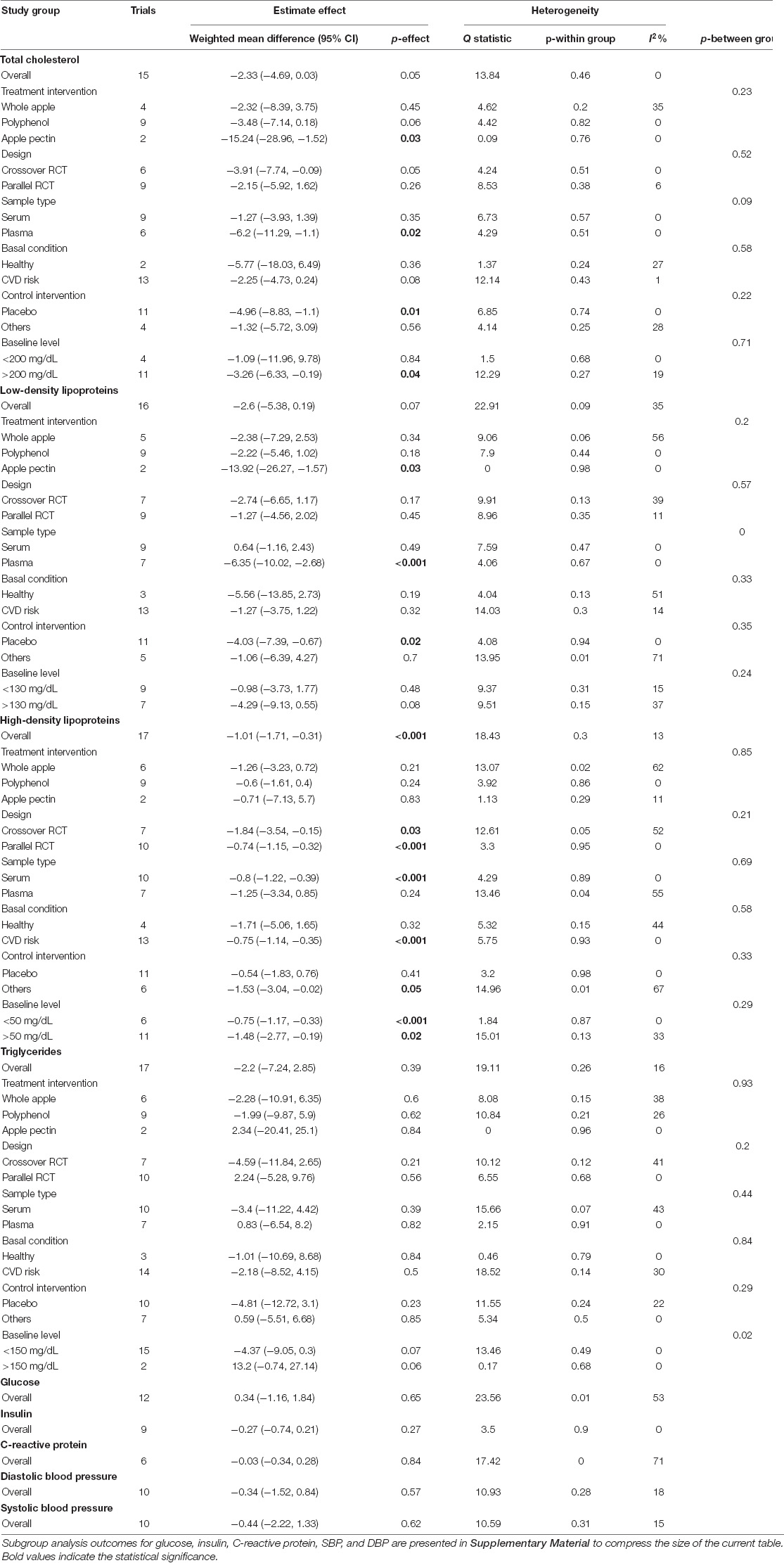
Table 4. Meta-analysis summarization of total cholesterol (TC), low-density lipoprotein (LDL), high-density lipoprotein (HDL), triglycerides (TG), glucose, insulin, c-reactive protein (CRP), diastolic blood pressure (DBP), and systolic blood pressure (SBP).
Regarding LDL, when compared to the control diet, the overall result did not show a significant decrease in blood level (MD: −2.6, 95% CI: −5.38, 0.19 mg/dL; I2 = 35%). However, similar to TC, a clear tendency of reduction in LDL was easily recognized (p = 0.07) (Figure 3). Moreover, subgroup analysis on the placebo-controlled comparison showed a significant decrease in LDL (MD: −4.03, 95% CI: −7.39, −0.67 mg/dL; I2 = 0%). Regarding administration forms, apple pectin displayed a significant reduction in LDL concentration (MD: −13.92, 95% CI: −26.27, −1.57 mg/dL; I2 = 0%). The results were also similar to those of TC when the study was confined to the plasma sample (MD: −6.35, 95% CI: −10.02, −2.68 mg/dL, I2 = 0%).
