- 1Department of Agriculture and Environmental Sciences, National Institute of Food Technology Entrepreneurship and Management, Sonipat, India
- 2Department of Horticultural Science, Imam Khomeini International University, Qazvin, Iran
- 3Department of Plant Biology (Plant Physiology), Faculty of Biology, University of Murcia, Murcia, Spain
- 4Department of Horticultural Sciences, University of Florida, Gainesville, FL, United States
- 5Postharvest Research Laboratory, Department of Botany and Plant Biotechnology, University of Johannesburg, Johannesburg, South Africa
The efficacy of the signaling molecule melatonin for alleviating chilling injury (CI) in mango (Mangifera indica L.) fruit was studied to investigate the potential role of membrane integrity, energy charge, and ripening-related changes in the development of CI, and its management by melatonin. ‘Langra’ and ‘Gulab Jamun’ cultivar mango fruit was immersed in 100 μM of melatonin before storage for 28 days at 5°C with weekly transfers to shelf life at 25°C. CI symptom development was associated with compositional and enzymatic aspects of textural changes, cell membrane deterioration, and chemical energy status. Melatonin-treated ‘Langra’ fruit exhibited very low CI (5 vs. 21%) while ‘Gulab Jamun’ fruit exhibited higher CI (36 vs. 38%) during 28 days of storage at 5 ± 1°C. Higher chilling tolerance in melatonin-treated ‘Langra’ was associated with lower softening, ascribed to lower cell wall degrading exo- and endo-polygalacturonase, pectinesterase, and endo-1,4-β-D-glucanase. In addition, lower membrane deteriorating-phospholipase D and lipoxygenase activity in melatonin-treated ‘Langra’ corresponded to lower palmitic and stearic acids and higher oleic, linoleic, and linolenic acids accumulation, thus, higher unsaturated/saturated fatty acids ratio. Additionally, there was a higher intracellular energy supply with melatonin, represented by a higher adenylate energy charge (AEC) arising from higher ATP and ADP and lower AMP accumulation, related to higher H+-ATPase, Ca2+-ATPase, succinate dehydrogenase, and cytochrome c oxidase activities. This study for the first time provides evidence, suggesting that melatonin alleviation of CI is related to the preservation of membrane integrity, thereby protecting the intracellular energy supply, and preserving cell wall integrity via impeding cell wall degrading enzyme activities.
Introduction
Mango (Mangifera indica) is economically one of the most important fruits, possessing high nutritive value, pleasant flavor, and attractive color and aroma (1). The nutritional composition of mango includes the presence of bioactive components (carotenoids, phenolic compounds, and ascorbic acid), fibers, minerals, vitamins, and organic acids (2). The unique qualities of mango fruit have resulted in its worldwide production in nearly 90 countries (3). Mango is a tropical fruit with high perishability due to high respiration and ethylene production rates, a climacteric ripening pattern, and a tropical and sub-tropical nature, which limits postharvest life to 3–4 days under ambient conditions.
The low-temperature storage is the most commonly used technique to extend the shelf life and to assure the nutritional aspect and sensory quality of mangoes. Most Indian mango cultivars cannot tolerate temperatures below 13°C due to the development of chilling injury (CI); the cultivars ‘Langra’ and ‘Dashehari’ are exceptions to some extent, tolerating exposure to 8–9 C. Symptoms of CI include abnormal (uneven) softening and color development, peel darkening and pitting, poor flavor due to lack of aroma, and lenticel prominence (4–7). Therefore, methods that can alleviate CI may raise global marketing by reducing postharvest degradation and increasing shelf life.
Among all biological processes, bio-membrane alteration is considered the initiation event at the molecular level in CI (8). Further, this cell membrane alteration is partially related to the homeostasis of cellular energy status. Insufficient energy supply to meet the demand of physiological metabolism in stressful conditions will lead to excessive reactive oxygen species (ROS). The ROS damages the cellular membrane, resulting in exacerbated permeability via membrane fatty acids peroxidation, resulting in a lower unsaturated to saturated fatty acids (unSFA/SFA) ratio (9). Besides this, fruit softening, which is a characteristic feature of fruit ripening, is abnormal in fruit exposed to chilling temperature due to impeded activity of cell wall metabolizing enzymes, such as polygalacturonase (PG), pectin esterase (PE), and endo-1,4-β-D-glucanase (EGase) (10–13). Therefore, regulation of CI susceptibility in fruit and vegetables is related to a series of these mechanisms. Any innovation or chemical treatment that can mitigate these major causes of CI has the potential to promote chilling tolerance in horticultural crops.
In plants, melatonin (N-acetyl-5-methoxytryptamine) not only serves as a receptor-dependent signaling molecule but also exhibits a powerful receptor-independent ROS-scavenging cascade (14–16). Recently, postharvest preservation and quality maintenance of fruits and vegetables by MT have gained attention due to strong evidence for their role in delaying senescence and promoting tolerance against fungal decay (17–19). Furthermore, many studies have provided evidence that MT application demonstrates a prominent efficiency in conferring chilling tolerance in pomegranate (20, 21), tomato (22–24), peach (10, 25–27), cucumber (28), litchi (29, 30), bell pepper (31), plum (32), mango (4–7), and banana (33) fruits by (1) promoting endogenous MT accumulation accompanied by (2) promotion of reduced nicotinamide adenine dinucleotide phosphate (NADPH) oxidase enzyme activity and signaling H2O2 accumulation, (3) enhancing ROS protection and scavenging systems activity, (4) preserving membrane unsaturation status by higher expression of fatty acid desaturase (FADs) genes, along with (5) lower expression of phospholipase D (PLD) and lipoxygenase (LOX) genes and enzyme activities, (6) promoting oxidative pentose phosphate, shikimic acid and phenylpropanoid pathways activity, (7) enhancing arginase pathway activity by activating the ZAT2/6/12-CBF1 signaling pathway for triggering endogenous nitric oxide, polyamines, proline, and γ-aminobutyric acid (GABA) accumulation, (8) enhancing intracellular energy adenosine triphosphate (ATP) and reducing power of NADPH supply, and (9) activating GABA shunt pathway activity.
However, to the best of our knowledge, there has been no study that evaluated the effectiveness of MT application concerning mango fruit chilling tolerance in terms of cell wall modification, membrane lipid metabolism, and energy metabolism. Therefore, the present study was conducted to investigate the effect of exogenous MT treatment in relation to cultivar dependent variability of chilling tolerance due to (i) cell wall modification enzymes, (ii) membrane lipid metabolism, and (iii) energy metabolism of mango fruit stored at the chilling temperature of 5 ± 1°C for up to 28 days.
Materials and Methods
Mango Fruit and MT Treatment
Fruit of the mango (Mangifera indica L.) cultivars ‘Langra’ and ‘Gulab Jamun’ were used in this study. The fruit was physiologically mature but pre-ripe, having 29 ± 1 N firmness at harvest time; however; the data presented here is after 3 days of shelf life. The fruit was hand-harvested from an orchard located in Sonepat, Haryana, India. The harvested fruit was transported to the laboratory and fruit with any injury or defects was sorted out from the main lot. The sorted fruit was disinfected with 1% (v/v) sodium hypochlorite for 2 min followed by a random division of 300 fruit from each cultivar into six groups, consisting of 50 fruits in each group. The first three groups were immersed in distilled water (control) in three different buckets, and the other three groups were immersed in 100 μM MT (treated) for 2 h at 25 ± 2°C under the low light conditions in three different buckets (7). After air drying for 2 h at 25°C, the fruits were placed into storage at 5 ± 1°C and RH 85–90%. Observations were taken after 3 days of simulated shelf life at ambient temperature (25°C, 90–95% RH) on every 7 days interval during 28 days of storage. Each observation was recorded in triplicates and each replicate consisted of three fruits. For analyses, the fruit epicarp and mesocarp tissues were frozen with liquid nitrogen on the day of observation followed by storage at −80°C. Besides this, a lot of 45 fruits from each treatment were used for recording CI symptom development at ambient conditions (25°C, 90–95% RH) after 3 days of shelf life (7).
Chilling Injury Index and Firmness Evaluation
Visual assessment based on surface symptoms in the form of skin darkening, pitting, and lenticel prominence was used for providing the ranking to CI as described by Concellón et al. (34) with some modifications. In this study, rank 0 indicates none, rank 1 indicates 1–20%, rank 2 indicates 21–40%, rank 3 denotes 41–60%, rank 4 denotes 61–80%, and rank 5 denotes 81–100% severity in CI symptoms over mango fruit. The assessment was done with fruit in triplicates, which were kept at ambient conditions for 3 days after their removal from cold storage. The CII was calculated as the sum of the product of rank given and the number of fruits in that particular scale divided by the total number of fruits considered in that observation.
The firmness of fruit mesocarp was recorded with a texture analyzer (TA.HD Plus, Stable Micro Systems, United Kingdom) with 1.5 mm s–1 pre-test speed, 0.5 mm s–1 test speed, and 10 mm s–1 post-test speed. The probe used was 2 mm in diameter and the set penetration depth for observation at the equatorial region was taken as 5 mm. The fruit firmness was measured in triplicates on two opposite sides of the equatorial plane of each fruit.
Cell Wall Degrading Enzymes Extraction and Activity Assessment
The extraction and assessment of activities of exo-PG (EC 3.2.1.82), endo-PG (EC 3.2.1.15), pectin esterase (PE; EC 3.1.1.11), and EGase (EC 3.2.1.4) were done according to Khan and Singh (35) with some modifications. For extraction, 13 g of fruit peel and pulp tissues were homogenized (IKA T18 Digital Ultra-Turrax, Cole-Parmer, India) separately with 13 ml of chilled 0.2% (w/v) sodium bisulphite (NaHSO3) and 12% (w/v) polyethylene-glycol (PEG). The homogenate was centrifuged (3-18KS, Sigma, Germany) at 12,000 × g at 4°C for 30 min, and the pellets were each washed with 4 ml of 0.2% (w/v) of NaHSO3 solution. The pellets obtained after re-centrifuging (3-18KS, Sigma, Germany) at 12,000 × g for 40 min were stored at −80°C and were used to determine the peel and pulp activities of exo-PG, endo-PG, PE, and EGase. For exo-PG and endo-PG, a pellet immersed in 50 mM sodium acetate buffer (pH 5.0) containing 0.5 M sodium chloride was incubated for 1 h at 4°C on an incubator shaker. Incubation was followed by centrifugation (3-18KS, Sigma, Germany) at 12,000 × g at 4°C for 15 min and the supernatant was diluted with 50-mM sodium acetate buffer (pH 5.0). The diluted supernatant was used as a crude extract for the determination of the activities of exo-PG and endo-PG.
For the exo-PG and endo-PG activities, mixtures consisting of 0.15 ml of 50 ml sodium acetate buffer (pH 4.4) containing 0.5% (w/v) of polygalacturonic acid (PGA) and 0.15 ml of an enzyme extract were incubated for 18 h at 30°C. An assay tube consisting of the above mixture, 0.1 M borate buffer (pH 9.0), and 1% (w/v) cyanoacetamide was boiled for 10 min, cooled, and the absorbance of the reaction mixture was measured at 274 nm with a spectrophotometer (Specord 200plus, Analytik Jena, Germany). The activity of the exo-PG enzyme was expressed in terms of μg of galacturonic acid mg–1 protein h–1 according to the standard curve of D-galacturonic acid. For endo-PG, 3 ml of an enzyme extract and chilled 2% (w/v) PGA in a 50-mM sodium acetate buffer (pH 4.4) were measured for viscosity change during 18 h of incubation period at 30°C with a viscometer (LABMAN Digital Rotational Viscometer, LMDV-60, India). The activity of endo-PG was expressed as a change in viscosity, i.e., Δ viscosity (mg–1 protein h–1) in triplicates with three fruits in each replication.
