Genetically-Guided Medical Nutrition Therapy in Type 2 Diabetes Mellitus and Pre-diabetes: A Series of n-of-1 Superiority Trials
- 1Department of Biology, School of Medicine, National and Kapodistrian University of Athens, Athens, Greece
- 2Embiodiagnostics Biology Research Company, Heraklion, Greece
- 3Unit of Reproductive Endocrinology, First Department of Obstetrics and Gynecology, Faculty of Health Sciences, Medical School, Aristotle University of Thessaloniki, Thessaloniki, Greece
- 4Department of Nutritional Sciences and Dietetics, Faculty of Health Sciences, International Hellenic University, Thessaloniki, Greece
- 5Clinical Genomics and Pharmacogenomics Unit, Fourth Department of Internal Medicine, School of Medicine, National and Kapodistrian University of Athens, Athens, Greece
- 6Center of Basic Research, Biomedical Research Foundation of the Academy of Athens, Athens, Greece
Type 2 diabetes mellitus (T2DM) is a heterogeneous metabolic disorder of multifactorial etiology that includes genetic and dietary influences. By addressing the latter, medical nutrition therapy (MNT) contributes to the management of T2DM or pre-diabetes toward achieving glycaemic control and improved insulin sensitivity. However, the clinical outcomes of MNT vary and may further benefit from personalized nutritional plans that take into consideration genetic variations associated with individual responses to macronutrients. The aim of the present series of n-of-1 trials was to assess the effects of genetically-guided vs. conventional MNT on patients with pre-diabetes or T2DM. A quasi-experimental, cross-over design was adopted in three Caucasian adult men with either diagnosis. Complete diet, bioclinical and anthropometric assessment was performed and a conventional MNT, based on the clinical practice guidelines was applied for 8 weeks. After a week of “wash-out,” a precision MNT was prescribed for an additional 8-week period, based on the genetic characteristics of each patient. Outcomes of interest included changes in body weight (BW), fasting plasma glucose (FPG), and blood pressure (BP). Collectively, the trials indicated improvements in BW, FPG, BP, and glycosylated hemoglobin (HbA1c) following the genetically-guided precision MNT intervention. Moreover, both patients with pre-diabetes experienced remission of the condition. We conclude that improved BW loss and glycemic control can be achieved in patients with pre-diabetes/T2DM, by coupling MNT to their genetic makeup, guiding optimal diet, macronutrient composition, exercise and oral nutrient supplementation in a personalized manner.
Introduction
The prevalence and incidence of diabetes mellitus (DM) is increasing due to lifestyle shifts toward western-type diets and sedentary behaviors. There is, however, considerable heterogeneity in the association of dietary intake with the risk for developing type 2 diabetes mellitus (T2DM) and glycemic traits, which has been attributed to human genetic variation. Indeed, studies in twins have indicated a major contribution of genetics to the development of T2DM, which is comparable to the impact of diet (1). Genome-wide association studies (GWAS) have identified more than 140 genomic loci linked to increased T2DM predisposition (2) and individual meal responses (3). Several of these variants have been explored in combination to formulate genetic risk scores (GRS) to better predict the relative risk of T2DM development (4).
Medical nutrition therapy (MNT) is endorsed by both the Academy of Nutrition and Dietetics and the American Diabetes Association (ADA) for the management of adults with T2DM or pre-diabetes toward achieving euglycemia (5, 6). However, specific recommendations for the proportion of macronutrients, or oral nutrient supplementation (ONS) guidelines for the MNT of T2DM are lacking (6). As an example, the Obesity Society recommends a carbohydrate (CHO) intake between 45–65% of the energy intake (EI), 15–35% from protein, and 30% from total fat [10% from polyunsaturated fatty acid (PUFA), 15–20% from monounsaturated fatty acids (MUFA), 7% from saturated fatty acids (SFA)] for the management of T2DM (7). On the other hand, the Mediterranean diet, which confers significant benefits to T2DM prevention, is considered a high-fat diet (>40% of the EI from total fat), rich in MUFA but poor in SFA (8–10). This ambiguity in the MNT recommendations for patients with T2DM likely reflects the need to provide dietary and lifestyle advice personalized to the patient's bioclinical, phenotypic, lifestyle, and genetic data, thus maximizing its impact (11).
Several lines of clinical and experimental evidence call for a major conceptual and practical shift in dietetics toward the provision of precision nutrition care, parallel to the progress in precision medicine. Genetic variation is an important source of nutrition-related metabolic heterogeneity (12). Thus far, many observational studies have reported associations between specific genetic variants, predominantly single nucleotide polymorphisms (SNPs), and the health outcomes to the provision of specific macro- and micro-nutrients and ONS, including T2DM (11, 13, 14). However, how this information could be combined to provide dietary and lifestyle recommendations in T2DM remains poorly explored.
Since Hogben and Sim first conceived n-of-1 trials as components of pragmatic clinical practice in the 1950s (15), their application has gained residence. Today, the n-of-1 trial design represents the cornerstone of precision medicine and nutrition, offering insight on the individual patient response without requiring post-hoc analyses (16–19). The implementation of n-of-1 trials is used to compare interventions and find the most effective one for each patient, increasing treatment-response, reducing polypharmacy, minimizing adverse events, while conserving health care resources (20).
In recent years, nutrigenetic investigations have aided the delivery of MNT interventions in large-scale dietary trials, with the PREDIMED, the Look AHEAD and the POUNDS Lost clinical trials being notable examples. These trials reported milestone findings for the association of single SNPs with the outcome of MNT, including the interaction of a TCF7L2 variant with a Mediterranean diet (MD) pattern for glycemic traits (21) and the interaction of a MTNR1b variant with dietary fat intake for glycemic traits (22–24). They have also identified associations between GRS for diabetes, and protein intake influencing insulin resistance (25). However, despite promising results, research and validations in different populations are still required. The conduction of nutritional n-of-1 trials may represent an advantageous method for gene-nutrition and diabetes-nutrition related research. Determination of the optimal personalized nutritional strategies, based on such trials is an essential step toward the delivery of precision prevention in the treatment of diabetes in the near future.
The present case series of n-of-1 cross-over superiority trials aimed to assess the effectiveness of precision, genetically guided MNT interventions vs. conventional MNT, in patients with pre-diabetes or T2DM.
Materials and Methods
Research Question, Trial Protocol, and Registration
The present series of n-of-1 cross-over, superiority trials is presented based on the standard protocol items recommendations for interventional trials (SPIRIT) extension for n-of-1 trial protocols (SPENT) statements (26). The protocol was registered at the Center for Open Sciences Framework (OSF) (https://bit.ly/3hmz3FJ). The research question based on the PICO acronym is detailed in Supplementary Table 1.
Participants and Study Design
A quasi-experimental design was applied in three patients with pre-diabetes or T2DM (Supplementary Figure 1). A quasi-experimental design lacks patient randomization, and treatment allocation is dictated by the researchers. This design is the most appropriate one for a personalized nutrition intervention in which patients act as their own controls. Individuals provided consent to receiving personalized MNT based on their genetic profile, as well as for the anonymous publication of their data.
