- Chongqing Key Laboratory of Nutrition and Food Safety, Research Center for Nutrition and Food Safety, Chongqing Medical Nutrition Research Center, Institute of Military Preventive Medicine, Army Medical University (Third Military Medical University), Chongqing, China
Mounting evidence suggested that high loading intensity of exercise might be detrimental to human health, especially the gastrointestinal tract. Pterostilbene (PTE), derived from grapes and blueberries, might reach a high concentration of intestinal contents. Our study aimed to evaluate PTE’s ability to prevent the loss of intestinal epithelial barrier in high loading intensity of exercise. The exercise model was established by the forced running of mice. An effective HPLC-UV method was developed to quantify PTE concentration in intestinal content. The mRNA changes were detected by quantitative polymerase chain reaction (qPCR). The structure of intestinal flora was analyzed by 16S rRNA sequencing. The PTE (100 mg/kg/d) could significantly attenuate exercise-induced intestinal epithelial barrier loss. Moreover, the HPLC-UV assay showed that the PTE concentration of intestinal content could last 12 h. Furthermore, the exercise increased the abundance of Alistipes, which was related to lipopolysaccharide (LPS) production but could not be reversed by PTE intervention. Besides, cell experiments showed that PTE could promote the expression of intestinal epithelial tight junction (TJ) molecules in vitro. In conclusion, PTE has a significant interest in preventing exercise-induced intestinal damage.
Introduction
It is a known fact that moderate exercise can prevent and cure various metabolic diseases and enhance immunity (1–3). Otherwise, the sustained high loading intensity of exercise, which exceeds 60% maximal oxygen uptake (VO2 max) or 70% of maximum heart rate reserve, such as a 50-km forced march, marathon, or triathlon, may cause many health hazards to the body (4, 5). High loading intensity of exercise causes metabolic disorder of skeletal muscle, leading to repetitive tissue micro-trauma of muscle, connective tissue, bone structure, and chronic inflammation (6). In severe cases, some studies show that 86% of athletes have severe gastrointestinal syndrome (GIS) (7).
The intestinal flora plays an essential role in host physiology and health. Mounting evidence suggests that exercise could alter the structure of intestinal flora (8, 9) and is a crucial modulator of intestinal flora (10). Besides, recent evidence shows that stress during exercise is highly related to the changes in the intestinal flora (11). However, there is no direct evidence that high loading intensity of exercise causes dysbiosis of intestinal flora. The effect of high loading intensity of exercise on intestinal flora is still unknown. Therefore, our study aimed to explore the effect of high loading intensity of exercise on intestinal flora.
The intestinal barrier has become a focus of biomedical research, divided into chemical, mechanical, biological, and immune barriers according to their functions (12, 13). The mechanical barrier is composed of occludin, claudins, and zonula occluden (14, 15), which could prevent lipopolysaccharide (LPS) from transferring into serum (13). The LPS could cause harmful inflammatory reactions. LPS could cause TJ dysfunction through the activation of the immune system or the inflammatory process, closely associated with the initiation or development of intestinal diseases (16). Besides, LPS could cause altered membrane permeability, through the disruption or relocation of tight junction (TJ) proteins, following redox-sensitive mitogen-activated protein kinases (MAPKs) modulation (17). A growing body of research indicates that high loading intensity of exercise could cause intestinal epithelial barrier damage (18–20). As for athletes, the sustained high loading intensity of exercise training is frequent (7), and barrier integrity is vital for athletes to prevent LPS from transferring into the serum to avoid GIS. Therefore, a new strategy for preventing intestinal barrier damage from high loading intensity of exercise is required for athletes.
Given the crucial role of the practical application for athletes, dietary approaches are preferentially recommended to prevent intestinal barrier loss (21). In this sense, natural bioactive substances such as plant polyphenols are emerging as sports nutrition supplements for GIS prevention (22, 23). Research studies have recently indicated that a Mediterranean diet containing a high proportion of polyphenolic compounds (24) could significantly prevent intestinal barrier dysfunction (25). The phenolic compounds such as pterostilbene (PTE) are widely reported and rooted in blueberries and grapes (26). Mounting evidence has further proved that PTE and its metabolites could improve the alteration in epithelial permeability induced by LPS (17). PTE might slow LPS induced transepithelial electrical resistance decrease, preserve TJ proteins levels, and reduce MAPK phosphorylation to attenuate alteration of epithelial permeability. Furthermore, some studies find that PTE protects the intestinal epithelial barrier through the NF-κBMLCK/p-MLC signal pathway in mice (27). Besides, PTE could attenuate oxidative stress-induced intestinal injury by improving mitochondrial redox homeostasis (28). However, the protective role of PTE in exercise-induced intestinal barrier damage remains unclear.
In this study, we attempted to investigate the effect of PTE against damage induced by high loading intensity of exercise. Our findings revealed that exercise caused the intestinal epithelial barrier loss by altering intestinal flora’s structure. Otherwise, the intervention of PTE (100 mg/kg/d) could promote the expression of intestinal epithelial TJ molecules to prevent intestinal damage.
Materials and methods
Chemicals and reagents
The LPS and 4′,6-diamidino-2-phenylindole (DAPI) were purchased from Sigma (St. Louis, MO, United States). PTE (≥ 98% purity analyzed by HPLC) was obtained from Chengdu Must Bio-Technology. Occludin antibodies were purchased from Proteintech (Chicago, United States). The 3,5,4′,-trimethoxy-trans-stilbene (TMS) was obtained from Solarbio (Beijing, China). The penicillin–streptomycin was purchased from Beyotime (Shanghai, China). The fetal bovine serum was obtained from HyClone (Logan, UT, United States).
Experimental animals and design
The C57BL/6 mice (7 weeks, male) weighing 20–22 g were obtained from the Laboratory Animal Centre of the Army Medical University (Chongqing, China) and housed in a controlled environment (22–25°C, 50–55%). They were provided standard food (D12450B; 10% fat, 70% carbohydrate, 20% protein) and obtained water freely (29). The body weight and food intake of mice were weighed every day. All animal experiments described herein followed the National Research Council Guidelines, approved by the Animal Care and Use Committee of the Army Medical University. The experimental design is shown in Figure 1A and followed the National Research Council Guidelines. Animal experiment 1: the C57BL/6 mice (n = 24) were randomly distributed into six groups (n = 4/group), gavaged with pterostilbene (100 mg/kg/day) (30) at 9:00 a.m. and sacrificed at 0, 2, 6, 10, 12, and 24 h after intragastric administration, respectively. The contents of small intestine and colon were collected and stored at −80°C. Animal experiment 2: mice (n = 48) were randomly divided into four groups (n = 12/group): control group (CON), pterostilbene group (PTE), exercise group (EX), and exercise with pterostilbene group (EX + PTE). The experiment lasted for 2 weeks. In the first week, mice ran on the treadmill at a speed of 15 m/min for 10 min one time a day and rested for the weekend on a motorized treadmill (SANS Biological Technology, Jiangsu, China). In the second week, referred to Bedford’s method (31, 32), mice in exercise groups ran at the speed of 25 m/min until exhaustion one time a day for 7 days, and the exhaustion statue according to the literature reported (33). The PTE and EX + PTE groups were orally gavaged with pterostilbene (100 mg/kg/day) for 1 week, as previous study reported (30). The PTE was administered 12 h before exercise, and the mice of the EX and EX + PTE groups were sacrificed 1 h after the last exercise session. Finally, the serum, small intestine, cecum, and colon contents were removed and stored at −80°C.