For PE activity, the pellet obtained through the above-mentioned extraction process was re-suspended in 15 ml of chilled 7.5% (w/v) sodium chloride, 0.75% (w/v) ethylenediaminetetraacetic acid (EDTA) solution at pH 6.5. After re-suspension, the mixture was incubated for 10 min at 4°C followed by centrifugation (3-18KS, Sigma, Germany) at 12,000 × g for 15 min. A total of 20 m of a citrus pectin solution (1%, w/v; pH 7.5) was mixed with 5 ml of the supernatant obtained as above, followed by titration with 0.01 N sodium hydroxide (NaOH). The activity of PE was estimated in triplicates (with three fruits in each replication) and was calculated from the total volume of NaOH solution required to maintain the pH of the reaction mixture up to 7.4 and expressed as mM NaOH mg–1 protein h–1.
The EGase activity was determined by stirring the extraction pellet in 15 ml of 0.1 M citrate-phosphate buffer (pH 6.0) consisting of 1 M NaCl followed by incubation for 1 h. The mixture was centrifuged (3-18KS, Sigma, Germany) at 12,000 × g for 15 min, and the supernatant obtained was mixed with 6 ml of 0.2% (w/v) carboxymethyl cellulose in citrate-phosphate buffer (pH 6.0). The activity of EGase was measured in triplicates with three fruits in each group. Estimation was done as a change in viscosity during 18 h of incubation at 30°C with a viscometer (LABMAN Digital Rotational Viscometer, LMDV-60, India) and was expressed as Δ viscosity (mg–1 protein h–1).
Cell Membrane Deteriorating Enzymes Extraction and Activity Assessment
The activity of PLD (EC 3.1.1.4) was determined according to Liu et al. (36) involving some modifications. For extraction, 1 g of fruit tissue was homogenized (IKA T18 Digital Ultra-Turrax, Cole-Parmer, India) with 5 ml of chilled 100-mM Tris-HCl buffer (pH 7.0), followed by centrifugation (3-18KS, Sigma, Germany) at 12,000 × g at 4°C for 15 min. The supernatant obtained was used for PLD estimation. First, the reaction substrate was prepared by the addition of 0.4 g of lecithin (phosphatidylcholine) to 50 ml of ether followed by its evaporation using a rotary evaporator (Buchi, Germany) at 35°C. The dried solution was re-dissolved in 1 L of 100 mM of acetate buffer (pH 5.5) consisting of 5 mM of dithiothreitol (DTT) and 25 mM of calcium chloride. In an assay tube, 1 ml of enzyme extract was mixed with 3 ml of reaction substrate, followed by 1 h shaking at 28°C. The reaction substrate was washed with petroleum ether and the aqueous layer was collected. Furthermore, 2 g of Reinecke salt (NH4[Cr(NCS)4(NH3)2]⋅H2O) was dissolved in 100 ml of methanol and added to the reaction mixture in order to get a precipitate after centrifugation (3-18KS, Sigma, Germany) at 12,000 × g for 10 min. The precipitate was re-dissolved in 3 ml acetone, followed by spectrophotometric (Specord 200plus, Analytik Jena, Germany) analysis at 520 nm. The standard curve of choline chloride in 100-mM acetate buffer (pH 5.6) was used for estimation of PLD activity from triplicate samples (in which each replication consisted of three fruit), where 1 U was defined as mM of choline produced min–1.
For LOX (EC 1.13.11.12) enzyme, 1 g of fruit tissue was homogenized from triplicate samples (three fruit in each replication) with 5 ml of Tris-HCl buffer (100 mM, pH 7.0), followed by centrifugation (3-18KS, Sigma, Germany) at 10,000 × g at 4°C for 20 min. The LOX activity was assayed according to the method of Lin et al. (37) with some modifications. The standard assay mixture was prepared by mixing 200 μl Tween 20 and 40 μl linoleic acid in 40 m of sodium phosphate buffer (100 mM, pH 7.0). In a cuvette, 200 μl of LOX extract was added to a 1 ml standard assay mixture, and the absorbance was recorded (Specord 200plus, Analytik Jena, Germany) at 234 nm. The result was expressed in terms of U mg–1 protein, where U was defined as the amount of enzyme that causes an increase in absorption at 25°C by acting over linoleic acid.
Fatty Acid Profiling (Unsaturated and Saturated)
The extraction of fatty acids was done in triplicates (three fruit in each replicate), according to Cao et al. (38), with some modifications. For the process of extraction, 20 g of fruit tissue was homogenized (IKA T18 Digital Ultra-Turrax, Cole-Parmer, India) with 10 ml of a mixture consisting of methanol, 0.1 M HCl, and water at a ratio of 200:100:1, followed by centrifugation (3-18KS, Sigma, Germany) at 12,000 × g for 10 min. The organic phase was dried, and methylation of fatty acids was done at boiling temperature with the addition of 1 ml of 140 M boron trifluoride dissolved in methanol. Furthermore, extraction of methylated fatty acids was done with hexane, again taken to dryness, followed by re-dissolving in 200 μl of chloroform prior to injection. Separation and quantification of fatty acids were done according to Mirdehghan et al. (39) with some modifications, using gas chromatography (Shimadzu GC-MS-TQ 8040, Kyoto, Japan).
Estimation of Ca2+-ATPase and H+-ATPase Activities
The preparation of the extract for determination of Ca2+-ATPase (EC 7.2.2.10) and H+-ATPase (EC 3.6.3.6) activities was done in triplicates (each replication consisting of three fruit), according to Jin et al. (40), incorporating some modifications. The extraction buffer consisting of 50 mM Tris-HCl buffer (pH 8.0), 0.25 M sucrose, 0.3 M mannite, 0.5 g L–1 polyvinyl pyrrolidone, and 1 M EDTA was prepared. Afterward, 1 g of fruit tissue was homogenized (IKA T18 Digital Ultra-Turrax, Cole-Parmer, India) with 10 ml of pre-cooled extraction buffer. The homogenate was centrifuged (3-18KS, Sigma, Germany) at 5,000 × g at 4°C for 10 min, and the obtained supernatant was collected. The remaining sediment was washed with washing buffer prepared from 10-mM Tris-HCl, 0.25-M sucrose, 1-mM EDTA, and 0.3 M mannite. Finally, the sediment in dissolution with washing buffer was used as an enzyme extract.
For the assessment of Ca2+-ATPase and H+-ATPase, the reaction mixtures were prepared and the procedure followed was as per Jin et al. (40) with some modification. The reaction mixture of Ca2+-ATPase consists of Tris-HCl buffer (30 mM, pH 8.0), 3 mM magnesium sulfate (Mg2SO4), 0.1 mM sodium orthovanadate (Na3VO4), 50 mM sodium nitrate (NaNO3), 0.1 mM ammonium molybdate, 3 mM calcium nitrate [Ca(NO3)2], and an enzyme extract. On the other hand, the H+-ATPase reaction mixture consists of all the above mentioned constituents except 3 mM Ca(NO3)2. An assay tube consisting of a reaction mixture was added with 100 μl of 30-mM ATP-Tris (pH 8.0), followed by 20 min incubation at 37°C. Afterward, the reaction was terminated with the addition of 30 mM trichloroacetic acid (TCA), and absorbance was recorded at 660 nm with a spectrophotometer (Specord 200plus, Analytik Jena, Germany), and 1 unit of activity was expressed as a release of 1 μM phosphorus min–1.
AMP, ADP, and ATP Measurement and Adenylate Energy Charge Calculation
The measurement of AMP, ADP, ATP, and AEC was done according to Yi et al. (41) with some modifications. For extract preparation, 2 g of fruit tissue was homogenized (IKA T18 Digital Ultra-Turrax, Cole-Parmer, India) with 5 ml of 0.6 M perchloric acid, followed by centrifugation (3-18KS, Sigma, Germany) at 12,000 × g at 4°C for 20 min. The supernatant volume was immediately adjusted to have a pH between 6.5–6.8 using potassium hydroxide solution. The final volume of supernatant was maintained at 4 ml and passed through a 0.45-μm filter (Millipore, HiMedia, India). Furthermore, AMP, ADP, and ATP were examined by high-performance liquid chromatography (HPLC) (Waters Corporation, United States) using a reserved-phase C18 column (Waters 80 Å, 5 μm particle size, 4.6 mm × 250 mm), accompanied by ultraviolet detector at wavelength 254 nm. Mobile phase A consisted of 0.04 M potassium dihydrogen phosphate and 0.06 M dipotassium hydrogen phosphate dissolved in distilled water and maintained at pH 7.0 using 0.1 M potassium hydroxide, whereas acetonitrile was used as mobile phase B. Programming consisted of a linear gradient program with 75–100% and 0–25% of mobile phase A and B, respectively, for 7 min at a flow rate 1.2 ml min–1. Lastly, the sample (10 μl) was injected into the HPLC for analysis of AMP, ADP, and ATP as per the external standard program. The energy charge was calculated from triplicates (consisting of three fruits in each replication) as ATP+1/2 ADP divided by the summation of AMP, ADP, and ATP.
Protein Estimation
Protein estimation was done using the Bradford (42) method with some modifications. For extraction, 1 g of fruit tissue was homogenized (IKA T18 Digital Ultra-Turrax, Cole-Parmer, India) with 50 mM sodium phosphate buffer consisting of 0.5 mM magnesium chloride, 2 mM phenylmethylsulfonyl fluoride (PMSF), and polyvinylpyrrolidone (PVP). The homogenate was centrifuged (3-18KS, Sigma, Germany) at 12,000 × g at 4°C for 15 min and the supernatant obtained was used as the extract for protein determination. Furthermore, 0.1 ml of extract was mixed with 5 ml of Bradford reagent and was examined spectrophotometrically (Specord 200plus, Analytik Jena, Germany) at 595 nm after 5 min of incubation at ambient conditions. Bovine serum albumin (BSA) was used as the standard to estimate protein content in terms of mg g–1 fresh weight (FW).
Statistical Analysis
Analysis of variance (ANOVA) was used for interpretation of the experimental results using a completely randomized design in triplicates. Additionally, the means were further compared using Duncan’s Multiple Range Test using SPSS (Version 20) with a 0.05 level of significance.
Results
Chilling Injury Index
The visual symptoms of CI, such as pitting, blackening, wrinkling, and browning over the peel of mango fruit, were regarded as CI visual signs, which were observed on the third day of observation at ambient conditions after removal from cold storage (5 ± 1°C) at every 7-day interval. ‘Langra’ mangoes without MT treatment exhibited CI symptoms just after 7 days of cold storage. However, MT-treated ‘Langra’ mangoes exhibited the first signs of CI on 14 days of storage (Figure 1A).
