Circulating trace elements status in COVID-19 disease: A meta-analysis
- 1Clinical Laboratory, PLA North Military Command Region General Hospital, Shenyang, China
- 2Department of Medical Engineering, PLA North Military Command Region General Hospital, Shenyang, China
- 3Department of Bioinformatics, Key Laboratory of Cell Biology, Ministry of Public Health, and Key Laboratory of Medical Cell Biology, Ministry of Education, School of Life Sciences, China Medical University, Shenyang, China
Trace elements are a group of essential metals or metalloids, which are necessary for life, and present in minute amounts. Despite substantial researches highlighting the importance of trace elements in Coronavirus disease 2019 (COVID-19) diseases, a thorough evaluation of the levels of circulating trace elements is lacking. Therefore, we conducted a systematic review and meta-analysis to evaluate the trace element status (Zn, Fe, Cu, Mg, and Se) in COVID-19 disease. We also assessed the relationship between circulating trace elements and COVID-19 disease severity and survival status during follow-up. We searched comprehensively MEDLINE, Web of Science, CNKI, and WangFang databases without language restriction, between November 1, 2019 and April 1, 2022. The search identified 1,566 preliminary references. A total of 49 studies met the eligibility criteria and were included in the review, and 42 studies were included in the final meta-analysis. Meta-analysis showed that COVID-19 patients had significantly lower circulating Zn (SMD: −0.83, 95% CI: −1.19 to −0.46, P < 0.001), Fe (SMD: −1.56, 95% CI: −2.90 to −0.21, P = 0.023), and Se (SMD: −0.75, 95% CI: −0.94 to −0.56, P < 0.001) levels than healthy controls, and circulating Zn (SMD: −0.47, 95% CI: −0.75 to −0.18, P = 0.002), Fe (SMD: −0.45, 95% CI: −0.79 to −0.12, P = 0.008), and Se (SMD: −0.27, 95% CI: −0.49 to −0.04, P = 0.020) levels were associated with the presence of severity status in COVID-19 patients. Moreover, circulating Fe levels in non-survivors were significantly lower than survivors in COVID-19 (SMD: −0.28, 95% CI: −0.44 to −0.12, P = 0.001). However, there was no significant difference in Cu and Mg levels between COVID-19 patients and controls, severity and non-severity status, and survivors and non-survivors (all P > 0.05). Taken together, COVID-19 patients displayed lower circulating levels of Zn, Fe, and Se, and their levels were associated with severity status. Moreover, circulating Fe levels may provide part of the explanation for the unfavorable survival status. Therefore, we presumed optimistically that supplements of trace elements might provide an adjutant treatment in the early stages of COVID-19.
Systematic review registration: [https://www.crd.york.ac.uk/prospero], identifier [CRD42022348599].
Introduction
The global Coronavirus disease 2019 (COVID-19), caused by Severe Acute Respiratory Syndrome Coronavirus-2 (SARS-CoV-2), has becoming a pandemic disease in March, 2020 (1). Based on the World Health Organization (WHO) declaration, as of May13, 2022, there have been 517,648,631 confirmed cases of COVID-19, including 6,261,708 deaths (2). COVID-19 presents with a broad clinical spectrum, ranging from mild fever to fatigue, cough, severe pneumonia, acute respiratory distress syndrome (ARDS), affecting the kidneys, brain, liver, gastrointestinal tract, heart, and other organs (1, 3, 4). The present studies indicated that the mortality varies according to sex, age, disease severity, circumstance, and patient comorbidities (mainly chronic lung diseases, hypertension, diabetes, and coronary heart disease) (5, 6). In view of the rapid spread and much more contagiousness of SARS-CoV-2 variants (7), the COVID-19 pandemic has posed a serious threat to the lives and health of people around the world.
Trace elements are a group of essential metals or metalloids, which are necessary for life, and present in minute amounts (8). Trace elements are involved in various biological, chemical and molecular processes, which regulates cellular homeostasis, humoral and cellular immune responses and acts as cofactors for many enzymes and antioxidant molecules (9). It has been established that zinc (Zn), iron (Fe), copper (Cu), magnesium (Mg), and selenium (Se) play vital and synergistic roles at every stage of the immune response (10). Deficiency of trace elements could affect innate and adaptive immune response, which predisposes to infections and further aggravates malnutrition (11). At present, there are also a number of studies and reviews highlighting a potentially important roles of trace elements in the pathogenesis of COVID-19, and investigating the possibility of utilization of trace elements in diagnosis, prognosis and supplements in therapeutic procedures (12–14).
Zn is an essential trace element which has a variety of fundamental biological functions, such as antioxidant, anti-inflammatory, and apoptotic effects (15–17). Zn element also involved in DNA synthesis, cellular integrity, cell division, cell proliferation, cell differentiation, and cell signal transduction as a second messenger (18). Besides being essential for a fundamental biological functions, the present study indicated that Zn deficiency could weaken the ability of the human body toward SARS-CoV-2 infection and increases the risk of overactive immune response to cause tissue damage(19). Moreover, Ivanova ID et al. found that Zn and Cu levels were abnormal dynamically during the course of COVID-19, and were mainly associated with the inflammation response (20). Based on previous literature, it is clear that maintaining optimum levels of Zn and Cu may stimulate both innate and adaptive immune systems in the course of viral infection. Another important metal element, Fe, is a key functional components for many proteins and enzymes involved in vital cellular processes, and a vital nutrient affecting immunity (21, 22). Previous studies suggested that Fe deficiency was associated with decreased immunity to pathogens and poorer response to some vaccines (23, 24). Domenico Girelli et al. indicated that severe COVID-19 appears to be characterized by marked functional Fe deficiency, which was possibly related to impaired immune response (21). Mg is an essential nutrient required for many different metabolic and biochemical function. In view of the importance of Mg in maintaining proper immune, vascular and pulmonary function, Valentina Trapani et al. had proposed that Mg homeostasis could affect the susceptibility and the response to SARS-CoV-2 (25). Subsequent preclinical works showed that Mg does have protective effects against COVID-19 infection, and derangement in Mg homeostasis might contribute to and aggravate COVID-19 syndrome (26–28). To date, accumulating evidence has indicating that Se play a role in anti-inflammatory, antiviral, and oxidative stress and immune-cell activity, and is a prerequisite for proper immune system functioning (29–33). The recent researches suggested that a deficiency of Se may decrease the immune defenses against COVID-19 and is associated with COVID-19 disease severity and mortality (34–36). Based on these experimental and clinical data, Zn, Cu, Fe, Mg, and Se were considered as the important trace elements, which involved in the process of COVID-19.
Despite substantial researches highlighting the importance of trace elements in COVID-19 disease, a thorough evaluation of the levels of circulating trace elements is lacking. Due to a variety of populations, different measuring methods, different reference values, geographic features, and dietary habits, previous studies have shown the conflicting results on the association of circulating trace elements and COVID-19. Therefore, it is hard to extrapolate a univocal conclusion from the existing evidence. The overall objective of the present study was to evaluate the trace element status in COVID-19 disease by conducting a meta-analysis. We also assessed the relationships between circulating trace elements and COVID-19 disease severity and survival status during follow-up.