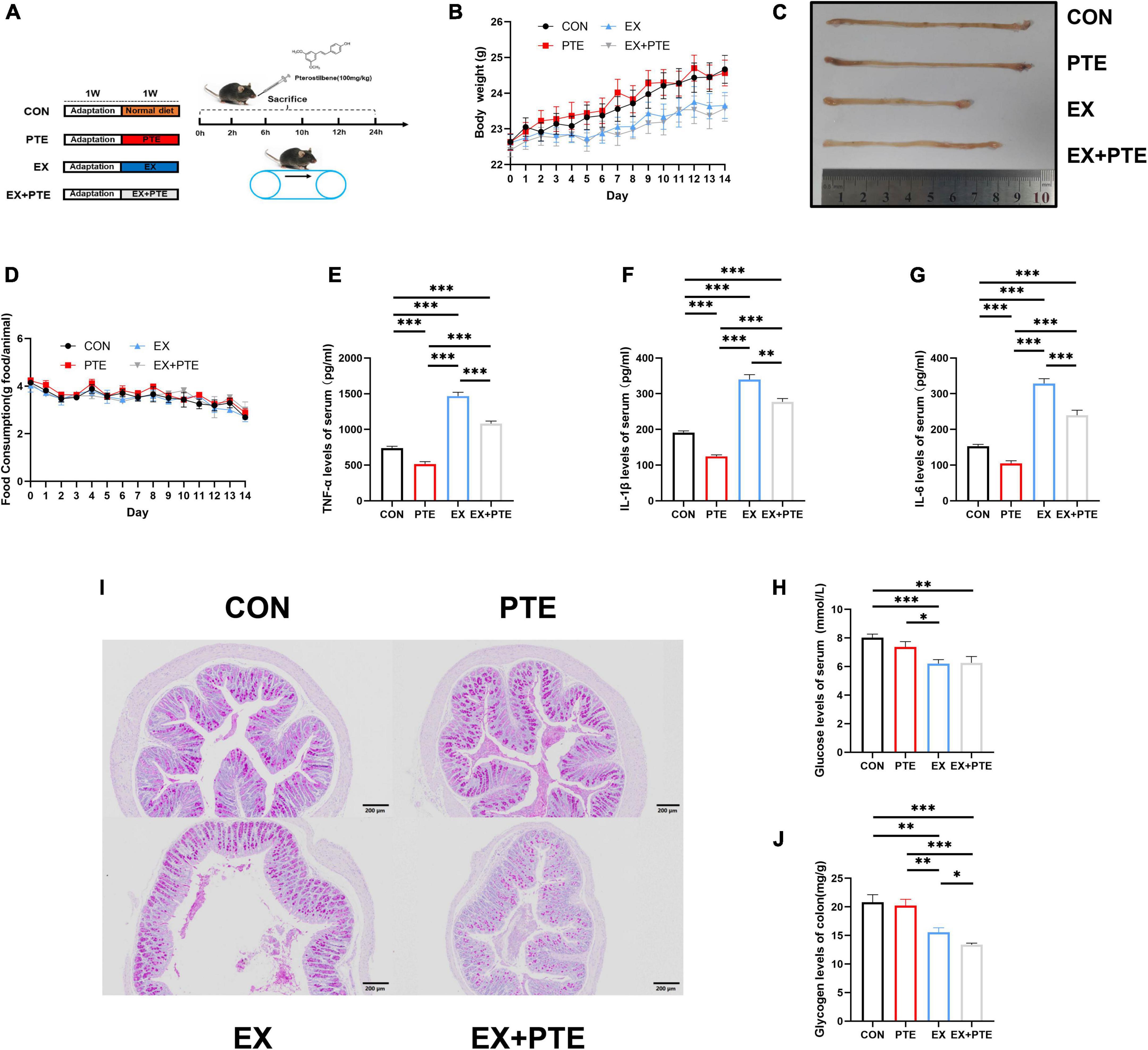
Figure 1. Exercise-induced intestinal barrier disruption and inflammation in C57BL/6 mice. (A) Part1: mice gavaged with pterostilbene (100 mg/kg/day) and sacrificed at 0, 2, 6, 10, 12, 24 h (n = 4); Part 2: mice treatment with pterostilbene (100 mg/kg/day) or exhaustive exercise for 7 days. (B) Body weight was measured one time a day (F = 1.384, p = 0.261). (C) Representative photographs of colons from CON, PTE, EX, and EX + PTE. (D) Food intake was measured one time a day (F = 0.612, p = 0.626). (E) TNF-α (PTE: F = 63.793, p < 0.001; EX: F = 287.397, p < 0.001; interaction: F = 4.354, p < 0.05), (F) IL-1β (χ2 = 28.102, p < 0.001), (G) IL-6 (PTE: F = 40.789, p < 0.001; EX: F = 209.477, p < 0.001; interaction: F = 3.625, p < 0.05), and (H) glucose were measured by ELISA kits immediately (PTE: F = 0.774, p = 0.384; EX: F = 18.084, p < 0.001;interaction: F = 1.079, p = 0.305). (I) Representative PAS images of the colon at a magnification of 100×. (J) The colon glycogen was measured (χ2 = 20.616, p < 0.001). Data were expressed as means ± SEM, and the statistical significance was showed using asterisks denote (*p < 0.05; **p < 0.01; ***p < 0.001).
Cell experiment and design
As the literature reported (34), Caco-2 and CCD 841 CoN cell lines, which were used as intestinal epithelium models frequently, were obtained from the Chinese academy of sciences cell bank or American Type Culture Collection (ATCC) (Manassas, VA, United States). The cells were cultured in Dulbecco’s Modified Eagle’s Medium (DMEM) (Gibco, Carlsbad, CA, United States) containing 10% fetal bovine serum and 1% penicillin–streptomycin in a controlled environment (37°C, 95% air, 5% CO2).
CCK-8 kits: Cells were seeded at an initial density of 1 × 105 cells/well in a 200 μl DMEM medium. After 24 h, cells were exposed to the freshly prepared medium containing PTE (0, 5, 10, 20, 40, 60, 80, and 100 mg/L) or LPS (0, 0.2, 0.4, 0.6, 0.8, 1.0, 2.0, 4.0, 6.0, 8.0, 10.0 μg/ml) for 24 h. Subsequently, we added 10 μl of CCK-8 solution to each well and measured the absorbance at 450 nm.
The scratch test: Cells were seeded at an initial density of 5 × 105 cells/well in 1 ml DMEM medium. After 24 h, the fused monolayer cells were scraped with the tip of a 10 μl sterile pipette and treated with PTE (20 mg/L) or LPS (1 μg/mL). The cells were photographed 0, 12, 24, and 36 h after the scratch.
Quantitative polymerase chain reaction: (1). Cells were seeded at an initial density of 5 × 105 cells/well in a 2 ml DMEM medium. After 24 h, the PTE and LPS + PTE groups were treated with PTE (20 mg/L). The LPS and LPS + PTE groups were treated with LPS (1 μg/ml) 1 h after PTE treatment. (2). Cells were seeded at an initial density of 5 × 105 cells/well in a 2 ml DMEM medium complemented with the components given above and allowed to attach and grow. After 24 h, cells were treated with LPS (1 μg/ml) and collected at 0, 1, 2, 4, 6, 8, and 12 h. The remaining methods refer to qPCR.
High-performance liquid chromatography assays for pterostilbene
Apparatus: The assay was set up by an integrated HPLC system (Waters 2695 Liquid Chromatograph, Waters, America). The HPLC system contained a Pntulips QS-C18 Plus column (250 × 4.6 mm i.d., 5 μm), and the Empower 2 software (Waters, America) was used to analyze the data and control the system. HPLC assays: According to the previous method (35), the colon contents (20 mg) were mixed with methanol (500 μl), followed by vortex oscillation (20 s), and ultrasonic extraction (80 Hz, 10 min, ≤ 30°C, 12 min). Then, the supernatant was taken as the sample detection solution after standing (4°C,1 h) and centrifugation (10,000 g,10 min, 4°C). The TMS was used as an internal standard (200 ng/ml). The mobile/water phase was performed by gradient transportation of acetonitrile and 0.1% (v/v) formic acid for 12 min at a flow rate of 1.2 ml/min. The UV absorbance at 320 nm was recorded (36). Program setting: column temperature 35°C; gradient procedure:(a) 0–4 min, 60% acetonitrile; (b) 4–8 min, 60–90% acetonitrile; (c) 8–12 min, 90% acetonitrile. The system is rebalanced for 10 min before the next injection.
Biochemical analysis
As the literature reported (37), serum (10 μl), intestinal contents (50–100 mg), and tissues (50 mg) were weighed and dissolved in 500 μl phosphate-buffered saline (PBS), vibrated (5 min), and centrifugated (12,000 g, 4°C, 20 min) to collect the liquid supernatant. The inflammatory cytokines (TNF-α, IL-1β, and IL-6), GSH levels of serum, and LPS levels in the supernatant of intestinal contents were detected by ELISA kits (Quanzhou, Ruixing Biological).
Histological analysis
Eosin (H&E) staining: The intestine tissues were fixed in 4% paraformaldehyde fixative overnight. The tissues were sectioned at 5 μm and stained with hematoxylin–eosin, observed, and photographed by light microscope as previously described (13). Glycogen staining: Paraffin-embedded sections (5 μm) of the small intestine and colon were dyed in schiff periodic acid shiff (PAS) staining solution B (10 min) and PAS staining solution A (25 min) under dark conditions, respectively. Then, sections were stained with PAS staining solution C (30 s) and observed by a light microscope according to the instruction. Immunofluorescence staining: Paraffin-embedded sections (5 μm) of the colon were prepared and incubated with 0.5% TrixonX-100 (1 h, RT) according to a previous study (13). After that, sections were blocked with 2% horse serum (1 h, RT) and against with primary antibodies (Occludin, Proteintech, 1:1,000) (12 h, 4°C) and secondary antibodies (FITC, Beyotime, 1:500) (1 h, RT). After being washed with PBS, sections were incubated with DAPI (Sigma,5 μg/ml) (10 min, RT) and visualized with a fluorescence microscope (Olympus, Japan). The ImageJ software was used to measure the mean fluorescence intensity. Transmission electron microscopy: Tissue samples were sealed with 2.5% glutaraldehyde for 24 h, washed, and fixed in osmium solution for 2 h. Tissue was dehydrated, permeabilized, embedded, and sectioned at 60 nm and observed with a JEM-1400 microscope (JEOL, Tokyo, Japan).
Quantitative polymerase chain reaction
As previously reported (38–40), the PrimeScript RT Reagent Kit (Takara, Japan) and TRIzol reagent (Invitrogen Life Technologies, Grand Island, NY) were used to extract the total RNAs. Then, we reversed transcript mRNA into cDNA by qTower 2.2 real-time PCR system (Analytik Jena, Germany). The oligonucleotide primers were synthesized by Sangon Biotech (Shanghai, China), as listed in Supplementary Table 1.
Western blot
As the literature described (39), proteins were extracted from the colon, separated by 12% SDS-PAGE, and transferred onto PVDF membranes (Bio-Rad, CA). After that, membranes were blocked with 5% dried skimmed milk (RT,1 h) and were incubated with primary antibodies (24 h, 4°C) under rotation following antibodies against ZO-1 (Abcam, 1:1,000), occludin (Proteintech, 1:1,000), claudin 1 (Thermo Fisher Scientific, 1:1,000). Then, we incubated the membranes with a secondary antibody (1 h, RT). Finally, we used the ImageJ software (NIH, MD) for the quantitative analysis.
Sequencing of the intestinal flora
We used the DNA Stool kit (Beijing, China) to extract the genomic DNA of bacteria according to the instruction. We analyzed the 16S rRNA gene of the DNA sequence on the Illumina MiSeq platform (Illumina, San Diego, CA, United States), performed by QIIME2 software (41).