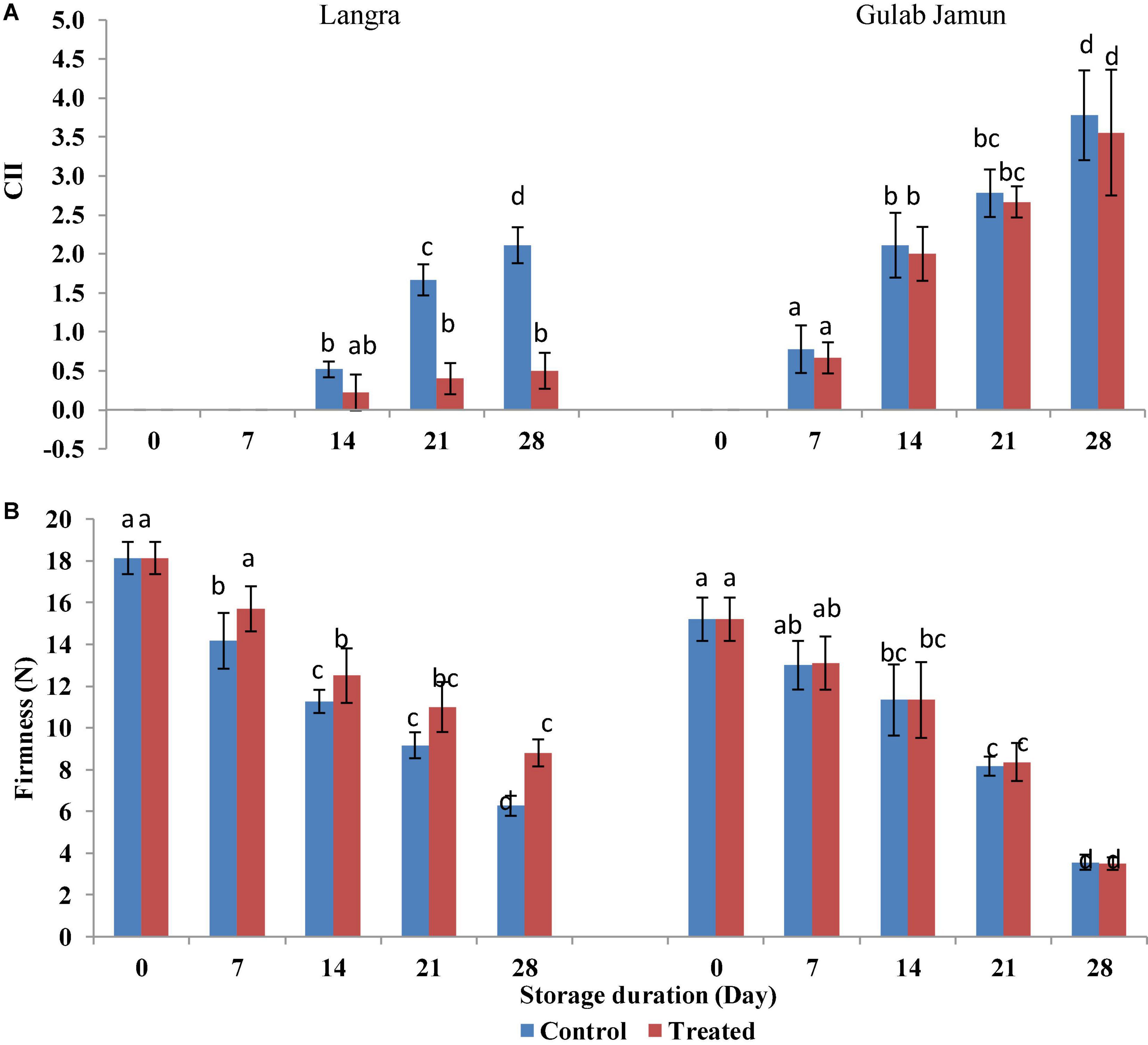
Figure 1. (A) Chilling injury index (CII), (B) firmness in ‘Langra’ and ‘Gulab Jamun’ mango fruit treated with 0 μM (control) or 100 μM (treated) MT for 2 h followed by storage at 5 ± 1°C. Readings were taken on every 7 days of 5 ± 1°C storage followed by 3 days of shelf life at room temperature (25°C, 90–95% RH). Each value is the mean for three replicates and vertical bars indicate the standard error. Error bars with different small letters on the same storage period show a significant difference (P ≤ 0.05).
Firmness
Decreasing trends in fruit firmness were seen with increasing storage duration. However, MT treatment exerted a positive effect in maintaining higher firmness of ‘Langra’ mangoes (Figure 1B). Whereas, no such effect was seen in ‘Gulab Jamun’ mangoes with exogenous MT application.
Cell Wall Degrading Enzymes Activities
The activities of fruit softening enzymes in the peel and pulp of the studied mango cultivars were positively affected by the progressive storage duration. The exo-PG (Figures 2A–D), endo-PG (Figures 2E,F), and EGase (Figures 3A–D) showed increasing trends as the duration of storage increased irrespective of the cultivar. Whereas, PE showed a somewhat decreasing trend with increasing storage duration. However, exogenous MT application was successful in suppressing the activity of exo-PG, endo-PG, and EGase, but in a cultivar dependent manner. Here, MT-treated peel and pulp of ‘Langra’ mangoes showed significant (p < 0.05) suppression in exo-PG (Figures 2A,B), endo-PG (Figures 2E,F), EGase (Figures 3A,B), and PE (Figures 3E,F) activities on one or another day. On the contrary, MT treatment resulted in increased activity of exo-PG (Figures 2C,D) and EGase (Figures 3C,D) in ‘Gulab Jamun’ mangoes; whereas no effect of MT application was noticed for endo-PG (Figures 2G,H) activity in ‘Gulab Jamun’ mangoes.
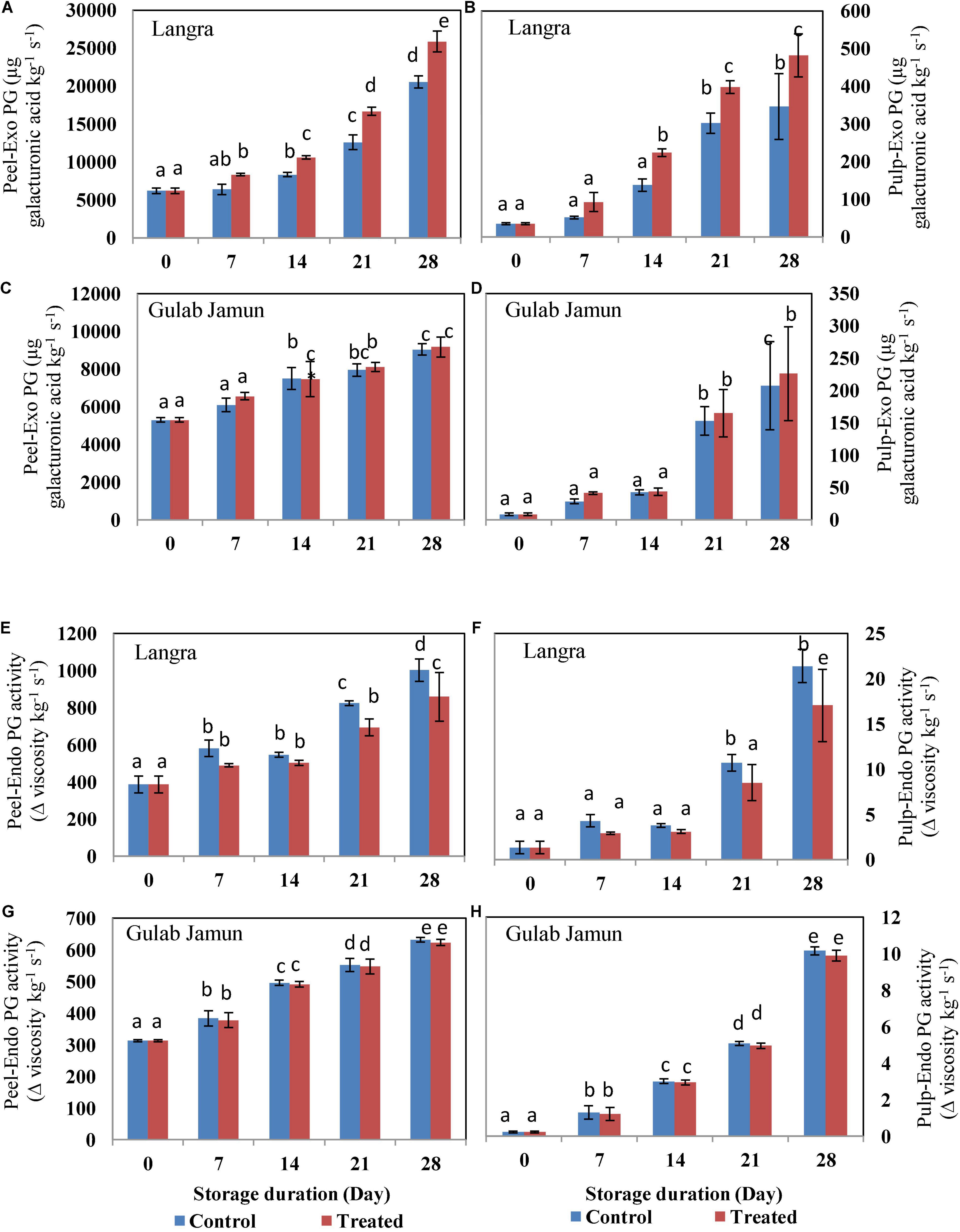
Figure 2. Exo-polygalacturonase (Exo-PG) activity in (A) ‘Langra’ peel, (B) ‘Langra’ pulp, (C) ‘Gulab Jamun’ peel, and (D) ‘Gulab Jamun’ pulp and endo-polygalacturonase (Endo-PG) activities in (E) ‘Langra’ peel, (F) ‘Langra’ pulp, (G) ‘Gulab Jamun’ peel, and (H) ‘Gulab Jamun’ pulp treated with 0 μM (control) or 100 μM (treated) MT for 2 h, followed by 28 days of storage at 5 ± 1°C and 3 days of shelf life at room temperature (25°C, 90–95% RH). Measurements were taken on every 7 days of storage. Each value is the mean of three replicates ± standard error. Error bars with different small letters on the same storage period show a significant difference (P ≤ 0.05).