Materials and methods
Search strategy
The systematic review and meta-analysis were conducted following a recently published protocol (37), and reported according to the guidelines of the PRISMA (Preferred Reporting Items for Systematic Reviews and Meta-Analysis)(38). We searched comprehensively MEDLINE, Web of Science, CNKI, and WangFang databases without language restriction, between 1st November 2019 and 1st April 2022. In literature search, we used MeSH terms: (COVID-19[MeSH Terms]) AND (trace elements [MeSH Terms]). The related key words were used for all of the databases: (“trace elements” OR “zinc” OR “iron” OR “copper” OR “magnesium” OR “selenium”) AND (“COVID-19” or “SARS-CoV-2” OR “coronavirus”) AND (“blood” OR “serum” OR “plasma” OR ‘circulating”). In addition, the reference lists of the primary studies were evaluated and screened to find other relevant studies.
Selection criteria
In order to investigate the difference between blood trace element levels in COVID-19 patients and controls, and associations between blood trace element levels and COVID-19 severity and survival status, we used the inclusion and exclusion criteria to identify relevant articles. The inclusion criteria were as follows: (a) A diagnosed COVID-19 disease, (b) reporting blood levels of trace elements (Zn, Cu, Fe, Mg, and Se) in COVID-19 patients and matched controls, (c) COVID-19 patients with different degree of disease severity or survival status. The detailed COVID-19 severity classification criteria used in the evaluation of original studies and meta-analyses was based on international guidelines or Acute Physiology and Chronic Health Evaluation. Severe and critical categories were defined as severe, mild and moderate as non-severe in data analysis. The exclusion criteria were: (a) studies with incomplete data; (b) pregnant COVID-19 cases and pediatric cases; (c) case reports, letters, reviews, comment, and animal studies; (d) duplicate publication. All literature was independently reviewed by two authors (LB and LYH). Any discrepancy was solved through discussion.
Data extraction and quality assessment
The extract data included first author, publication year, country, sample size, study design, ages of COVID-19 patients and controls, sex, disease severity, and survival outcome. Two authors independently collected the data, and finally reached an agreement. The quality of included studies were assessed using Newcastle-Ottawa Scale (NOS).
Statistical analysis
STATA 14.0 software (STATA Corp., College Station, TX, United States) was used to analyze the available data. The standard mean difference (SMD) with 95% confidence intervals (CIs) was estimated the difference of circulating trace element levels between COVID-19 patients and controls, severe COVID-19 infection and non-severe COVID-19 infection, survivors and non-survivors. Assessment of heterogeneity was performed using Cochran’s Q statistics (P < 0.1) and I2 statistics (I2 > 50%), in which a P < 0.10 or I2 > 50% indicated significant heterogeneity. A random-effect model was adopted to calculate the pooled SMD and 95% CI in the presence of significant heterogeneity, otherwise, a fixed-effect model was conducted. Sensitivity analyses were performed to evaluate the influence of each study on the overall effect size using the leave-one-out method. The funnel plots, Egger’s test, and Begg’s test were used to evaluate publication bias. A P < 0.05 was considered statistically significant.
Results
Identification of included studies
A total of 1,566 articles were obtained from MEDLINE, Web of Science, CNKI, and WanFang databases. After screening, 49 articles were selected for eligibility in the analysis. After reviewing the full text, 9 articles were excluded due to non-blood samples, inappropriate grouping, data unavailable, and inappropriate samples (pregnant cases and children cases). Finally, there were 40 studies met the inclusion and exclusion criteria in the meta-analysis. A flow chart of the literature search was shown in Figure 1.
Characteristics of included studies
The characteristics of all included studies were present in Tables 1–3, respectively. These studies were published ranging from 2020 to 2022. Of 40 studies, 18 articles reported Zn levels (11 articles: COVID-19 vs. controls; 8 articles: severity vs. non-severity; 4 articles: survivor vs. non-survivors)(12, 20, 27, 39–53), 15 articles reported Fe levels (4 articles: COVID-19 vs. controls; 12 articles: severity vs. non-severity; 6 articles: survivor vs. non-survivors) (12, 27, 40, 51, 54–64), 9 articles reported Cu levels (5 articles: COVID-19 vs. controls; 4 articles: severity vs. non-severity; 3 articles: survivor vs. non-survivors) (12, 20, 27, 39, 47, 49–51, 65), 10 articles reported Mg levels (3 articles: COVID-19 vs. controls; 5 articles: severity vs. non-severity; 6 articles: survivor vs. non-survivors)(27, 28, 40, 50, 51, 66–70), and 9 articles reported Se levels (5 articles: COVID-19 vs. controls; 4 articles: severity vs. non-severity; 3 articles: survivor vs. non-survivors) (34, 36, 39, 44, 47, 49, 51, 71, 72). Overall, the studies were performed in 16 countries across Asia, Europe, America and Oceania. Moreover, it is noteworthy that 3,735 participants (1,336 COVID-19 cases and 2,399 controls, Table 1), 2,076 participants (818 severe COVID-19 cases and 1,258 non-severe COVID-19 cases, Table 2), and 3,248 participants (2,677 survivors and 571 non-survivors, Table 3) were included in the present analysis, respectively. The samples types in all included studies were serum or plasma or whole blood. The risk of bias assessment based on NOS was displayed in Supplementary Table 1.