Statistical analysis
All experimental data were expressed as means ± SEM, including at least three biological replicates. The details of the statistical analysis were as follows: experiments between two groups were analyzed with Student’s t-test. For multiple group comparisons, we used a two-way ANOVA to analyze the factors’ main effect and interaction and the t-test for individual effect analysis after two-way ANOVA. The two analyzed factors of animal experiments were PTE and EX, and the two analyzed factors of cell experiments were LPS and PTE. Moreover, we used the non-parametric Kruskal–Wallis tests if the variance was not the same.
Finally, we used the two-way ANOVA to analyze body weight, food intake, TNF-α, IL-1β, IL-6, glucose, glycogen, quantity analysis of WB, immunofluorescence staining, and results of qPCR of four groups. Besides, we used the t-test for two groups’ analysis, such as the healing rate of scratch or other experiments which needed the comparison between the two groups. All analyses were performed by SPSS 19.0 (Chicago, IL). A p < 0.05 was considered statistically significant. The statistical significance was showed using asterisks denote (*p < 0.05; **p < 0.01; ***p < 0.001).
Results
Exercise-induced intestinal barrier disruption and inflammation in mice
As shown in Supplementary Figure 1A, sweating and injury of paws in C57BL/6 were significantly observed after running until exhaustive. The body weight (Figure 1B) and food intake (Figure 1D) were the same among the four groups, which indicated that exercise did not change body weight and food intake. There were significant differences in the colonic length (Figure 1C) between the EX group and other groups, and the colonic length of the EX group was shorter than the CON group. After high loading intensity of exercise for 7 days, as previously reported (13), the serum levels of inflammatory cytokines, including TNF-α, IL-1β, and IL-6 in the exercise group, significantly increased (Figures 1E–G). Besides, to confirm the influence on glycogen consumption by exercise, the glucose levels in serum (Figure 1H) and glycogen in intestine tissues of mice were detected by ELISA kits and PAS staining. Moreover, the glycogen level was significantly decreased in the EX group of small intestinal (Supplementary Figures 1B,C) and colon (Figure 1I,J). Histological staining was performed in the small intestine and colon of C57BL/6 mice. The villi of the small intestine (Supplementary Figure 1D) and colon (Figure 2A) were obviously changed with a structural disorder in the EX group compared with the CON group.
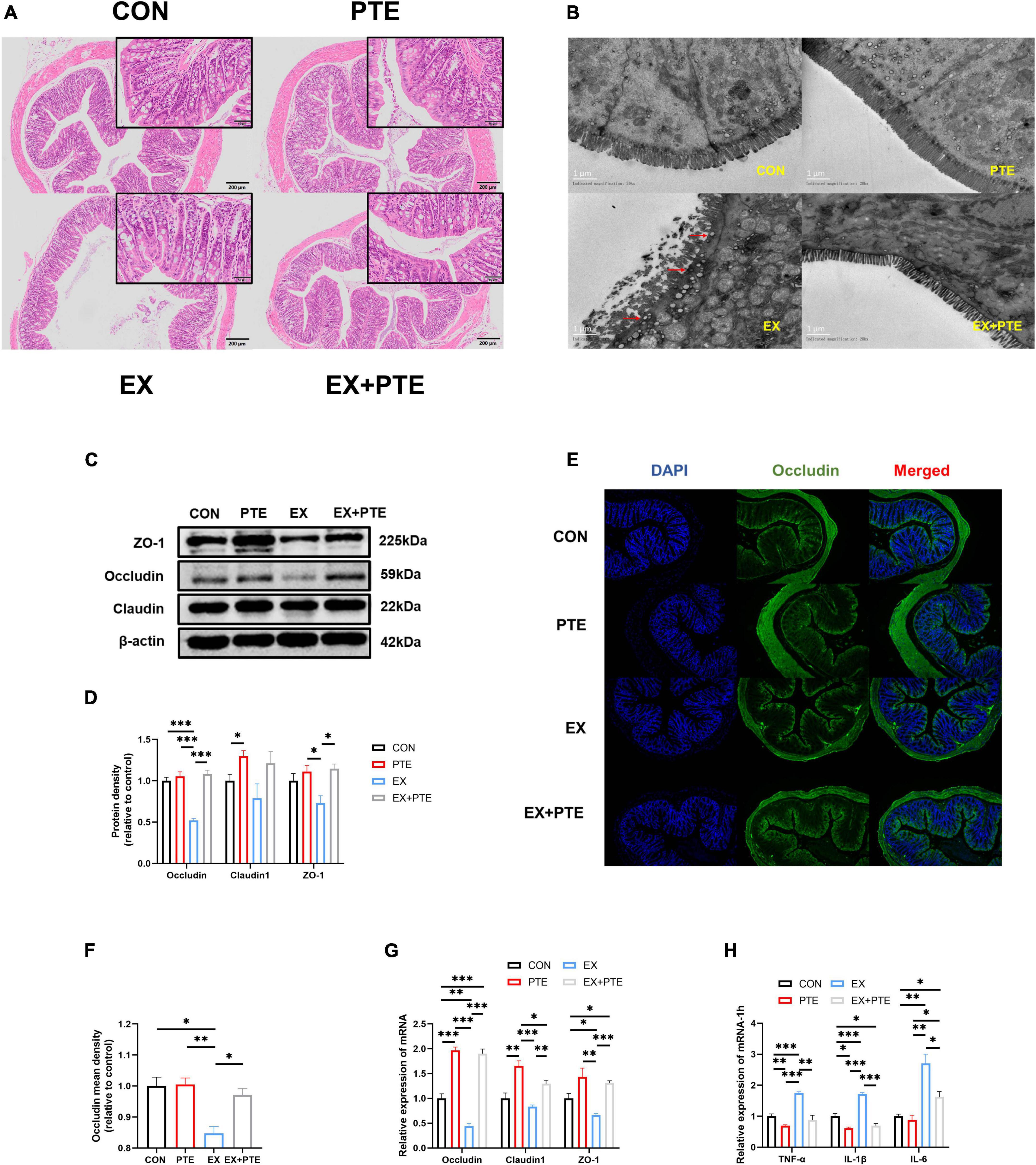
Figure 2. PTE (100 mg/kg/d) inhibited exercise-induced intestinal injury. (A) Histological staining of the colon. (B) Transmission electron microscopy. (C,D) Protein levels of TJ were analyzed by western blotting (occludin: PTE: F = 51.611, p < 0.001; EX: F = 28.061, p < 0.001; interaction: F = 34.992, p < 0.001.Claudin1: PTE: F = 8.435, p < 0.05; EX: F = 1.431, p = 0.266; interaction: F = 0.269, p = 0.618.ZO-1: PTE: F = 11.587, p < 0.01; EX: F = 2.287, p = 0.169; interaction: F = 3.893, p = 0.084). (E,F) Colon was observed by the immunofluorescence staining (PTE: F = 7.793, p < 0.05; EX: F = 16.164, p < 0.01; interaction: F = 6.612, p < 0.05). The expression of the intestinal barrier (G) (occludin: PTE: F = 245.310, p < 0.001; EX: F = 16.339, p < 0.01; interaction: F = 9.892, p < 0.01. Claudin1: PTE: F = 43.313, p < 0.001; EX: F = 9.654, p < 0.01; interaction: F = 1.303, p = 0.276.ZO-1: PTE: F = 26.905, p < 0.001; EX: F = 4.891, p < 0.05; interaction: F = 1.061, p = 0.323) and the inflammatory factor (H) (TNF-α: PTE: F = 44.853, p < 0.001; EX: F = 28.804, p < 0.001; interaction: F = 10.280, p < 0.01.IL-1β: PTE: F = 125.588, p < 0.001; EX: F = 40.647, p < 0.001; interaction: F = 25.763, p < 0.001.IL-6: PTE: F = 10.094, p < 0.01; EX: F = 42.662, p < 0.001; interaction: F = 6.593, p < 0.05) were measured by qPCR. Data were expressed as means ± SEM, and the statistical significance was showed using asterisks denote (*p < 0.05; **p < 0.01; ***p < 0.001).
To further observe the intestinal damage, transmission electron microscopy was performed. The number of microvilli on the surface of the small intestine (Supplementary Figure 1E) and colon (Figure 2B) villi was reduced and disordered. Furthermore, we analyzed protein levels of the TJ-related genes in the colon tissue by western blotting, and the protein expression of ZO-1, occludin, and claudin1 was decreased significantly in the EX group (Figures 2C,D). Moreover, as observed by the immunofluorescence staining, the mean density of occludin was reduced in the colon of C57BL/6 mice from the EX group (Figures 2E,F). Meanwhile, the mRNA expressions of TJ-related genes were decreased in the EX group (Figure 2G), revealing a disrupted intestinal barrier in the colon induced by the high loading intensity of exercise (13). Besides, the mRNA levels of TNF-α, IL-1β, and IL-6 were significantly increased in the EX group compared with the CON group (Figure 2H), suggesting an inflammatory response in intestinal tissues after exercise. Additionally, the GSH levels of serum were significantly decreased in the EX group (Supplementary Figure 1F), showing that high loading intensity of exercise could induce oxidative stress injury in mice. In brief, the results demonstrated high loading intensity of exercise could cause intestinal barrier disruption and inflammation in C57BL/6 mice.