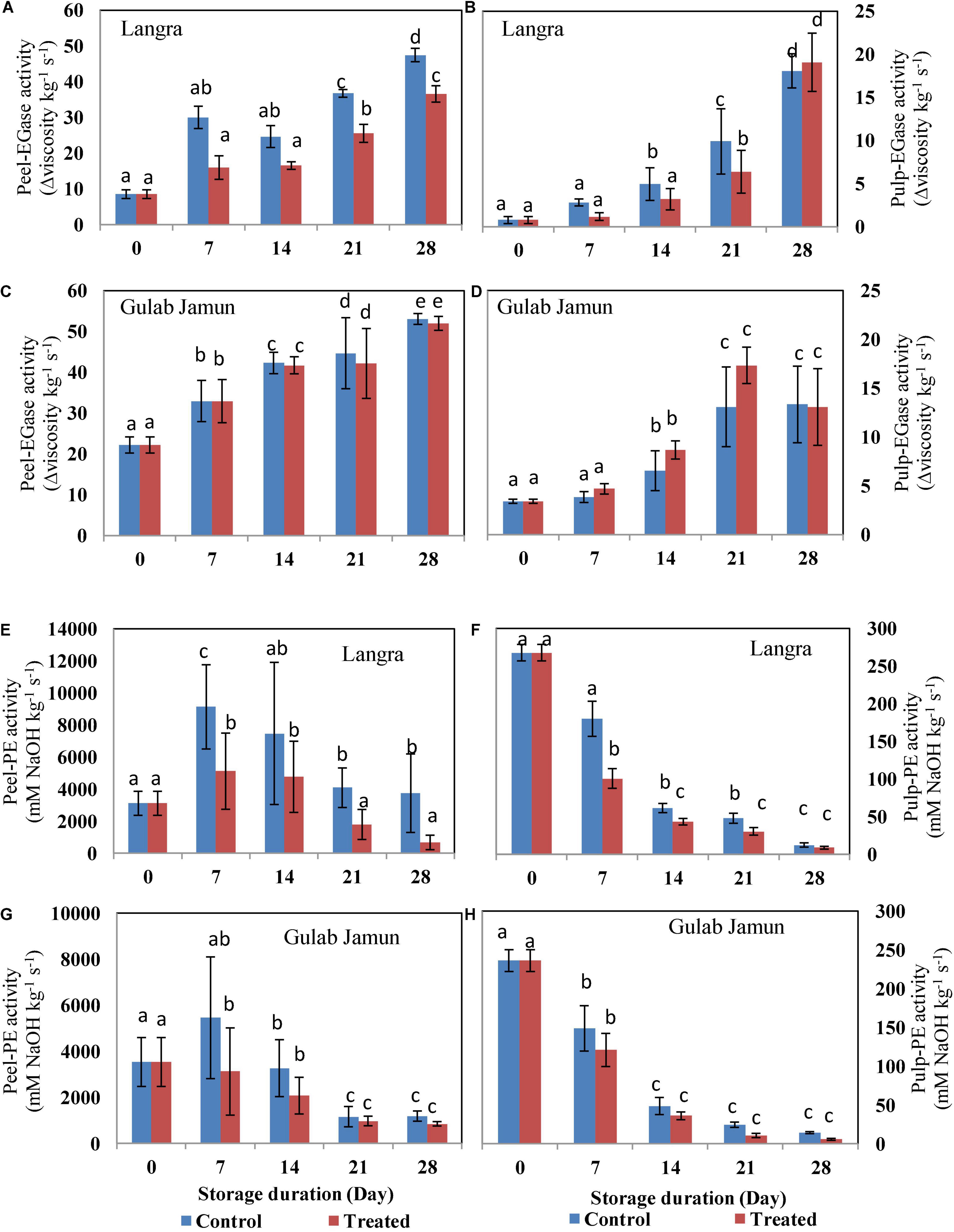
Figure 3. Endo-1,4-β-D-glucanase (EGase) activity in (A) ‘Langra’ peel, (B) ‘Langra’ pulp, (C) ‘Gulab Jamun’ peel, and (D) ‘Gulab Jamun’ pulp and pectin esterase (PE) activity in (E) ‘Langra’ peel, (F) ‘Langra’ pulp, (G) ‘Gulab Jamun’ peel, and (H) ‘Gulab Jamun’ pulp treated with 0 μM (control) or 100 μM (treated) MT for 2 h, followed by 28 days of storage at 5 ± 1°C and 3 days of shelf life at room temperature (25°C, 90–95% RH). Measurements were taken on every 7 days of storage. Each value is the mean of three replicates ± standard error. Error bars with different small letters on the same storage period show a significant difference (P ≤ 0.05).
Cell Membrane Deteriorating Enzymes Activity
Phospholipase (PLD) is a primary enzyme involved in phospholipid degradation and is thereby involved in the integrity and functioning of biomembranes during environmental stress. However, no definite trend was observed in the activity of the PLD enzyme throughout the duration of 28 days at low-temperature storage (Figures 4A–D). The activity of PLD in control fruit was higher than in MT-treated mango peel and pulp for both the ‘Langra’ (Figures 4A,B) and ‘Gulab Jamun’ (Figures 4C,D) cultivars. Lower LOX activity was observed in the peel and the pulp of MT-treated ‘Langra’ (Figures 4E,F) and ‘Gulab Jamun’ (Figures 4G,H) mangoes.
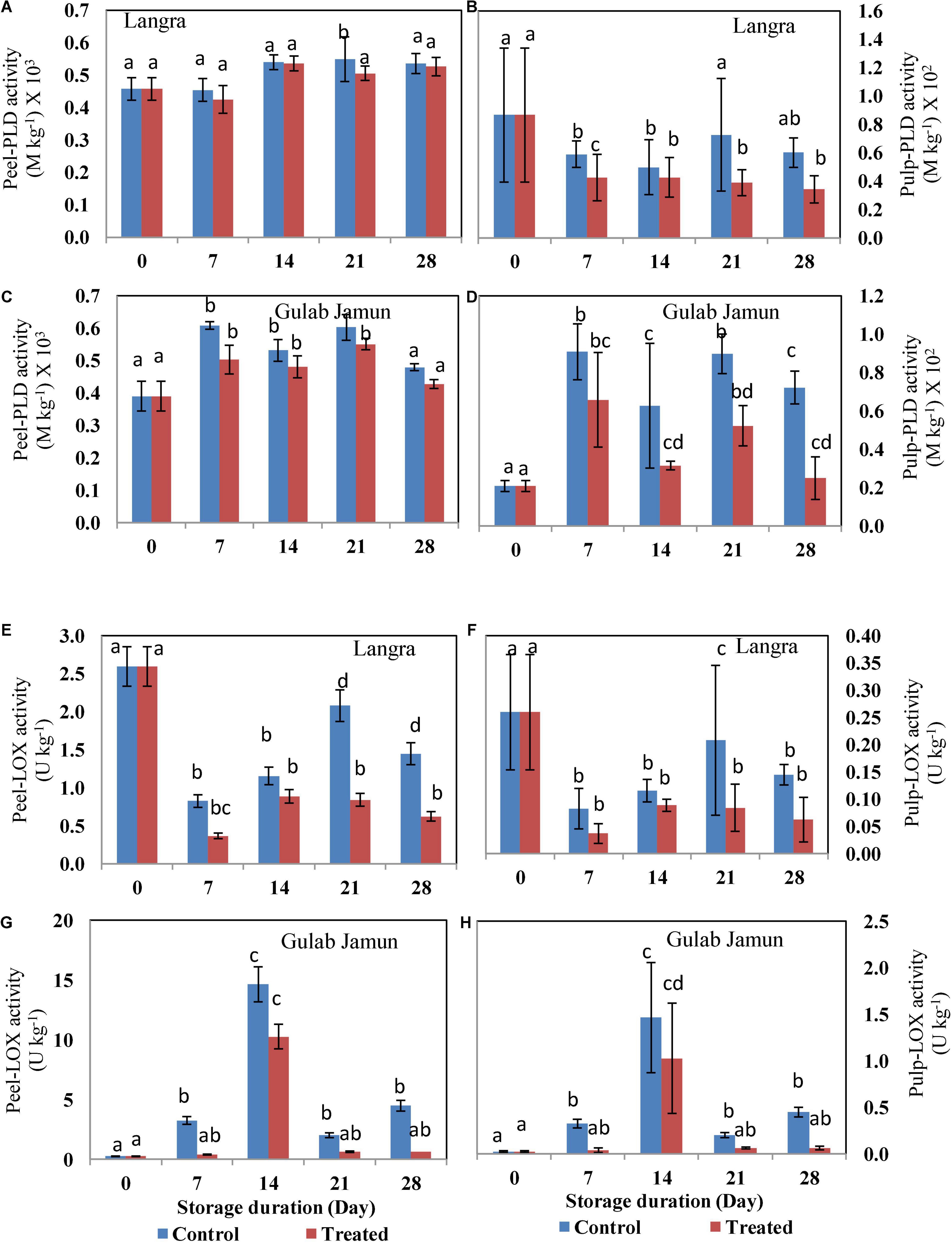
Figure 4. Phospholipase D (PLD) activity in (A) ‘Langra’ peel, (B) ‘Langra’ pulp, (C) ‘Gulab Jamun’ peel, and (D) ‘Gulab Jamun’ pulp and lipoxygenase (LOX) activity in (E) ‘Langra’ peel, (F) ‘Langra’ pulp, (G) ‘Gulab Jamun’ peel, and (H) ‘Gulab Jamun’ pulp treated with 0 μM (control) or 100 μM (treated) MT for 2 h, followed by 28 days of storage at 5 ± 1°C and 3 days of shelf life at room temperature (25°C, 90–95% RH). Measurements were taken on every 7 days of storage. Each value is the mean of three replicates ± standard error. Error bars with different small letters on the same storage period show a significant difference (P ≤ 0.05).
Membrane Unsaturation Status
Major amounts of five fatty acids were detected in the peel and pulp (Table 1) of mango fruit including two saturated fatty acids (palmitic acid and stearic acid) and three unsaturated fatty acids (palmitoleic acid, oleic acid, and linoleic acid). Palmitic acid and stearic acid showed rising trends (Table 1). However, fruit treated with MT had a lower accumulation of palmitic and stearic acids in both the peel and pulp of both cultivars with significant differences (p < 0.05) in ‘Langra’ mangoes. On the contrary, palmitoleic acid, oleic acid, and linoleic acid showed decreasing trends in ‘Langra’ mangoes in contrast to ‘Gulab Jamun’ mangoes. Additionally, ‘Langra’ mangoes treated with MT maintained significantly (p < 0.05) higher palmitoleic, oleic, and linoleic acid accumulation in their peel or pulp. Furthermore, the unsaturated to saturated fatty acid (unSFA/SFA) ratio was prominently higher in the peel and the pulp of MT-treated ‘Langra’ mangoes in contrast to ‘Gulab Jamun’ mangoes (Table 1). Therefore, treating mango fruit with MT may be advantageous in terms of unsaturated fatty acid maintenance during low temperature storage.