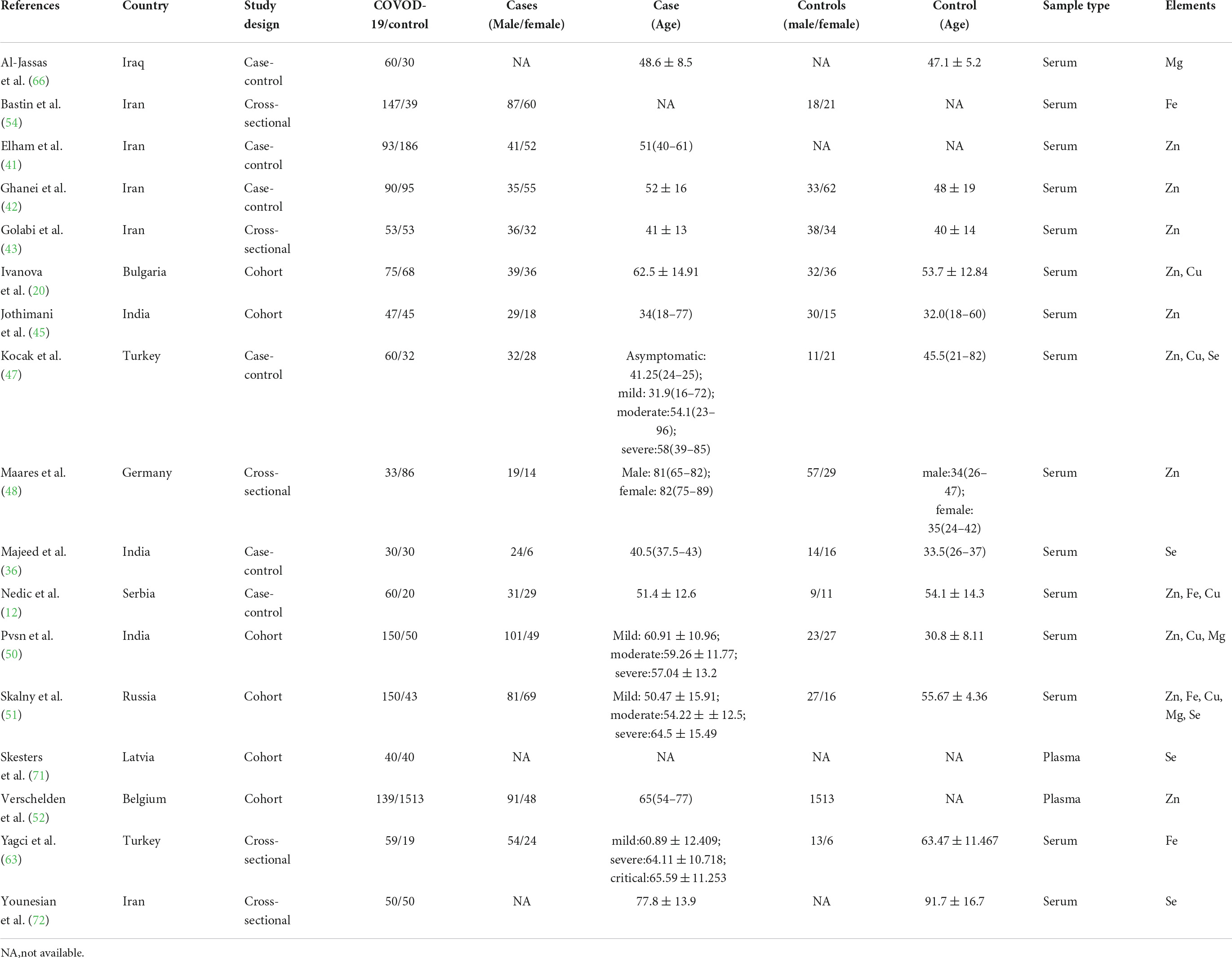
Table 1. The main characteristics of included studies in comparison of trace elements between COVID-19 cases and controls.
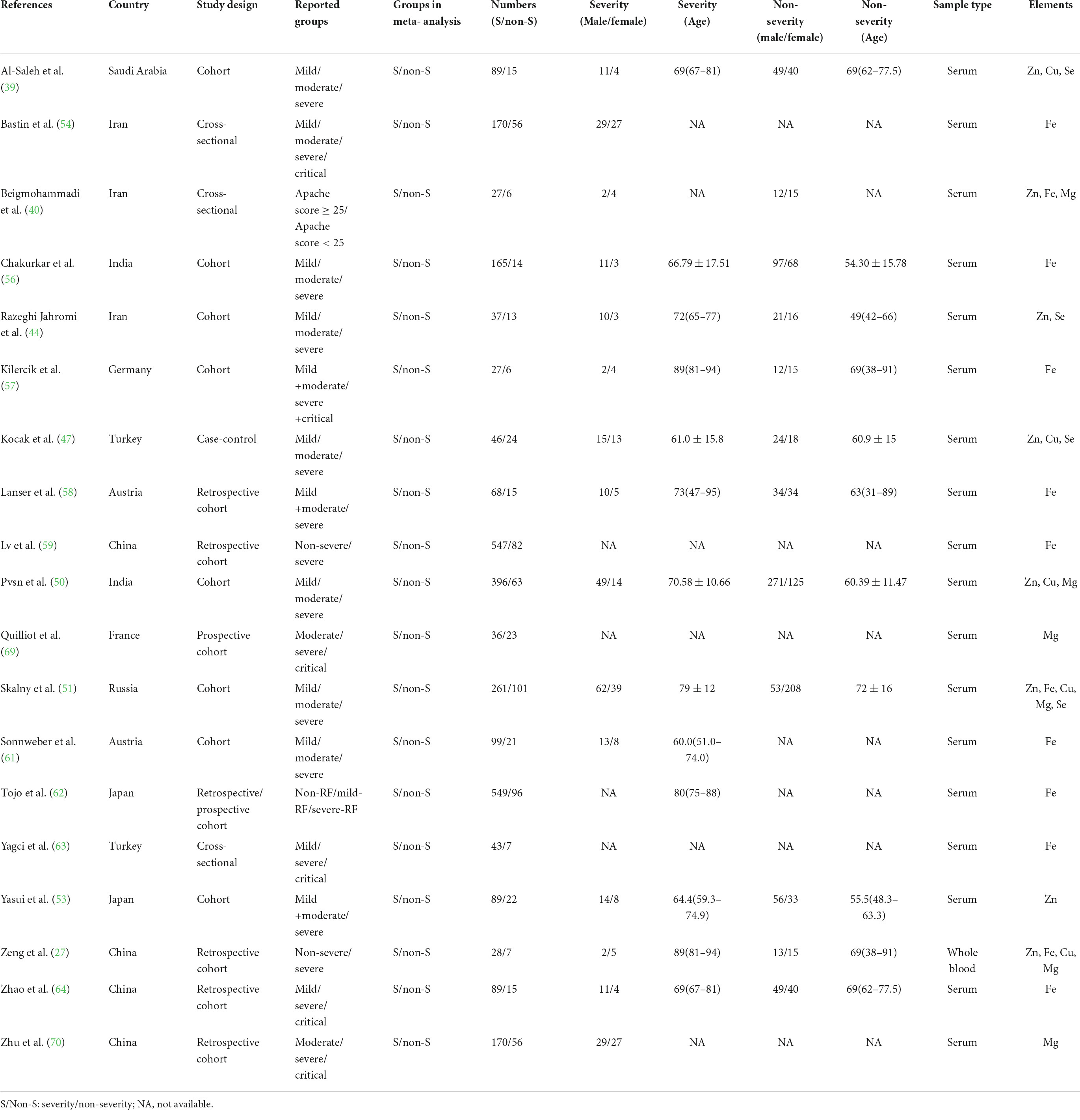
Table 2. The main characteristics of included studies in comparison of trace elements between severity status and non-severity status in COVID-19 patients.