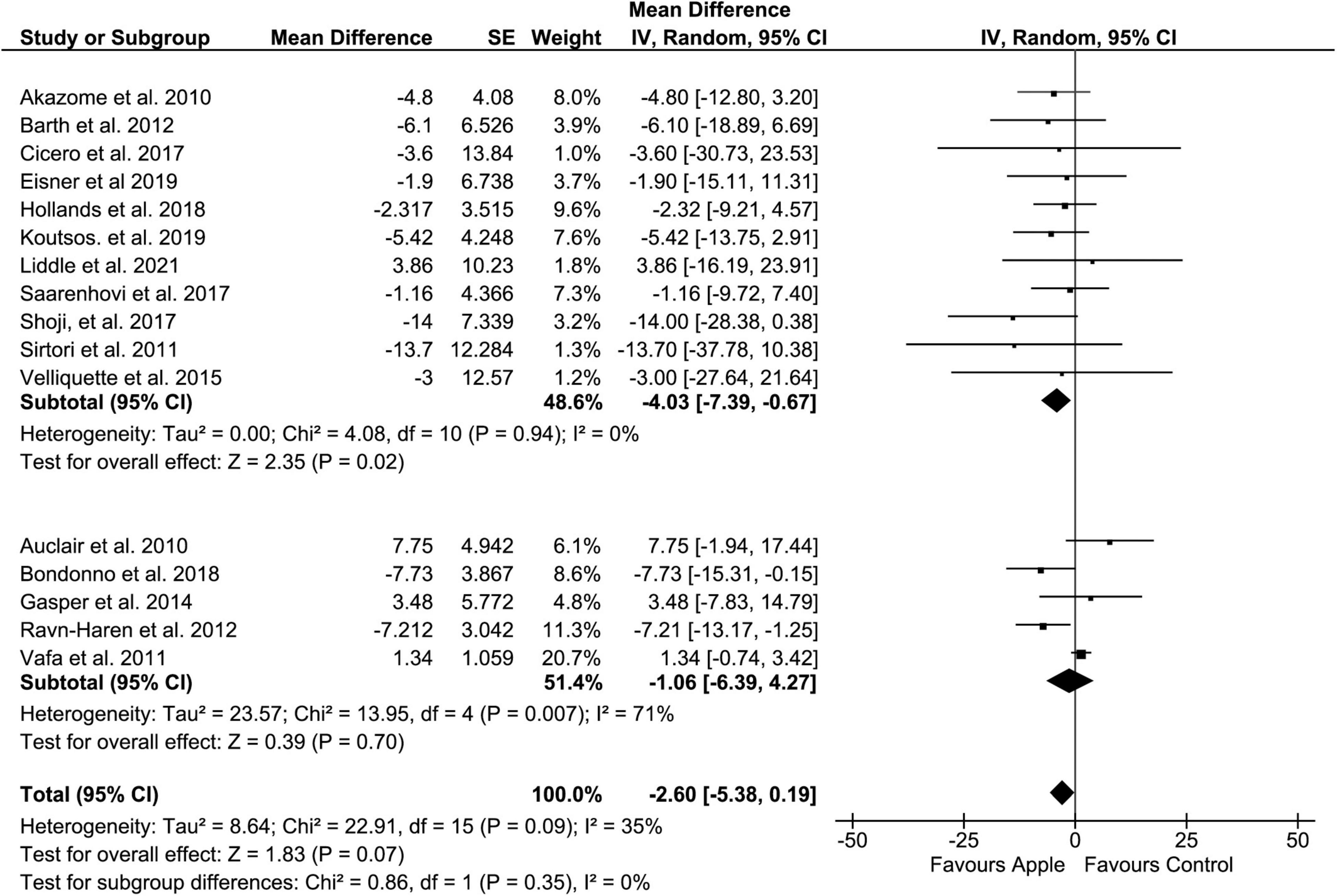
Figure 3. Meta-analysis results of low-density lipoprotein. Forest plot is stratified by placebo diet or other alternative diets.
Overall, HDL concentration was slightly but significantly decreased (MD: −1.01, 95% CI: −1.71, −0.31 mg/dL; I2 = 13%) (Figure 4). Most of the subgroup analyses also showed a slight reduction in HDL, except for the subgroup on the placebo-controlled comparison, healthy subjects, and the plasma sample. However, there was no evidence of alteration in TG compared to that in control diets (MD: −2.2, 95% CI: −7.24, 2.85 mg/dL; I2 = 16%) (Figure 5). Intriguingly, significant differences were observed when the subgroup was stratified to the high and low baseline TG value, as the subjects with low baseline (<150 mg/dL) showed a trend of reduction (MD: −4.37, 95% CI: −9.05, 0.3 mg/dL; I2 = 0%), whereas the opposites (>150 mg/dL) showed huge increase in the TG level (MD: 13.2, 95% CI: −0.74, 27.14 mg/dL; I2 = 0%).
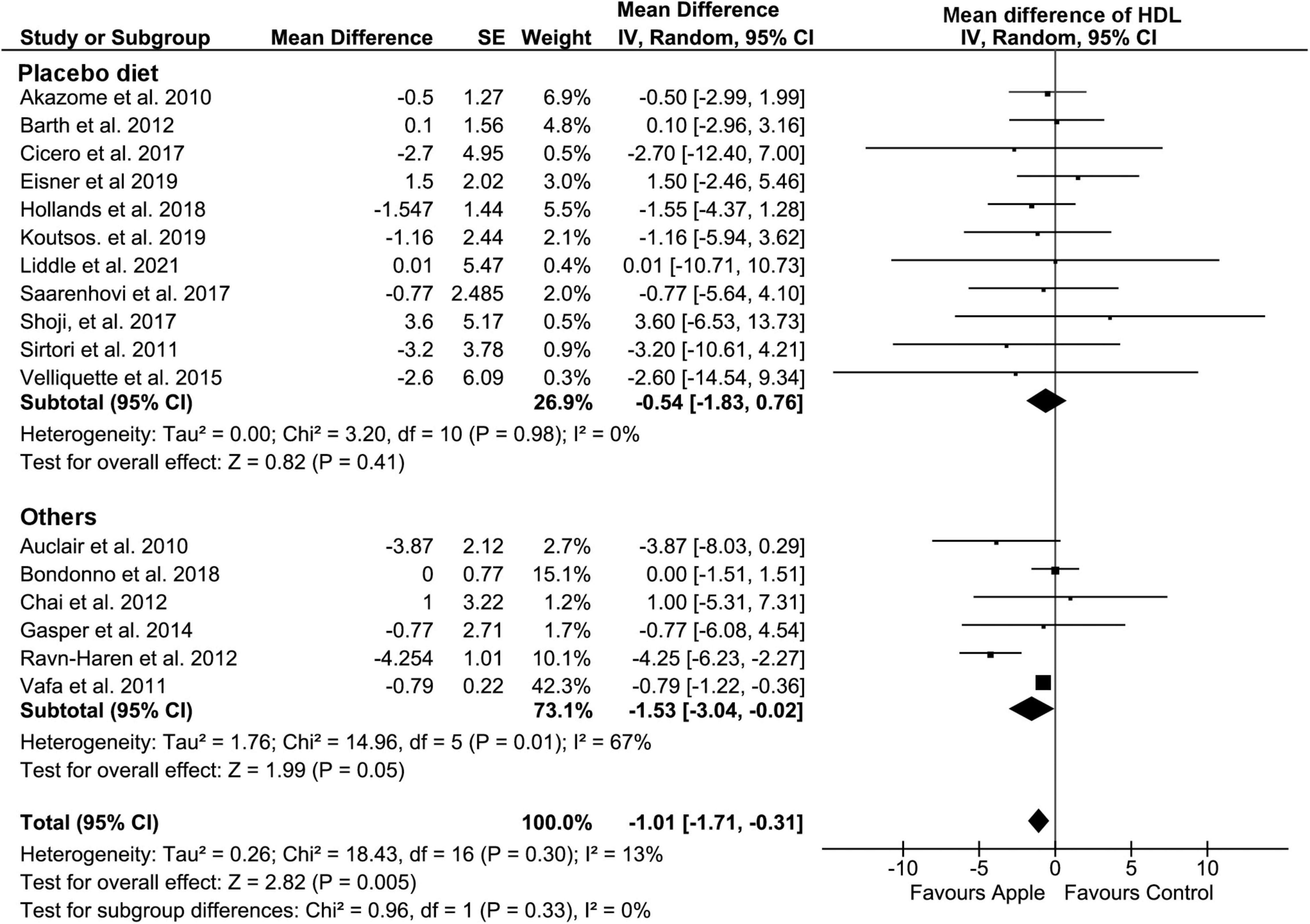
Figure 4. Meta-analysis results of high-density lipoprotein. Forest plot is stratified by placebo diet or other alternative diets.