Exercise remodeled the intestinal flora related to lipopolysaccharide production
The cecum contents of mice were collected to investigate exercise’s effect on the intestinal flora. There was no significant difference between CON and EX groups on α-diversity (Supplementary Figures 2A–G), including community richness (Sobs, Chao, and Ace), community diversity (Shannon and Simpson), and community evenness (Simpsoneven and Shannoneven). Moreover, a clear separation was observed by principal coordinate analysis (PCoA) based on unweighted UniFrac distances along the primary ordination axis (PC1), which accounted for 43.16% of the variation (Figure 3A). Based on the average distance, the genus-level species composition of 16 samples was displayed, and hierarchical cluster analysis was performed, indicating that the intestinal flora structure was remodeled by exercise (Figures 3B,C). The analysis of the LEfSe showed that exercise caused an increase in the relative abundance of members from the order Lactobacillales, family Lactobacillaceae, genus Lactobacillus, and genus Alistipes compared with control mice (Figure 3D). Besides, at the genus level, exercise markedly increased the abundance of Lactobacillaceae and Alistipes (Figure 3E), which was related to LPS production as reported (42–44). As accumulating evidence indicated that LPS contributed to intestinal injury, the level of LPS in intestinal contents was measured. The results showed that exercise increased the concentration of LPS significantly (Figures 3F–H) (45, 46). In brief, these results indicated that exercise remodeled the structure of intestinal flora and induced LPS production, which was a major cause of intestinal barrier injury.
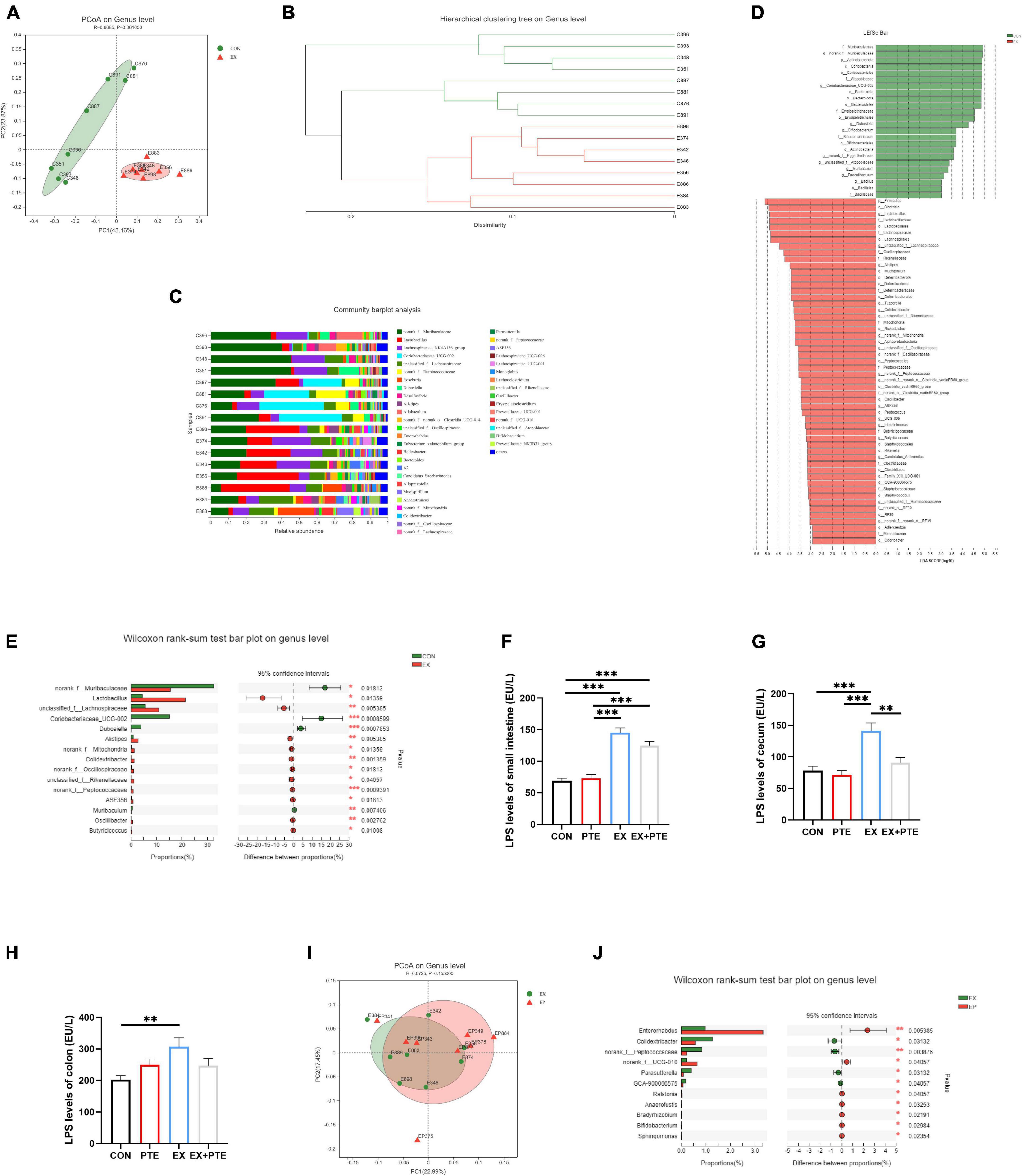
Figure 3. Exercise treatment modified the gut microbiota and induced LPS production. (A) Principal coordinate analysis (PCoA) of the β-diversity based on the unweighted UniFrac distance matrix (at the ASV level) of the CON and EX groups. (B) Hierarchical cluster analysis. (C) The species composition of 16 samples at the genus level, based on the average distance. (D) Bar graph of linear discriminant analysis (LDA) scores, showing the biomarker taxa (LDA score of > 2 and a significance of p < 0.05 determined by the Wilcoxon signed-rank test). (E) The Kruskal–Wallis H test bar plot at the genus level of CON and EX groups. (F–H) LPS was detected by ELISA kits of intestinal contents (F: PTE: F = 1.769, p = 0.194; EX: F = 104.762, p < 0.001; interaction: F = 3.724, p = 0.064; G: χ2 = 14.966, p < 0.05; H: PTE: F = 0.091, p = 0.765; EX: F = 5.766, p < 0.05; interaction: F = 6.364, p < 0.05). (I) PCoA of EX and EX + PTE group. (J) Kruskal–Wallis H test bar plot at the genus level of EX and EX + PTE groups. Data were expressed as means ± SEM, and the statistical significance was showed using asterisks denote (**p < 0.01; ***p < 0.001).
Pterostilbene inhibited intestinal injury induced by exercise
We further detected whether orally garaged with PTE (100 mg/kg/d) in C57BL/6 mice could inhibit intestinal barrier loss induced by the high loading intensity of exercise in the literature (17). We observed that the structural disorder of the small intestine and colon had been improved by histological staining and transmission electron microscopy in the EX + PTE group compared with the EX group (Figures 2A,B and Supplementary Figures 1D,E). Besides, there were significant differences in the gene and protein expression between groups treated with exercise alone or garaged with PTE through a series of indicators (Figures 2C–H). Then, the 16S rRNA gene sequence of cecum content was performed to observe whether PTE could reverse the structure of intestinal flora. At the genus level, there were no significant differences in α-diversity (Supplementary Figures 3A–G), β-diversity (Figure 3I), and the abundance of Lactobacillaceae and Alistipes (Figure 3J) between EX and EX + PTE groups. Besides, based on the average distance, the genus-level species composition was displayed, and hierarchical cluster analysis was performed (Supplementary Figures 3H,I), indicating that PTE could not inhibit LPS production, which was induced by a specific genus. Additionally, the results showed that PTE could improve intestinal barrier loss directly by promoting TJ-related gene expression instead of altering intestinal flora structure.
High concentration of pterostilbene in intestinal
A convenient and effective high-performance liquid chromatography-ultraviolet (HPLC-UV) method was developed to quantify PTE concentration in intestinal contents, and the TMS was used as an internal standard (Supplementary Figures 4A,B). The peak area ratio (pterostilbene and TMS) was used as the analytical response, and the calibration standards of these concentrations (1,500, 3,000, 4,500, 6,000, 7,500, 9,000, 10,500, and 12,000 ng/ml) were used to generate the calibration curve and assess the linearity (y = 0.0057x + 0.3681, R2 = 0.999) (Figure 4A and Supplementary Figure 4C). The PTE and TMS eluted from the system at 7.2 and 10.6 min, respectively. Detected by HPLC-UV, the maximum concentration was achieved at 2 and 6 h in small intestinal and colon contents after oral administration of pterostilbene (Figures 4B,C). Besides, an unidentified metabolite that might be rooted in PTE was present at 2–4 min (Figures 4D,E). However, no PTE analytical response was detected in small intestinal and colon contents 12 h after oral administration. In addition, the PTE could keep high concentration in intestinal tissues within 12 h, which might play a crucial role in intestinal barrier repair.