Ethical Approval
Ethical approval for the study was obtained by the Bioethics Committee of the National and Kapodistrian University of Athens Medical School (1718034127/03-07-2018). Each participant provided consent prior to participation and additional consent for the collection and analysis of biological specimens. A detailed description of the methods is provided as Supplementary Material.
Results
Case 1
Patient Characteristics
A 45-year-old male patient with obesity, symptoms of hyperglycaemia (nocturia), suffering from night eating syndrome and fasting plasma glucose (FPG) 112 mg/dL reported involuntary body weight (BW) gain during the past 5 years despite exercising twice weekly, and difficulty in controlling BW and appetite since adolescence (Table 1). The patient also consumed branched-chain amino acid (BCAA) supplements, aiming at decreasing muscle soreness during resistance exercise sessions. The patient's main objectives were to reduce BW and maintain euglycemia.
Conventional Dietary Intervention
A diet high in fiber and carbohydrates and low in protein was initially prescribed by a registered dietician, divided into three meals and an equal number of snacks, aiming to reduce postprandial glucose peaks and prolonged fasting intervals (Table 2) (6). He was also prescribed vitamin D ONS to improve low serum vitamin D levels. Adherence to this dietary plan for 8 weeks reduced BW by 3 kg (2.9%) without altering his weight status. No change was noted in the HbA1c levels and a marginal improvement in the FPG (106 mg/dL) and systolic blood pressure (BP) was achieved.
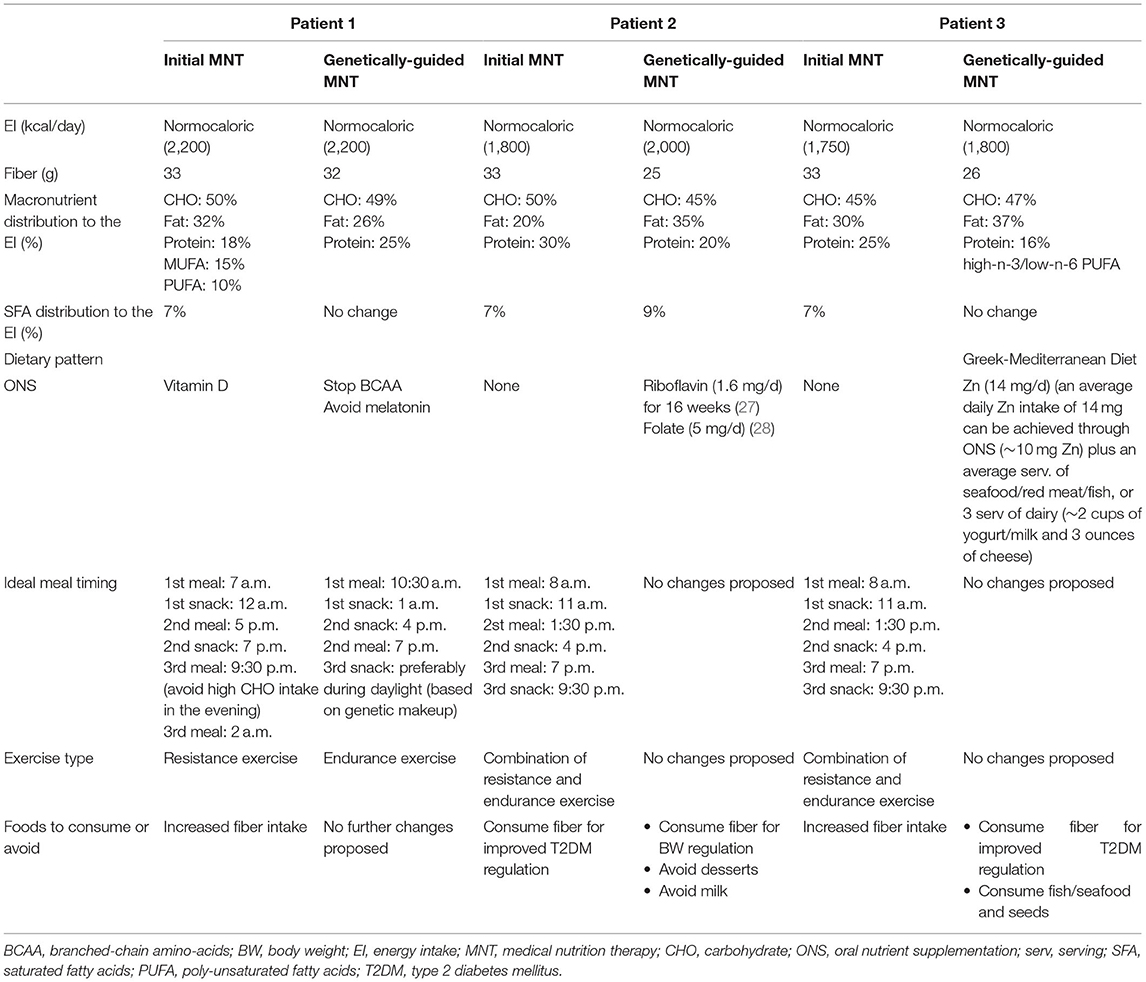
Table 2. Initial MNT prescribed to each case, and genetically-guided MNT prescribed post-nutrigenetic testing.
Personalized Lifestyle Intervention
A new dietary intervention was initiated in our Unit (Table 2), based on the results of a nutrigenetic test (11) (Table 3). The most relevant genetic information extracted from this analysis included: (i) a high GRS for T2DM, indicating that the patient could particularly benefit from a high-protein diet in improving insulin resistance (IR) and β-cell function (25); (ii) a high GRS for habitual coffee consumption which is linked to improved glycaemic responses to a low-fat diet (29), and (iii) a high GRS for FPG, associated with improved glucose metabolism when consuming low-fat diets.
Informative SNPs for macronutrient intake included DHCR7 rs12785878, PPM1K rs1440581, and MTNR1B rs1387153, which further pointed to the putative beneficial effects of a low-calorie, low-fat and low-carbohydrate diet (11). The MTNR1B rs10830963 GG genotype (which is in linkage disequilibrium with rs1387153 in Greeks) was used to implement lifestyle and food-timing changes, as G homozygotes exhibit higher early melatonin onset during the evening, a longer duration of elevated melatonin concentrations and a delayed melatonin decline in the morning (31), the latter being associated with an increased risk for DM upon early waking up (32). Furthermore, the concurrence of meal timing with elevated endogenous melatonin is associated with impaired glucose tolerance in GG homozygotes (33). As a result, people carrying this variant display worse glucose tolerance when consuming dinner late when melatonin levels are high (34). Moreover, because of the prolonged duration of elevated melatonin levels, risk allele carriers may show a slower decline in melatonin concentrations during the morning hours; thus, delaying breakfast might reduce the risk of developing T2DM (35). These observations underpin opportunities for behavioral changes regarding the timing of food intake and hence specific recommendations for the optimal timing of breakfast and dinner were provided to the patient.
