- 1Department of Biomedical, Biological and Chemical Engineering, University of Missouri, Columbia, MO, United States
- 2Center for Agroforestry, School of Natural Resources, University of Missouri, Columbia, MO, United States
- 3Department of Chemistry, University of Missouri, Columbia, MO, United States
- 4Molecular Imaging and Theranostics Center, University of Missouri, Columbia, MO, United States
- 5Department of Food Technology, Can Tho University, Can Tho, Vietnam
- 6School of Natural Resources, University of Missouri, Columbia, MO, United States
- 7Division of Plant Sciences, Southwest Research Center, University of Missouri, Columbia, MO, United States
- 8Division of Food, Nutrition and Exercise Sciences, University of Missouri, Columbia, MO, United States
This paper aims to quantify the micronutrients in black walnut and address its human health benefits. The metabolic profiling of 11 black walnut cultivars was accomplished using ultra-high-performance liquid chromatography coupled with quadrupole time-of-flight high-resolution mass spectrometer. Results revealed that the highest concentration of vitamin B9 was present in cultivar “Daniel” (avg. relative signal intensity 229.53 × 104 mAU). “Surprise” and “Daniel” cultivars had the highest amount of vitamin B5. However, vitamin A, D3, E, and K showed no significant difference among the cultivars. The vitamin content levels among the cultivars were compared by applying one way ANOVA method with (P < 0.05) significance level. Mineral analysis for the black walnut kernel, Persian walnut, and black walnut protein powder was done using Inductively Coupled Plasma Optical Emission spectroscopy. The experimental data for black walnut kernel is 0.04 mg/g for Fe and 0.03 mg/g for Zn, and for black walnut, protein powder is 0.07 mg/g for Fe and 0.07 mg/g for Zn. The amino acid analysis and comparison with black walnut kernel show that black walnut flour and protein powder have a higher amount of essential and non-essential amino acids. Therefore, researchers, food process engineers, and food product developers should consider the health benefits of black walnuts and explore the commercial potential of this native agroforestry crop.
Introduction
Black walnut (Juglans nigra L.) belongs to the family Juglandaceae and is indigenous to the Eastern and Midwestern United States (1). It is also known as Eastern black walnut or American walnut. It's the second-most-produced walnut species in the U.S., with Missouri leading the way (2). Because the wood is beautifully colored, sturdy, and easy to work with, black walnut is one of the most valuable North American hardwood timber species commercially and economically. The Persian walnut, sometimes known as the common or English walnut, is a closely related species (Juglans regia L.) indigenous to Central Asia and widely produced in most European countries.
The black walnut tree is a large, fast-growing tree that may attain 30–38 meters in height and 76–120 centimeters in diameter, and individual trees can live 100 years or more (3). The fruit consists of the pericarp or hull (fleshy outer cover), a very hard endocarp (shell), and the kernel (cotyledons and embryo) (Figure 1); the latter is commonly eaten raw or cooked. The shell of the black walnut is processed for use in a variety of industrial applications (4). The kernel is sweet, oily, and high in protein (4). Black walnuts are distinguished from other nut species by their distinct, robust flavor, incredible culinary diversity, and impressive health advantages. They are ideal ingredients for consumers concerned with food additives because they are all-natural product (5). Black walnut is one of the most beneficial and fully utilized native trees grown in natural forests in the United States.
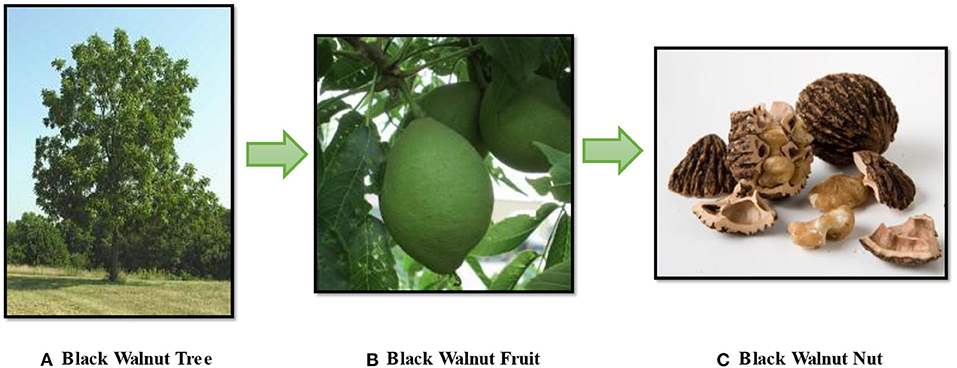
Figure 1. Black Walnut (A) Tree, (B) Fruit, (C) Nut (Photo Courtesy: Hammons Products Company, Stockton, Missouri).
While the health benefits of Persian walnuts, especially their vitamin and mineral profiles, have been well-documented (6), the indigenous black walnut has received little sporadic attention. Walnuts are known to be high in vitamin E and antioxidants (tocopherol) content, but black walnut contains a significantly greater amount of α-tocopherol and γ-tocopherol (a prevailing isomer) compared with Persian walnut (7, 8). A recent study by Zheng et al. (9) suggest that if the relationship and differences of different chemical components of walnuts are determined efficiently then it can be further applied in food industry (9). Although there is no significant research on the association of black walnut consumption with the health benefits of α-tocopherol and γ-tocopherol, recent studies have reported that these molecules are associated with preventing cancer and cardiovascular diseases (10).
Black and Persian walnuts have differing fatty acid, antioxidant, and amino acid profiles, according to USDA food and nutrition database statistics (8). According to recent studies, black walnuts contain protein, minerals, flavonoids, gallotannins, fatty acids, phytosterols, and phenolic compounds (2, 8, 11) although compared to Persian walnut, black walnut contains the same amount of phenolic compounds (12). The phenolic compounds available in black walnuts and tocopherol increase the antioxidant potential of this nut (2). The primary phenolic compounds available in black walnuts are tannins (13, 14), ellagic acid, gallic acid, ellagitannins, and hydrocinnamic acid. Compared with their commercially produced counterpart, Persian walnuts, the nutrient profile of black walnuts is promising. Black walnuts contain 24.06 g of protein per 100 g of kernels, which is ~15% of energy and substantially greater than Persian walnut (2). In addition, black walnuts are dense with dietary fiber, monounsaturated fatty acid (MUFA), and polyunsaturated fatty acid (PUFA), which is more than many other nuts (15). The PUFA (Linolenic acid and α- Linolenic acid) make up more than half of the total lipid content, which is significant given that these fatty acids are not produced in humans (16).