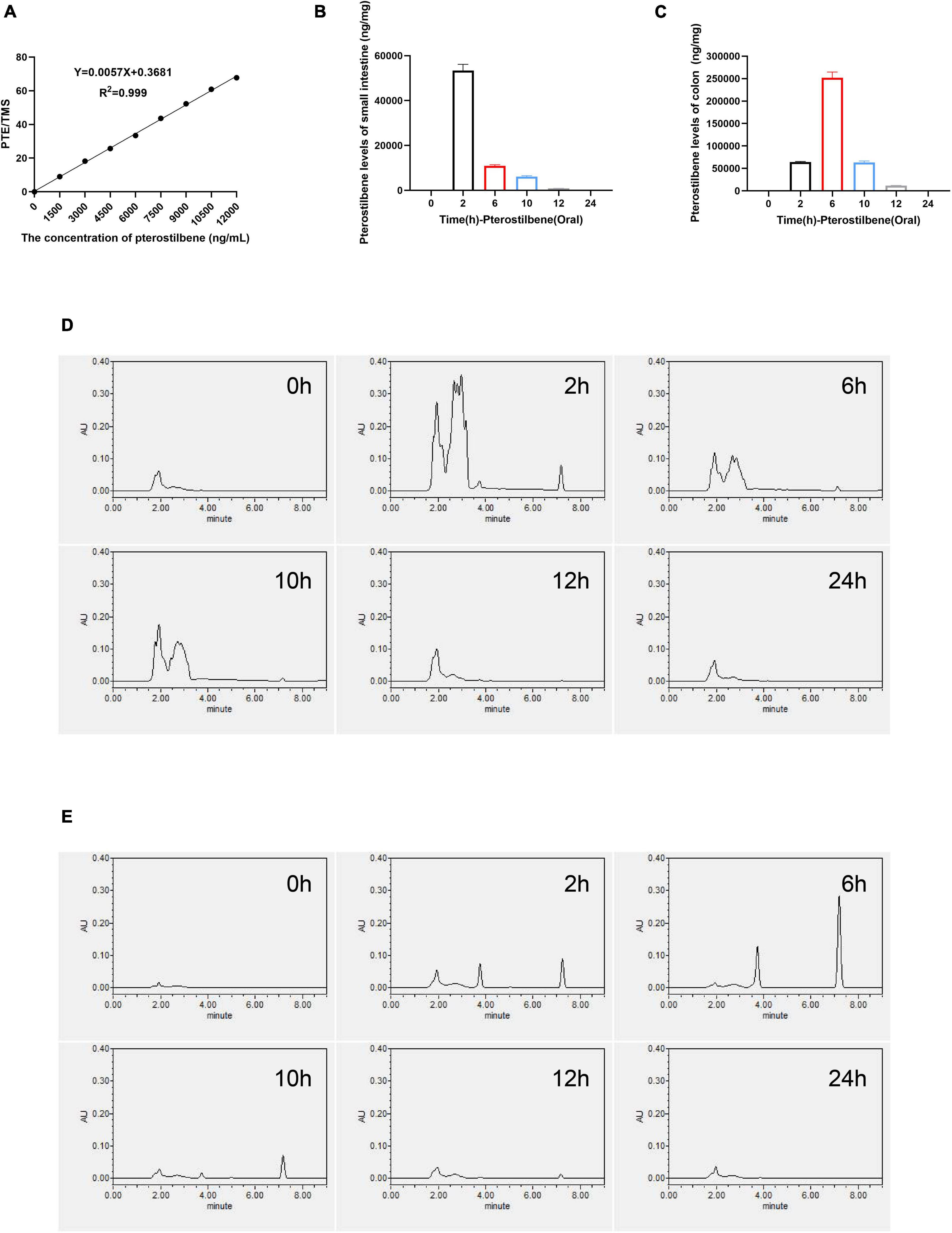
Figure 4. High concentration of pterostilbene in intestinal. (A) the calibration curve of pterostilbene obtained by HPLC method at 320 nm in standard solution. (B,C) Levels of small intestinal and colon gavaged with pterostilbene (100 mg/kg/day). (D) Pterostilbene chromatograms of small intestinal. (E) Pterostilbene chromatograms of colon. Data were expressed as means ± SEM.
Pterostilbene preserve tight junction integrity in vitro
Cell experiments were performed to verify PTE’s potential effects against intestinal barrier injury in vitro. The cell viability of Caco-2 did not change in the presence of a chosen concentration of PTE (0–20 mg/L) and LPS (0–10 mg/L) (Figures 5A,B). Reverse transcription polymerase chain reaction (RT-PCR) analysis showed that LPS-induced inflammatory response occurred in Caco-2 cells (Figure 5C) at early stages (1–3 h), which were subsequently decreased after 6 h, further confirming the results of animal experiments in Figures 1E–G. Besides, there were no significant differences in TJ-related genes (occludin, claudin-1, ZO-1) in Caco-2 cells at different points (Supplementary Figure 4D), indicating that LPS could not affect TJ-related gene expression.
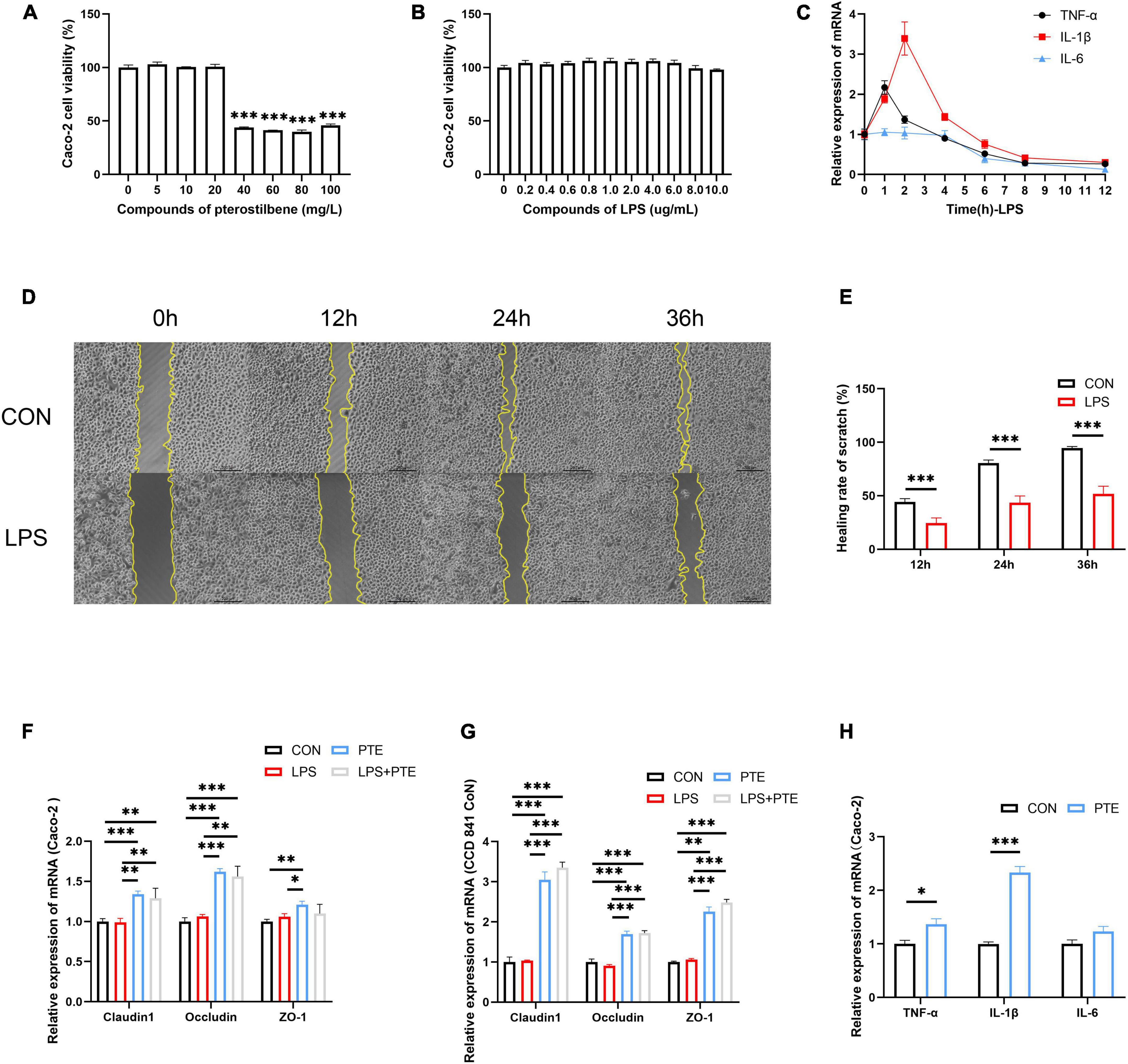
Figure 5. PTE preserve TJ integrity in vitro. (A,B) The cell viability of Caco-2 cells after pterostilbene (0, 5, 10, 20, 40, 60, 80, and 100 mg/L) or LPS (0, 0.2, 0.4, 0.6, 0.8, 1.0, 2.0, 4.0, 6.0, 8.0, and 10.0 μg/ml) intervention. (C) The mRNA expression of inflammatory gene (TNF-α, IL-1β, and IL-6) induced by LPS (1 mg/ml) at 1, 2, 4, 6, 8, and 12 h. (D,E) The healing rate of LPS-treated cells by the scratch test. (F) The expression of TJ-related gene after PTE (20 mg/L) treatment by qPCR analysis in Caco-2 cells (claudin1:LPS: F = 0.398, p = 0.540; PTE: F = 44.602, p < 0.001; interaction: F = 0.174, p = 0.684. occludin: χ2 = 1.333, p = 0.248.ZO-1: LPS: F = 0.380, p = 0.549; PTE: F = 8.755, p < 0.05; interaction: F = 4.126, p = 0.065), (G) CCD 841 CoN cells (claudin1: LPS: F = 1.930, p = 0.190; PTE: F = 327.213, p < 0.001; interaction: F = 1.196, p = 0.296. occludin: LPS: F = 0.283, p = 0.604; PTE: F = 166.214, p < 0.001; interaction: F = 1.125, p = 0.310.ZO-1: χ2 = 2.083, p = 0.149). (H) the expression of inflammatory gene in Caco-2 cell between CON and PTE (20 mg/L). Data were expressed as means ± SEM, and the statistical significance was showed using asterisks denote (*p < 0.05; **p < 0.01; ***p < 0.001).