The patient was advised to avoid melatonin supplementation as prescription of melatonin to rs10830963 GG carriers is not linked to beneficial health outcomes (35, 36) (Table 3). In the absence of genetic information precluding vitamin D ONS, the patient continued its use. Considering that individuals genetically prone to obesity appear to benefit less from resistance exercise (37) and CC homozygotes for SLC30A8 rs13266634 are more susceptible to soreness during resistance training (38), the patient was advised to replace resistance exercise with endurance training. Adherence to this diet and exercise intervention for 8 weeks achieved a 7.5 kg BW loss (7.4%) and a notable improvement in FPG levels (89 mg/dL; Table 4). Regarding metabolic control, improvements were noted in both the HbA1c and FPG concentrations, as well as in all lipid parameters. Moreover, the reduction in BW resulted in remission of pre-diabetes (39, 40). Diastolic BP was marginally improved following the genetically-guided MNT interventions.
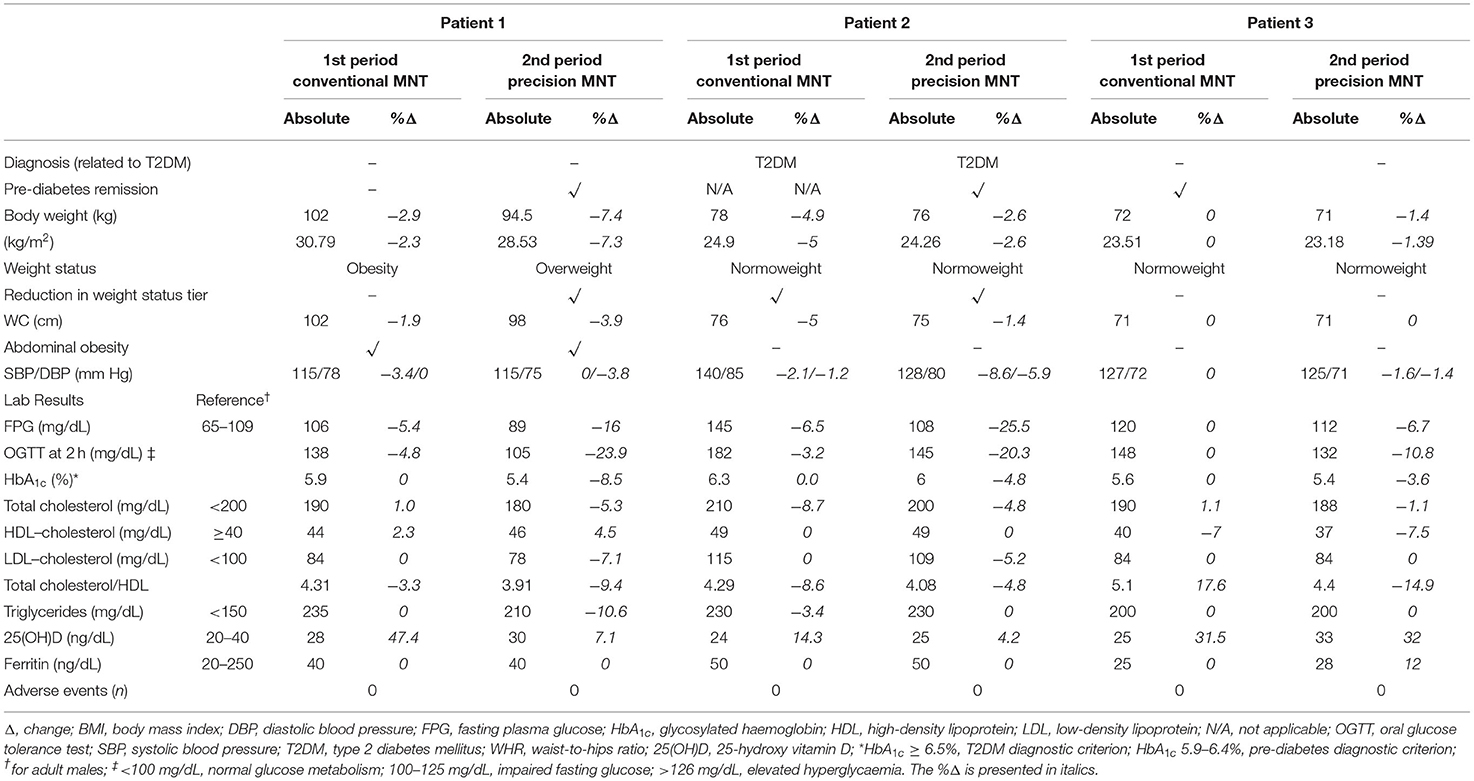
Table 4. End-of-treatment results and % of change for each endpoint and cases after implementation of the conventional (1st) and precision (2nd) MNT intervention periods.
Case 2
Patient Characteristics
A 54-year-old male patient with overweight and T2DM (Table 1) aimed at reducing FPG (from 155 mg/dL), BW, serum triglycerides and cholesterol levels, and to control blood pressure through lifestyle changes. The patient reported exercising four times per week and claimed to be very careful with his nutritional choices.
Conventional Dietary Intervention
A normocaloric (2,000 kcal/day), high-fiber diet (30 g/day), with the EI divided in CHO (50%), fats (20%), and proteins (30%), was prescribed in three main meals and an equal number of snacks daily (Table 2). Food timing and exercise regimes were adjusted to his everyday schedule. Following 8 weeks of MNT, BW was reduced by 4 kg (4.9%), altering the weight status of the patient to normoweight. The intervention failed to induce any change in the HbA1c concentrations and resulted in a marginal improvement in the FPG (145 mg/dL) and BP levels (Table 4).
Personalized Lifestyle Intervention
A new dietary intervention pattern was designed based on the patient's genetic profile (Tables 2, 3). The most nutritionally relevant genetic information included: (i) a low GRS for habitual coffee consumption (associated with beneficial health outcomes following a high-fat diet); (ii) the PPM1K rs1440581 TT genotype associated with a reduction in insulin and the β-cell function homeostatic model assessment (HOMA-B) following a high-fat diet (41), (iii) homozygosity of the risk C allele of CRY1 rs2287161, linked with an impaired glycemic control following high-carbohydrate consumption (42); and (iv) homozygosity of the T allele of TCF7L2 rs7903146, associated with a greater risk for T2DM and related comorbidities when consuming diets rich in saturated fatty acids (SFA ≥15.5% of EI) (43) desserts and milk (44).
Concerning ONS, the most informative SNP was MTHFR rs1801133, as administration of riboflavin supplements to TT homozygotes suffering from hypertension can improve blood pressure (BP) more efficiently than state-of-the-art antihypertensive drugs (45). Furthermore, due to the MTHFR homozygosity, the patient was asked to test blood concentrations of homocysteine (46) which were found to be elevated and the patient's dietary plan was supplemented with folate (0.5 mg/day) (28). Based on the genetic information, personalized dietary advice was provided as outlined on Table 2. Following 8 weeks of adhering to this intervention, an additional BW loss of 2 kg was achieved (2.6% in 2 months), coupled with a notable improvement in FPG (108 mg/dL) and BP (−12 mm/Hg) levels (Table 4).