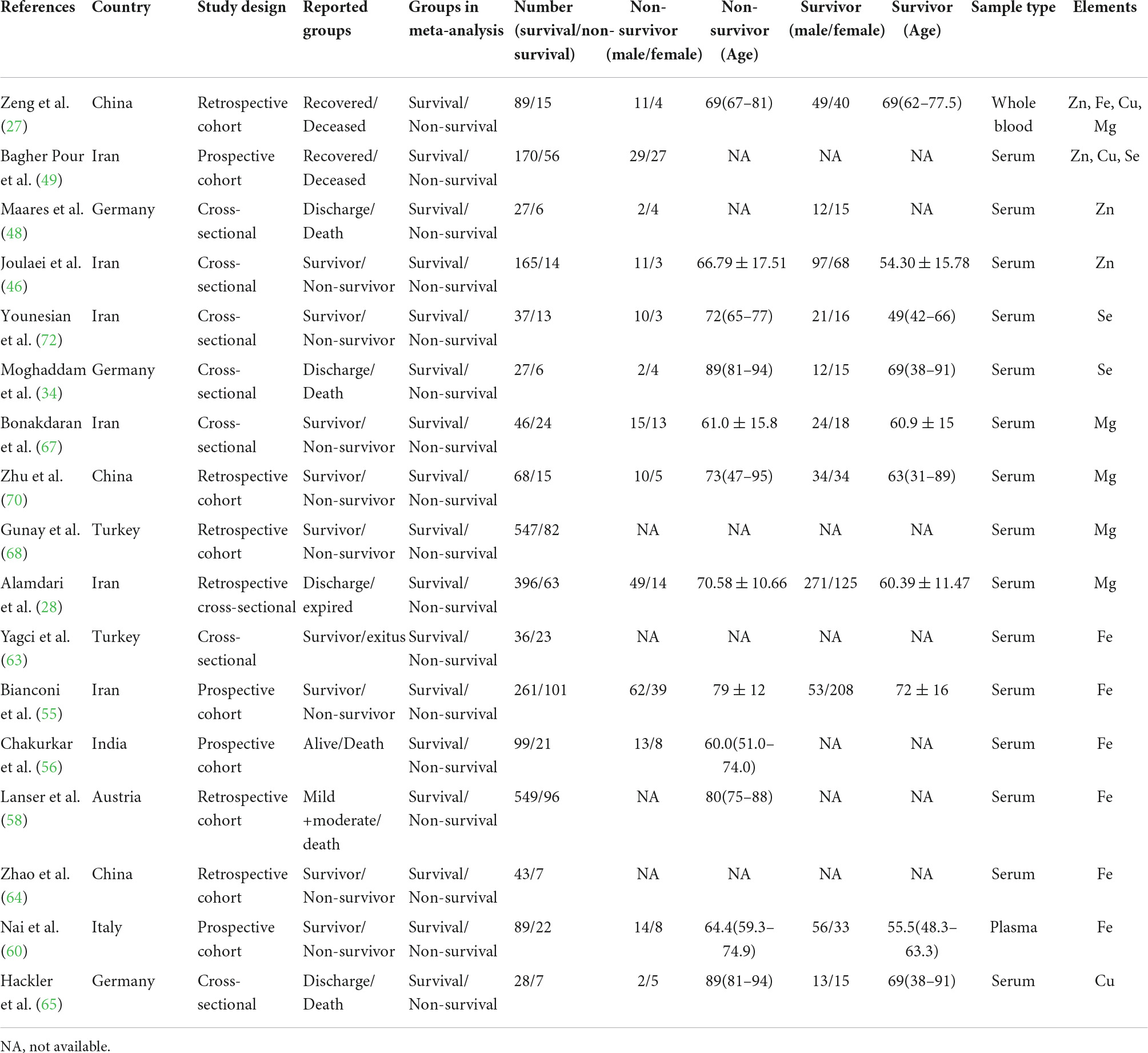
Table 3. The main characteristics of included studies in comparison of trace elements between survival and non-survival in COVID-19 patients.
Meta-analysis of trace elements in COVID-19 patients
Zn levels in COVID-19 patients
Figures 2A–C depicts the forest plots for the pooled estimates of the SMD on Zn levels between the COVID-19 patients and controls, severity and non-severity, and non-survivors and survivors. Due to the significant heterogeneity (COVID-19 patients vs. controls: I2 = 92.2%, P < 0.001; severity vs. non-severity: I2 = 72.5%, P < 0.001; survivors vs. non-survivors I2 = 98.5%, P < 0.001), a random-effect model was used. As shown in Figure 2A, combining 11 effective sizes from 11 studies showed that the overall SMD in Zn levels between the COVID-19 patients and controls was −0.83(−1.19 to 0.46, P < 0.001), indicating that COVID-19 patients had significantly lower Zn levels. Moreover, we evaluated the differences of Zn level between severity and non-severity, or survivors and non-survivors. The COVID-19 patients with severity status had significantly lower Zn levels than COVID-19 patients with non-severity status (SMD: −0.47, 95% CI: −0.75 to −0.18, P = 0.002). However, Zn levels in the non-survivors were not significantly different from the survivors in COVID-19 patients (SMD: −1.46, 95%CI: −3.98 to 1.06, P = 0.256). There was no significant publication bias in the analysis using Begg’s test (P = 0.350) and Egger’s test (P = 0.312, Figure 2D). As shown in Supplementary Figure 1, sensitivity analysis revealed that the exclusion of any single study had no significant impact on pooled SMD.
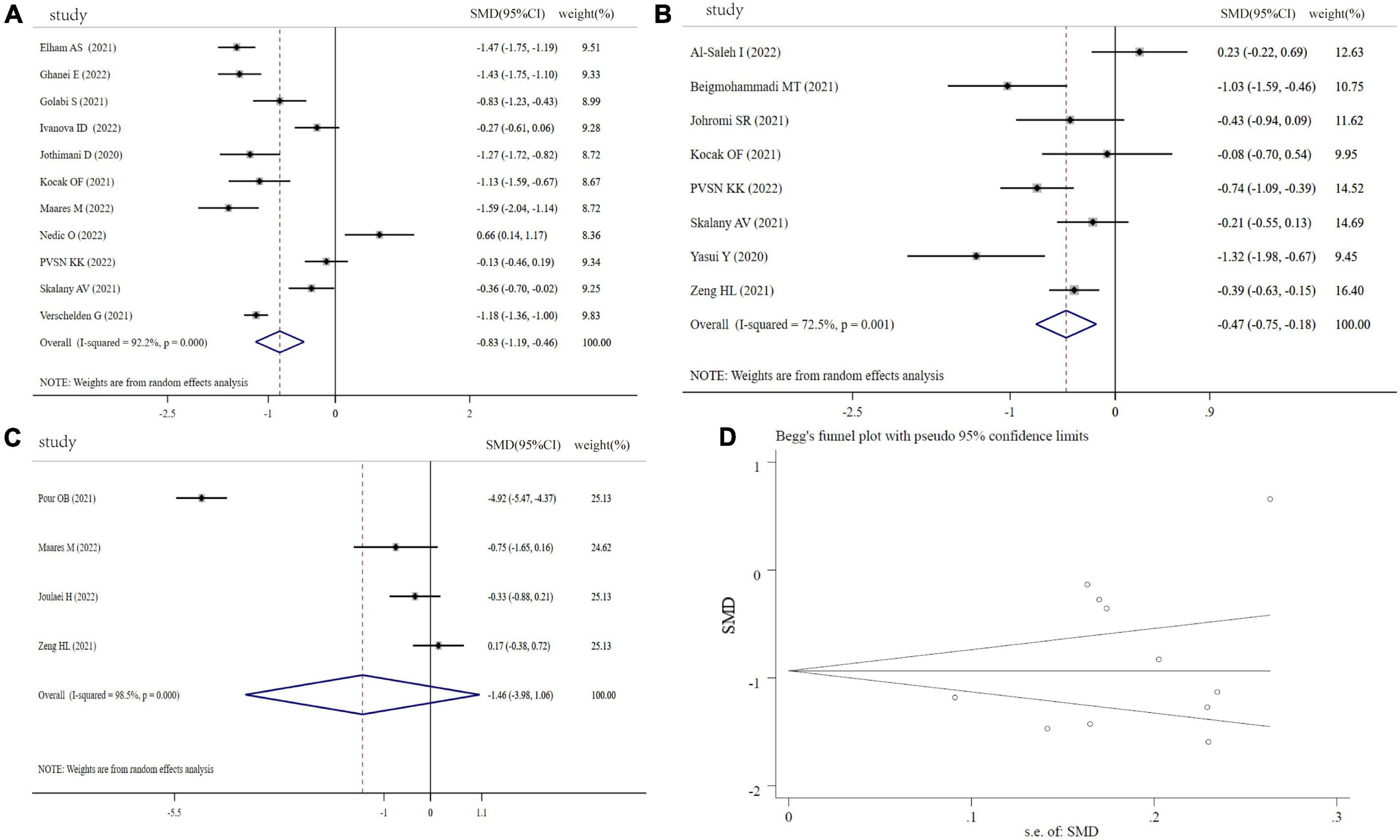
Figure 2. Forest plot of Zn levels. (A) Zn levels between COVID-19 patients and controls; (B) Zn levels between severity status and non-severity status in COVID-19 patients; (C) Zn levels between non-survivors and survivors in COVID-19 patients; (D) funnel plot of the meta-analysis on Zn levels between COVID-19 patients and controls.