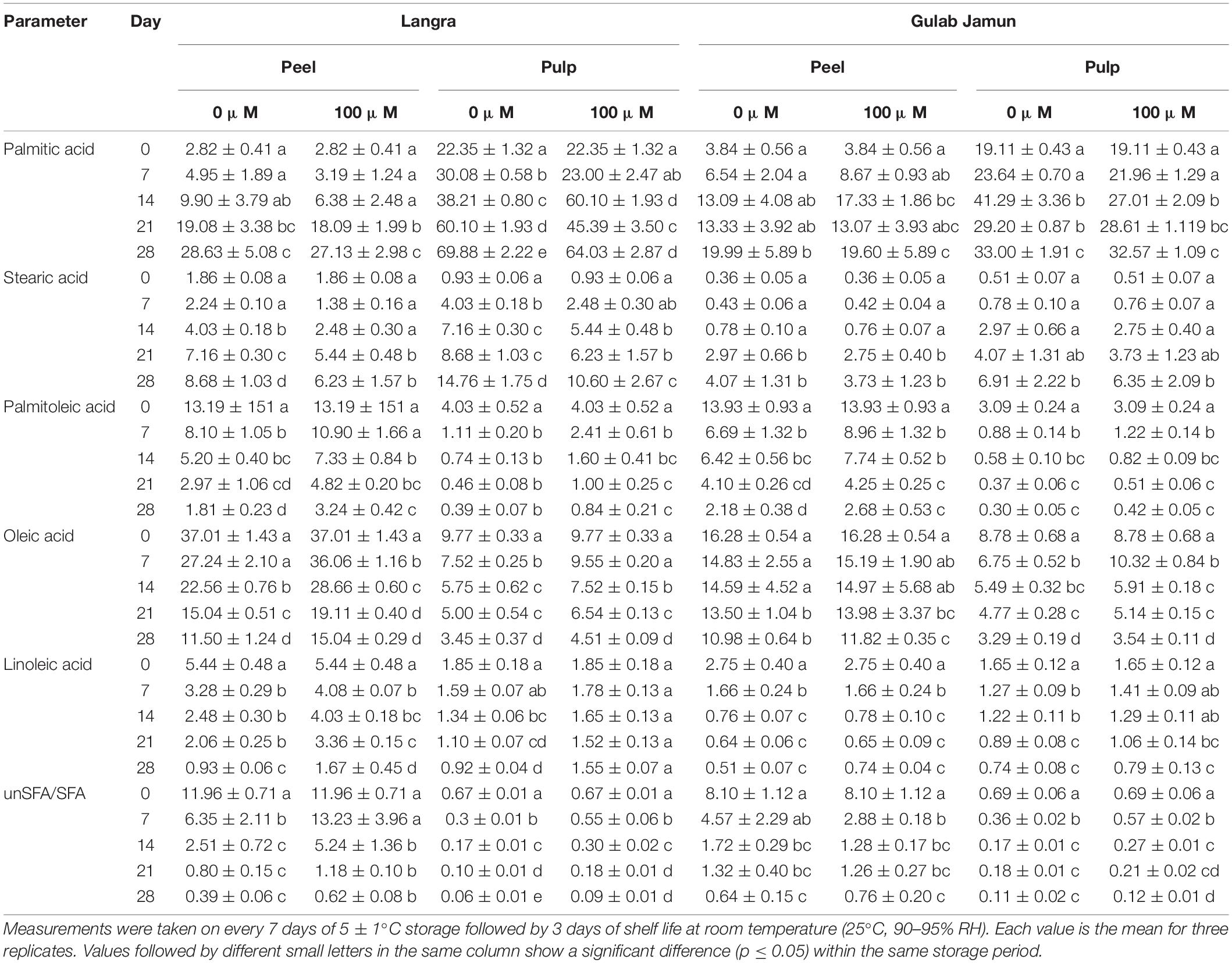
Table 1. Palmitic acid, stearic acid, palmitoleic acid, oleic acid, linoleic acid, and unsaturated/saturated fatty acid (USFA/SFA) in peel and pulp of ‘Langra’ and ‘Gulab Jamun’ mangoes treated with 0 μM (control) or 100 μM (treated) melatonin for 2 h followed by low temperature storage at 5 ± 1°C.
Energy Providing Enzyme Activities
The activity of Ca2+-ATPase for both the cultivars followed an uneven pattern throughout the 28-day duration of storage (Figures 5A–D). Exogenous application of MT maintained higher activity of Ca2+-ATPase in both peel and pulp of ‘Langra’ mangoes (Figures 5A,B). However, peel (Figure 5C) and pulp (Figure 5D) of ‘Gulab Jamun’ mangoes maintained higher Ca2+-ATPase activity only on day 7 of observation, afterward MT-treated fruit were observed to have lower Ca2+-ATPase activity. Similarly, an apparent rise in the activity of H+-ATPase with MT treatment was observed in the peel (Figure 5E) and pulp (Figure 5F) of ‘Langra’ mangoes; whereas, some inconsistent rise and fall were observed in the peel (Figure 5G) and pulp (Figure 5H) of ‘Gulab Jamun’ mangoes.
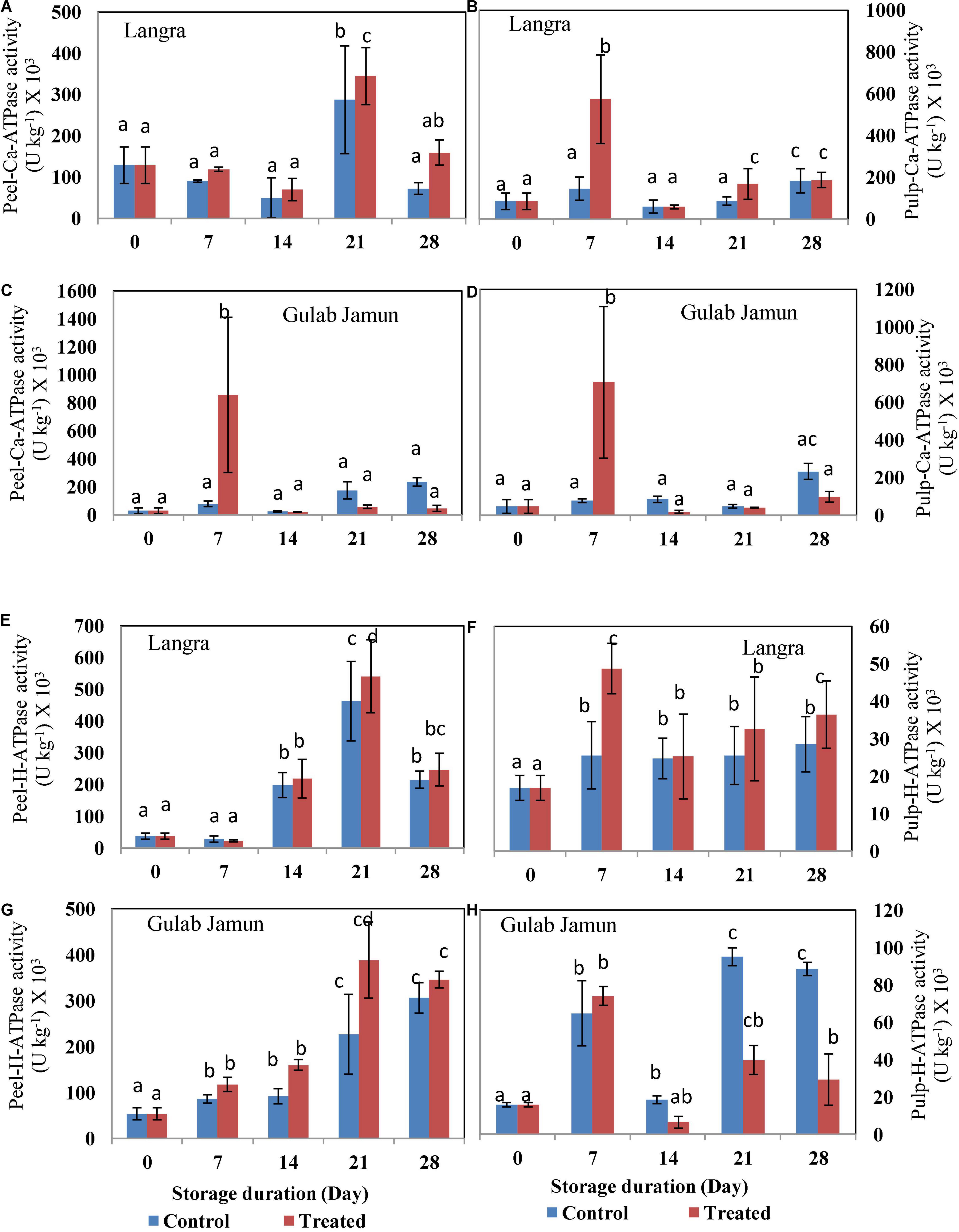
Figure 5. Ca2+-ATPase activity in (A) ‘Langra’ peel, (B) ‘Langra’ pulp, (C) ‘Gulab Jamun’ peel, and (D) ‘Gulab Jamun’ pulp and H+-ATPase activity in (E) ‘Langra’ peel, (F) ‘Langra’ pulp, (G) ‘Gulab Jamun’ peel and (H) ‘Gulab Jamun’ pulp treated with 0 μM (control) or 100 μM (treated) MT for 2 h, followed by 28 days of storage at 5 ± 1°C and 3 days of shelf life at room temperature (25°C, 90–95% RH). Measurements were taken on every 7 days of storage. Each value is the mean of three replicates ± standard error. Error bars with different small letters on the same storage period show a significant difference (P ≤ 0.05).
Intracellular Energy Status
The trend seen in ADP and ATP accumulation was one of decline over time while AMP accumulation increased as time progressed (Table 2). A significant difference (p < 0.05) was observed in the peel and pulp ADP and ATP accumulation of MT-treated ‘Langra’ mangoes only. However, a relatively higher AMP and AEC accumulation were noted with MT application in ‘Langra’ mangoes (Table 2). On the contrary to this, ‘Gulab Jamun’ mangoes did not exhibit evidence of any influence of MT application on the cellular energy status (i.e., AMP, ADP, ATP, and AEC; Table 2).
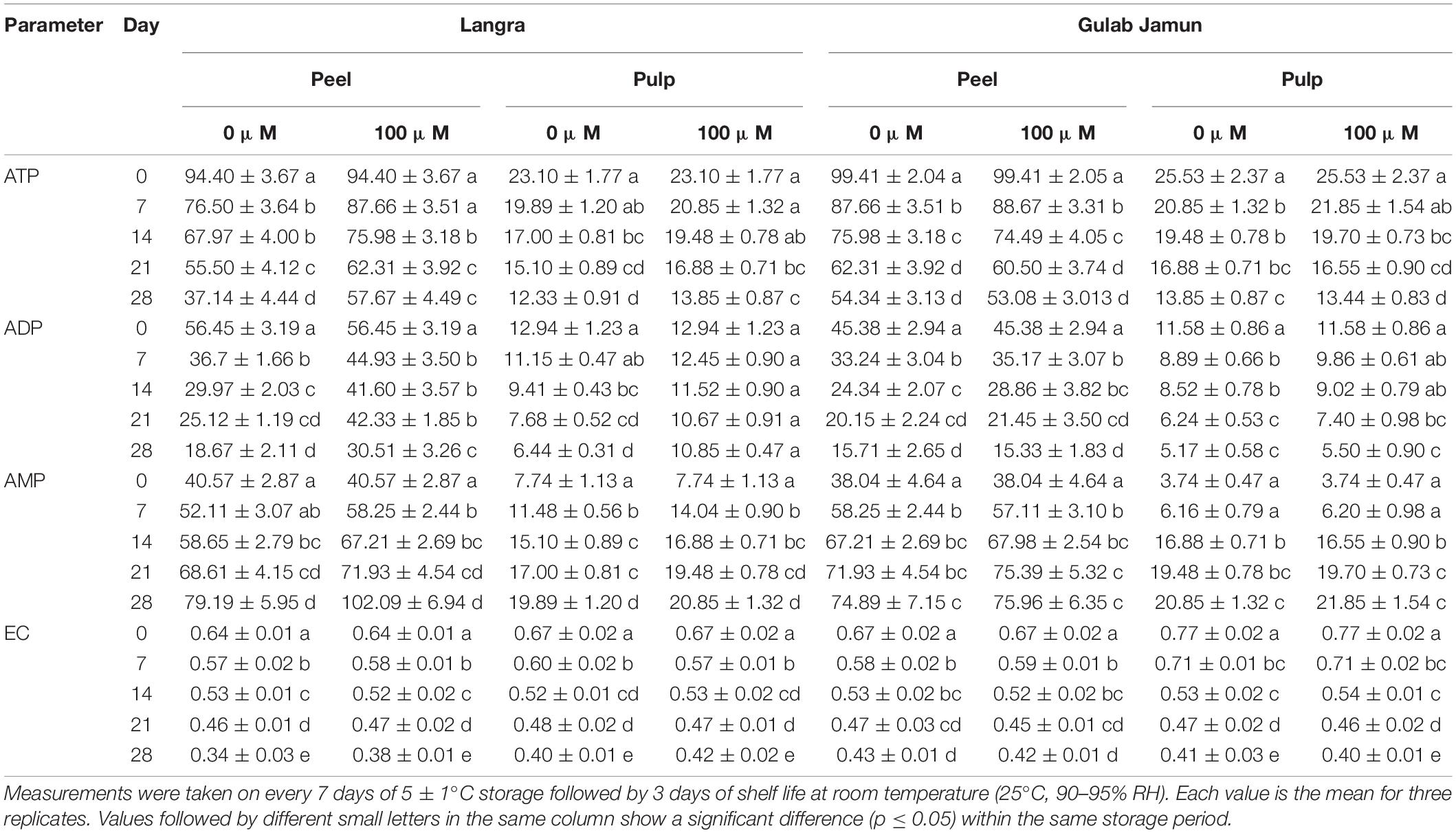
Table 2. Adenosine triphosphate (ATP), adenosine diphosphate (ADP), adenosine monophosphate (AMP), and adenylate energy charge (AEC) in peel and pulp of ‘Langra’ and ‘Gulab Jamun’ mangoes treated with 0 μM (control) or 100 μM (treated) melatonin for 2 h followed by low temperature storage at 5 ± 1°C.