Black walnuts are a significant source of bioactive compounds with antioxidant and anti-inflammatory properties (17–19). Moreover, several black walnut cultivars possess antibacterial properties, which were identified through metabolomics analysis combined with bioassay-guided purification (11). The phenolic acids available in black walnuts, such as ferulic, p-coumaric, p-hydroxybenzoic, syringic, and vanillic acids, are beneficial to human health for their bioactivities (20). Syringic and vanillic acids found in Persian and black walnut have been reported to contribute to antioxidant activities (21, 22). Forty-nine phenolic compounds, which are well-known for their health benefits, were identified in black walnut kernels, with the types and levels of phenols differing significantly among cultivars studied (21).
This present study evaluates the concentration of vitamins among 11 black walnut cultivars using UHPLC coupled with QTOF high-resolution mass spectrometer. Liquid chromatography-tandem mass spectrometry (LC-MS/MS) analysis detected differences in these compounds' occurrence among 11 cultivars. The data from vitamin contents among the 11 cultivars were analyzed using ANOVA, and the difference between the means was analyzed using Tukey's honestly significance test (HSD). The amino acid profile of black walnut flour and black walnut protein powder were also determined. The mineral analysis of black walnut kernel and black walnut protein powder was evaluated following Schuster's 1988 method, and the data found is promising. Knowing the nutritional characteristics of various black walnut cultivars can provide more practical guidelines for health benefits and application in the food industry.
Materials and Methods
Black Walnut Collection
Samples of nuts from 11 black walnut cultivars were collected at the University of Missouri Horticulture and Agroforestry Research Center (39° 0'55 “N 92°45'5” W, New Franklin, MO, USA): Daniel, Davidson, Hay, Jackson, Kwik-Krop, Mystery, Shessler, Sparks 147, Sparrow, Surprise, and Tomboy. The black walnut trees were planted on soils that were well-drained and had a pH (6.5–7.2) that was close to neutral (23), and were well-managed for nut production with typical practices (e.g., pruning, fertilization, weed control, and pest and disease management) (24). The nut samples were collected in November 2017 upon maturity. They were mechanically de-hulled, dried at 24°C for 15 days, and then stored at −20°C until analysis.
Additionally, bulk, commercially produced black walnut kernels, flour, and protein powder samples were obtained from Hammons Products Co. (Stockton, MO) and stored in a freezer (−20°C). The black walnut protein powder is made from defatted black walnut kernels ground to a fine texture. Black walnut flour is made from unprocessed black walnut kernels ground to a fine texture. Persian walnut kernels from Natural Shelled Walnuts (California Walnuts, CA, USA) were purchased from the local market of Columbia, Missouri, USA.
Sample Preparation
The 11 black walnut cultivar samples were prepared by extracting with methanol, as described in Ho et al. (11). First, the hulled nuts were gently split to extract the kernels from the shell. The kernels were then frozen at −20°C before being blended with a coffee grinder (Black + Decker, Beachwood, OH, USA, CBG100S). As described earlier, the kernels (10 g, 20–30 mesh) from each cultivar were recovered in 60 ml methanol for metabolomics analysis (11). The methanolic extract was sonicated for 60 min in a water bath (4°C), then centrifuged for 10 min at 8,000 rpm. The resultant supernatant was filtered via a syringe membrane filter (0.2 m, Whatman Anotop, Sigma-Aldrich, St. Louis, MO, USA) before being fed into high-resolution mass spectrometry using ultra-high-performance liquid chromatography (UHPLC-HRMS). Each cultivar was examined three times, with a methanol blank serving as a control.
UHPLC-QTOF-MS Analysis
To measure the concentration of the major vitamins, UHPLC was used in conjunction with a maXis impact quadrupole time-of-flight (QTOF) high-resolution mass spectrometer (Bruker Co., Billerica, MA, USA) operating in both negative and positive electrospray ionization (ESI) modes with the nebulization gas pressure at 43.5 psi to analyze kernel extracts (2 l per injection) derived from 11 black walnut cultivars (11). Waters Acquit UHPLC ethylene bridged hybrid (BEH) C18 column (2.1 × 150 mm, 1.7 m particle size) maintained at 60°C was used to separate the black walnut samples. A mobile phase of 0.1% formic acid in water (A) and 100% acetonitrile was used (B). The gradient elution process began with a linear gradient of 95%: 5–30% eluents A: B in 30 min, followed by a linear wash gradient of 70–95% B in 30–33 min, 95% B in 33–35 min, 95–5% B in 35–36 min, and 5% B in 37–40 min at a flow rate of 0.56 ml/min. After data capture, mass spectrum data from 100 to 1,500 m/z were automatically gathered, three precursors were selected for auto MS/MS, and the m/z range was auto calibrated using sodium format.
Data Processing
The UHPLC-MS data were analyzed based on the procedure described by Ho et al. (25) with some modifications. The LC-MS data obtained from UHPLC analysis were converted to NetCDF format files and then were processed by XCMS online platform (25)1. Multigroup analysis was performed with parameters optimized for UHPLC data as follows: a centWave method was used for feature detection (1 m/z = 10 ppm, minimum peak width = 5 s, and maximum peak width = 20 s); an obiwarp mode was selected for retention time correction (profStep = 1); chromatogram alignment was set as minfrac = 0.5, bw = 5, mzwid = 0.015, max = 100, minsamp = 1; adducts were optimized for UPLC/Bruker Q-TOF in both ESI(C) and ESI(–); and plant was selected for sample biosource for identification. Metabolites of significant features (intensity > 10,000) were selected for the annotation. These metabolites, with the accurate mass of the molecular ions (m/z values) that match with the m/z values of vitamin, referenced to METLIN metabolite2 mass spectral database containing over 1 million molecules, were used to determine vitamin metabolic profiles in each cultivar (26).
Statistical Analysis
Standard Microsoft Excel TM spreadsheet was used to determine whether the vitamin levels among the 11 cultivars were statistically different from each other. Data were reported as mean ± standard deviation (S.D.), and the average intensity values of the vitamins among cultivars were compared by applying a one-way ANOVA test with a significance level of P < 0.05. The differences between means were determined using Tukey's honestly significant difference test using JMP 14.0 software: SAS Institute Inc., Cary, NC.