Case 3
Patient Characteristics
A 67-year-old, normal-weight male with T2DM experienced dysregulated FPG levels (122 mg/dL) (Table 1). Moreover, the patient reported experiencing depressive symptoms which were verified by the Beck Depression Inventory (BDI) (47) score of 15.
Conventional Dietary Intervention
A normocaloric (1,750 kcal/day), high-fiber (30 g) diet was initially prescribed (Table 2), divided into three meals and an equal number of snacks, to avoid postprandial glucose peaks and prolonged fasting intervals. ONS with vitamin D was also recommended. After 8 weeks of MNT, no improvements were detected in the BW, BP, HbA1c, or FPG concentrations (Table 4).
Personalized Lifestyle Intervention
A new dietary intervention was designed based on the patient's genetic profile (Table 3). The most informative scores related to macronutrient intake included: (i) low GRS for T2DM, for which low-protein diets have been shown to improve outcomes (25), (ii) low GRS for habitual coffee intake which has been associated with improved health following a high-fat diet (29), and (iii) low GRS for FPG, for which a high-fat diet may improve glucose metabolism (48). This patient was also carrier of the LEPR rs3790433 GG genotype which is associated with reduced risk for hyperinsulinemia and IR upon adherence to high-n-3/low-n-6 polyunsaturated fatty acid (PUFA) diet. The SLC30A8 rs13266634 SNP was informative in relation to zinc supplementation which has been reported to particularly benefit those carrying no risk alleles (49).
No other usable genetic information was recorded with respect to carbohydrate intake, lifestyle changes, and exercise. Based on this genetic data, a high-fat traditional Cretan-Greek MD was prescribed (Table 2) that is enriched in n-3 PUFA (50, 51).
Despite the patient being normoweight from the start of the interventions, after 8 weeks of adhering to this personalized MNT program (Table 4), improvements were noted in the glycaemic profile (FPG 112 mg/dL and HbA1c of 5.4%) resulting in the remission of pre-diabetes. Moreover, a significant improvement was noted in the subjective depressive symptoms reported by the patient, identified by a 10-point reduction in the BDI scale and a small improvement was recorded regarding the BP levels. No adverse events were reported regarding the conventional or precision interventions by either patient.
Discussion
The horizontal approach to the provision of dietary recommendations has been questioned (52) as it often fails to address particularities at the individual patient level. Approximately 70 years ago, Williams (53) was the first to envisage personalized nutritional recommendations that take into account “genetic patterns” and suggested that patients are “far from standardized specimens.” Although this concept remained dormant for several decades, the potential of precision nutrition in health and disease management is nowadays increasingly recognized (11, 13, 14, 54) and corroborated by several observational studies linking dietary outcomes to genetic variants (55–59). Along these lines, results from the Diabetes Prevention Program have suggested that, although a standardized intensive lifestyle intervention reduces DM risk (60), its efficacy varies across individuals (61), and is determined by genetic factors (62).
Conceptually, the series of n-of-1 trials presented herein addresses the reverse. It explores combinations of genetic variants associated with responses to dietary and lifestyle parameters to provide personalized dietary plans for patients with T2DM or pre-diabetes. We applied a quasi-experimental n-of-1 trials design as the most appropriate to address the effectiveness of conventional vs. genotype-based MNT treatment in the same patient. This methodology has been successfully explored in patient-centered medicine and nutrition studies (18, 63, 64), including DM (65), and can form a pragmatic research extension of the standard care.
The results provide proof-of-principle that we can achieve better glycaemic control in T2DM patients by coupling MNT to genetic information as a predictor of optimal macronutrient composition and ONS in a personalized manner. Both patients with pre-diabetes experienced remission of the condition. We particularly note that the successful outcome of these interventions entailed very different macronutrient compositions, ranging from a high-fat traditional Mediterranean dietary pattern to a diet with greater-protein intake. We thus propose that genetically-guided MNT may in fact aid in the resolution of the “ambiguity” regarding the ideal macronutrient composition for the management of DM (6–8). Furthermore, opting for genetically-informed dietary recommendations may enhance patient adherence. For example, informing the second patient of his genetic susceptibility to increased risk for T2DM at high BCAA intake led to voluntary changes in lifestyle choices toward abandoning BCAA ONS that he was unwilling to do otherwise. Thus, adherence to genetically-guided lifestyle recommendations is likely to amplify health outcomes. Indeed, several surveys conclude that individuals are more likely to adhere to dietary recommendations if these are based on their genetic profile (66, 67), while, on the other hand, improving adherence to healthy dietary patterns could attenuate the genetic predisposition for weight gain (57).
Despite the complexity of gene-nutrient interactions, genetic (GRS) or polygenic risk scores (PRS) coupled with mathematical predictive models and machine-learning algorithms are expected to accelerate the translation of genetic information to nutritional recommendations (68) and to have a major impact on the practical applications of nutrigenetics to precision nutrition (13). Results presented herein support this notion and confirm the utility of GRS in guiding nutritional recommendations in T2DM. It could be argued that the GRS used herein have not been adjusted for ancestry and this may indeed be a limitation of our study. Nevertheless, our results confirm the utility of GRS in guiding nutritional recommendations in T2DM in Greek similar to other populations (25, 46).
Other limitations of the present study include the possible carryover effect of the first intervention cycle which was addressed by testing for treatment-by-period interaction, as suggested for nutrition clinical trials (69). We note that a rule of thumb for the ideal duration of the wash-out period in dietary interventions does not exist, with the exception of ONS trials (70). Nevertheless, according to Senn (71), performing analyses for assessing the carryover effect of cross-over trials is not advised and analyzing data according to treatment-by-period interaction is the preferred strategy. In this manner, the Cochrane handbook suggests that although long wash-out periods can avoid the carryover effect, this is not deemed essential (72). Wash-out periods should also take into consideration ethical aspects related to maintenance of MNT in T2DM patients, including the violation of the equipoise principle (73–75). Of note, the importance of a wash-out period separating treatment phases in n-of-1 trials has also been debated (76). According to the DEcIDE methods center N-of-1 guidance Panel (20), often, the benefits of an intervention wash-out quickly, but the risks of adverse events persist.
An additional limitation of the present series may involve the small number of participants. However, the n-of-1 design is unanimously the most appropriate one for the implementation of precision medicine/nutrition interventions in the pragmatic setting (77, 78). As per Mizra and Guyatt (79), for the design to be suitable, the disease course must involve a chronic and relatively stable condition, such as in T2DM. A possible bottleneck in this design may involve the higher probability for a type-I error, however, aggregation of n-of-1 trials appears to outperform traditional RCTs in many methodological domains (80). According to Kravitz et al. (81), the importance of precision medicine stems from the individual heterogeneity in the treatment effect, thus n-of-1 trials represent the most appropriate method to gather information regarding the comparative effectiveness and adverse events of a novel therapy as compared to the conventional treatment, in order to inform pragmatic treatment decisions. In this regard, n-of-1 trials retain promise for the advancement of precision medicine (81) and precision nutrition (14, 63). We argue that the only caveat in relation to the small number of participants included herein might stem from the high individual heterogeneity and the subsequent inability to pool findings to perform group statistical analyses with respect to the two intervention cycles.