Additionally, the scratch test showed that the healing rate of LPS-treated cells (24.5 ± 0.7%;43.5 ± 0.9%; 51.8 ± 1.0%) was significantly decreased than control (44.2 ± 0.4%;80.6 ± 0.4%; 94.6 ± 0.2%) at 12, 24, and 36 h (Figures 5D,E). The control experiments were carried out to investigate the potential effects of PTE (20 mg/L,12 h) after LPS (1 mg/L, 1 h) exposure. There were significant improvements in TJ-related genes after PTE treatment compared with other groups by RT-PCR analysis in Caco-2 cells or CCD 841 CoN cells (Figures 5F,G). However, the expression of inflammatory factor genes (TNF-α, IL-1β, and IL-6) was increased obviously after PTE (20 mg/L) intervention in Caco-2 cells (Figure 5H) compared with CCD 841 CoN cells (Supplementary Figure 4E), owing to the anticancer effect of PTE. In addition, treatment with PTE could induce the expression of TJ-related genes.
Discussion
Many studies have proved that exercise is beneficial for health, but the high loading intensity of exercise is harmful to human health. It has been well established that substantial exercise might induce GIS and decrease exercise performance for the athlete population (7, 47). Besides, a common feature of GIS is the altered intestinal permeability (48). The high loading intensity of exercise might affect the downregulation of the TJ-related genes to increase intestinal permeability. Some studies found that the loss of barrier integrity contributes to inflammatory bowel disease and other metabolic diseases (49). The barrier integrity could prevent LPS produced by gut microbiota from transferring into the serum. In this study, we found that the high loading intensity of exercise-induced GIS in C57BL/6 mice mode was related to the disrupted intestinal barrier integrity (4). However, the potential mechanisms remained to be elucidated.
A previous study showed that change in intestinal flora composition was related to metabolism disturbance (50), which might damage intestinal integrity (51). Gut-derived LPS, which is induced by the increased proportions of harmful microorganisms (such as Alistipes) (42), plays a crucial role in causing intestinal inflammatory responses (52). In this study, we found that the intestinal flora composition of C57BL/6 mice was altered by the high loading intensity of exercise, which increased the abundance of Alistipes and caused a high concentration of LPS in contents.
The PTE has been largely investigated for its’ anti-inflammation (53), anticancer (54), antiobesity (55), and antifibrosis effects (56) in the past decade. The PTE reduces blood pressure in adults at 250 mg/day doses (57). Besides, the PTE at 100 mg/kg/day doses could preserve the exercise endurance of mice subjected to sleep restriction in mice (30). PTE at 50 mg/kg/day doses could promote skeletal muscle adaptations to exercise training in rats (58). However, the effect of high doses of PTE on athletes is still unknown. In this study, we aimed to investigate the role of PTE in the occurrence of intestinal barrier repair to prevent perturbed intestinal function followed by high load intensity exercise. The PTE has a significant interest in preventing TJ integrity via promoting the expression of intestinal epithelial TJ molecules. Furthermore, some studies found that PTE protected the intestinal epithelial barrier through the NF-κBMLCK/p-MLC signal pathway in mice (27). Our results showed that high load intensity of exercise could induce disrupted intestinal barrier integrity and inflammation response in the C57BL/6 mice running model, which might be due to the LPS produced by intestinal flora (59). Otherwise, oral administration of PTE could significantly prevent intestinal barrier damage by improving the expression of the TJ-related genes.
Unexpected in vitro experiment, we found that there was no obvious change in the expression of the TJ gene after LPS treatment, showing that LPS-induced intestinal barrier disruption might be related to the immune microenvironment of intestinal and deserves further study (Supplementary Figure 4D). Overall, this is the first report on PTE improving intestinal barrier integrity disrupted by the high loading intensity of the exercise via promoting the expression of intestinal epithelial TJ molecules. However, the potential mechanism is still unclear, which will also be a part of our follow-up study in the future. Besides, there were some limitations in our study. We found that PTE (100 mg/kg/d) could not reverse the intestinal flora structure induced by high load intensity exercise, which indicated that PTE could not prevent the dysbiosis of intestinal flora composition. Moreover, our study only obtained the results from animal and cell experiments, which could be different in humans. Therefore, we will further observe the effect of PTE on athletes, which will also be a part of our follow-up study in the future.
Conclusion
In summary, high load intensity of exercise would affect intestinal permeability caused by LPS, which might be related to altering intestinal flora structure in the cecum (Figure 6). Certain dietary supplements might contribute to the prevention of injury induced by exercise (60). Therefore, we found PTE emerging as a promising candidate for a new generation of sports nutrition supplements for athletes.
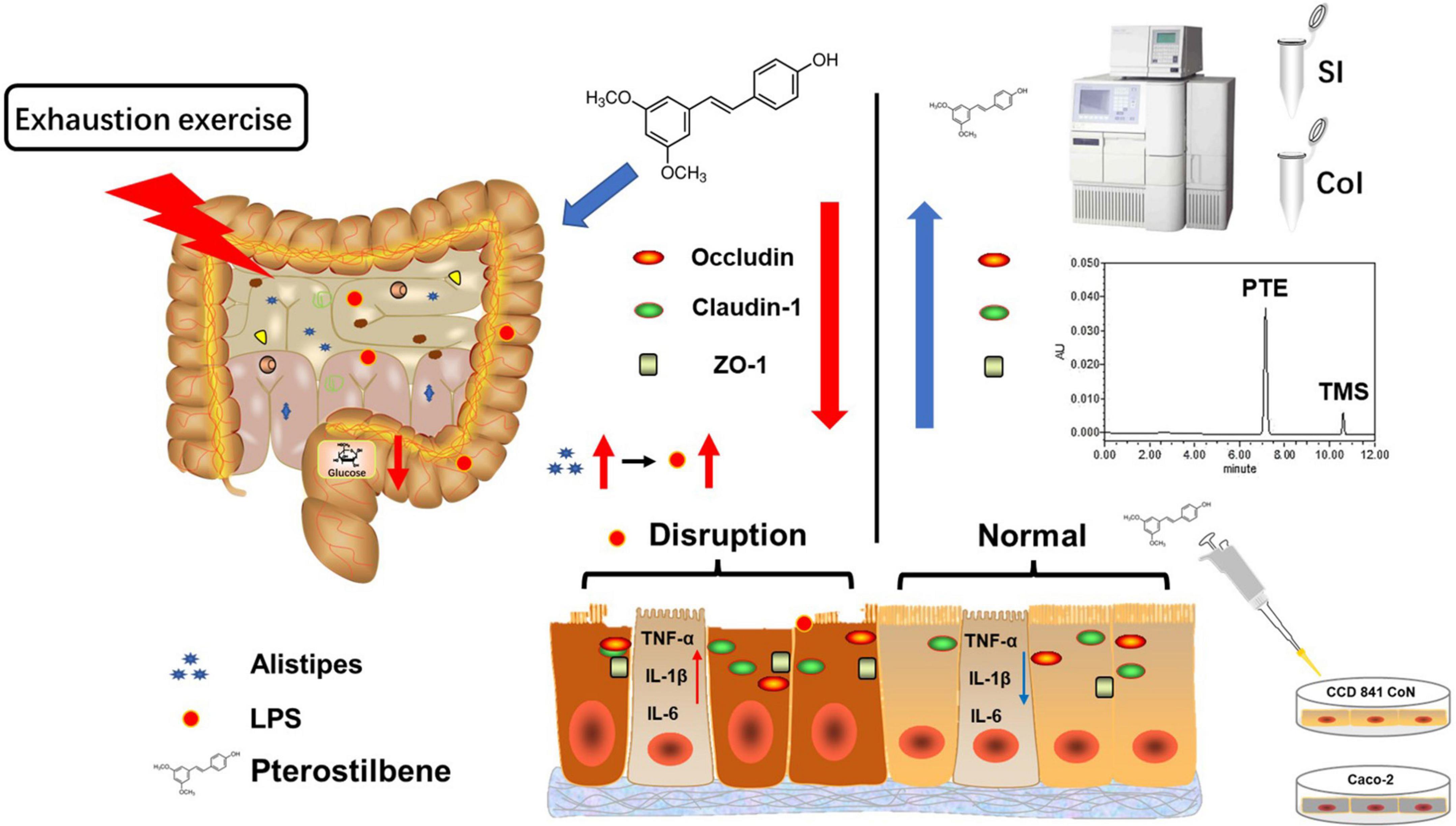
Figure 6. Schematic summary of the results. High loading intensity of exercise disrupted the intestinal epithelial barrier through remodeling the gut flora related to LPS production. Pterostilbene attenuated exercise-induced intestinal barrier injury by improving the expression of the TJ-related genes and suppressing inflammation.
Data availability statement
The original data supporting the conclusions of this article will be provided by the corresponding author, without undue reservation. The raw data of 16S rRNA sequencing reported in this manuscript are deposited in the NCBI database (accession number PRJNA846737). Available online at: https://www.ncbi.nlm.nih.gov/bioproject/846737.
Ethics statement
All animal experiments described herein followed the National Research Council Guidelines, approved by the Animal Care and Use Committee of the Army Medical University.
Author contributions
LZ designed the experiments and drafted the manuscript. GT and LZ collected the samples and performed the experiments. LH assisted in running training and aided in analyzing the data. MZ contributed to technical support. MM, LY, and JZ obtained funding and provided many suggestions on the experiments and the article. All authors contributed to the article and approved the submitted final manuscript.
Funding
This work was supported by the research grants from the Key Projects for Scientific Research (AWS17J014).
Conflict of interest
The authors declare that the research was conducted in the absence of any commercial or financial relationships that could be construed as a potential conflict of interest.