Mineral Analysis Using ICP-OES
Black walnut kernel and Persian walnut kernel were analyzed for minerals using Inductively Coupled Plasma Optical Emission spectroscopy (ICP-OES). ICP-OES is a widely used multielement technique with a large linear dynamic range, excellent analytical sensitivity, and high sample throughput (27, 28). The model Varian Vista-MPX CCD simultaneous ICP-OES was used for this analysis. Microwave digestion was used to prepare the kernel samples for total metal analysis of Zn, Fe, Mn, Cu, K, Ca, Mg and P. For 0.5 g of sample, 10 μl concentrated HNO3 was added. A preloaded method for the MARS microwave digestion system (CEM Corporation, USA) was used to digest the sample. Once cooled, the solution was diluted to 50 ml using deionized water (DI water). To determine total nitrogen (N), the combustion method was used by the Elementar CNS. The mineral analysis was conducted at the Soil and Plant Testing Laboratory, Columbia, University of Missouri. The mineral analysis of black walnut protein powder was performed by Eurofins Microbiology Laboratories (Des Moines, Iowa) (Lab-2), where sample preparation and analysis were conducted following AOAC 984.27, 927.02, 985.01, 965.17 mod, respectively (29).
Amino Acid Analysis
The amino acid data of black walnut kernel and black walnut protein powder were collected from two companies, both using HPLC: Intertek Agricultural Services, New Orleans, LA (Lab-1), and Eurofins Microbiology Laboratories (Des Moines, Iowa) (Lab-2). All the samples were prepared, and values were determined using TAALC_S described by 28 except for tryptophan which was determined using the TRPLC_S method (30). The amino acid composition was reported as mg amino acid per g of protein.
Results and Discussion
Macronutrient Profile of Black Walnut
Vitamins
Among all the vitamins detected in the 11 black walnut cultivars shown in Figures 2, 3 (refer Supplementary Table S1), all cultivars had numerically the largest amount of vitamin B5. Vitamin B5, also known as pantothenic acid, is a necessary component for human health. Pantothenic acid deficiency can cause numbness and burning feeling in the hand and feet, a decrease in antibody production, insomnia, and fatigue (31).
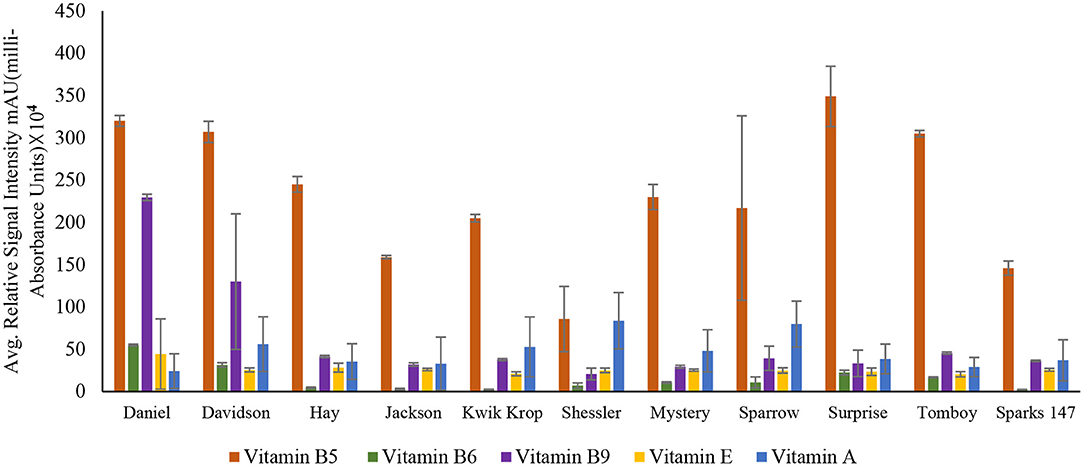
Figure 2. Concentration of vitamin B5 (Pantothenic acid), vitamin B6 (Pyridoxine), vitamin B9 (Folic acid), vitamin E (Tocopherol), and vitamin A (Retinol) in 11 Black Walnut cultivars.
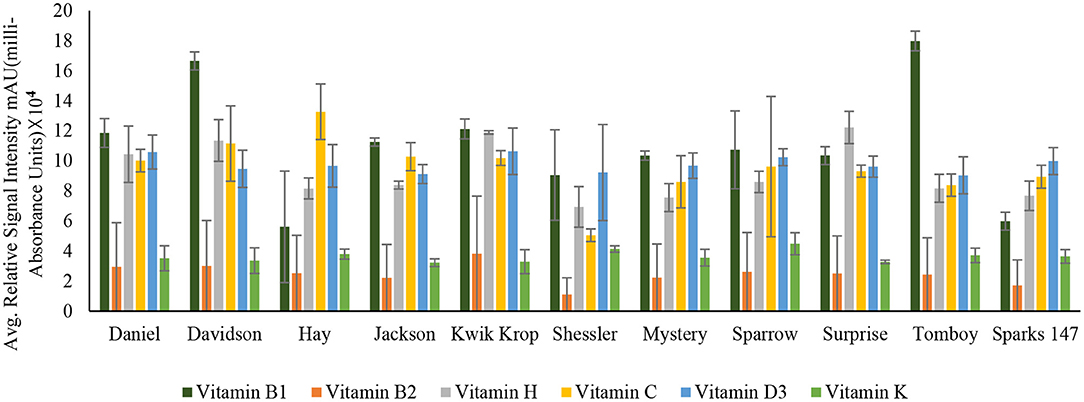
Figure 3. Concentration of Vitamin B1 (Thiamin), Vitamin B2 (Riboflavin), Vitamin H (Biotin), Vitamin C (Ascorbic acid), Vitamin D3 (Cholecalciferol) and Vitamin K (Phylloquinone) in 11 black walnut cultivars.
The results indicated that for vitamins B1, B2, B5, B6, B9, H, and C, there were significant differences (P < 0.05) in vitamin content among the cultivars, likely due to genetic variation. Vitamin B1 (thiamin) and B2 (riboflavin) are essential nutrients for the body's correct blood function (32) and immune system, as well as good skin and hair (33). Other vitamins, such as pyridoxine and folate, require B2 for their metabolism (33, 34). The average amount of Vitamin H and C found in all 11 cultivars was 9.2 ± 0.94 and 9.5 ± 1.39, respectively. Vitamin H (biotin) is mainly required for protein and keratin synthesis, thereby playing an essential role in maintaining healthy hair and nail growth in the human body (35). Compared to Persian walnut, Black walnuts are higher in Vitamin C concentration (8). Vitamin C, commonly known as ascorbic acid, is an antioxidant required to produce certain amino acids. It also acts as a skin barrier and has anti-aging properties (36).
For vitamins B6 and B9, the cultivars Daniel and Davidson had statistically higher levels compared with other cultivars. The concentration of vitamin B6 in cultivar Daniel and Davidson is 55.00 ± 1.06 and 31.5 ± 2.60 mAU, respectively. Vitamin B6 (pyridoxine) is the most functional coenzyme that helps in the cognitive development of neurotransmitter synthesis, immune function, gene expression, and hemoglobin formation (37, 38). The concentration of vitamin B9 found in Daniel and Davidson is 229.53 ± 3.72 and 130.01 ± 80.20 mAU, respectively. Vitamin B9, or folic acid, is required for cognitive function, cardiovascular diseases, cancer DNA synthesis, and gene expression (39). For vitamins A, D3, E, and K, the significance level was >0.05, suggesting that they are statistically similar amongst the cultivars and may not be influenced by genetics. However, Supplementary Table S1 shows the average amount of vitamin A found in all the 11 cultivars is 47.1 ± 25.39.