Fe levels in COVID-19 patients
Fe levels were analyzed between COVID-19 patients and controls (4 studies), severity and non-severity status (12 studies), and the non-survivors and survivors (6 studies) in COVID-19 patients. Compared with controls, COVID-19 patients showed a significantly lower circulating levels of Fe (SMD: −1.56, 95% CI: −2.90 to −0.21, P = 0.023, Heterogeneity: I2 = 96.8%, P < 0.001), shown in Figure 3A. Figures 3B,C revealed that COVID-19 patients with severity status or non-survivors had a significant lower Fe levels than COVID patients with non-severe status or survivors (severity vs. non-severity: SMD: −0.45, 95% CI: −0.79 to −0.12, P = 0.008, Heterogeneity: I2 = 90%, P < 0.001; non-survivors vs. survivors: SMD: −0.28, 95% CI: −0.44 to −0.12, P < 0.001, Heterogeneity: I2 = 0%, P = 0.948). The sensitivity analysis demonstrated the stability of the pooled SMD (Supplementary Figure 2), and funnel plot, Begg’s test (P = 1.00) and Egger’s test (P = 0.711) displayed no significant publication bias (Figure 3D).
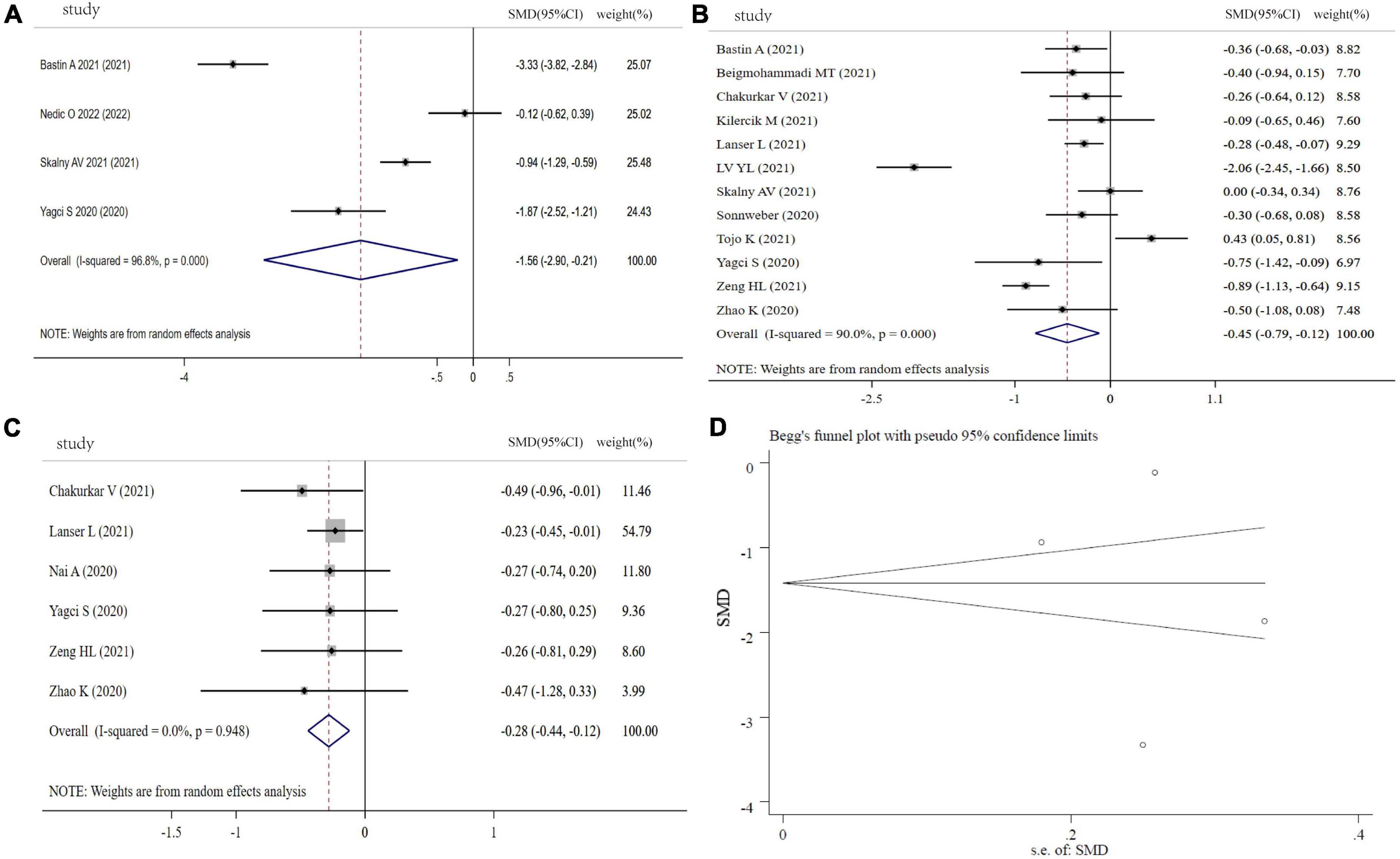
Figure 3. Forest plot of Fe levels. (A) Fe levels between COVID-19 patients and controls; (B) Fe levels between severity status and non-severity status in COVID-19 patients; (C) Fe levels between non-survivors and survivors in COVID-19 patients; (D) funnel plot of the meta-analysis on Fe levels between COVID-19 patients and controls.