Publisher’s note
All claims expressed in this article are solely those of the authors and do not necessarily represent those of their affiliated organizations, or those of the publisher, the editors and the reviewers. Any product that may be evaluated in this article, or claim that may be made by its manufacturer, is not guaranteed or endorsed by the publisher.
Supplementary material
The Supplementary Material for this article can be found online at: https://www.frontiersin.org/articles/10.3389/fnut.2022.965180/full#supplementary-material
Abbreviations
PTE, pterostilbene; LPS, lipopolysaccharide; ATCC, American Type Culture Collection; DMEM, Dulbecco’s Modified Eagle’s Medium; TMS, 3,5,4’-trimethoxy-trans-stilbene; DAPI, 4’,6-diamidino-2-phenylindole; GIS, gastrointestinal syndrome; TJ, tight junction; PCoA, principal coordinate analysis; PC1, primary ordination axis.
References
1. Mora S, Cook N, Buring JE, Ridker PM, Lee IM. Physical activity and reduced risk of cardiovascular events: potential mediating mechanisms. Circulation. (2007) 116:2110–8. doi: 10.1161/CIRCULATIONAHA.107.729939
2. Fan W, Evans RM. Exercise mimetics: impact on health and performance. Cell Metab. (2017) 25:242–7. doi: 10.1016/j.cmet.2016.10.022
3. Sato S, Dyar KA, Treebak JT, Jepsen SL, Ehrlich AM, Ashcroft SP, et al. Atlas of exercise metabolism reveals time-dependent signatures of metabolic homeostasis. Cell Metab. (2022) 34:329–45. doi: 10.1016/j.cmet.2021.12.016
4. Costa RJS, Snipe RMJ, Kitic CM, Gibson PR. Systematic review: exercise-induced gastrointestinal syndrome-implications for health and intestinal disease. Aliment Pharmacol Ther. (2017) 46:246–65. doi: 10.1111/apt.14157
5. Wei C, Zhao S, Zhang Y, Gu W, Kumar Sarker S, Liu S, et al. Effect of multiple-nutrient supplement on muscle damage, liver, and kidney function after exercising under heat: based on a pilot study and a randomised controlled trial. Front Nutr. (2021) 8:740741. doi: 10.3389/fnut.2021.740741
6. Smith LL. Tissue trauma: the underlying cause of overtraining syndrome? J Strength Cond Res. (2004) 18:185–93. doi: 10.1519/1533-428720040182.0.co;2
7. Pugh JN, Fearn R, Morton JP, Close GL. Gastrointestinal symptoms in elite athletes: time to recognise the problem? Br J Sports Med. (2018) 52:487–8. doi: 10.1136/bjsports-2017-098376
8. Zhao X, Zhang Z, Hu B, Huang W, Yuan C, Zou L. Response of gut microbiota to metabolite changes induced by endurance exercise. Front Microbiol. (2018) 9:765. doi: 10.3389/fmicb.2018.00765
9. Kulecka M, Fraczek B, Mikula M, Zeber-Lubecka N, Karczmarski J, Paziewska A, et al. The composition and richness of the gut microbiota differentiate the top Polish endurance athletes from sedentary controls. Gut Microbes. (2020) 11:1374–84. doi: 10.1080/19490976.2020.1758009
10. Ticinesi A, Lauretani F, Tana C, Nouvenne A, Ridolo E, Meschi T. Exercise and immune system as modulators of intestinal microbiome: implications for the gut-muscle axis hypothesis. Exerc Immunol Rev. (2019) 25:84–95.
11. Clark A, Mach N. Exercise-induced stress behavior, gut-microbiota-brain axis and diet: a systematic review for athletes. J Int Soc Sports Nutr. (2016) 13:43. doi: 10.1186/s12970-016-0155-6
12. Dai X, Wang B. Role of gut barrier function in the pathogenesis of non-alcoholic Fatty liver disease. Gastroenterol Res Pract. (2015) 2015:287348. doi: 10.1155/2015/287348
13. Hou P, Zhou X, Yu L, Yao Y, Zhang Y, Huang Y, et al. Exhaustive exercise induces gastrointestinal syndrome through reduced ILC3 and IL-22 in mouse model. Med Sci Sports Exerc. (2020) 52:1710–8. doi: 10.1249/MSS.0000000000002298
14. Bergmann KR, Liu SXL, Tian R, Kushnir A, Turner JR, Li H-L, et al. Bifidobacteria stabilize claudins at tight junctions and prevent intestinal barrier dysfunction in mouse necrotizing enterocolitis. Am J Pathol. (2013) 182:1595–606. doi: 10.1016/j.ajpath.2013.01.013
15. Zuhl M, Schneider S, Lanphere K, Conn C, Dokladny K, Moseley P. Exercise regulation of intestinal tight junction proteins. Br J Sports Med. (2014) 48:980–6. doi: 10.1136/bjsports-2012-091585
16. Lee SH. Intestinal permeability regulation by tight junction: implication on inflammatory bowel diseases. Intest Res. (2015) 13:11–8. doi: 10.5217/ir.2015.13.1.11
17. Serreli G, Melis MP, Zodio S, Naitza MR, Casula E, Peñalver P, et al. Altered paracellular permeability in intestinal cell monolayer challenged with lipopolysaccharide: modulatory effects of pterostilbene metabolites. Food Chem Toxicol. (2020) 145:111729. doi: 10.1016/j.fct.2020.111729
18. Gomes JR, Freitas JR, Grassiolli S. Effects of physical exercise on the intestinal mucosa of rats submitted to a hypothalamic obesity condition. Anat Rec. (2016) 299:1389–96. doi: 10.1002/ar.23453
19. Holland AM, Hyatt HW, Smuder AJ, Sollanek KJ, Morton AB, Roberts MD, et al. Influence of endurance exercise training on antioxidant enzymes, tight junction proteins, and inflammatory markers in the rat ileum. BMC Res Notes. (2015) 8:514. doi: 10.1186/s13104-015-1500-6
20. Davison G, Marchbank T, March DS, Thatcher R, Playford RJ. Zinc carnosine works with bovine colostrum in truncating heavy exercise-induced increase in gut permeability in healthy volunteers. Am J Clin Nutr. (2016) 104:526–36. doi: 10.3945/ajcn.116.134403
21. Bibi S, Kang Y, Du M, Zhu M-J. Dietary red raspberries attenuate dextran sulfate sodium-induced acute colitis. J Nutr Biochem. (2018) 51:40–6. doi: 10.1016/j.jnutbio.2017.08.017
22. Li C, Wu G, Zhao H, Dong N, Wu B, Chen Y, et al. Natural-derived polysaccharides from plants, mushrooms, and seaweeds for the treatment of inflammatory bowel disease. Front Pharmacol. (2021) 12:651813. doi: 10.3389/fphar.2021.651813
23. Wang Q, Liu F, Chen X, Yang Z, Cao Y. Effects of the polysaccharide SPS-3-1 purified from Spirulina on barrier integrity and proliferation of Caco-2 cells. Int J Biol Macromol. (2020) 163:279–87. doi: 10.1016/j.ijbiomac.2020.06.203
24. Pirozzi C, Lama A, Simeoli R, Paciello O, Pagano TB, Mollica MP, et al. Hydroxytyrosol prevents metabolic impairment reducing hepatic inflammation and restoring duodenal integrity in a rat model of NAFLD. J Nutr Biochem. (2016) 30:108–15. doi: 10.1016/j.jnutbio.2015.12.004
25. Pistol GC, Bulgaru CV, Marin DE, Oancea AG, Taranu I. Dietary grape seed meal bioactive compounds alleviate epithelial dysfunctions and attenuates inflammation in colon of DSS-treated piglets. Foods. (2021) 10:530. doi: 10.3390/foods10030530
26. Hseu YC, Vudhya Gowrisankar Y, Wang LW, Zhang YZ, Chen XZ, Huang PJ, et al. The in vitro and in vivo depigmenting activity of pterostilbene through induction of autophagy in melanocytes and inhibition of UVA-irradiated α-MSH in keratinocytes via Nrf2-mediated antioxidant pathways. Redox Biol. (2021) 44:102007. doi: 10.1016/j.redox.2021.102007
27. Wang J, Zhao H, Lv K, Zhao W, Zhang N, Yang F, et al. Pterostilbene ameliorates DSS-induced intestinal epithelial barrier loss in mice via suppression of the NF-κB-mediated MLCK-MLC signaling pathway. J Agric Food Chem. (2021) 69:3871–8. doi: 10.1021/acs.jafc.1c00274
28. Chen Y, Zhang H, Ji S, Jia P, Chen Y, Li Y, et al. Resveratrol and its derivative pterostilbene attenuate oxidative stress-induced intestinal injury by improving mitochondrial redox homeostasis and function via SIRT1 signaling. Free Radic Biol Med. (2021) 177:1–14. doi: 10.1016/j.freeradbiomed.2021.10.011
29. Chen M, Hui S, Lang H, Zhou M, Zhang Y, Kang C, et al. SIRT3 deficiency promotes high-fat diet-induced non-alcoholic fatty liver disease in correlation with impaired intestinal permeability through gut microbial dysbiosis. Mol Nutr Food Res. (2019) 63:e1800612. doi: 10.1002/mnfr.201800612
30. Liu Y, Lang H, Zhou M, Huang L, Hui S, Wang X, et al. The preventive effects of pterostilbene on the exercise intolerance and circadian misalignment of mice subjected to sleep restriction. Mol Nutr Food Res. (2020) 64:e1900991. doi: 10.1002/mnfr.201900991