Vitamin A (retinol) insufficiency can lead to major health problems; it is estimated that 30% of children under the age of 5 are vitamin deficient and vitamin A deficiency accounts for 2% of all deaths in specific age groups (36). The average amount of vitamin D3 found in all black walnut cultivars was 9.7 ± 1.22 mAU. Vitamin D3 or cholecalciferol is necessary for the regulation of physical functions in the human body, especially required for bone health (40). The large amount of γ- tocopherol (vitamin E) available in black walnuts compared to Persian Walnut (8) associated with protection against prostate cancer (41). γ- Tocopherol, for example, inhibited the proliferation of tumor cells and cancer cells, including prostate cancer cells (42), also known to prevent the effects of cardiovascular diseases (20). Vitamin K or Phylloquinone was found in minimal amounts in all the cultivars. However, vitamin K insufficiency can be life-threatening since it can cause excessive bleeding and is also linked to osteoporosis and cancer (43).
Mineral Analysis
The mineral analysis of black walnut kernel and black walnut protein powder obtained from Hammons shows promising results compared to Persian walnut. Figure 4 demonstrates that the black walnut kernel and black walnut protein powder contain high amounts of N, P, and K content compared to Persian walnut. The sequence is determined by the contents (mg/g) of black walnut kernels was N>P>K>Mg>Ca>Mn>Fe>Zn>Cu, while the order in Persian walnut was N>K>P>Mg>Ca>Zn>Mn>Fe>Cu, and for black walnut, protein powder was N>P>K>Mg>Ca>Zn>Mn>Fe>Cu. The data published by USDA (34) and the data reported show similarity among minerals Ca, Fe, Mg, P, K, Zn, Mn, and Cu for both black and Persian walnut kernels.
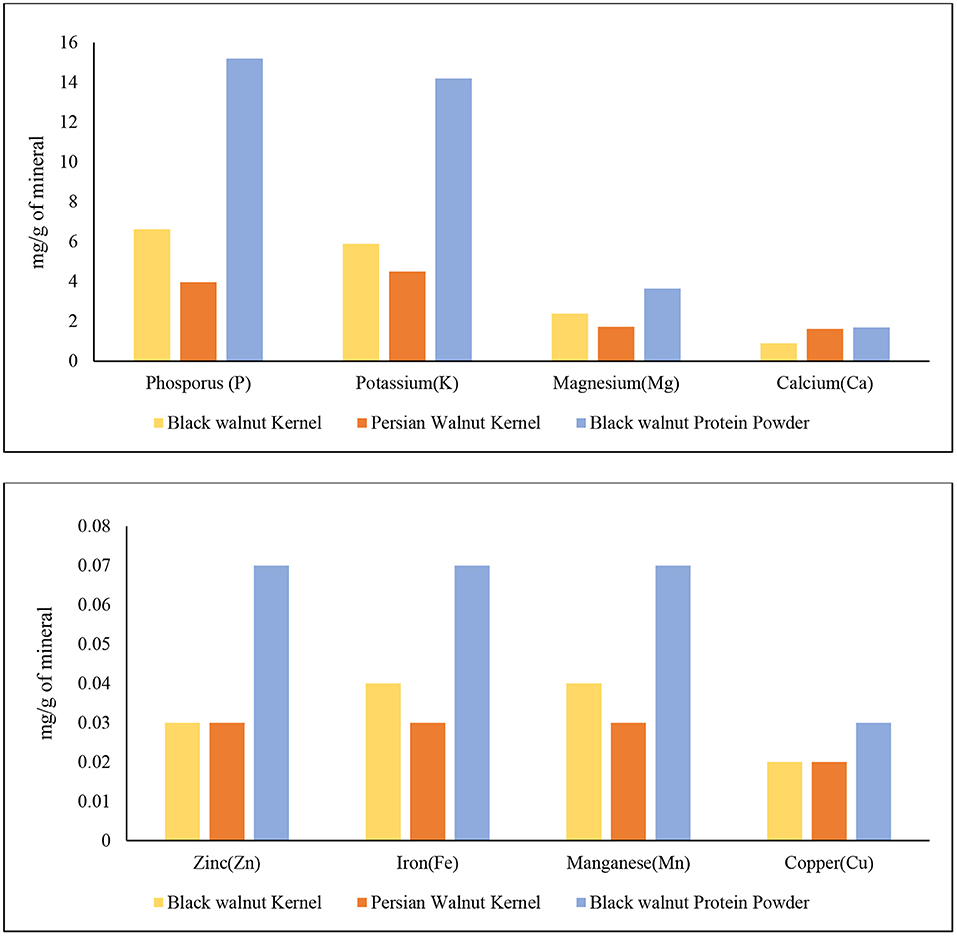
Figure 4. Mineral analysis of Black walnut kernel, Persian walnut, and Black walnut protein powder (Lab-2).
Nitrogen is necessary for all life because it is the building block of all amino acids. Malfunctions in the ubiquitin protein degradation pathway are one of the most common disorders linked with nitrogen metabolism. This route is essential for maintaining or destroying the correct proteins within a cell (44). Black walnut protein powder contains higher amount of nitrogen which is 96.6 mg/g compared to black walnut kernel 43.5 mg/g and Persian walnut 27.6 mg/g, respectively. Phosphorus plays a vital role in the human body by helping in bone mineralization, signal transduction, and ATP synthesis (45). Phosphorus deficiency can cause bone loss (46, 47), myopathy (48), and frailty (45). The mineral profile of black walnut kernel and black protein powder shows that the Phosphorus content was higher for the protein powder (15.2 mg/g) and black walnut kernel (6.63 mg/g) compared to Persian walnut, which was 3.97 (mg/g). Figure 4 also shows that potassium is the third-highest mineral found in black walnuts, which is also higher than in Persian walnuts. Potassium supplementation has been demonstrated to improve human health, especially in terms of cardiovascular diseases, in epidemiological research, animal experiments, and therapy trials (49).