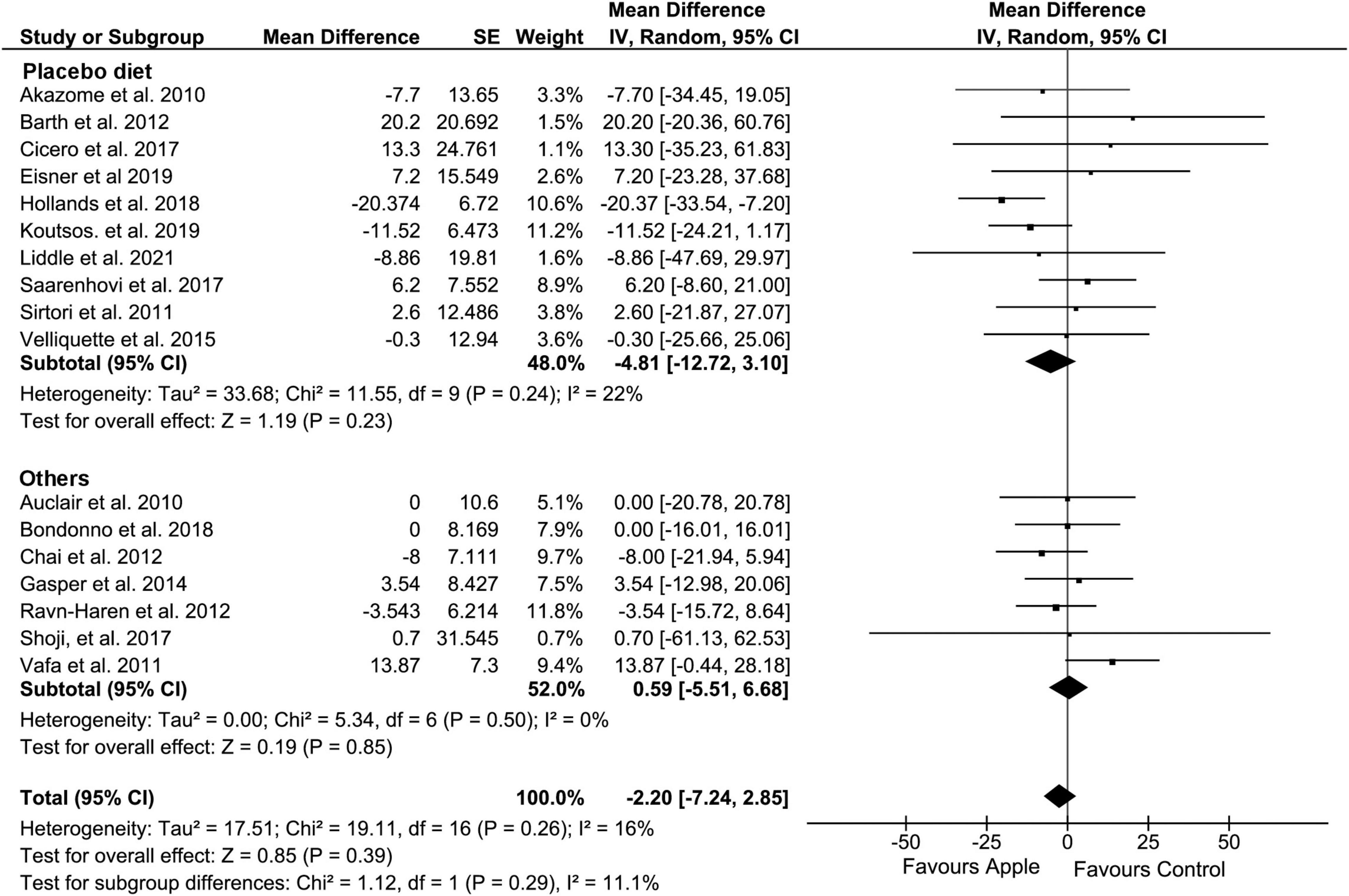
Figure 5. Meta-analysis results of triglycerides. Forest plot is stratified by placebo diet or other alternative diets.
Glucose, Insulin, and C-Reactive Protein
Glucose, insulin, and CRP levels were reported in 12 studies (1, 19, 21, 23, 24, 26–28, 31, 33–35), nine studies (1, 19, 23, 24, 26–28, 32, 33), and six studies (20, 25, 27, 30, 34, 35), respectively. Serum and plasma samples were collected under fasting conditions in all included studies, except for the study conducted by Akazome et al. which did not provide clear information (23). No significant effects were found for glucose, insulin, or CRP levels. The results were consistent regardless of subgroup analysis. Details of the meta-analysis results are presented in Supplementary Figure 1 and Supplementary Table 3.