Regarding the lack of repetition of the AB intervention phases, the AB quasi-experimental design is considered as the most appropriate in defining the most effective, adverse-event-free therapy for each patient (18, 82). On the other hand, repetitions of the AB phases in a sequential manner is to n-of-1 trials, what sample size is for parallel arm randomized controlled trials (20) and can defend against random error. Nevertheless, the simple quasi-experimental design employed herein allows for the direct comparison of treatments A (conventional MNT) and B (genotype-guided MNT) and protects against several forms of systematic error (including history, testing, regression to the mean) (20, 83).
Finally, an additional limitation involves the lack of recorded data regarding the patients' perspective with respect to the treatments. Future studies based on the proof-of-principle results presented herein will address these issues.
Through these limited in number, yet carefully designed trials and investigated cases, we have herein addressed a major gap in linking the observational nature of gene-diet interactions to an effective step-by-step process required for the implementation of precision nutrition interventions (14, 84). Supplementary Figure 2 outlines the strategy followed in the present case series, based on the nutrition care process (NCP) model that may serve as a guide for the implementation of innovative genotype-directed MNTs in T2DM as well as other polygenic, multifactorial pathologies. Overall, the present series of n-of-1 superiority trials indicates that precision nutrition is both feasible and effective in adults with pre-diabetes and T2DM. Given the worldwide prevalence of T2DM, we anticipate that this approach may assist nutritionists in providing more personalized nutritional recommendations, enabling patient adherence and improved health outcomes.
Data Availability Statement
The raw data supporting the conclusions of this article will be made available by the authors, without undue reservation.
Ethics Statement
The studies involving human participants were reviewed and approved by Bioethics Committee of the National and Kapodistrian University of Athens Medical School. The patients/participants provided their written informed consent to participate in this study. Written informed consent was obtained from the individual(s) for the publication of any potentially identifiable images or data included in this article.
Author Contributions
KG and AE: conceptualization, methodology, and resources. KG: assays. EL and KG: data collection. KG, AE, and MG: data curation. DS, EL, and DG: writing—review and editing. AE: visualization and project administration. All authors have read and agreed to the published version of the manuscript. All authors contributed to the article and approved the submitted version.
Conflict of Interest
Author KG was employed by Embiodiagnostics Biology Research Company.
The remaining authors declare that the research was conducted in the absence of any commercial or financial relationships that could be construed as a potential conflict of interest.
Publisher's Note
All claims expressed in this article are solely those of the authors and do not necessarily represent those of their affiliated organizations, or those of the publisher, the editors and the reviewers. Any product that may be evaluated in this article, or claim that may be made by its manufacturer, is not guaranteed or endorsed by the publisher.
Acknowledgments
The authors acknowledge the cooperation of Margarita Gavala, Theano Goumenaki, Aggelos Klitsas, Tsampika Kontogiannou, Dimitra Kotsarini, George Solidakis, Christina Pavlou, Maria Toumpi, Konstantina Tsichli, and Athanasios Tsioudas.
Supplementary Material
The Supplementary Material for this article can be found online at: https://www.frontiersin.org/articles/10.3389/fnut.2022.772243/full#supplementary-material
References
1. Kaprio J, Tuomilehto J, Koskenvuo M, Romanov K, Reunanen A, Eriksson J, et al. Concordance for type 1 (insulin-dependent) and type 2 (non-insulin-dependent) diabetes mellitus in a population-based cohort of twins in Finland. Diabetologia. (1992) 35:1060–7. doi: 10.1007/BF02221682
2. Xue A, Wu Y, Zhu Z, Zhang F, Kemper KE, Zheng Z, et al. Genome-wide association analyses identify 143 risk variants and putative regulatory mechanisms for type 2 diabetes. Nat Commun. (2018) 9:2941. doi: 10.1038/s41467-018-04951-w
3. Mazidi M, Valdes AM, Ordovas JM, Hall WL, Pujol JC, Wolf J, et al. Meal-induced inflammation: postprandial insights from the Personalised REsponses to DIetary Composition Trial (PREDICT) study in 1000 participants. Am J Clin Nutr. (2021) 114:1028–38. doi: 10.1093/ajcn/nqab132
4. Dietrich S, Jacobs S, Zheng J-S, Meidtner K, Schwingshackl L, Schulze MB. Gene-lifestyle interaction on risk of type 2 diabetes: a systematic review. Obes Rev. (2019) 20:1557–71. doi: 10.1111/obr.12921
5. Morris SF, Wylie-Rosett J. Medical nutrition therapy: a key to diabetes management and prevention. Clin Diabetes. (2010) 28:12–8. doi: 10.2337/diaclin.28.1.12
6. Evert AB, Dennison M, Gardner CD, Garvey WT, Lau KHK, MacLeod J, et al. Nutrition therapy for adults with diabetes or prediabetes: a consensus report. Diab Care. (2019) 42:731–54. doi: 10.2337/dci19-0014
7. Gonzalez-Campoy JM, St Jeor ST, Castorino K, Ebrahim A, Hurley D, Jovanovic L, et al. Clinical practice guidelines for healthy eating for the prevention and treatment of metabolic and endocrine diseases in adults: cosponsored by the American Association of Clinical Endocrinologists/the American College of Endocrinology and the Obesity Society. Endocr Pract. (2013) 19(Suppl 3):1–82. doi: 10.4158/EP13155.ESGL
8. Huo R, Du T, Xu Y, Xu W, Chen X, Sun K, et al. Effects of Mediterranean-style diet on glycemic control, weight loss and cardiovascular risk factors among type 2 diabetes individuals: a meta-analysis. Eur J Clin Nutr. (2015) 69:1200–8. doi: 10.1038/ejcn.2014.243
9. Grammatikopoulou MG, Nigdelis MP, Theodoridis X, Gkiouras K, Tranidou A, Papamitsou T, et al. How fragile are Mediterranean diet interventions? A research-on-research study of randomized controlled trials. BMJ Nutr Prev Heal. (2021) 4:115–31. doi: 10.1136/bmjnph-2020-000188
10. Willett WC, Sacks F, Trichopoulou A, Drescher G, Ferro-Luzzi A, Helsing E, et al. Mediterranean diet pyramid: a cultural model for healthy eating. Am J Clin Nutr. (1995) 61:1402S−6S. doi: 10.1093/ajcn/61.6.1402S
11. Gkouskou K, Lazou E, Skoufas E, Eliopoulos AG. Genetically guided mediterranean diet for the personalized nutritional management of type 2 diabetes mellitus. Nutrients. (2021) 13:355. doi: 10.3390/nu13020355
12. Zeisel SH. A conceptual framework for studying and investing in precision nutrition. Front Genet. (2019) 10:200. doi: 10.3389/fgene.2019.00200
13. Gkouskou K, Vlastos I, Karkalousos P, Chaniotis D, Sanoudou D, Eliopoulos AG. The ‘Virtual Digital Twins' concept in precision nutrition. Adv Nutr. (2020) 11:1405–13. doi: 10.1093/advances/nmaa089
14. Ordovas J, Ferguson L, Tai E, Mathers J. Personalised Nutrition and Health. BMJ. (2018) 361:k2173. doi: 10.1136/bmj.k2173
15. Hogben L, Sim M. The self-controlled and self-recorded clinical trial for low-grade morbidity. Br J Prev Soc Med. (1953) 7:163–79. doi: 10.1136/jech.7.4.163
17. Schork NJ. Personalized medicine: time for one-person trials. Nature. (2015) 520:609–11. doi: 10.1038/520609a
18. Schork NJ, Goetz LH. Single-subject studies in translational nutrition research. Annu Rev Nutr. (2017) 37:395–422. doi: 10.1146/annurev-nutr-071816-064717
19. Zheng J-S, Ordovás JM. Precision nutrition for gut microbiome and diabetes research: application of nutritional n-of-1 clinical trials. J Diabetes. (2021)13:1059–61. doi: 10.1111/1753-0407.13220
20. Kravitz RL, Duan N, Vohra S, Li J, the the DEcIDE Methods Center n-of-1 Guidance Panel. Introduction to n-of-1 trials: indications and barriers. In: Kravitz RL, Duan N, DEcIDE Methods Center N-of-1 Guidance Panel, editors. Design and Implementation of N-of-1 Trials: A User's Guide. Rockville, MD: Agency for Healthcare Research and Quality (US) (2014). p. 1–11.