Discussion
Due to the need for refrigeration to extend shelf life, the occurrence of CI is the major limiting factor that restricts the expansion of marketing for mango fruit. The CI reduction in ‘Langra’ mangoes with 100-μM MT application shown here is in line with previous studies done with peach (27), pomegranate (21), and tomato (22, 23). In accordance with the previous studies, the result of the present study has shown contrasting results for MT application in CI alleviation in cultivars of mango (Figure 1A). Here, ‘Langra’ mangoes have shown maximum CI alleviation with MT application, whereas ‘Gulab Jamun’ mangoes have not exhibited any influence of MT application. The contrasting behavior of ‘Langra’ and ‘Gulab Jamun’ mangoes may be because of differences in the hormonal, physiological, and molecular mechanisms that are responsible for CI occurrence (43).
We recently proposed that ethylene is the important factor that regulates CI in mango cultivars with MT treatment (7). Ethylene biosynthesis arises from higher ACC synthase (ACS) and ACC oxidase (ACO) gene expressions. During low temperature storage, ACS is suppressed, resulting in lower production of ethylene. However, when fruit was reconditioned at room temperature, a notable rise in ethylene production occurred due to subsequent ACC production, which led to severe signs of CI (43). The results of the present study depict the delayed rise in the climacteric peak of ethylene in concurrence with the respiration peak in ‘Langra’ mangoes in contrast to ‘Gulab Jamun’ mangoes (Supplementary Figure 1). The delayed climacteric peak of ethylene and respiration in MT-treated ‘Langra’ mangoes is supported by the study done with tomato fruit (44). The ‘Gulab Jamun’ mango ethylene pattern with MT application can be related to the banana fruit study done by He et al. (45). This contrasting ethylene behavior within the same species requires further investigation.
Furthermore, in addition to ethylene control of the ripening pattern, there is a synergistic effect on cell wall degrading enzymes that conclude with fruit softening (46). Higher ethylene biosynthesis and signaling promote cell wall degrading enzyme gene expression and enzyme activity that promotes fruit softening during cold storage (47). Insoluble cell wall polymer breakdown into soluble cellulose and pectin results in softening and, thereby, results in that aspect of ripening of fruit (48) through the cell wall modifying enzymes PG, PE, and EGase. With progressive ripening, pectin degradation is accomplished with the hydrolyzing action of PG on the pectic acid α-1,4 glycosidic bond. PG is classified as exo-PG and endo-PG on the basis of the mode of action. Exo-PG degrades pectic chains’ non-reducing free ends and endo-PG is responsible for random degradation of the pectic chain.
Internal sites on the glucan backbone of xyloglucan chains are the EGase enzyme action sites that lead to softening of fruit (49). We also observed that the rising activity of PG (exo-PG and endo-PG) and EGase lead to mango fruit softening in line with the studies done by Zaharah et al. (48) and Chourasia et al. (49)in mangoes. The present study highlights the efficacy of MT application in suppressing PG (Figure 2) activity in addition to higher maintenance of ‘Langra’ mangoes firmness (Figure 1B) and, thereby, delayed senescence with MT application at 5 ± 1°C. Similarly, the suppressed activity of EGase enzyme in peel (Figure 3A) and pulp (Figure 3B) of ‘Langra’ mangoes with the application of MT is consistent with the study done by Zaharah et al. (48), in which nitric oxide was shown to reduce EGase activity and also suppress ethylene production during ripening and, thereby, alleviate CI.
Whereas, demethylation of galacturonyl residues is accomplished through the action of the PE enzyme. In contrast to PG and EGase, the activity of PE (Figures 3E–H) followed a declining pattern over the period of storage. The probable reason for the declining trend of PE in relation to maintenance of firmness in MT-treated ‘Langra’ mangoes is because methyl group removal from polygalacturon will provide more binding sites for PE activity and, thereby, result in high calcium interaction, which would tend to strengthen the rigidity of cell walls (11). The inconsistency in mango cultivars as reported in the present study is the possible cause of CI variability among them. In ‘Langra’ mangoes treated with MT, lower ethylene and ABA biosynthesis accompanied by lower PG, PME, and β-Galactosidase enzyme activities could be responsible for delaying softening (11) and, henceforth, maintaining the firmness of fruit (Figure 1).
During cold storage, the NaCl transcription factor in green bell pepper fruit (50) and MYB21/MYB54 transcription factor in pear fruit (44) were responsible for triggering PLD gene expression and membrane phospholipids degradation. Moreover, higher ethylene signaling is represented by higher expression of ethylene response factor (ERF) genes (51). By triggering ethylene signaling in mango fruit during cold storage, phosphatidylinositol 3-kinase (PI3K) gene expression and enzyme activity could be responsible for supplying phosphatidylinositol 3-phosphate (PI3P) for PLD enzyme activity. PLD enzyme activity produces phosphatidic acid (PA), which could trigger cytosolic Ca2+ accumulation and promote NADPH oxidase enzyme activity (52–54). By promoting cytosolic Ca2+ accumulation, the triggering of calcium−dependent protein kinases (CDPKs) could be responsible for promoting shikimate and phenylpropanoid pathway activities for phenol accumulation, which could be responsible for pulp browning in mango fruit during the cold storage via polyphenol oxidase (PPO) enzyme activity (54). In addition, PA produced by PLD activity could trigger ethylene signaling by suppressing CTR1 activity, which serves as a negative regulator of ethylene signal transduction (53).
Along with PLD activity, lipolytic acyl-hydrolase (LAH) enzyme activity would provide free fatty acid substrate for the linolenic acid oxidation pathway via either LOX enzyme activity or non-enzymatic peroxidation by ROS, which would give rise to the malondialdehyde (MDA) accumulation (54, 55). The associated membrane deterioration could be responsible for the impedance of H+-ATPase and Ca2+-ATPase activity, which promotes PLD autocatalytic activity by cytosolic Ca2+ accumulation (53, 54). Therefore, maintenance of membrane integrity by employing safe and eco-friendly approaches such as MT application could be beneficial for the maintenance of the energy status of cells and, thereby, would be able to alleviate CI in fruits and vegetables during cold storage. Accordingly, as per the present study, exogenous application of MT to ‘Langra’ mangoes significantly (p < 0.05) reduced PLD activity (Figures 4A,B), LOX activity (Figures 4E,F), and MDA content (Supplementary Figure 2) in contrast to ‘Gulab Jamun’ mangoes.
Furthermore, maintenance of membrane fluidity due to higher membrane unSFA/SFA accumulation would be expected to result in the preservation of membrane integrity as represented by lower MDA accumulation. This would be accomplished by means of impeding PLD and LOX gene expressions and enzyme activities, which have been found to be beneficial for alleviating CI in tomato (23), pomegranate (21), and bell pepper fruits (31) treated with MT. Accordingly, the present work also manifests the effectiveness of 100-μM MT application in CI alleviation in ‘Langra’ mangoes in contrast to ‘Gulab Jamun’ mangoes (Figure 1A). The present discrepancy among the studied cultivars might be because of physiological, hormonal, and molecular mechanisms (43) that somehow regulate chilling tolerance. Conclusively, the MT-based suppression of CI was variable in both of the tested cultivars of mango fruit; whereas ‘Langra’ mangoes were found to better develop chilling tolerance by improving the unSFA/SFA ratio due to suppressed PLD (Figure 4) and LOX (Figure 4) activities, probably because of suppressed CaPLD and CaLOX gene expressions (31). This suppression of PLD and LOX in MT-treated ‘Langra’ mangoes was further evident by the significantly (p < 0.05) lower MDA accumulation (Supplementary Figure 2) (4), which is the end-product of lipid peroxidation.
The loss of antioxidant capacity is also notable with the progression of senescence and ripening, which further adds to the disintegration of membranes. In addition, membrane integrity deterioration during progressive ripening and senescence hampers mitochondrial electron transport system activity and disturbs cellular homeostasis. This situation could be responsible for higher ROS (O2–. and H2O2) accumulation, along with lower ATP biosynthesis (9). However, in the present study, the application of MT exhibited a positive response in the alleviation of ROS (Supplementary Figures 3, 4) and thereby was able to maintain antioxidant activity in ‘Langra’ mangoes (Supplementary Figures 5, 6) in contrast to ‘Gulab Jamun’ mangoes.
In addition to triggering membrane deterioration in blueberry fruit treated with nitric oxide, delaying softening could be ascribed to preserving membrane fluidity and integrity resulting from higher ATP-dependent fatty acid-biosynthezing acetyl-CoA carboxylase (ACCase), along with fatty acid unsaturation of FADs gene expressions concomitant with lower fatty acid-peroxidizing LOX gene expression and enzyme activity (56). In mango fruit treated with 6-benzylaminopurine, suppression of ethylene biosynthesis by lower ACS and ACO enzymes activity, prevention of O2– and H2O2 accumulation, and preservation of membrane integrity, as shown by lower MDA accumulation arising from lower PLD and LOX genes expression and enzymes activity, could be responsible for retarding fruit softening (57). In addition to preserving membrane integrity, supplying sufficient intracellular ATP could be beneficial for delaying softening via support of cell wall biosynthesis and reinforcement (58). During the postharvest period, intracellular ATP is the universal energy currency arising from intracellular ATP biosynthesis. ATP is produced in the glycolysis pathway, the tricarboxylic acid cycle, and the electron transport system, along with the GABA shunt pathway and the energy dissipating alternative oxidase (AOX). Uncoupling proteins (U) activity, energy signaling target of rapamycin (TOR), and sucrose non-fermenting-1 (SNF1)-related protein kinase 1 (SnRK1) kinases, accompanied by H+-ATPase, Ca2+-ATPase, and Mg2+-ATPase enzymes activity, are also responsible for alleviating stresses, delaying senescence, and preserving quality in fruits and vegetables during postharvest life (9).