Systolic Blood Pressure and Diastolic Blood Pressure
Alterations in SBP and DBP levels were reported in ten studies (1, 19, 21, 23, 28, 31–35). There were no significant changes in SBP and DBP in the overall outcomes, as well as in other subgroup analyses. The results of the overall meta-analysis and subgroup analysis are shown in Supplementary Figure 2 and Supplementary Table 4. Overall meta-analysis results of glucose, insulin, CRP, SBP, and DBP are presented in Table 4.
Sensitivity Analysis
Sensitivity analysis revealed that several values that were close to the null hypothesis border became a significant result with a correlation coefficient of 0.8 and was conserved with an imputation of 0.2. The most notable alterations were the overall results of TC and LDL, which became significant with a correlation coefficient of 0.8 (MD: −3.71, 95% CI: −6.63, −0.79 mg/dL; I2 = 48%; MD: −2.98, 95% CI: −5.81, −0.15 mg/dL; I2 = 68%, respectively), whereas the non-significant values remained so when 0.2 was plugged in. Detailed results of the sensitivity analysis with different correlation coefficients are presented in Supplementary Tables 5, 6. Through leave-one-out sensitivity analysis, we found that the overall meta-analysis results of TC and LDL were highly influenced by a single study by Vafa et al. (22), because it accounted for 46.0 and 20.7% of the total weight, respectively, while it included only 23 participants in each group. Therefore, removing this study dramatically changed the overall outcomes of TC (MD: −4.60, 95% CI: −7.81, −1.39 mg/dL; I2 = 0%) and LDL (MD: −3.83, 95% CI: −6.39, −1.27 mg/dL; I2 = 0%) levels.
Heterogeneity
I2 values for the overall meta-analysis ranged from 0% to 66%. We removed two studies (36, 37) because they were conducted in the same year and hospital with similar criteria, and showed inconsistent data reports. Therefore, the duplicated data was suspected. More importantly, a circumstance that the heterogeneity dramatically changed (e.g., from 0 to 92% for overall TC) in their sensitivity analysis, made us decide to exclude them from the meta-analysis. In addition, another study by Soriano-Maldonado et al. that investigated the effect of apple juice with different polyphenol and vitamin C concentrations was excluded after careful consideration due to the ambiguous study objective (38). As the study compared a mixture of two components with different concentrations, it was not feasible to indicate which is the treatment or control group as well as the component accounting for the main functionality for individual effects.
Adverse Effects
From the 18 included trials, nine described information about the side effects potentially induced on account of the pharmacological use of apple (Table 3) (1, 19, 21, 23, 24, 29, 31, 33, 35). There were no observable adverse effects in six trials, whilst, non-fatal and mild side effects such as headache and rash were reported in 3 trials (24, 29, 35).
Publication Bias
Tests of asymmetry showed a potential risk of publication bias on LDL (Egger’s test, P = 0.06) and SBP (Egger’s test, P = 0.01; Begg’s test, P = 0.09) (Supplementary Table 7). Nevertheless, no significant visual evidence of publication bias for LDL was observed when funnel plots were inspected (Supplementary Figure 3). There was no statistical evidence of publication bias for the other parameters measured by Egger’s regression test and Begg’s rank test.
Quality Assessment
Individual quality assessment outcome is presented in Supplementary Table 8. The majority of included RCTs were evaluated to possess a certain risk of bias overall, mainly owing to the potential risk of bias due to deviations from the intended interventions. This was attributed to the general study design of the included RCTs, where they frequently treated apple products without concealing the flavor or smell, such as apple juice, or the whole apple itself.
Quality of Evidence
The evidence profile of GRADE assessment is described in Supplementary Table 9. The confidence for reporting TC, LDL, TG, DBP, and SBP was rated as moderate quality (all downgraded for indirectness), whereas low quality for HDL, glucose, and insulin and very low quality for CRP were rated.
Discussion
The current study aimed to introduce the present state of studies investigating the effect of apples on metabolic and cardiovascular markers. Consequently, 18 RCTs were systematically reviewed, and the commonly reported markers were meta-analyzed. Our study revealed significant reductions in TC and LDL concentrations upon apple and ADP intake when compared with the placebo control. Stratification by baseline level revealed potential beneficial effect of apple intake on the subjects with high TC and LDL. Intriguingly, HDL levels were also significantly decreased, although this cannot be considered beneficial. We found no evidence of changes in other markers, including TG, glucose, insulin, CRP, SBP, and DBP. To the best of our knowledge, this is the first comprehensive meta-analysis of RCTs regarding the effect of apples on metabolic and cardiovascular risk factors, which encompasses an unprecedentedly large sample size (up to 793 participants).
Although the current meta-analysis did not observe significant overall TC and LDL alteration, it revealed that consumption of apple and ADPs potentially lowers TC and LDL, especially in the subgroup with the so-called “placebo-controlled diet.” The absence of significant TC and LDL reduction in alterative diets potentially owes to other unidentified functional components. For example, the control intervention in the RCTs by Bondonno et al. and Auclair et al. was still a form of apple, albeit with a lower polyphenol concentration, and it may not rule out the effect of other components of the whole food matrix. Our findings broadly support previous systematic reviews and meta-analyses, which reported an inverse correlation between the intake of fruits and vegetables and the risk of CVD, including hypertension and coronary heart disease (CHD) (39–42). Gayer et al. conducted a meta-analysis on apples, pears, and their products and suggested the beneficial effects of apples and ADPs by reporting the trend of reduced risk of CVD and diabetes (10). Similarly, Launholt et al. conducted a systematic review of apple vinegar’s effects on metabolic parameters and suggested the potential blood glucose- and lipid-lowering effects of apple vinegar consumption (11).
The effects on TC and LDL were greater in the population with cardiovascular risk in the basal state. More specifically, a significant reduction in TC was observed in a group of people with high baseline cholesterol levels (>200 mg/dL), and a similar but non-significant trend was observed in the high LDL group (>130 mg/dL). Non-significant decreasing trends were also observed for TC and LDL when stratified for basal disease condition (CVD risk) indicated by each study, and removing data from Vafa et al. reversed the statistical outcome. In a recent meta-analysis of prospective studies, high apple and pear intakes were inversely associated with CHD and total stroke events (43). A prospective cohort study investigating the correlation between fruit intake and abdominal aortic calcification reported a significantly low odds ratio in a high apple intake fractile group (44). On the other hand, TG level was highly increased in high TG group (>150 mg/dL). However, it only included two research data and again, removing data from Vafa et al. turns the phenomenon back to baseline.