21. Corella D, Carrasco P, Sorlí J V, Estruch R, Rico-Sanz J, Martínez-González MÁ, et al. Mediterranean diet reduces the adverse effect of the TCF7L2-rs7903146 polymorphism on cardiovascular risk factors and stroke incidence: a randomized controlled trial in a high-cardiovascular-risk population. Diabetes Care. (2013) 36:3803–11. doi: 10.2337/dc13-0955
22. Mirzaei K, Xu M, Qi Q, De Jonge L, Bray GA, Sacks F, et al. Variants in glucose- and circadian rhythm–related genes affect the response of energy expenditure to weight-loss diets: the POUNDS LOST Trial. Am J Clin Nutr. (2014) 99:392–9. doi: 10.3945/ajcn.113.072066
23. Goni L, Sun D, Heianza Y, Wang T, Huang T, Martínez JA, et al. A circadian rhythm-related MTNR1B genetic variant modulates the effect of weight-loss diets on changes in adiposity and body composition: the POUNDS Lost trial. Eur J Nutr. (2019) 58:1381–9. doi: 10.1007/s00394-018-1660-y
24. Goni L, Sun D, Heianza Y, Wang T, Huang T, Cuervo M, et al. Macronutrient-specific effect of the MTNR1B genotype on lipid levels in response to 2 year weight-loss diets. J Lipid Res. (2018) 59:155–61. doi: 10.1194/jlr.P078634
25. Huang T, Ley SH, Zheng Y, Wang T, Bray GA, Sacks FM Qi L. Genetic susceptibility to diabetes and long-term improvement of insulin resistance and β cell function during weight loss: the Preventing Overweight Using Novel Dietary Strategies (POUNDS LOST) trial. Am J Clin Nutr. (2016) 104:198–204. doi: 10.3945/ajcn.115.121186
26. Porcino AJ, Shamseer L, Chan A-W, Kravitz RL, Orkin A, Punja S, et al. SPIRIT extension and elaboration for n-of-1 trials: SPENT 2019 checklist. BMJ. (2020) 368:m122. doi: 10.1136/bmj.m122
27. McNulty H, Strain JJ, Hughes CF, Ward M. Riboflavin, MTHFR genotype and blood pressure: a personalized approach to prevention and treatment of hypertension. Mol Aspects Med. (2017) 53:2–9. doi: 10.1016/j.mam.2016.10.002
28. Lind MV, Lauritzen L, Kristensen M, Ross AB, Eriksen JN. Effect of folate supplementation on insulin sensitivity and type 2 diabetes: a meta-analysis of randomized controlled trials. Am J Clin Nutr. (2019) 109:29–42. doi: 10.1093/ajcn/nqy234
29. Han L, Ma W, Sun D, Heianza Y, Wang T, Zheng Y, et al. Genetic variation of habitual coffee consumption and glycemic changes in response to weight-loss diet intervention: the Preventing Overweight Using Novel Dietary Strategies (POUNDS LOST) trial. Am J Clin Nutr. (2017) 106:1321–6. doi: 10.3945/ajcn.117.156232
30. Ruchat S-M, Rankinen T, Weisnagel SJ, Rice T, Rao DC, Bergman RN, et al. Improvements in glucose homeostasis in response to regular exercise are influenced by the PPARG Pro12Ala variant: results from the HERITAGE Family Study. Diabetologia. (2010) 53:679–89. doi: 10.1007/s00125-009-1630-2
31. Lane JM, Vlasac I, Anderson SG, Kyle SD, Dixon WG, Bechtold DA, et al. Genome-wide association analysis identifies novel loci for chronotype in 100,420 individuals from the UK Biobank. Nat Commun. (2016) 7:10889. doi: 10.1038/ncomms10889
32. Lane JM, Chang A-M, Bjonnes AC, Aeschbach D, Anderson C, Cade BE, et al. Impact of common diabetes risk variant in MTNR1B on sleep, circadian, and melatonin physiology. Diabetes. (2016) 65:1741–51. doi: 10.2337/db15-0999
33. Garaulet M, Qian J, Florez JC, Arendt J, Saxena R, Scheer FAJL. Melatonin effects on glucose metabolism: time to unlock the controversy. Trends Endocrinol Metab. (2020) 31:192–204. doi: 10.1016/j.tem.2019.11.011
34. Lopez-Minguez J, Saxena R, Bandín C, Scheer FA, Garaulet M. Late dinner impairs glucose tolerance in MTNR1B risk allele carriers: a randomized, cross-over study. Clin Nutr. (2018) 37:1133–40. doi: 10.1016/j.clnu.2017.04.003
35. Karamitri A, Jockers R. Melatonin in type 2 diabetes mellitus and obesity. Nat Rev Endocrinol. (2019) 15:105–25. doi: 10.1038/s41574-018-0130-1
36. Tuomi T, Nagorny CLF, Singh P, Bennet H, Yu Q, Alenkvist I, et al. Increased melatonin signaling is a risk factor for type 2 diabetes. Cell Metab. (2016) 23:1067–77. doi: 10.1016/j.cmet.2016.04.009
37. Klimentidis YC, Bea JW, Lohman T, Hsieh P-S, Going S, Chen Z. High genetic risk individuals benefit less from resistance exercise intervention. Int J Obes. (2015) 39:1371–5. doi: 10.1038/ijo.2015.78
38. Sprouse C, Gordish-Dressman H, Orkunoglu-Suer EF, Lipof JS, Moeckel-Cole S, Patel RR, et al. SLC30A8 nonsynonymous variant is associated with recovery following exercise and skeletal muscle size and strength. Diabetes. (2014) 63:363–8. doi: 10.2337/db13-1150
39. McCombie L, Leslie W, Taylor R, Kennon B, Sattar N, Lean MEJ. Beating type 2 diabetes into remission. BMJ. (2017) 358:j4030. doi: 10.1136/bmj.j4030
40. Hallberg SJ, Gershuni VM, Hazbun TL, Athinarayanan SJ. Reversing type 2 diabetes: a narrative review of the evidence. Nutrients. (2019) 11:1–16. doi: 10.3390/nu11040766
41. Goni L, Qi L, Cuervo M, Milagro FI, Saris WH, MacDonald IA, et al. Effect of the interaction between diet composition and the PPM1K genetic variant on insulin resistance and β cell function markers during weight loss: results from the Nutrient Gene Interactions in Human Obesity: implications for dietary guidelines (NUGENOB) randomized trial. Am J Clin Nutr. (2017) 106:902–8. doi: 10.3945/ajcn.117.156281