Cu levels in COVID-19 patients
Figure 4A shows the results of six studies comparing the circulating Cu levels between COVID-19 cases and controls. Compared with the controls, the COVID-19 patients displayed no significant difference in Cu levels (SMD: 0.28, 95% CI: −0.15 to 0.70, P = 0.246, heterogeneity: I2 = 88.1%, P < 0.001). Moreover, we found that COVID−19 patients with severity status and the non-survivors had similar Cu levels in comparison with COVID-19 patients with non-severity status (SMD: 0.12, 95% CI: −0.27 to 0.50, P = 0.126, heterogeneity: I2 = 81.6%, P = 0.001) and the survivors (SMD: −1.16, 95% CI: −3.41 to 1.09, P = 0.312, heterogeneity: I2 = 97.5%, P < 0.001), respectively (Figures 4B,C). Sensitivity analyses generated similar results with the primary meta-analysis (Supplementary Figure 3). Based on funnel plot, Begg’s test (P = 0.707) and Egger’s test (P = 0.244), there was no publication bias in this meta-analysis (Figure 4D).
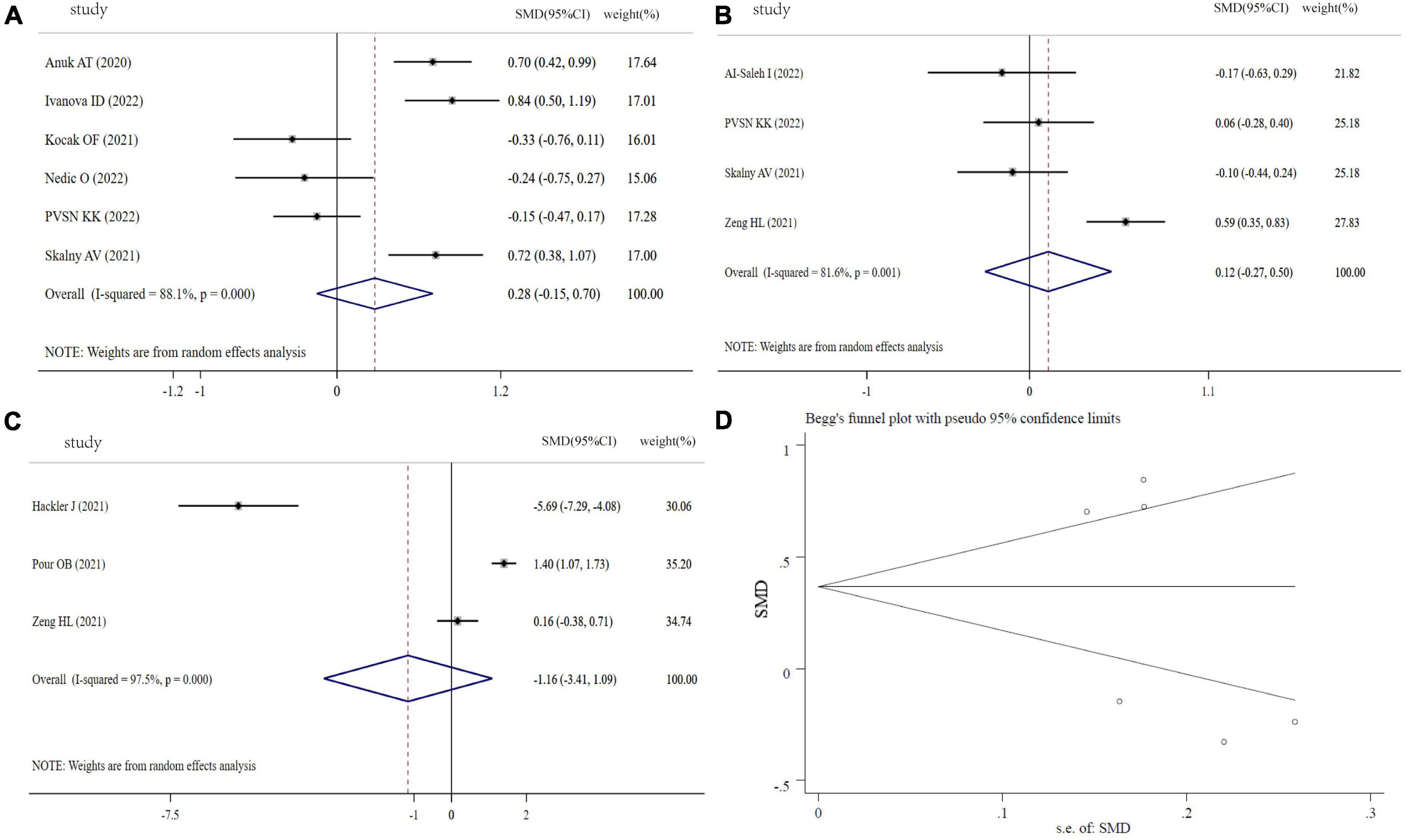
Figure 4. Forest plot of Cu levels. (A) Cu levels between COVID-19 patients and controls; (B) Cu levels between severity status and non-severity status in COVID-19 patients; (C) Cu levels between non-survivors and survivors in COVID-19 patients; (D) funnel plot of the meta-analysis on Cu levels between COVID-19 patients and controls.
Mg levels in COVID-19 patients
As indicated in Figure 5A, the SMD of circulating Mg levels between COVID-19 patients and controls was pooled from 3 studies, and no significant difference was found (SMD: −0.36, 95% CI: −0.76 to 0.05, P = 0.08, heterogeneity: I2 = 72.7%, P = 0.026). Figure 5B showed that there was no significant difference in COVID-19 patients between severity status and non-severity status (SMD: 0.40, 95% CI: −0.47 to 1.26, P = 0.30, heterogeneity: I2 = 96.8%, P < 0.001). The COVID-19 non-survivors had the same Mg levels with survivors (SMD: −0.35, 95% CI: −0.74 to 0.04, P = 0.079, heterogeneity: I2 = 80.8%, P < 0.001), as shown in Figure 5C. The sensitivity analysis suggested that the result is not due to the effect of any single study (Supplementary Figure 4). Visual inspection of funnel plot symmetry suggested a significant potential publication bias, examined by Begg’s test (P = 0.296) and Egger’s test (P = 0.004) (Figure 5D).