Even though there was an absence of statistical significance, the subgroup analysis related to treatment intervention suggested that the polyphenol-rich fraction of apples showed a high tendency to reduce TC and LDL. Both became significant after removal of the data from Auclair et al. The beneficial effects of apple and ADPs enriched with polyphenols have not been investigated in any other meta-analysis. The results of this study particularly revealed a direction for future research to explore the potential functions of apple polyphenols in lowering the risk of CVD. A possible explanation for the beneficial effects on the cardiovascular system is the abundance of phytochemicals such as procyanidins, hydroxycinnamic acids, and flavonols. These components have been investigated for their efficient antioxidant activity, lipid-lowering activity, and reduction in cardiotoxicity (45–48). Among the phenolic groups, anthocyanins were reported to have TC- and LDL-lowering effects, which were deduced by a meta-analysis of 30 and 27 RCTs (49). Dietary epicatechin, for which apples account for 28% of its intake, was also inversely correlated with CVD and CHD according to the 25-year follow-up data of elderly subjects (50). A recent longitudinal study on over 10,000 women with 12–15 years of follow-up reported a significantly low relative risk in the group with high flavone and flavonol intake, of which one of their major sources was apples (51). However, our analysis had limitations in that we could not separately consider all the various doses and ADP forms, which may be a potential reason for the absence of statistical significance. Therefore, additional well-designed RCTs on dose-response are warranted to elucidate the effect of apple polyphenols on lipid management. Apple pectin, a soluble fiber fraction, also significantly reduced in TC and LDL levels. However, the results should be interpreted with caution, as only a limited number of studies were involved in drawing this conclusion. Although the overall HDL level decreased with apple consumption, the quality of evidence was evaluated low according to GRADE assessment and more data are needed to confirm this phenomenon as a subgroup of placebo-controlled diets did not follow the overall outcome.
Overall, blood glucose, insulin, blood pressure, and CRP concentration were not associated with apple or ADP consumption. This could be explained by the fact that most of the included studies investigated these factors as secondary outcomes. A few studies have investigated the attributes of apple polyphenols in glycemic management, and most of them focused on acute post-prandial responses (52–54). However, apples have been suggested to improve insulin sensitivity (55, 56). Notably, subgroup analysis of serum sample type revealed a potential effect of apple intake on the insulin sensitivity increase (MD: −0.51, 95% CI: −1.07, 0.05 μU/mL; p = 0.07; I2 = 0%). Considering the general consensus that serum is recommended over plasma for blood insulin measurement (57), we expect further trials to reverse the current outcome. Despite the lack of significant favorable effects observed in our present meta-analysis, several studies have reported the beneficial effects of apple extract on blood pressure and endothelial function (31, 58). However, the evidence remains uncertain due to the inconsistency of results presented in our meta-analysis. Finally, the effect of apples and ADPs on CRP showed no potential association except in a subgroup analysis of two studies on plasma samples.
There are a few limitations to this study that need to be addressed. First, some subgroups (e.g., apple pectin) have a limited number of studies; therefore, the statistical power is relatively weak for such an analysis. A dose-dependent analysis was not conducted in our study, neither was a more sub-categorized analysis of each specific type of apple intervention. This is because various specific components were used in each study, for example, flavanol, epicatechin, or total polyphenol in the apple polyphenol group. In the same vein, the heterogeneity in the intervention of apple and control positions all 9 markers at below the good quality of evidence. Finally, there have been multiple data imputations due to missing correlation information, which potentially distorts the original outcome. To avoid bias due to imputation, we counter-checked the results with a sensitivity analysis by plugging in different correlation coefficients including 0.2 and 0.8.
Conclusion
The current meta-analysis demonstrated that more than a week of apple and ADP intake can reduce TC and LDL levels when compared to a placebo-controlled diet. HDL levels were also significantly reduced upon apple consumption, while TG, glucose, insulin, CRP, SBP, and DBP were unaffected (p > 0.05) by the intervention. We anticipate that our results will guide the direction of future investigations and be statistically reinforced by further follow-up clinical trials with larger populations and longer study periods.
Data Availability Statement
The original contributions presented in the study are included in the article/Supplementary Material, further inquiries can be directed to the corresponding author/s.
Author Contributions
SJK, NA, NL, and SWK designed the research (project conception, development of overall research plan, and study oversight). SJK, NA, and CWJ conducted the research (systematic search, article screening, and data synthesis). NA, YCY, EGL, MK, and EYS conducted the research (data extraction). SJK, CWJ, THK, and YD conducted the research (quality assessment). SP, YHC, and JL performed the statistical analysis. SJK and NA wrote the manuscript. SWK had primary responsibility for final content. All authors read and approved the final manuscript.
Funding
This research was supported by the Rural Development Administration of Korea (PJ01420102).
Conflict of Interest
The authors declare that the research was conducted in the absence of any commercial or financial relationships that could be construed as a potential conflict of interest.
Publisher’s Note
All claims expressed in this article are solely those of the authors and do not necessarily represent those of their affiliated organizations, or those of the publisher, the editors and the reviewers. Any product that may be evaluated in this article, or claim that may be made by its manufacturer, is not guaranteed or endorsed by the publisher.