Magnesium deficiency has been associated with osteoporosis, hypertension, coronary heart disease, congestive heart failure, arrhythmia, diabetes mellitus, asthma, migraine headaches, and pre-eclampsia (50). In Figure 4, both black walnut kernel and black walnut protein powder had Mg in the highest amount. Then there's Ca, which is recognized to be essential for bone and tooth formation and health. Furthermore, Ca serves as a vital electrolyte for the human body (51). Although black walnut kernel and protein powder had a numerically higher amount of Mn, Zn, Fe, and Cu than Persian walnut, the difference was minimal. In the human body, zinc is one of the most prevalent nutritionally necessary minerals. Muscle and bone contain 85% of total Zn, skin, and liver contain 11%, and all other tissues contain the remaining 4% (52). Mn is related to the metabolism process in the human body and is important in the neurological and heart function process (53). Fe is known to be an essential micronutrient for good health and normal functioning of the body. Cu serves as a cofactor for a various enzyme in the human body (52, 54). It is a dynamic element that has anti-inflammatory, antiviral properties.
Black walnuts have robust, bold taste compared to Persian walnut (55), this may be due to high potassium, and phosphorus content as potassium has a salty, bitter taste. However, it is also reported that the color of the black walnut kernel can be affected by delayed harvesting and hulling which may lead to unpleasant flavor (56). Thus, further research on volatile and mineral compounds linked to flavor of black walnuts should be conducted to understand the quality, shelf-life, nature, and develop value added nutritional products of this nut.
Amino Acid Content
The amino acids phenylalanine, valine, threonine, tryptophan, isoleucine, methionine, leucine, lysine, and histidine are critical for human life (57). These are essential amino acids which the human body cannot produce. Since humans do not manufacture essential amino acids, they serve a critical function in body development. Conditionally essential amino acids are arginine, cysteine, glycine, glutamine, histidine, proline, serine, and tyrosine (58, 59). Four different varieties of walnut kernels are said to have 16 different amino acids (60), and among them, leucine was found in highest amounts. Figure 5 shows that leucine content is highest among all the amino acids in black walnut. Moreover, leucine was comparatively higher in black walnut flour than in protein powder. In terms of protein synthesis and degradation, leptin secretion, energy balance modulation, and so on, leucine appears to be the most potent (61). The order depending on the content of essential amino acid (mg/g) in black walnut flour is leucine> valine> phenylalanine> isoleucine> threonine> lysine> histidine> methionine> tryptophan, while the order in black walnut protein powder was leucine> phenylalanine> valine> isoleucine> threonine> lysine> histidine> tryptophan> methionine. The second highest content is valine which is comparatively higher in black walnut flour than in protein powder. The essential acid methionine is found to be the lowest amount in black walnut flour than in protein powder, but the difference is negligible.
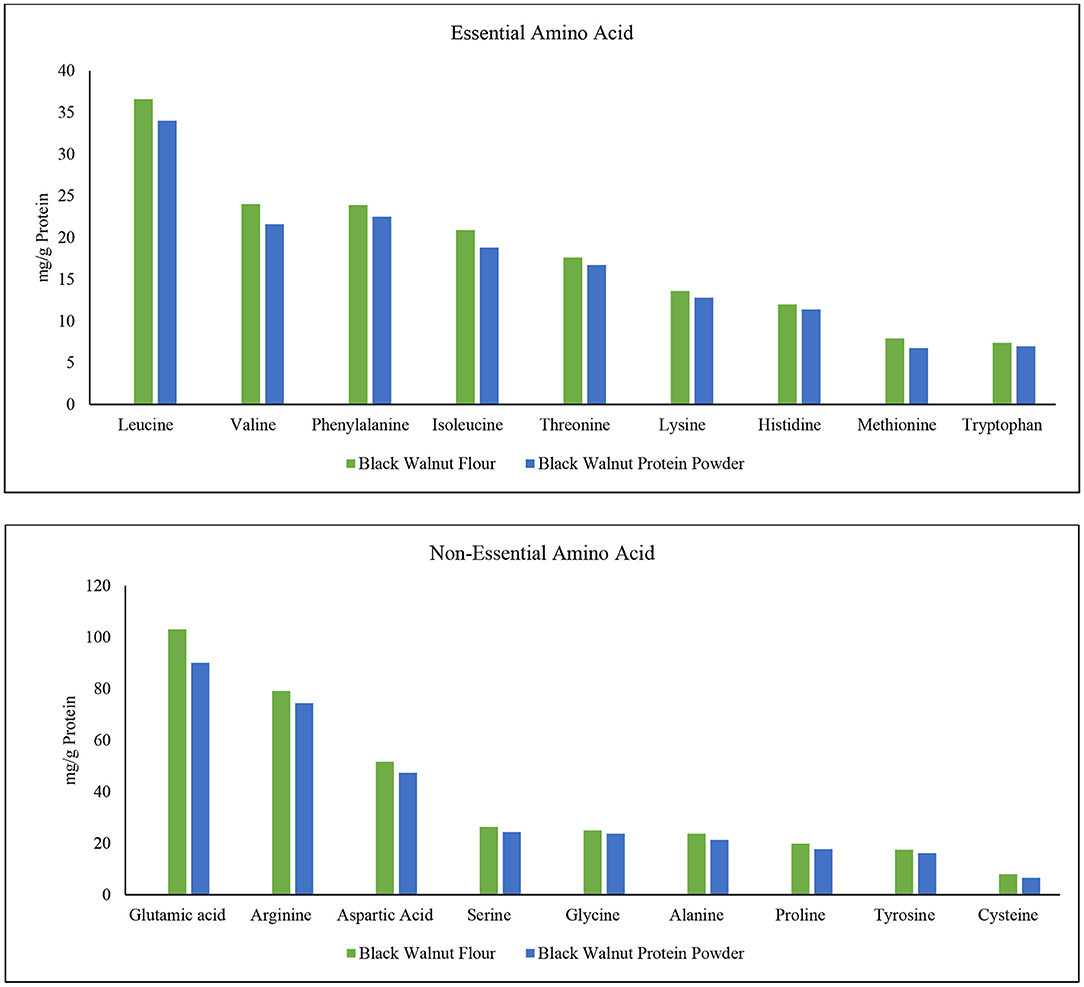
Figure 5. Essential and non-essential amino acid composition in black walnut flour (Lab-1) and black walnut protein powder (Lab-2).
Unlike essential amino acids, a healthy human body could produce non-essential amino acids if food enriched with protein is taken on a daily dietary basis. Non-essential amino acids, on the other hand, help with tissue growth and repair, immunological function, hormone production, and the formation of red blood cells in the human body (34). The order depending on the content of non-essential amino acid (mg/g of protein) in black walnut flour is glutamic acid> arginine> aspartic acid> serine> glycine> alanine> proline> tyrosine> cysteine, while the order in black walnut protein powder was glutamic acid> arginine> aspartic acid> serine> glycine> alanine> proline> tyrosine> cysteine.