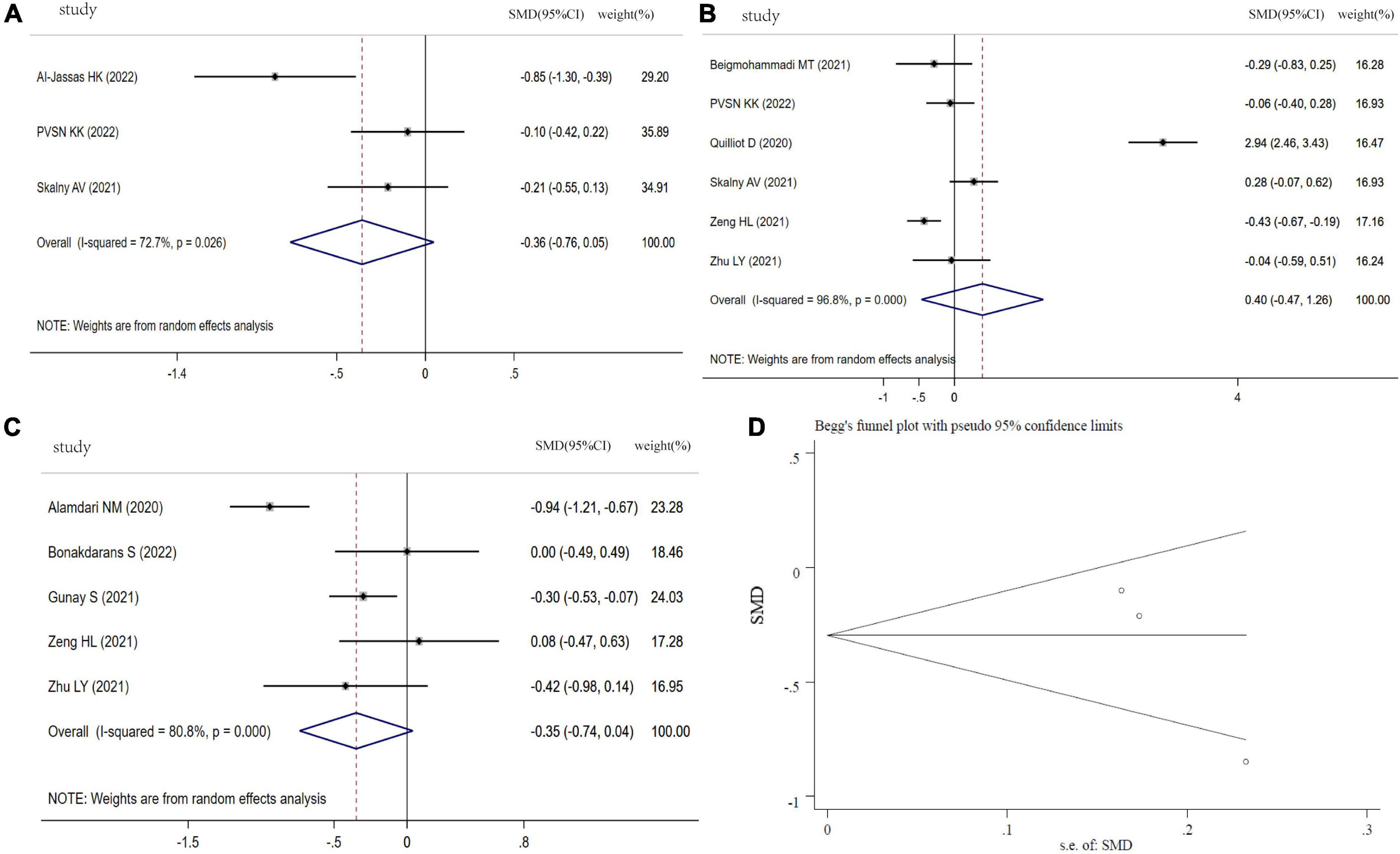
Figure 5. Forest plot of Mg levels. (A) Mg levels between COVID-19 patients and controls; (B) Mg levels between severity status and non-severity status in COVID-19 patients; (C) Mg levels between non-survivors and survivors in COVID-19 patients; (D) funnel plot of the meta-analysis on Mg levels between COVID-19 patients and controls.
Se levels in COVID-19 patients
Figure 6A showed the results of 5 studies comparing the Se levels between COVID-19 patients and controls. From this forest plot, COVID-19 patients had significantly decreased Se levels in comparison with controls (SMD: −0.75, 95% CI: −0.94 to −0.56, P < 0.001, heterogeneity: I2 = 0%, P = 0.52). Moreover, the pooled SMD of Se levels from 4 studies was significantly different between severity status and non-severity status (SMD: −0.27, 95% CI: −0.49 to −0.04, P = 0.02, heterogeneity: I2 = 45.2%, P = 0.140, Figure 6B). However, three studies focused on Se levels between non-survivors and survivors in COVID-19, and Se levels in non-survivors was not different with survivors (SMD: 0.10, 95% CI: −1.27 to 1.48, P = 0.882, heterogeneity: I2 = 96.8%, P < 0.001, Figure 6C). Sensitivity analysis indicated that the pooled SMD was not altered when any single study was excluded (Supplementary Figure 5). Funnel plot, Begg’s (P = 0.462) and Egger tests (P = 0.082) revealed no significant publication bias (Figure 6D).
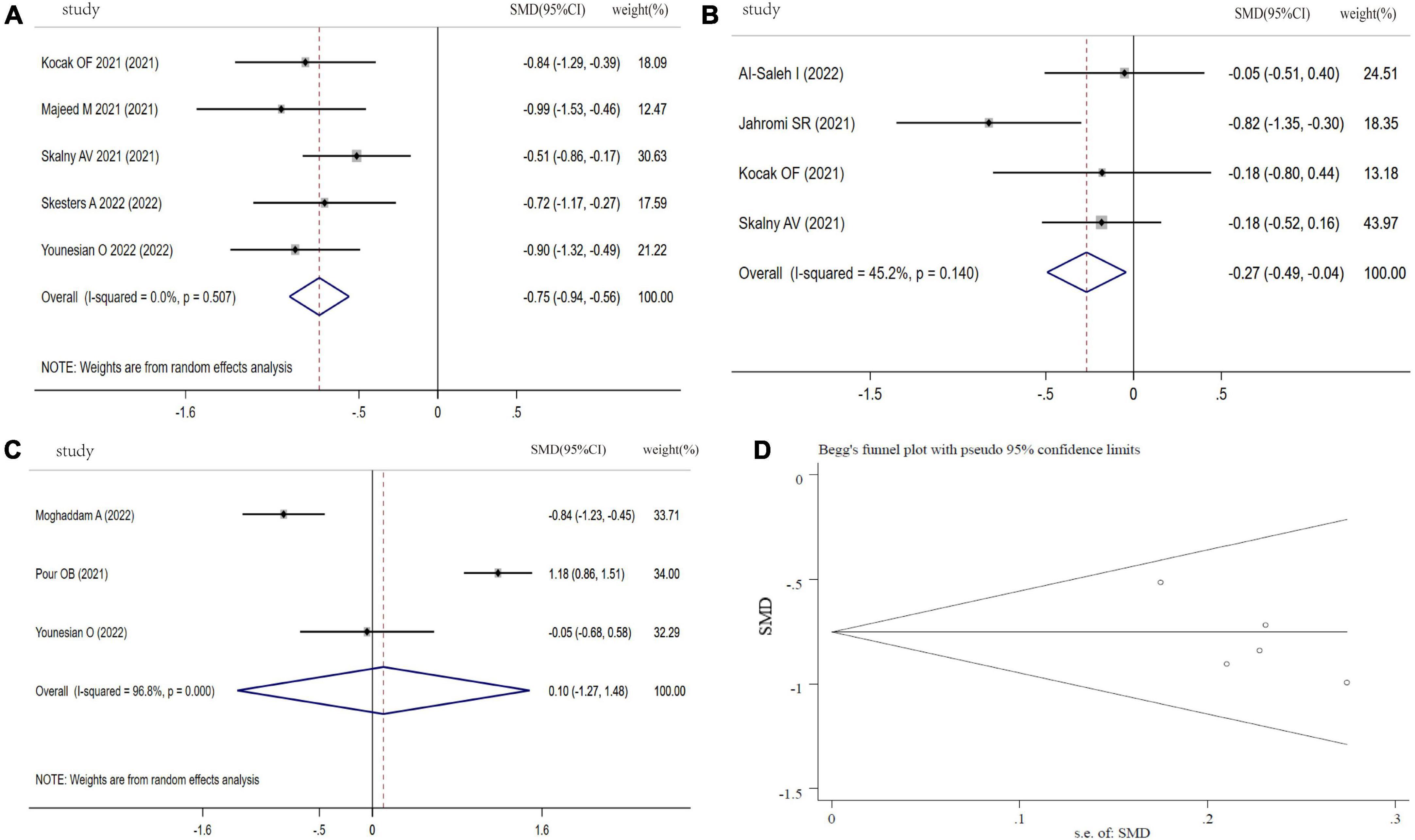
Figure 6. Forest plot of Se levels. (A) Se levels between COVID-19 patients and controls; (B) Se levels between severity status and non-severity status in COVID-19 patients; (C) Se levels between non-survivors and survivors in COVID-19 patients; (D) funnel plot of the meta-analysis on Se levels between COVID-19 patients and controls.