Supplementary Material
The Supplementary Material for this article can be found online at: https://www.frontiersin.org/articles/10.3389/fnut.2022.766155/full#supplementary-material
References
1. Koutsos A, Riccadonna S, Ulaszewska MM, Franceschi P, Trošt K, Galvin A, et al. Two apples a day lower serum cholesterol and improve cardiometabolic biomarkers in mildly hypercholesterolemic adults: a randomized, controlled, crossover trial. Am J Clin Nutr. (2019) 111:307–18. doi: 10.1093/ajcn/nqz282
2. Davis MA, Bynum JPW, Sirovich BE. Association between apple consumption and physician visits: appealing the conventional wisdom that an apple a day keeps the doctor away. JAMA Intern Med. (2015) 175:777–83. doi: 10.1001/jamainternmed.2014.5466
3. Almeida DPF, Gião MS, Pintado M, Gomes MH. Bioactive phytochemicals in apple cultivars from the Portuguese protected geographical indication “Maçã de Alcobaça:” basis for market segmentation. Int J Food Prop. (2017) 20:2206–14. doi: 10.1080/10942912.2016.1233431
4. Asgary S, Rastqar A, Keshvari M. Weight loss associated with consumption of apples: a review. J Am Coll Nutr. (2018) 37:627–39. doi: 10.1080/07315724.2018.1447411
5. Patocka J, Bhardwaj K, Klimova B, Nepovimova E, Wu Q, Landi M, et al. Malus domestica: a review on nutritional features, chemical composition, traditional and medicinal value. Plants. (2020) 9:1408. doi: 10.3390/plants9111408
6. Sesso HD, Gaziano JM, Liu S, Buring JE. Flavonoid intake and the risk of cardiovascular disease in women. Am J Clin Nutr. (2003) 77:1400–8. doi: 10.1093/ajcn/77.6.1400
7. Knekt P, Jarvinen R, Reunanen A, Maatela J. Flavonoid intake and coronary mortality in Finland: a cohort study. BMJ. (1996) 312:478–81. doi: 10.1136/bmj.312.7029.478
8. Conceição de Oliveira M, Sichieri R, Sanchez Moura A. Weight loss associated with a daily intake of three apples or three pears among overweight women. Nutrition. (2003) 19:253–6. doi: 10.1016/s0899-9007(02)00850-x
9. Guo XF, Yang B, Tang J, Jiang JJ, Li D. Apple and pear consumption and type 2 diabetes mellitus risk: a meta-analysis of prospective cohort studies. Food Funct. (2017) 8:927–34. doi: 10.1039/c6fo01378c
10. Gayer BA, Avendano EE, Edelson E, Nirmala N, Johnson EJ, Raman G. Effects of intake of apples, pears, or their products on cardiometabolic risk factors and clinical outcomes: a systematic review and meta-analysis. Curr Dev Nutr. (2019) 3:nzz109. doi: 10.1093/cdn/nzz109
11. Launholt TL, Kristiansen CB, Hjorth P. Safety and side effects of apple vinegar intake and its effect on metabolic parameters and body weight: a systematic review. Eur J Nutr. (2020) 59:2273–89. doi: 10.1007/s00394-020-02214-3
12. Page MJ, McKenzie JE, Bossuyt PM, Boutron I, Hoffmann TC, Mulrow CD, et al. The PRISMA 2020 statement: an updated guideline for reporting systematic reviews. BMJ. (2021) 372:n71. doi: 10.1136/bmj.n71
13. Ouzzani M, Hammady H, Fedorowicz Z, Elmagarmid A. Rayyan—a web and mobile app for systematic reviews. Syst Rev. (2016) 5:210. doi: 10.1186/s13643-016-0384-4
14. Elbourne DR, Altman DG, Higgins JPT, Curtin F, Worthington HV, Vail A. Meta-analyses involving cross-over trials: methodological issues. Int J Epidemiol. (2002) 31:140–9. doi: 10.1093/ije/31.1.140
15. Higgins JP, Li T, Deeks JJ. Choosing effect measures and computing estimates of effect. 2nd ed. In: Higgins JPT, Thomas J, Chandler J editors. Cochrane Handbook for Systematic Reviews of Interventions. (Hoboken, NJ: John Wiley & Sons) (2019). p. 143–76. doi: 10.1002/9781119536604.ch6
16. Higgins JP, Eldridge S, Li T. Including variants on randomized trials. In: Higgins JPT, Thomas J, Chandler J, Cumpston M, Li T, Page MJ editors. Cochrane Handbook for Systematic Reviews of Interventions. (London: Cochrane) (2019). p. 569–93. doi: 10.1002/9781119536604.ch23
17. Sterne JAC, Savović J, Page MJ, Elbers RG, Blencowe NS, Boutron I, et al. RoB 2: a revised tool for assessing risk of bias in randomised trials. BMJ. (2019) 366:l4898. doi: 10.1136/bmj.l4898
18. Guyatt GH, Oxman AD, Vist GE, Kunz R, Falck-Ytter Y, Alonso-Coello P, et al. GRADE: an emerging consensus on rating quality of evidence and strength of recommendations. BMJ. (2008) 336:924–6. doi: 10.1136/bmj.39489.470347.AD
19. Liddle DM, Lin X, Cox LC, Ward EM, Ansari R, Wright AJ, et al. Daily apple consumption reduces plasma and peripheral blood mononuclear cell–secreted inflammatory biomarkers in adults with overweight and obesity: a 6-week randomized, controlled, parallel-arm trial. Am J Clin Nutr. (2021) 114:752–63. doi: 10.1093/ajcn/nqab094
20. Chai SC, Hooshmand S, Saadat RL, Payton ME, Brummel-Smith K, Arjmandi BH. Daily apple versus dried plum: impact on cardiovascular disease risk factors in postmenopausal women. J Acad Nutr Diet. (2012) 112:1158–68. doi: 10.1016/j.jand.2012.05.005
21. Cicero AFG, Caliceti C, Fogacci F, Giovannini M, Calabria D, Colletti A, et al. Effect of apple polyphenols on vascular oxidative stress and endothelium function: a translational study. Mol Nutr Food Res. (2017) 61:1700373. doi: 10.1002/mnfr.201700373
22. Vafa MR, Haghighatjoo E, Shidfar F, Afshari S, Gohari MR, Ziaee A. Effects of apple consumption on lipid profile of hyperlipidemic and overweight men. Int J Prev Med. (2011) 2:94–100.