In general, plant-based protein contains less calories and fat than animal-based protein while being high in fiber and essential nutrients, making these protein sources a good alternative for healthy diets. As a plant-based edible product, black walnuts are a rich source of protein, containing a low amount of sugar compared to Persian walnut (8). The edible nut rich in bioactive and other compounds could be a source of protein for maintaining a healthy diet. For instance, Chickpea and pea contain 20.5 g and 5.42 g of protein in a 100g portion (34) respectively, which is low compared to black walnut. The amount of sugar in black walnut is 1.1g/ 100g, which is low compared to chickpea and pea at 10.7g and 5.67g in 100g portion, respectively (34). Black walnut protein powder contains a high level of mineral content which could also be incorporated into a daily diet to promote health benefits.
Conclusion
Black walnuts are prized for the kernels' bold, distinctive, earthy flavor and fine-quality hardwood timber. This study demonstrated that vitamin analysis of 11 black walnut cultivars, vitamin B1, B2, B5, B6, B9, H, and C, there were significantly different in vitamin content among the cultivars, but vitamin A, D3, E, and K showed no significant difference among the cultivars. The significant differences in vitamin content among black walnut cultivars in this study suggest a genetic basis for these important nutrients, which could likely be further improved via breeding.
The mineral profile of black walnut kernel, Persian walnut, and black walnut protein powder also shows that N, P, and K are available in high concentrations compared to other micronutrients. Moreover, the amount of Zn, Fe, Mn, and Cu are almost similar in the Black walnut kernel, Persian walnut, and black walnut protein powder. Amino acid analysis among black walnut flour and black walnut protein powder show that black walnut flour contains a higher amount of essential and non-essential amino acids than black walnut protein powder.
Black walnuts are also dense with high nutrient compounds, including lipids, protein, fiber, vitamins, minerals, and bioactive molecules such as phenolic compounds. However, the current market value of black walnut kernels is not as high as Persian walnut due to unfamiliarity. In addition, the distinct flavor makes this nut less likely to be used as a snacking or table nut. By determining the potential health-promoting micronutrients (vitamins and minerals) present in black walnuts, the case for its economic production and increased marketing might be strengthened. The excellent vitamin and mineral contents increase the potential for black walnuts in the competitive market. The reported micronutrient analysis shows that the kernel and some kernel products such as flour and protein powder contain a high level of bioactive compounds (vitamin B9, B5, A, Fe, Zn) required to support human health. Despite the lack of research into the capacity of black walnut to reduce disease, based on the reported data, it can be assumed that consumption of black walnut could contribute to protecting humans against numerous diseases, including diabetes, neurodegenerative, cardiovascular diseases, and cancer.
Moreover, black walnuts are used in different recipes like cake, ice cream, salad, and desserts to increase the food's palatability and flavor. In summary, black walnuts have a rich micronutrient profile, offering great potential to promote human health compared to other commercial nuts available on the market. This research will provide recommendations for the usage of black walnut resources for researchers as well as new notion for future research. In addition, a greater understanding of the health benefits of black walnut and the preservation of bioactive compounds should be the focus of future research and clinical investigations to support human health effectively.
Data Availability Statement
The raw data supporting the conclusions of this article will be made available by the authors, without undue reservation.
Author Contributions
SA: writing—original draft, formal analysis, investigation, data curation, and visualization. K-VH: formal analysis, investigation, and writing—review and editing. C-HL: formal analysis, resources, investigation, and writing—review and editing. AT: writing, editing, and contextualizing the work. SL: writing—review and editing and funding acquisition. KK: conceptualization, supervision, formal analysis, resources, writing—review and editing, project administration, and fund acquisition. All authors contributed to the article and approved the submitted version.
Funding
This work was supported by USDA Hatch Funds (MO-HAFE0003) Food Engineering and Sustainable Technologies (FEAST), University of Missouri, the University of Missouri Center for Agroforestry, and the USDA/ARS Dale Bumpers Small Farm Research Center, Agreement number 58-6020-0-007 from the USDA Agricultural Research Service.
Conflict of Interest
The authors declare that the research was conducted in the absence of any commercial or financial relationships that could be construed as a potential conflict of interest.
Publisher's Note
All claims expressed in this article are solely those of the authors and do not necessarily represent those of their affiliated organizations, or those of the publisher, the editors and the reviewers. Any product that may be evaluated in this article, or claim that may be made by its manufacturer, is not guaranteed or endorsed by the publisher.
Acknowledgments
We would like to thank Zhentian Lei and Lloyd W. Sumner at the M.U. Metabolomic Center for the analytical support and also thank the Hammons Product Company, Stockton, Missouri for black walnut samples.
Supplementary Material
The Supplementary Material for this article can be found online at: https://www.frontiersin.org/articles/10.3389/fnut.2022.936189/full#supplementary-material
Abbreviations
ANOVA, analysis of variance; BEH, ethylene bridged hybrid; CHD, coronary heart diseases; DI water, deionized water; ESI, electrospray mass spectrometer; ICP-OES, Inductively Coupled Plasma Optical Emission spectroscopy; I.L., interleukin; LDL, low density lipoprotein; mAU, milli Absorbance Unit; MCP, monocyte chemoattractant protein; MI, myocardial infection; QTOF-HRMS, Quadrupole Time-of-flight high resolution mass spectrometer; UHPLC, ultra-high-performance liquid chromatography; UHPLC-HRMS, ultra-high-performance liquid chromatography high-resolution mass spectrometry.
Footnotes
References
1. Sitz RA, Luna EK, Ibarra Caballero J, Tisserat NA, Cranshaw WS, McKenna JR. Eastern Black Walnut (Juglans nigra L.) originating from native range varies in their response to inoculation with geosmithia morbida. Front Forests Global Change. (2021) 4:12. doi: 10.3389/ffgc.2021.627911
2. Câmara CRS, Schlegel V. A review on the potential human health benefits of the black walnut: a comparison with the English walnuts and other tree nuts. Int J Food Prop. (2016) 19:2175–89. doi: 10.1080/10942912.2015.1114951
3. USDA-NRCS. The PLANTS Database, v. 3.5. Baton Rouge, LA: National Plant Data Center. Available online at: http://plants.usda.gov (accessed April 20, 2022).
4. Woeste K, Michler C. Juglans. In: Wild Crop Relatives: Genomic and Breeding Resources (Berlin, Heidelberg: Springer) (2011). p. 77–88.
5. Hammons, Black Walnuts. Hammons Products Company. Available online at: http://blackwalnuts.wpengine.com/learn-about-black-walnuts/ (accessed May 5, 2020).