Employing exogenous MT application has been beneficial for alleviating CI in tomato (23) and litchi (30) fruits by promoting intracellular ATP supply by means of promoting H+-ATPase, Ca2+-ATPase, SDH, and CCO enzyme activities. A similar alleviation with MT application is seen in the present study but with variability between two mango cultivars. This cultivar-dependent CI variability is in accordance with the difference in ATP and ADP accumulation, where ‘Langra’ mangoes treated with MT maintained the energy units in significantly (p < 0.05) higher amounts than ‘Gulab Jamun’ mangoes (Table 2). Furthermore, the higher energy status in MT-treated ‘Langra’ mangoes can be briefly explained by the higher activity of energy metabolizing enzymes, namely, H+-ATPase (Figures 5E–H) and Ca2+-ATPase (Figures 5A–D). The transmembrane potential through H+ transportation out from the cell is assisted by H+-ATPase, whereas Ca2+-ATPase, more properly Ca2+ pump, results in the production of ADP and phosphate due to ATP hydrolysis. Additionally, Ca2+-ATPase stabilizes the intracellular concentration of Ca2+ and thereby maintains the physiological functioning of the plant cells (9). Similarly, in the present work, the higher enzymatic activity of H+-ATPase and Ca2+-ATPase in MT-treated ‘Langra’ mangoes could be responsible for the better-maintained energy status; hence, the observed maximum chilling tolerance in the cultivar than ‘Gulab Jamun’ mangoes.
Exogenous MT application could be responsible for alleviating CI in mango fruit by preserving membrane integrity via suppression of PLD and LOX enzymes activity, protecting the intracellular energy supply via promotion of H+-ATPase, Ca2+-ATPase, SDH, and CCO activities, and preserving cell wall integrity via impeding increased of PG, PE, and EGase activities at the epigenetic level by DNA methylation (55, 56), transcriptional level by transcription factors NACs/MYBs (31, 44, 50), and miRNA (33), and post-translational SUMOylation via SUMO E3 ligase SIZ1 (59).
Conclusion
It can be concluded that chilling tolerance in mango is cultivar-dependent with some variability after pre-storage MT (100 μM) application. MT-treated mangoes of the ‘Langra’ cultivar were found to be CI-tolerant. This chilling tolerance with MT treatment in response to the chilling temperature of 5 ± 1°C in ‘Langra’ mangoes was found to be associated with lower activity of cell wall-modifying enzymes, better-maintained cell membrane, and unsaturated-to-saturated fatty acid ratio, in addition to maintenance of higher cellular energy status in both peel and pulp of the fruit. On the contrary, ‘Gulab Jamun’ mangoes failed to maintain the same preservative metabolisms neither in peel nor in pulp in response to MT application and there was no development of chilling tolerance.
Data Availability Statement
The raw data supporting the conclusions of this article will be made available by the authors, without undue reservation.
Author Contributions
RB and SP: conceptualization, methodology, investigation, and writing—original draft preparation. RB: software, validation, and formal analysis. SP: resources, visualization, supervision, and project administration. RB, SP, and MSA: data curation. SP, MSA, MBA, JB, and OF: writing—review and editing. SP and OF: funding acquisition. All authors have read and agreed to the submitted version of the manuscript.
Funding
This study was supported National Institute of Food Technology Entrepreneurship and Management (NIFTEM), Sonipat, Haryana funded the research and provided Infrastructural Facilities and the National Research Foundation of South Africa, Grant Number: 129295, partially supports the Open Access Article Processing Fee.
Conflict of Interest
The authors declare that the research was conducted in the absence of any commercial or financial relationships that could be construed as a potential conflict of interest.
Publisher’s Note
All claims expressed in this article are solely those of the authors and do not necessarily represent those of their affiliated organizations, or those of the publisher, the editors and the reviewers. Any product that may be evaluated in this article, or claim that may be made by its manufacturer, is not guaranteed or endorsed by the publisher.
Acknowledgments
We are thankful to Sarvanan Mani, Lab In-charge of Biochemistry, and Ankur Kumar, Lab In-charge of Central Instrumentation Laboratory, National Institute of Food Technology Entrepreneurship and Management (NIFTEM) for providing their constant support throughout the work. We are highly thankful to Ashutosh Upadhyay, J. S. Rana, and C. Sarvanan for reviewing the work and their suggestions. Additionally, we acknowledge the funding provided by NIFTEM, Kundli, India, and the National Research Foundation of South Africa (Grant Number: 129295).
Supplementary Material
The Supplementary Material for this article can be found online at: https://www.frontiersin.org/articles/10.3389/fnut.2022.936932/full#supplementary-material
References
1. Tharanathan R, Yashoda H, Prabha T. Mango (Mangifera indica L.),“The king of fruits”—an overview. Food Rev Int. (2006) 22:95–123. doi: 10.1080/87559120600574493
2. Ribeiro SMR, Schieber A. Bioactive compounds in mango (Mangifera indica L.). In: R Wattson, V Preedy editors. Bioactive Foods in Promoting Health. Amsterdam: Elsevier (2010). p. 507–23.
3. Ibarra-Garza IP, Ramos-Parra PA, Hernández-Brenes C, Jacobo-Velázquez DA. Effects of postharvest ripening on the nutraceutical and physicochemical properties of mango (Mangifera indica L. cv Keitt). Postharvest Biol Technol. (2015) 103:45–54. doi: 10.1016/j.postharvbio.2015.02.014
4. Bhardwaj R, Pareek S, González-Aguilar GA, Domínguez-Avila JA. Changes in the activity of proline-metabolizing enzymes is associated with increased cultivar-dependent chilling tolerance in mangos, in response to pre-storage melatonin application. Postharvest Biol Technol. (2021) 182:111702. doi: 10.1016/j.postharvbio.2021.111702
5. Bhardwaj R, Pareek S, Saravanan C, Yahia EM. Contribution of pre-storage melatonin application to chilling tolerance of some mango fruit cultivars and relationship with polyamines metabolism and γ-aminobutyric acid shunt pathway. Environ Exp Bot. (2022) 194:104691. doi: 10.1016/j.envexpbot.2021.104691
6. Bhardwaj R, Pareek S, Domínguez-Avila JA, González-Aguilar GA, Valero D, Serrano M. An exogenous pre-storage melatonin alleviates chilling injury in some mango fruit cultivars, by acting on the enzymatic and non-anzymatic antioxidant system. Antioxidants. (2022) 11:384. doi: 10.3390/antiox11020384
7. Bhardwaj R, Pareek S, Mani S, Domínguez-Avila JA, González-Aguilar GA. A melatonin treatment delays postharvest senescence, maintains quality, reduces chilling injury, and regulates antioxidant metabolism in mango fruit. J Food Qual. (2022) 2022:2359556. doi: 10.1155/2022/2379556
8. Aghdam MS, Bodbodak S. Physiological and biochemical mechanisms regulating chilling tolerance in fruits and vegetables under postharvest salicylates and jasmonates treatments. Sci Hortic. (2013) 156:73–85. doi: 10.1016/j.scienta.2013.03.028
9. Aghdam MS, Jannatizadeh A, Luo Z, Paliyath G. Ensuring sufficient intracellular ATP supplying and friendly extracellular ATP signaling attenuates stresses, delays senescence and maintains quality in horticultural crops during postharvest life. Trends Food Sci Technol. (2018) 76:67–81. doi: 10.1016/j.tifs.2018.04.003
10. Cao S, Bian K, Shi L, Chung HH, Chen W, Yang Z. Role of melatonin in cell-wall disassembly and chilling tolerance in cold-stored peach fruit. J Agric Food Chem. (2018) 66:5663–70. doi: 10.1021/acs.jafc.8b02055
11. Liu S, Huang H, Huber DJ, Pan Y, Shi X, Zhang Z. Delay of ripening and softening in ‘Guifei’ mango fruit by postharvest application of melatonin. Postharvest Biol Technol. (2020) 163:111136. doi: 10.1016/j.postharvbio.2020.111136
12. Tang Q, Li C, Ge Y, Li X, Cheng Y, Hou J, et al. Exogenous application of melatonin maintains storage quality of jujubes by enhancing anti-oxidative ability and suppressing the activity of cell wall-degrading enzymes. LWT Food Sci Technol. (2020) 127:109431. doi: 10.1016/j.lwt.2020.109431
13. Zhai R, Liu J, Liu F, Zhao Y, Liu L, Fang C, et al. Melatonin limited ethylene production, softening and reduced physiology disorder in pear (Pyrus communis L.) fruit during senescence. Postharvest Biol Technol. (2018) 139:38–46. doi: 10.1016/j.postharvbio.2018.01.017
14. Aghdam MS, Mukherjee S, Flores FB, Arnao MB, Luo Z, Corpas FJ. Functions of melatonin during postharvest of horticultural crops. Plant Cell Physiol. (2021) 2021:cab175. doi: 10.1093/pcp/pcab175
15. Arnao MB, Hernandez-Ruiz J. Melatonin: a new plant hormone and/or a plant master regulator? Trends Plant Sci. (2019) 24:38–48. doi: 10.1016/j.tplants.2018.10.010
16. Arnao MB, Cano A, Hernandez-Ruiz J. Phytomelatonin: an unexpected molecule with amazing performances in plants. J Exp Bot. (2022):erac009 [Online ahead of print]. doi: 10.1093/jxb/erac009
17. Sagar NA, Pareek S. Safe storage and preservation techniques in commercialized agriculture. In: C Egbuna, B Sawicka editors. Natural Remedies for Pest, Disease and Weed Control. Amsterdam: Elsevier (2019). p. 221–34.