42. Dashti HS, Smith CE, Lee Y-C, Parnell LD, Lai C-Q, Arnett DK, et al. CRY1 circadian gene variant interacts with carbohydrate intake for insulin resistance in two independent populations: Mediterranean and North American. Chronobiol Int. (2014) 31:660–7. doi: 10.3109/07420528.2014.886587
43. Phillips CM, Goumidi L, Bertrais S, Field MR, McManus R, Hercberg S, et al. Dietary saturated fat, gender and genetic variation at the TCF7L2 locus predict the development of metabolic syndrome. J Nutr Biochem. (2012) 23:239–44. doi: 10.1016/j.jnutbio.2010.11.020
44. Ouhaibi-Djellouli H, Mediene-Benchekor S, Lardjam-Hetraf SA, Hamani-Medjaoui I, Meroufel DN, Boulenouar H, et al. The TCF7L2 rs7903146 polymorphism, dietary intakes and type 2 diabetes risk in an Algerian population. BMC Genet. (2014) 15:134. doi: 10.1186/s12863-014-0134-3
45. Wilson CP, McNulty H, Ward M, Strain JJ, Trouton TG, Hoeft BA, et al. Blood pressure in treated hypertensive individuals with the MTHFR 677TT genotype is responsive to intervention with riboflavin: findings of a targeted randomized trial. Hypertens. (2013) 61:1302–8. doi: 10.1161/HYPERTENSIONAHA.111.01047
46. Clifford AJ, Chen K, McWade L, Rincon G, Kim S-H, Holstege DM, et al. Gender and single nucleotide polymorphisms in MTHFR, BHMT, SPTLC1, CRBP2, CETP, and SCARB1 are significant predictors of plasma homocysteine normalized by RBC folate in healthy adults. J Nutr. (2012) 142:1764–71. doi: 10.3945/jn.112.160333
47. Beck A, Steer R, Brown G. Beck Depression Inventory. 2nd ed. San Antonio, TX: Psychological Corporation (1996). doi: 10.1037/t00742-000
48. Wang T, Huang T, Zheng Y, Rood J, Bray GA, Sacks FM Qi L. Genetic variation of fasting glucose and changes in glycemia in response to 2-year weight-loss diet intervention: the POUNDS LOST trial. Int J Obes. (2016) 40:1164–9. doi: 10.1038/ijo.2016.41
49. Maruthur NM, Mitchell BD. Zinc-rs13266634 and the arrival of diabetes pharmacogenetics: the ‘zinc mystique'. Diabetes. (2014) 63:1463–4. doi: 10.2337/db14-0151
50. Simopoulos AP. The Mediterranean diets: what is so special about the diet of Greece? The scientific evidence. J Nutr. (2001) 131:3065S−73S. doi: 10.1093/jn/131.11.3065S
51. Phillips CM, Goumidi L, Bertrais S, Field MR, Ordovas JM, Cupples LA, et al. Leptin receptor polymorphisms interact with polyunsaturated fatty acids to augment risk of insulin resistance and metabolic syndrome in adults. J Nutr. (2010) 140:238–44. doi: 10.3945/jn.109.115329
52. Martínez-González MA, Kim H-S, Prakash V, Ramos-Lopez O, Zotor F, Martinez JA. Personalised, population and planetary nutrition for precision health. BMJ Nutr Prev Heal. (2021) 4:355–8. doi: 10.1136/bmjnph-2021-000235
53. Williams RJ. Concept of genetotrophic disease. Nutr Rev. (1950) 8:257–60. doi: 10.1111/j.1753-4887.1950.tb02469.x
54. Mullins VA, Bresette W, Johnstone L, Hallmark B, Chilton FH. Genomics in personalized nutrition: can you “eat for your genes?” Nutrients. (2020) 12:1–23. doi: 10.3390/nu12103118
55. Gardner CD, Trepanowski JF, Del Gobbo LC, Hauser ME, Rigdon J, Ioannidis JPA, et al. Effect of low-fat vs low-carbohydrate diet on 12-month weight loss in overweight adults and the association with genotype pattern or insulin secretion: the DIETFITS randomized clinical trial. JAMA. (2018) 319:245: doi: 10.1001/jama.2018.0245
56. Bray GA, Krauss RM, Sacks FM, Qi L. Lessons learned from the POUNDS lost study: genetic, metabolic, and behavioral factors affecting changes in body weight, body composition, and cardiometabolic risk. Curr Obes Rep. (2019) 8:262–83. doi: 10.1007/s13679-019-00353-1
57. Wang T, Heianza Y, Sun D, Huang T, Ma W, Rimm EB, et al. Improving adherence to healthy dietary patterns, genetic risk, and long term weight gain: gene-diet interaction analysis in two prospective cohort studies. BMJ. (2018) 360:j5644. doi: 10.1136/bmj.j5644
58. Qi Q, Chu AY, Kang JH, Huang J, Rose LM, Jensen MK, et al. Fried food consumption, genetic risk, and body mass index: gene-diet interaction analysis in three US cohort studies. BMJ. (2014) 348:g1610. doi: 10.1136/bmj.g1610
59. Bermingham KM, Brennan L, Segurado R, Barron RE, Gibney ER, Ryan MF, et al. Genetic and environmental influences on covariation in reproducible diet–metabolite associations. Am J Clin Nutr. (2021) 113:1232–40. doi: 10.1093/ajcn/nqaa378
60. Knowler WC, Barrett-Connor E, Fowler SE, Hamman RF, Lachin JM, Walker EA, et al. Reduction in the incidence of type 2 diabetes with lifestyle intervention or metformin. N Engl J Med. (2002) 346:393–403. doi: 10.1056/NEJMoa012512
61. Delahanty LM, Pan Q, Jablonski KA, Aroda VR, Watson KE, Bray GA, et al. Effects of weight loss, weight cycling, and weight loss maintenance on diabetes incidence and change in cardiometabolic traits in the Diabetes Prevention Program. Diabetes Care. (2014) 37:2738–45. doi: 10.2337/dc14-0018
62. Papandonatos GD, Pan Q, Pajewski NM, Delahanty LM, Peter I, Erar B, et al. Genetic predisposition to weight loss and regain with lifestyle intervention: analyses from the diabetes prevention program and the look AHEAD randomized controlled trials. Diabetes. (2015) 64:4312–21. doi: 10.2337/db15-0441
63. Tian Y, Ma Y, Fu Y, Zheng J-S. Application of n-of-1 clinical trials in personalized nutrition research: a trial protocol for westlake n-of-1 trials for macronutrient intake (WE-MACNUTR). Curr Dev Nutr. (2020) 4:nzaa143. doi: 10.1093/cdn/nzaa143