6. Newton L, Fowler G, Neeley AD, Schall RA, Takeuchi Y. Pathway Assessment: Geosmithia sp. Pityophthorus juglandis Blackman Movement From The Western Into the Eastern United States. Washington, DC: U.S. Department of Agriculture, Animal Plant Health Inspection Service (2009). Available online at: https://www.uaex.uada.edu/environment-nature/ar-invasives/invasive-diseases/docs/Thousand-Cankers%20Disease.pdf (accessed December 20 2020).
7. Ros E, Mataix J. Fatty acid composition of nuts–implications for cardiovascular health. Br J Nutr. (2006) 96:S29–35. doi: 10.1017/BJN20061861
8. U.S. Department of Agriculture, Agricultural Research Service. USDA National Nutrient Database for Standard Reference, Release 25. Nutrient Data Laboratory (2012). Available online at http://www.ars.usda.gov/ba/bhnrc/ndl (accessed April 20, 2020).
9. Zheng Y, Wu S, Wang R, Wu Y, Zhang W, Han Y, et al. Analysis and correlationship of chemical components of various walnut (Juglans regia L.) cultivars. J Food Measure Character. (2020) 14:3605–14. doi: 10.1007/s11694-020-00603-0
10. Wagner KH, Kamal-Eldin A, Elmadfa I. Gamma-tocopherol–an underestimated vitamin? Ann Nutr Metab. (2004) 48:169–88. doi: 10.1159/000079555
11. Ho KV, Lei Z, Sumner LW, Coggeshall MV, Hsieh HY, Stewart GC, et al. Identifying antibacterial compounds in black walnuts (Juglans nigra) using a metabolomics approach. Metabolites. (2018) 8:58. doi: 10.3390/metabo8040058
12. Vu DC, Vo PH, Coggeshall MV, Lin CH. Identification and characterization of phenolic compounds in black walnut kernels. J Agric Food Chem. (2018) 66:4503–11. doi: 10.1021/acs.jafc.8b01181
13. Labuckas DO, Maestri DM, Perello M, Martínez ML, Lamarque AL. Phenolics from walnut (Juglans regia L.) kernels: Antioxidant activity and interactions with proteins. Food Chem. (2008) 107:607–12. doi: 10.1016/j.foodchem.2007.08.051
14. Ito H, Okuda T, Fukuda T, Hatano T, Yoshida T. Two novel dicarboxylic acid derivatives and a new dimeric hydrolyzable tannin from walnuts. J Agric Food Chem. (2007) 55:672–9. doi: 10.1021/jf062872b
15. Guijas C, Montenegro-Burke JR, Domingo-Almenara X, Palermo A, Warth B, Hermann G, et al. METLIN: a technology platform for identifying knowns and unknowns. Anal Chem. (2018) 90:3156–64. doi: 10.1021/acs.analchem.7b04424
16. Das UN. Essential fatty acids-a review. Curr Pharm Biotechnol. (2006) 7:467–82. doi: 10.2174/138920106779116856
17. Reddy LALINI, Odhav B, Bhoola KD. Natural products for cancer prevention: a global perspective. Pharmacol Ther. (2003) 99:1–13. doi: 10.1016/S0163-7258(03)00042-1
18. Mathew A, Peters U, Chatterjee N, Kulldorff M, Sinha R. Fat, fiber, fruits, vegetables, and risk of colorectal adenomas. Int J Cancer. (2004) 108:287–92. doi: 10.1002/ijc.10984
19. González CA, Salas-Salvadó J. The potential of nuts in the prevention of cancer. Br J Nutr. (2006) 96:S87–94. doi: 10.1017/BJN20061868
20. Vu DC, Nguyen TH, Ho TL. An overview of phytochemicals and potential health-promoting properties of black walnut. RSC Adv. (2020) 10:33378–88. doi: 10.1039/D0RA05714B
21. Vu DC, Park J, Ho KV, Sumner LW, Lei Z, Greenlief CM, et al. Identification of health-promoting bioactive phenolics in black walnut using cloud-based metabolomics platform. J Food Meas Charact. (2020) 14:770–7. doi: 10.1007/s11694-019-00325-y
22. Zhang Z, Liao L, Moore J, Wu T, Wang Z. Antioxidant phenolic compounds from walnut kernels (Juglans regia L.). Food Chem. (2009) 113:160–5. doi: 10.1016/j.foodchem.2008.07.061
23. Ponder F. Soils Nutrition Management for Black Walnut. United States Department of Agriculture Forest Service General Technical Report Nc, 243 (2004). 71 p. Available online at: https://www.nrs.fs.fed.us/pubs/4711 (accessed April 20, 2022).
24. Reid W, Coggeshall M, Garrett HE, Van Sambeek J. Growing Black Walnut for Nut Production. AF1011-2009. Agroforestry in Action. Columbia, MO: University of Missouri Center for Agroforestry (2009). 16 p. Available online at: https://www.kansasforests.org/forest_products/forest_product_docs/Growing%20BW%20for%20Nut%20Production.pdf (accessed April 20, 2022).
25. Ho KV, Schreiber KL, Park J, Vo PH, Lei Z, Sumner LW, et al. Identification and quantification of bioactive molecules inhibiting pro-inflammatory cytokine production in spent coffee grounds using metabolomics analyses. Front Pharmacol. (2020) 11:229. doi: 10.3389/fphar.2020.00229
26. Tautenhahn R, Patti GJ, Rinehart D, Siuzdak G. XCMS Online: a web-based platform to process untargeted metabolomic data. Anal Chem. (2012) 84:5035–9. doi: 10.1021/ac300698c
27. Barnes KW. A streamlined approach to the determination of trace elements in foods. Atomic Spectrosc Norwalk Connect. (1998) 19:31–9.
28. Naozuka J, da Veiga MAMS, Oliveira PV, de Oliveira E. Determination of chlorine, bromine and iodine in milk samples by ICP-OES. J Anal At Spectrom. (2003) 18:917–21. doi: 10.1039/b303897c
29. Horwitz W. Official methods of analysis of AOAC International. In: W. Horwitz, editor. Agricultural Chemicals, Contaminants, Drugs. Gaithersburg, MD: AOAC International (2010).
30. Schuster R. Determination of amino acids in biological, pharmaceutical, plant and food samples by automated precolumn derivatization and high-performance liquid chromatography. J Chromatogr B Biomed Sci Appl. (1988) 431:271–84. doi: 10.1016/S0378-4347(00)83096-0
31. Miller JW, Rucker RB. Pantothenic acid. In: Marriott BP, Birt DF, Stallings VA, Yates AA, editors. Present Knowledge in Nutrition. 7th ed. Academic Press (2020). p. 273–87. doi: 10.1016/B978-0-323-66162-1.00016-0
33. Powers HJ. Hilary. Riboflavin (vitamin B-2) and health. Am J Clin Nutr. (2003) 77:1352–60. doi: 10.1093/ajcn/77.6.1352
34. U. S. Department of Agriculture, Agricultural Research Service, Nutrient Data Laboratory. USDA Branded Food Products Database. (2018). Available online at: https://www.ars.usda.gov/nutrientdata (accessed April 20, 2022).