31. Bedford TG, Tipton CM, Wilson NC, Oppliger RA, Gisolfi CV. Maximum oxygen consumption of rats and its changes with various experimental procedures. J Appl Physiol Respir Environ Exerc Physiol. (1979) 47:1278–83. doi: 10.1152/jappl.1979.47.6.1278
32. Lezi E, Lu J, Selfridge JE, Burns JM, Swerdlow RH. Lactate administration reproduces specific brain and liver exercise-related changes. J Neurochem. (2013) 127:91–100. doi: 10.1111/jnc.12394
33. Zou D, Chen K, Liu P, Chang H, Zhu J, Mi M. Dihydromyricetin improves physical performance under simulated high altitude. Med Sci Sports Exerc. (2014) 46:2077–84. doi: 10.1249/MSS.0000000000000336
34. Wang Y, Ding X, Chen Y, Xie J, Zheng B, Chang X, et al. “Dialogue” between Caco-2 and DCs regulated by Ganoderma atrum polysaccharide in intestinal-like Caco-2/DCs co-culture model. Food Res Int. (2021) 144:110310. doi: 10.1016/j.foodres.2021.110310
35. Lin H-S, Yue B-D, Ho PC. Determination of pterostilbene in rat plasma by a simple HPLC-UV method and its application in pre-clinical pharmacokinetic study. Biomed Chromatogr. (2009) 23:1308–15. doi: 10.1002/bmc.1254
36. Paul B, Masih I, Deopujari J, Charpentier C. Occurrence of resveratrol and pterostilbene in age-old darakchasava, an ayurvedic medicine from India. J Ethnopharmacol. (1999) 68:71–6. doi: 10.1016/s0378-8741(99)00044-6
37. Colldén H, Landin A, Wallenius V, Elebring E, Fändriks L, Nilsson ME, et al. The gut microbiota is a major regulator of androgen metabolism in intestinal contents. Am J Physiol Endocrinol Metab. (2019) 317:E1182–92. doi: 10.1152/ajpendo.00338.2019
38. Hui S, Liu Y, Chen M, Wang X, Lang H, Zhou M, et al. Capsaicin improves glucose tolerance and insulin sensitivity through modulation of the gut microbiota-bile acid-FXR axis in type 2 diabetic db/db Mice. Mol Nutr Food Res. (2019) 63:e1900608. doi: 10.1002/mnfr.201900608
39. Zeng X, Yang J, Hu O, Huang J, Ran L, Chen M, et al. Dihydromyricetin ameliorates non-alcoholic fatty liver disease by improving mitochondrial respiratory capacity and redox homeostasis through modulation of SIRT3 signaling. Antioxid Redox Signal. (2019) 30:163–83. doi: 10.1089/ars.2017.7172
40. Hui S, Liu Y, Huang L, Zheng L, Zhou M, Lang H, et al. Resveratrol enhances brown adipose tissue activity and white adipose tissue browning in part by regulating bile acid metabolism via gut microbiota remodeling. Int J Obes. (2020) 44:1678–90. doi: 10.1038/s41366-020-0566-y
41. Bolyen E, Rideout JR, Dillon MR, Bokulich NA, Abnet CC, Al-Ghalith GA, et al. Reproducible, interactive, scalable and extensible microbiome data science using QIIME 2. Nat Biotechnol. (2019) 37:852–7. doi: 10.1038/s41587-019-0209-9
42. Kang Y, Li Y, Du Y, Guo L, Chen M, Huang X, et al. Konjaku flour reduces obesity in mice by modulating the composition of the gut microbiota. Int J Obes. (2019) 43:1631–43. doi: 10.1038/s41366-018-0187-x
43. Ding S, Jiang H, Fang J, Liu G. Regulatory effect of resveratrol on inflammation induced by lipopolysaccharides reprograming intestinal microbes and ameliorating serum metabolism profiles. Front Immunol. (2021) 12:777159. doi: 10.3389/fimmu.2021.777159
44. D’hennezel E, Abubucker S, Murphy LO, Cullen TW. Total lipopolysaccharide from the human gut microbiome silences toll-like receptor signaling. mSystems. (2017) 2:e00046–17. doi: 10.1128/mSystems.00046-17
45. Barberio MD, Elmer DJ, Laird RH, Lee KA, Gladden B, Pascoe DD. Systemic LPS and inflammatory response during consecutive days of exercise in heat. Int J Sports Med. (2015) 36:262–70. doi: 10.1055/s-0034-1389904
46. Antunes BM, Campos EZ, Dos Santos RVT, Rosa-Neto JC, Franchini E, Bishop NC, et al. Anti-inflammatory response to acute exercise is related with intensity and physical fitness. J Cell Biochem. (2019) 120:5333–42. doi: 10.1002/jcb.27810
47. Ter Steege RWF, Geelkerken RH, Huisman AB, Kolkman JJ. Abdominal symptoms during physical exercise and the role of gastrointestinal ischaemia: a study in 12 symptomatic athletes. Br J Sports Med. (2012) 46:931–5. doi: 10.1136/bjsports-2011-090277
48. Bensemmane L, Squiban C, Demarquay C, Mathieu N, Benderitter M, Le Guen B, et al. The stromal vascular fraction mitigates radiation-induced gastrointestinal syndrome in mice. Stem Cell Res Ther. (2021) 12:309. doi: 10.1186/s13287-021-02373-y
49. Chelakkot C, Ghim J, Ryu SH. Mechanisms regulating intestinal barrier integrity and its pathological implications. Exp Mol Med. (2018) 50:1–9. doi: 10.1038/s12276-018-0126-x
50. Ridaura VK, Faith JJ, Rey FE, Cheng J, Duncan AE, Kau AL, et al. Gut microbiota from twins discordant for obesity modulate metabolism in mice. Science. (2013) 341:1241214. doi: 10.1126/science.1241214
51. Cani PD, Possemiers S, Van De Wiele T, Guiot Y, Everard A, Rottier O, et al. Changes in gut microbiota control inflammation in obese mice through a mechanism involving GLP-2-driven improvement of gut permeability. Gut. (2009) 58:1091–103. doi: 10.1136/gut.2008.165886
52. Guo S, Nighot M, Al-Sadi R, Alhmoud T, Nighot P, Ma TY. Lipopolysaccharide regulation of intestinal tight junction permeability is mediated by TLR4 signal transduction pathway activation of FAK and MyD88. J Immunol. (2015) 195:4999–5010. doi: 10.4049/jimmunol.1402598
53. Teng WL, Huang PH, Wang HC, Tseng CH, Yen FL. Pterostilbene attenuates particulate matter-induced oxidative stress, inflammation and aging in keratinocytes. Antioxidants. (2021) 10:1552. doi: 10.3390/antiox10101552
54. Obrador E, Salvador-Palmer R, Jihad-Jebbar A, López-Blanch R, Dellinger TH, Dellinger RW, et al. Pterostilbene in cancer therapy. Antioxidants. (2021) 10:492. doi: 10.3390/antiox10030492
55. Trepiana J, Gómez-Zorita S, Fernández-Quintela A, González M, Portillo MP. Effects of resveratrol and its analogue pterostilbene, on NOV/CCN3 adipokine in adipose tissue from rats fed a high-fat high-sucrose diet. J Physiol Biochem. (2019) 75:275–83. doi: 10.1007/s13105-019-00680-w
56. Peng Y, Zhang Y, Zhang Y, Wang X, Xia Y. Pterostilbene alleviates pulmonary fibrosis by regulating ASIC2. Chin Med. (2021) 16:66. doi: 10.1186/s13020-021-00474-7
57. Riche DM, Riche KD, Blackshear CT, Mcewen CL, Sherman JJ, Wofford MR, et al. Pterostilbene on metabolic parameters: a randomized, double-blind, and placebo-controlled trial. Evid Based Complement Alternat Med. (2014) 2014:459165. doi: 10.1155/2014/459165
58. Zheng J, Liu W, Zhu X, Ran L, Lang H, Yi L, et al. Pterostilbene enhances endurance capacity via promoting skeletal muscle adaptations to exercise training in rats. Molecules. (2020) 25:186. doi: 10.3390/molecules25010186
59. Pires W, Veneroso CE, Wanner SP, Pacheco DAS, Vaz GC, Amorim FT, et al. Association between exercise-induced hyperthermia and intestinal permeability: a systematic review. Sports Med. (2017) 47:1389–403. doi: 10.1007/s40279-016-0654-2
Keywords: exercise, pterostilbene (PTE), lipopolysaccharide (LPS), intestinal barrier, intestinal flora
Citation: Zhang L, Tian G, Huang L, Zhou M, Zhu J, Yi L and Mi M (2022) Pterostilbene attenuates intestinal epithelial barrier loss induced by high loading intensity of exercise. Front. Nutr. 9:965180. doi: 10.3389/fnut.2022.965180
Received: 09 June 2022; Accepted: 11 July 2022;
Published: 04 August 2022.
Edited by:
Marcin Maciejczyk, University of Physical Education in Krakow, PolandReviewed by:
Nicola Sponsiello, Independent Researcher, Padua, ItalyMicah Zuhl, Central Michigan University, United States
David Christopher Nieman, Appalachian State University, United States
Copyright © 2022 Zhang, Tian, Huang, Zhou, Zhu, Yi and Mi. This is an open-access article distributed under the terms of the Creative Commons Attribution License (CC BY). The use, distribution or reproduction in other forums is permitted, provided the original author(s) and the copyright owner(s) are credited and that the original publication in this journal is cited, in accordance with accepted academic practice. No use, distribution or reproduction is permitted which does not comply with these terms.
*Correspondence: Mantian Mi, bWlfbWFudGlhbkBzaW5hLmNvbQ==
†These authors have contributed equally to this work