64. Soldevila-Domenech N, Boronat A, Langohr K, de la Torre R. N-of-1 clinical trials in nutritional interventions directed at improving cognitive function. Front Nutr. (2019) 6:110. doi: 10.3389/fnut.2019.00110
65. Ma Y, Fu Y, Tian Y, Gou W, Miao Z, Yang M, et al. Individual postprandial glycemic responses to diet in n-of-1 trials: westlake n-of-1 trials for macronutrient intake (WE-MACNUTR). J Nutr. (2021) 151:3158–67. doi: 10.1093/jn/nxab227
66. Nielsen DE, Shih S, El-Sohemy A. Perceptions of genetic testing for personalized nutrition: a randomized trial of DNA-based dietary advice. J Nutrigenet Nutrigenomics. (2014) 7:94–104. doi: 10.1159/000365508
67. Vallée Marcotte B, Cormier H, Garneau V, Robitaille J, Desroches S, Vohl M-C. Current knowledge and interest of French Canadians regarding nutrigenetics. Genes Nutr. (2019) 14:5. doi: 10.1186/s12263-019-0629-7
68. Ramos-Lopez O, Cuervo M, Goni L, Milagro FI, Riezu-Boj JI, Martinez JA. Modeling of an integrative prototype based on genetic, phenotypic, and environmental information for personalized prescription of energy-restricted diets in overweight/obese subjects. Am J Clin Nutr. (2020) 111:459–70. doi: 10.1093/ajcn/nqz286
69. Lichtenstein AH, Petersen K, Barger K, Hansen KE, Anderson CAM, Baer DJ, et al. Perspective: design and conduct of human nutrition randomized controlled trials. Adv Nutr. (2021) 12:4–20. doi: 10.1093/advances/nmaa109
70. Food and Drug Administration. Bioequivalence Testing. Guidance for Industry. Rockville, MD: Food and Drug Administration (2006).
71. Senn S (editor). Cross-Over Trials in Clinical Research. Chichester: John Wiley and Sons Ltd. (2002).
72. Higgins JP, Eldridge S, Li T. Chapter 23: including variants on randomized trials. Cochrane training. In: Higgins J, Thomas J, Chandler J, Cumpston M, Li T, Page M, Welch V, editors. Cochrane Handbook for Systematic Reviews of Interventions. Chichester: Cochrane (2021).
73. Miller FG, Brody H. What makes placebo-controlled trials unethical? Am J Bioeth. (2002) 2:3–9. doi: 10.1162/152651602317533523
74. Weijer C, Shapiro SH, Cranley Glass K, Enkin MW. For and against: clinical equipoise and not the uncertainty principle is the moral underpinning of the randomised controlled trial. BMJ Br Med J. (2000) 321:756–8. doi: 10.1136/bmj.321.7263.756
75. Hey SP, London AJ, Weijer C, Rid A, Miller F. Is the concept of clinical equipoise still relevant to research? BMJ. (2017) 359:j5787. doi: 10.1136/bmj.j5787
76. Matthews JN. Multi-period crossover trials. Stat Methods Med Res. (1994) 3:383–405. doi: 10.1177/096228029400300405
77. Potter T, Vieira R, de Roos B. Perspective: application of n-of-1 methods in personalized nutrition research. Adv Nutr. (2021) 12:579–89. doi: 10.1093/advances/nmaa173
78. Duan N, Kravitz RL, Schmid CH. Single-patient (n-of-1) trials: a pragmatic clinical decision methodology for patient-centered comparative effectiveness research. J Clin Epidemiol. (2013) 66:S21–8. doi: 10.1016/j.jclinepi.2013.04.006
79. Mirza RD, Guyatt GH A. Randomized clinical trial of n-of-1 trials—tribulations of a trial. JAMA Intern Med. (2018) 178:1378–9. doi: 10.1001/jamainternmed.2018.3979
80. Blackston JW, Chapple AG, McGree JM, McDonald S, Nikles J. Comparison of aggregated N-of-1 trials with parallel and crossover randomized controlled trials using simulation studies. Healthcare. (2019) 7:1–13. doi: 10.3390/healthcare7040137
81. Kravitz RL, Sim I, Duan N A. Case for n-of-1 Trials—Reply. JAMA Intern Med. (2019) 179:453–453. doi: 10.1001/jamainternmed.2018.7180
82. Shamseer L, Sampson M, Bukutu C, Schmid CH, Nikles J, Tate R, et al. CONSORT extension for reporting N-of-1 trials (CENT) 2015: explanation and elaboration. BMJ. (2015) 350:h1793–h1793. doi: 10.1136/bmj.h1793
83. Campbell DT, Stanley J. Experimental and Quasi-Experimental Designs for Research. Boston, MA: Houghton Mifflin Company (1966).
Keywords: nutrigenetics, T2DM (type 2 diabetes mellitus), obesity, precision nutrition, diet therapy, genetic risk score (GRS)
Citation: Gkouskou KK, Grammatikopoulou MG, Lazou E, Sanoudou D, Goulis DG and Eliopoulos AG (2022) Genetically-Guided Medical Nutrition Therapy in Type 2 Diabetes Mellitus and Pre-diabetes: A Series of n-of-1 Superiority Trials. Front. Nutr. 9:772243. doi: 10.3389/fnut.2022.772243
Received: 07 September 2021; Accepted: 12 January 2022;
Published: 21 February 2022.
Edited by:
Suresh T. Mathews, Samford University, United StatesReviewed by:
Venkata Saroja Voruganti, University of North Carolina at Chapel Hill, United StatesRamona Suharoschi, University of Agricultural Sciences and Veterinary Medicine of Cluj-Napoca, Romania
Copyright © 2022 Gkouskou, Grammatikopoulou, Lazou, Sanoudou, Goulis and Eliopoulos. This is an open-access article distributed under the terms of the Creative Commons Attribution License (CC BY). The use, distribution or reproduction in other forums is permitted, provided the original author(s) and the copyright owner(s) are credited and that the original publication in this journal is cited, in accordance with accepted academic practice. No use, distribution or reproduction is permitted which does not comply with these terms.
*Correspondence: Aristides G. Eliopoulos, eliopag@med.uoa.gr; Kalliopi K. Gkouskou, gkouskoukal@med.uoa.gr