35. Trüeb RM. Serum biotin levels in women complaining of hair loss. Int J Trichology. (2016) 8:73. doi: 10.4103/0974-7753.188040
36. Aşkin Ö, Uzunçakmak TKÜ, Altunkalem N, Tüzün Y. Vitamin deficiencies/hypervitaminosis and the skin. Clin Dermatol. (2021) 39:847–57. doi: 10.1016/j.clindermatol.2021.05.010
37. Spinneker A, Sola R, Lemmen V, Castillo MJ, Pietrzik K, Gonzalez-Gross M. Vitamin B6 status, deficiency and its consequences-an overview. Nutr Hosp. (2007) 22:7–24. Available online at: http://www.redalyc.org/articulo.oa?id=309226714003
38. Brown MJ, Beier K. Vitamin B6 Deficiency (Pyridoxine). Latest Version: July, 2021. Treasure Island, FL: StatPearls (2019).
40. Kechichian E, Ezzedine K. Vitamin D and the skin: an update for dermatologists. Am J Clin Dermatol. (2018) 19:223–35. doi: 10.1007/s40257-017-0323-8
41. Helzlsouer KJ, Huang HY, Alberg AJ, Hoffman S, Burke A, Norkus EP, et al. Association between α-tocopherol, γ-tocopherol, selenium, and subsequent prostate cancer. J Natl Cancer Inst. (2000) 92:2018–23. doi: 10.1093/jnci/92.24.2018
42. Gysin R, Azzi A, Visarius T. γ-Tocopherol inhibits human cancer cell cycle progression and cell proliferation by down-regulation of cyclins. FASEB J. (2002) 16:1952–4. doi: 10.1096/fj.02-0362fje
43. Ciechanover A. Ubiquitin-mediated proteolysis and male sterility. Nat Med. (1999) 2:1188–90. doi: 10.1038/nm1196-1188
45. Serna J, Bergwitz C. Importance of dietary phosphorus for bone metabolism and healthy aging. Nutrients. (2020) 12:3001. doi: 10.3390/nu12103001
46. Boullion R. Vitamin D: From Photosynthesis, Metabolism, and Action to Clinical Application. Philadelphia, PA: Elsevier Saunders (2006). p. 1435–1463.
47. Raisz LG, Trummel CL, Holick MF, DeLuca HL. 1, 25-dihydroxycholecalciferol: a potent stimulator of bone resorption in tissue culture. Science. (1972) 175:768–9. doi: 10.1126/science.175.4023.768
48. Halevy J, Bulvik S. Severe hypophosphatemia in hospitalized patients. Arch Intern Med. (1988) 148:153–5. doi: 10.1001/archinte.1988.00380010155016
49. He FJ, MacGregor GA. Beneficial effects of potassium on human health. Physiol Plant. (2008) 133:725–35. doi: 10.1111/j.1399-3054.2007.01033.x
51. Bryant RJ, Cadogan J, Weaver CM. The new dietary reference intakes for calcium: implications for osteoporosis. J Am Coll Nutr. (1999) 18(sup5):406S−12S. doi: 10.1080/07315724.1999.10718905
52. Tapiero H, Townsend DÁ, Tew KD. Trace elements in human physiology and pathology. Copper Biomed. Pharmacother. (2003) 57:386–98. doi: 10.1016/S0753-3322(03)00012-X
53. Ding Y, Luo Y, Fu J. Effects of Mn (II) on peroxynitrite nitrifying fibrinogen. Biomed Mater Eng. (2014) 24:901–7. doi: 10.3233/BME-130884
54. Fraga CG. Relevance, essentiality and toxicity of trace elements in human health. Mol Aspects Med. (2005) 26:235–44. doi: 10.1016/j.mam.2005.07.013
55. Warmund MR, Elmore J, Drake M, Yates MD. Descriptive analysis of kernels of selected black and Persian walnut cultivars. J Sci Food Agric. (2009) 89:117–21. doi: 10.1002/jsfa.3417
56. Warmund MR. Kernel color of three black walnut cultivars after delayed hulling at five successive harvest dates. HortScience. (2008) 43:2256–8. doi: 10.21273/HORTSCI.43.7.2256
57. Young VR. Adult amino acid requirements: the case for a major revision in current recommendations. J Nutr. (1994) 124(suppl_8):1517S−23S. doi: 10.1093/jn/124.suppl_8.1517S
58. Reeds PJ. Dispensable and indispensable amino acids for humans. J Nutr. (2000) 130:1835S−40S. doi: 10.1093/jn/130.7.1835S
59. Fürst P, Stehle P. What are the essential elements needed for the determination of amino acid requirements in humans? J Nutr. (2004) 134:1558S−65S. doi: 10.1093/jn/134.6.1558S
60. Wu K, Xiao Q, Tang L, Pang Y, Wei L, Zhang L. Differences analysis on the contents of crude fat and amino acids in kernel of walnut. Nonwood Forest Res. (2007) 25:15–8. doi: 10.14067/j.cnki.103-8981.2007.02.006
Keywords: agroforestry, cultivars, indigenous, metabolomics (OMICS), micronutrients, plant based food, food and health
Citation: Antora SA, Ho K-V, Lin C-H, Thomas AL, Lovell ST and Krishnaswamy K (2022) Quantification of Vitamins, Minerals, and Amino Acids in Black Walnut (Juglans nigra). Front. Nutr. 9:936189. doi: 10.3389/fnut.2022.936189
Received: 04 May 2022; Accepted: 22 June 2022;
Published: 27 July 2022.
Edited by:
Andrea Del Pilar Sánchez-Camargo, University of Los Andes, ColombiaReviewed by:
Esra Capanoglu, Istanbul Technical University, TurkeyShaobing Peng, Northwest A&F University, China
Khalid Gul, National Institute of Technology Rourkela, India
Copyright © 2022 Antora, Ho, Lin, Thomas, Lovell and Krishnaswamy. This is an open-access article distributed under the terms of the Creative Commons Attribution License (CC BY). The use, distribution or reproduction in other forums is permitted, provided the original author(s) and the copyright owner(s) are credited and that the original publication in this journal is cited, in accordance with accepted academic practice. No use, distribution or reproduction is permitted which does not comply with these terms.
*Correspondence: Kiruba Krishnaswamy, a3Jpc2huYXN3YW15a0B1bXN5c3RlbS5lZHU=