23. Akazome Y, Kametani N, Kanda T, Shimasaki H, Kobayashi S. Evaluation of safety of excessive intake and efficacy of long-term intake of beverages containing apple polyphenols. J Oleo Sci. (2010) 59:321–38. doi: 10.5650/jos.59.321
24. Sirtori CR, Triolo M, Bosisio R, Bondioli A, Calabresi L, De Vergori V, et al. Hypocholesterolaemic effects of lupin protein and pea protein/fibre combinations in moderately hypercholesterolaemic individuals. Br J Nutr. (2012) 107:1176–83. doi: 10.1017/S0007114511004120
25. Barth SW, Koch TC, Watzl B, Dietrich H, Will F, Bub A. Moderate effects of apple juice consumption on obesity-related markers in obese men: impact of diet-gene interaction on body fat content. Eur J Nutr. (2012) 51:841–50. doi: 10.1007/s00394-011-0264-6
26. Shoji T, Yamada M, Miura T, Nagashima K, Ogura K, Inagaki N, et al. Chronic administration of apple polyphenols ameliorates hyperglycaemia in high-normal and borderline subjects: a randomised, placebo-controlled trial. Diabetes Res Clin Pract. (2017) 129:43–51. doi: 10.1016/j.diabres.2017.03.028
27. Eisner A, Ramachandran P, Cabalbag C, Metti D, Shamloufard P, Kern M, et al. Effects of dried apple consumption on body composition, serum lipid profile, glucose regulation, and inflammatory markers in overweight and obese children. J Med Food. (2020) 23:242–9. doi: 10.1089/jmf.2019.0044
28. Gheflati A, Bashiri R, Ghadiri-Anari A, Reza JZ, Kord MT, Nadjarzadeh A. The effect of apple vinegar consumption on glycemic indices, blood pressure, oxidative stress, and homocysteine in patients with type 2 diabetes and dyslipidemia: a randomized controlled clinical trial. Clin Nutr ESPEN. (2019) 33:132–8. doi: 10.1016/j.clnesp.2019.06.006
29. Velliquette RA, Grann K, Missler SR, Patterson J, Hu C, Gellenbeck KW, et al. Identification of a botanical inhibitor of intestinal diacylglyceride acyltransferase 1 activity via in vitro screening and a parallel, randomized, blinded, placebo-controlled clinical trial. Nutr Metab (Lond). (2015) 12:27. doi: 10.1186/s12986-015-0025-2
30. Gasper A, Hollands W, Casgrain A, Saha S, Teucher B, Dainty JR, et al. Consumption of both low and high (-)-epicatechin apple puree attenuates platelet reactivity and increases plasma concentrations of nitric oxide metabolites: a randomized controlled trial. Arch Biochem Biophys. (2014) 559:29–37. doi: 10.1016/j.abb.2014.05.026
31. Bondonno NP, Bondonno CP, Blekkenhorst LC, Considine MJ, Maghzal G, Stocker R, et al. Flavonoid-rich apple improves endothelial function in individuals at risk for cardiovascular disease: a randomized controlled clinical trial. Mol Nutr Food Res. (2018) 62:1700674. doi: 10.1002/mnfr.201700674
32. Ravn-Haren G, Dragsted LO, Buch-Andersen T, Jensen EN, Jensen RI, Németh-Balogh M, et al. Intake of whole apples or clear apple juice has contrasting effects on plasma lipids in healthy volunteers. Eur J Nutr. (2013) 52:1875–89. doi: 10.1007/s00394-012-0489-z
33. Hollands WJ, Tapp H, Defernez M, Perez Moral N, Winterbone MS, Philo M, et al. Lack of acute or chronic effects of epicatechin-rich and procyanidin-rich apple extracts on blood pressure and cardiometabolic biomarkers in adults with moderately elevated blood pressure: a randomized, placebo-controlled crossover trial. Am J Clin Nutr. (2018) 108:1006–14. doi: 10.1093/ajcn/nqy139
34. Auclair S, Chironi G, Milenkovic D, Hollman PCH, Renard CMGC, Mégnien JL, et al. The regular consumption of a polyphenol-rich apple does not influence endothelial function: a randomised double-blind trial in hypercholesterolemic adults. Eur J Clin Nutr. (2010) 64:1158–65.
35. Saarenhovi M, Salo P, Scheinin M, Lehto J, Lovró Z, Tiihonen K, et al. The effect of an apple polyphenol extract rich in epicatechin and flavan-3-ol oligomers on brachial artery flow-mediated vasodilatory function in volunteers with elevated blood pressure. Nutr J. (2017) 16:73. doi: 10.1186/s12937-017-0291-0
36. Tenore GC, Caruso D, Buonomo G, D’Avino M, Ciampaglia R, Maisto M, et al. Lactofermented annurca apple puree as a functional food indicated for the control of plasma lipid and oxidative amine levels: results from a randomised clinical trial. Nutrients. (2019) 11:E122. doi: 10.3390/nu11010122
37. Tenore GC, Carotenuto A, Caruso D, Buonomo G, D’Avino M, Brancaccio D, et al. A nutraceutical formulation based on annurca apple polyphenolic extract is effective on intestinal cholesterol absorption: a randomised, placebo-controlled, crossover study. PharmaNutrition. (2018) 6:85–94. doi: 10.1016/j.phanu.2018.05.001
38. Soriano-Maldonado A, Hidalgo M, Arteaga P, de Pascual-Teresa S, Nova E. Effects of regular consumption of vitamin C-rich or polyphenol-rich apple juice on cardiometabolic markers in healthy adults: a randomized crossover trial. Eur J Nutr. (2014) 53:1645–57. doi: 10.1007/s00394-014-0670-7
39. Zhan J, Liu Y-J, Cai L-B, Xu F-R, Xie T, He Q-Q. Fruit and vegetable consumption and risk of cardiovascular disease: a meta-analysis of prospective cohort studies. Crit Rev Food Sci Nutr. (2017) 57:1650–63. doi: 10.1080/10408398.2015.1008980
40. Li B, Li F, Wang L, Zhang D. Fruit and vegetables consumption and risk of hypertension: a meta-analysis. J Clin Hypertens. (2016) 18:468–76. doi: 10.1111/jch.12777
41. Dauchet L, Amouyel P, Hercberg S, Dallongeville J. Fruit and vegetable consumption and risk of coronary heart disease: a meta-analysis of cohort studies. J Nutr. (2006) 136:2588–93. doi: 10.1093/jn/136.10.2588