18. Arnao MB, Hernandez-Ruiz J. Melatonin in flowering, fruit set and fruit ripening. Plant Reprod. (2020) 33:77–87. doi: 10.1007/s00497-020-00388-8
19. Hernandez-Ruiz J, Ruiz-Cano D, Giraldo-Acosta M, Cano A, Arnao MB. Melatonin in Brassicaceae: role in postharvest and interesting phytochemicals. Molecules. (2022) 27:1523. doi: 10.3390/molecules27051523
20. Aghdam MS, Luo Z, Li L, Jannatizadeh A, Fard JR, Pirzad F. Melatonin treatment maintains nutraceutical properties of pomegranate fruits during cold storage. Food Chem. (2020) 303:125385. doi: 10.1016/j.foodchem.2019.125385
21. Jannatizadeh A. Exogenous melatonin applying confers chilling tolerance in pomegranate fruit during cold storage. Sci Hortic. (2019) 246:544–9. doi: 10.1016/j.scienta.2018.11.027
22. Aghdam MS, Luo Z, Jannatizadeh A, Sheikh-Assadi M, Sharafi Y, Farmani B, et al. Employing exogenous melatonin applying confers chilling tolerance in tomato fruits by upregulating ZAT2/6/12 giving rise to promoting endogenous polyamines, proline, and nitric oxide accumulation by triggering arginine pathway activity. Food Chem. (2019) 275:549–56. doi: 10.1016/j.foodchem.2018.09.157
23. Jannatizadeh A, Aghdam MS, Luo Z, Razavi F. Impact of exogenous melatonin application on chilling injury in tomato fruits during cold storage. Food Bioprocess Technol. (2019) 12:741–50. doi: 10.1007/s11947-019-2247-1
24. Sharafi Y, Aghdam MS, Luo Z, Jannatizadeh A, Razavi F, Fard JR, et al. Melatonin treatment promotes endogenous melatonin accumulation and triggers GABA shunt pathway activity in tomato fruits during cold storage. Sci Hortic. (2019) 254:222–7. doi: 10.1016/j.scienta.2019.04.056
25. Cao S, Shao J, Shi L, Xu L, Shen Z, Chen W, et al. Melatonin increases chilling tolerance in postharvest peach fruit by alleviating oxidative damage. Sci Rep. (2018) 8:806. doi: 10.1038/s41598-018-19363-5
26. Cao S, Song C, Shao J, Bian K, Chen W, Yang Z. Exogenous melatonin treatment increases chilling tolerance and induces defense response in harvested peach fruit during cold storage. J Agric Food Chem. (2016) 64:5215–22. doi: 10.1021/acs.jafc.6b01118
27. Gao H, Lu Z, Yang Y, Wang D, Yang T, Cao M, et al. Melatonin treatment reduces chilling injury in peach fruit through its regulation of membrane fatty acid contents and phenolic metabolism. Food Chem. (2018) 245:659–66. doi: 10.1016/j.foodchem.2017.10.008
28. Madebo MP, Luo S-M, Wang L, Zheng Y-H, Jin P. Melatonin treatment induces chilling tolerance by regulating the contents of polyamine, γ-aminobutyric acid, and proline in cucumber fruit. J Integr Agric. (2021) 20:3060–74. doi: 10.1016/s2095-3119(20)63485-2
29. Liu J, Sun J, Pan Y, Yun Z, Zhang Z, Jiang G, et al. Endogenous melatonin generation plays a positive role in chilling tolerance in relation to redox homeostasis in litchi fruit during refrigeration. Postharvest Biol Technol. (2021) 178:111554. doi: 10.1016/j.postharvbio.2021.111554
30. Wang T, Hu M, Yuan D, Yun Z, Gao Z, Su Z, et al. Melatonin alleviates pericarp browning in litchi fruit by regulating membrane lipid and energy metabolisms. Postharvest Biol Technol. (2020) 160:111066. doi: 10.1016/j.postharvbio.2019.111066
31. Kong X-M, Ge W-Y, Wei B-D, Zhou Q, Zhou X, Zhao Y-B, et al. Melatonin ameliorates chilling injury in green bell peppers during storage by regulating membrane lipid metabolism and antioxidant capacity. Postharvest Biol Technol. (2020) 170:111315. doi: 10.1016/j.postharvbio.2020.111315
32. Du H, Liu G, Hua C, Liu D, He Y, Liu H, et al. Exogenous melatonin alleviated chilling injury in harvested plum fruit via affecting the levels of polyamines conjugated to plasma membrane. Postharvest Biol Technol. (2021) 179:111585. doi: 10.1016/j.postharvbio.2021.111585
33. Wang Z, Pu H, Shan S, Zhang P, Li J, Song H, et al. Melatonin enhanced chilling tolerance and alleviated peel browning of banana fruit under low temperature storage. Postharvest Biol Technol. (2021) 179:111571. doi: 10.1016/j.postharvbio.2021.111571
34. Concellón A, Añón MC, Chaves AR. Effect of chilling on ethylene production in eggplant fruit. Food Chem. (2005) 92:63–9. doi: 10.1016/j.foodchem.2004.04.048
35. Khan AS, Singh Z. 1-MCP regulates ethylene biosynthesis and fruit softening during ripening of ‘Tegan Blue’ plum. Postharvest Biol Technol. (2007) 43:298–306. doi: 10.1016/j.postharvbio.2006.10.005
36. Liu H, Song L, You Y, Li Y, Duan X, Jiang Y, et al. Cold storage duration affects litchi fruit quality, membrane permeability, enzyme activities and energy charge during shelf time at ambient temperature. Postharvest Biol Technol. (2011) 60:24–30. doi: 10.1016/j.postharvbio.2010.11.008
37. Lin Y, Lin H, Lin Y, Zhang S, Chen Y, Jiang X. The roles of metabolism of membrane lipids and phenolics in hydrogen peroxide-induced pericarp browning of harvested longan fruit. Postharvest Biol Technol. (2016) 111:53–61. doi: 10.1016/j.postharvbio.2015.07.030
38. Cao S, Zheng Y, Wang K, Jin P, Rui H. Methyl jasmonate reduces chilling injury and enhances antioxidant enzyme activity in postharvest loquat fruit. Food Chem. (2009) 115:1458–63. doi: 10.1016/j.foodchem.2009.01.082
39. Mirdehghan S, Rahemi M, Martínez-Romero D, Guillén F, Valverde J, Zapata P, et al. Reduction of pomegranate chilling injury during storage after heat treatment: role of polyamines. Postharvest Biol Technol. (2007) 44:19–25. doi: 10.1016/j.postharvbio.2006.11.001
40. Jin P, Zhu H, Wang J, Chen J, Wang X, Zheng Y. Effect of methyl jasmonate on energy metabolism in peach fruit during chilling stress. J Sci Food Agric. (2013) 93:1827–32. doi: 10.1002/jsfa.5973
41. Yi C, Qu HX, Jiang YM, Shi J, Duan XW, Joyce DC, et al. ATP-induced changes in energy status and membrane integrity of harvested litchi fruit and its relation to pathogen resistance. J Phytopath. (2008) 156:365–71. doi: 10.1111/j.1439-0434.2007.01371.x
42. Bradford MM. A rapid and sensitive method for the quantitation of microgram quantities of protein utilizing the principle of protein-dye binding. Anal Biochem. (1976) 72:248–54. doi: 10.1006/abio.1976.9999
43. Sevillano L, Sanchez−Ballesta MT, Romojaro F, Flores FB. Physiological, hormonal and molecular mechanisms regulating chilling injury in horticultural species. Postharvest technologies applied to reduce its impact. J Sci Food Agric. (2009) 89:555–73.
44. Sun H-J, Luo M-L, Zhou X, Zhou Q, Sun Y-Y, Ge W-Y, et al. PuMYB21/PuMYB54 coordinate to activate PuPLDβ1 transcription during peel browning of cold-stored “Nanguo” pears. Hortic Res. (2020) 7:136. doi: 10.1038/s41438-020-00356-3
45. He X, Li L, Sun J, Li C, Sheng J, Zheng F, et al. Adenylate quantitative method analyzing energy change in postharvest banana (Musa acuminate L.) fruits stored at different temperatures. Sci Hortic. (2017) 219:118–24. doi: 10.1016/j.scienta.2017.02.050
46. Chen H, Cao S, Fang X, Mu H, Yang H, Wang X, et al. Changes in fruit firmness, cell wall composition and cell wall degrading enzymes in postharvest blueberries during storage. Sci Hortic. (2015) 188:44–8. doi: 10.1016/j.scienta.2015.03.018
47. Zhu Y, Wang K, Wu C, Zhao Y, Yin X, Zhang B, et al. Effect of ethylene on cell wall and lipid metabolism during alleviation of postharvest chilling injury in peach. Cells. (2019) 8:1612. doi: 10.3390/cells8121612
48. Zaharah S, Singh Z. Mode of action of nitric oxide in inhibiting ethylene biosynthesis and fruit softening during ripening and cool storage of ‘Kensington Pride’ mango. Postharvest Biol Technol. (2011) 62:258–66. doi: 10.1016/j.postharvbio.2011.06.007
49. Chourasia A, Sane VA, Singh RK, Nath P. Isolation and characterization of the MiCel1 gene from mango: ripening related expression and enhanced endoglucanase activity during softening. Plant Growth Regul. (2008) 56:117–27. doi: 10.1007/s10725-008-9292-5
50. Kong X-M, Zhou Q, Zhou X, Wei B-D, Ji S-J. Transcription factor CaNAC1 regulates low-temperature-induced phospholipid degradation in green bell pepper. J Exp Bot. (2020) 71:1078–91. doi: 10.1093/jxb/erz463
51. Patil AS, Maurer D, Feygenberg O, Alkan N. Exploring cold quarantine to mango fruit against fruit fly using artificial ripening. Sci Rep. (2019) 9:1948. doi: 10.1038/s41598-019-38521-x
52. Aghdam MS, Razavi F. Octapeptide NOP-1 treatment delays yellowing in broccoli floret during low temperature storage. Postharvest Biol Technol. (2021) 180:111628.
53. Padmanabhan P, Paliyath G. Ethylene signal transduction during fruit ripening and senescence. In: G Paliyath, J Subramanian, L-T Lim, KS Subramanian, AK Handa, AK Mattoo editors. Postharvest Biology and Nanotechnology. Hoboken, NJ: Wiley-Blackwell (2018). p. 53–87.
54. Sivankalyani V, Sela N, Feygenberg O, Zemach H, Maurer D, Alkan N. Transcriptome dynamics in mango fruit peel reveals mechanisms of chilling stress. Front Plant Sci. (2016) 7:1579. doi: 10.3389/fpls.2016.01579
55. Sivankalyani V, Maoz I, Feygenberg O, Maurer D, Alkan N. Chilling stress upregulates α-linolenic acid-oxidation pathway and induces volatiles of C6 and C9 aldehydes in mango fruit. J Agric Food Chem. (2017) 65:632–8. doi: 10.1021/acs.jafc.6b04355
56. Dai H, Ji S, Zhou X, Wei B, Cheng S, Zhang F, et al. Postharvest effects of sodium nitroprusside treatment on membrane fatty acids of blueberry (Vaccinium corymbosum, cv. Bluecrop) fruit. Sci Hortic. (2021) 288:110307. doi: 10.1016/j.scienta.2021.110307
57. Zhang Y, Gao Z, Hu M, Pan Y, Xu X, Zhang Z. Delay of ripening and senescence in mango fruit by 6-benzylaminopurine is associated with inhibition of ethylene biosynthesis and membrane lipid catabolism. Postharvest Biol Technol. (2022) 185:111797. doi: 10.1016/j.postharvbio.2021.111797
58. Wang H, Cheng X, Wu C, Fan G, Li T, Dong C. Retardation of postharvest softening of blueberry fruit by methyl jasmonate is correlated with altered cell wall modification and energy metabolism. Sci Hortic. (2021) 276:109752.
Keywords: cell wall-degrading, cold storage, intracellular energy, Mangifera indica, membrane integrity
Citation: Bhardwaj R, Aghdam MS, Arnao MB, Brecht JK, Fawole OA and Pareek S (2022) Melatonin Alleviates Chilling Injury Symptom Development in Mango Fruit by Maintaining Intracellular Energy and Cell Wall and Membrane Stability. Front. Nutr. 9:936932. doi: 10.3389/fnut.2022.936932
Received: 05 May 2022; Accepted: 02 June 2022;
Published: 30 June 2022.
Edited by:
Fabián Guillén, Miguel Hernández University of Elche, SpainReviewed by:
Zhenfeng Yang, Zhejiang Wanli University, ChinaDomingo Martínez-Romero, Miguel Hernández University of Elche, Spain
Copyright © 2022 Bhardwaj, Aghdam, Arnao, Brecht, Fawole and Pareek. This is an open-access article distributed under the terms of the Creative Commons Attribution License (CC BY). The use, distribution or reproduction in other forums is permitted, provided the original author(s) and the copyright owner(s) are credited and that the original publication in this journal is cited, in accordance with accepted academic practice. No use, distribution or reproduction is permitted which does not comply with these terms.
*Correspondence: Olaniyi Amos Fawole, b2xhbml5aUB1ai5hYy56YQ==; Sunil Pareek, c3VuaWxfY2lhaEB5YWhvby5jby5pbg==