42. Wang Y, Gallegos JL, Haskell-Ramsay C, Lodge JK. Effects of chronic consumption of specific fruit (berries, citrus and cherries) on CVD risk factors: a systematic review and meta-analysis of randomised controlled trials. Eur J Nutr. (2020) 60:615–39. doi: 10.1007/s00394-020-02299-w
43. Aune D, Giovannucci E, Boffetta P, Fadnes LT, Keum N, Norat T, et al. Fruit and vegetable intake and the risk of cardiovascular disease, total cancer and all-cause mortality—a systematic review and dose-response meta-analysis of prospective studies. Int J Epidemiol. (2017) 46:1029–56. doi: 10.1093/ije/dyw319
44. Bondonno NP, Lewis JR, Prince RL, Lim WH, Wong G, Schousboe JT, et al. Fruit intake and abdominal aortic calcification in elderly women: a prospective cohort study. Nutrients. (2016) 8:159. doi: 10.3390/nu8030159
45. Hyun TK, Jang K-I. Apple as a source of dietary phytonutrients: an update on the potential health benefits of apple. EXCLI J. (2016) 15:565–9. doi: 10.17179/excli2016-483
46. Boyer J, Liu RH. Apple phytochemicals and their health benefits. Nutr J. (2004) 3:5. doi: 10.1186/1475-2891-3-5
47. Feldman F, Koudoufio M, Desjardins Y, Spahis S, Delvin E, Levy E. Efficacy of polyphenols in the management of dyslipidemia: a focus on clinical studies. Nutrients. (2021) 13:672. doi: 10.3390/nu13020672
48. Sandoval-Ramírez BA, Catalán Ú, Calderón-Pérez L, Companys J, Pla-Pagà L, Ludwig IA, et al. The effects and associations of whole-apple intake on diverse cardiovascular risk factors. A narrative review. Crit Rev Food Sci Nutr. (2020) 60:3862–75. doi: 10.1080/10408398.2019.1709801
49. Yang L, Ling W, Du Z, Chen Y, Li D, Deng S, et al. Effects of anthocyanins on cardiometabolic health: a systematic review and meta-analysis of randomized controlled trials. Adv Nutr. (2017) 8:684–93. doi: 10.3945/an.116.014852
50. Dower JI, Geleijnse JM, Hollman PC, Soedamah-Muthu SS, Kromhout D. Dietary epicatechin intake and 25-y risk of cardiovascular mortality: the zutphen elderly study. Am J Clin Nutr. (2016) 104:58–64. doi: 10.3945/ajcn.115.128819
51. do Rosario VA, Schoenaker DAJM, Kent K, Weston-Green K, Charlton K. Association between flavonoid intake and risk of hypertension in two cohorts of Australian women: a longitudinal study. Eur J Nutr. (2020) 60:2507–19. doi: 10.1007/s00394-020-02424-9
52. Castro-Acosta ML, Stone SG, Mok JE, Mhajan RK, Fu CI, Lenihan-Geels GN, et al. Acute effects of apple and blackcurrant polyphenol-rich extracts on postprandial glycaemia and vascular function in healthy men and women. Proc Nutr Soc. (2016) 75:E45.
53. Prpa EJ, Corpe CP, Atkinson B, Blackstone B, Leftley ES, Parekh P, et al. Apple polyphenol-rich drinks dose-dependently decrease early-phase postprandial glucose concentrations following a high-carbohydrate meal: a randomized controlled trial in healthy adults and in vitro studies. J Nutr Biochem. (2020) 85:108466. doi: 10.1016/j.jnutbio.2020.108466
54. Castro-Acosta ML, Stone SG, Mok JE, Mhajan RK, Fu C-I, Lenihan-Geels GN, et al. Apple and blackcurrant polyphenol-rich drinks decrease postprandial glucose, insulin and incretin response to a high-carbohydrate meal in healthy men and women. J Nutr Biochem. (2017) 49:53–62. doi: 10.1016/j.jnutbio.2017.07.013
55. Manzano M, Giron MD, Vilchez JD, Sevillano N, El-Azem N, Rueda R, et al. Apple polyphenol extract improves insulin sensitivity in vitro and in vivo in animal models of insulin resistance. Nutr Metab (Lond). (2016) 13:32. doi: 10.1186/s12986-016-0088-8
56. Sansone K, Kern M, Hong MY, Liu C, Hooshmand S. Acute effects of dried apple consumption on metabolic and cognitive responses in healthy individuals. J Med Food. (2018) 21:1158–64. doi: 10.1089/jmf.2017.0152
57. Henderson JR. Serum-insulin or plasma-insulin ? Lancet. (1970) 296:545–7. doi: 10.1016/s0140-6736(70)91348-6
58. Bondonno CP, Yang X, Croft KD, Considine MJ, Ward NC, Rich L, et al. Flavonoid-rich apples and nitrate-rich spinach augment nitric oxide status and improve endothelial function in healthy men and women: a randomized controlled trial. Free Radic Biol Med. (2012) 52:95–102. doi: 10.1016/j.freeradbiomed.2011.09.028
Keywords: placebo-controlled study, metabolic syndrome, cardiovascular disease, apple polyphenol, apple pectin, blood marker, lipid
Citation: Kim SJ, Anh NH, Jung CW, Long NP, Park S, Cho YH, Yoon YC, Lee EG, Kim M, Son EY, Kim TH, Deng Y, Lim J and Kwon SW (2022) Metabolic and Cardiovascular Benefits of Apple and Apple-Derived Products: A Systematic Review and Meta-Analysis of Randomized Controlled Trials. Front. Nutr. 9:766155. doi: 10.3389/fnut.2022.766155
Received: 28 August 2021; Accepted: 07 March 2022;
Published: 05 April 2022.
Edited by:
Jasminka Z. Ilich, Florida State University, United StatesReviewed by:
Federica Fogacci, University of Bologna, ItalyNicola Bondonno, Danish Cancer Society, Denmark
Copyright © 2022 Kim, Anh, Jung, Long, Park, Cho, Yoon, Lee, Kim, Son, Kim, Deng, Lim and Kwon. This is an open-access article distributed under the terms of the Creative Commons Attribution License (CC BY). The use, distribution or reproduction in other forums is permitted, provided the original author(s) and the copyright owner(s) are credited and that the original publication in this journal is cited, in accordance with accepted academic practice. No use, distribution or reproduction is permitted which does not comply with these terms.
*Correspondence: Sung Won Kwon, c3drd29uQHNudS5hYy5rcg==
†These authors have contributed equally to this work and share first authorship