Discussion
COVID-19 has been a global pandemic for more than two years, and already have a great impact on our life quality and daily lifestyle. In view of the absence of specific medicine and effective vaccine, host immune function and nutritional status have been accepted the key factors in defending against SARS-CoV-2 attack. Among the nutritional factors, trace elements have been demonstrated to be involved in the modulation of immune responses, and their deficiencies act as the factors strongly affecting the course of COVID-19 disease (6). To our knowledge, this is the first meta-analysis to evaluate the levels of trace elements in COVID-19 patients, and explore the associations of trace elements and COVID-19 severity and poor outcomes. Our results support the potential clinical roles of Zn, Fe, Se in the evaluation of COVID-19 patients. Specially, circulating levels of Zn, Fe, and Se were significantly correlated with COVID-19 severity, and non-survivors in COVID-19 diseases displayed much lower Fe levels than survivors. This also reinforces the call for a specific nutritional management of COVID-19 patients.
Zn, as the second most abundant trace element in human body, depends on the daily food intake to achieve and maintain the adequate level of human tissues (73). The main functions of Zn include structural component, catalytic action, and regulatory function (74). Zn homeostasis is essential for anti-inflammatory, anti-redox reaction, development and differentiation of immune cells (such as T cell, B cell, natural killer cell, dendritic cell, and mast cell), and key cytokine release (such as IL-2, IL-6, and TNF-α) (75, 76). Previous studies have demonstrated Zn was involved in the overall functioning of human immune system, affecting innate immunity and adaptive immunity (77, 78). In addition, Zn also plays an important role in pathogens’ survival and the propagation of virulence(79). Rnai I, et al. indicated that nutritional intervention with Zn provided an adjutant therapy by eliciting their virucidal effects in the process of SARS-CoV-2 infection (80). Zn not only disrupted the balance of immune response, but also affected the expression of ACE2 receptors, which are required for SARS-CoV-2 entry into target cells (81). Moreover, Jennifer A Frontera, et al. reported that Zn was associated with increased rates of recovery and reduced risk of mortality among COVID-19 patients (82). A retrospective study showed high prevalence of Zn deficiency was positively associated with the COVID-19 cases/1 million populations in Asian countries (83). However, some studies showed controversial results on the relationship between Zn levels and COVID-19 infection and poor outcomes. Our meta-analysis result found that Zn level in COVID-19 patients was significantly lower than controls, and correlated with disease severity status.
Fe is a critical element involved in a variety of physiological functions, such as DNA biosynthesis, ATP generation, oxygen transport and storage, ROS production, energy production, and host defense (84). Disruption of Fe homeostasis is tightly associated with infection, cancer, cardiovascular disease, renal disease, and hematological disease(85, 86). Ferroptosis, a term coined in 2012, is an iron-dependent cell death pathway driven by excessive lipid peroxidation, which has been implicated in the development and disease of various tissues and organisms(87). Moreover, alteration of Fe distribution in COVID-19 patients is hypoferremia, and low Fe levels may impair hypoxia sensing and immunity (88). Hal et al. highlighted the evidence that Fe deficiency limited adaptive immunity and responses to vaccines (22). Therefore, The European Hematology Association (EHA) states that the populations should correct the Fe deficiency before administration of the COVID-19 vaccine. However, contradictory opinion suggested that the virus lead to the release of Fe from porphyrins by attacking and destroying hemoglobin, and the consequential result was the discharge of more Fe into the blood (89). Our pooled results showed that Fe levels in COVID-19 patients, severity status, and non-survivors were significantly lower than controls, non-severity status, and survivors, respectively.
Se is an indispensable trace element, necessary for human innate and acquired immunity, antibody production, muscle function, and signaling transduction pathways (90). Amounting evidence had demonstrated that Se deficiency was linked to higher susceptibility to RNA viral infection and poor outcome (91). Zhang et al. found an association between Se status and cure rate in COVID-19 patients in China (92). Alexander et al. made a full literature search and concluded that adequate supply of Se is essential for resistance to viral infections, immune function, and reduced inflammation, and mitigate the course of COVID-19 (32). However, Sobczyk et al. adopted a Mendelian Randomization (MR) analysis, and did not found the supplementation with Zn, Se, and Cu could prevent SARS-CoV-2 infection, critical illness or hospitalization for COVID-19 (93). A recent study also indicated that Se status or Se intake had no effect on humoral immune after vaccination (94). Our result showed that Se level in COVID-19 patients was significantly lower than controls, and the COVID-19 patients with severe status had lower Se level in comparison with non-severity status.
Cu plays an essential role in immune function and antioxidant defense (14). It is well-documented that Cu deficiency was associated with exceptional susceptibility to varied viral infections (95). The underlying mechanism by which Cu causes inaction of pathogens was still elusive (96). Mg, an essential substance, participates in many kinds of biochemical reactions, and also has anti-inflammation and anti-oxidant function. A nationwide retrospective cohort study including 1,150 counties, 287,326,503 individuals, and 5,401,483 COVID-19 confirmed cases revealed that the infection risk of the populations was distributed in low-magnesium areas in COVID-19’s early transmission (97). Tang et al. suggested that Mg supplementation may be a supportive treatment in COVID-19 patients (98). However, Cu and Mg, showed no difference between COVID-19 patients and controls, and had no relationship with disease severity and mortality in our meta-analysis. The possible reason is that the sample size is relative small, or the Mg and Cu levels in blood sample were not representatives of total Mg and Cu levels in body.
This study has several limitations. First, given the limited number of published literature, more evidence was needed to confirm the final results, especially for Mg, Cu, and Se. Second, it is possible that some undefined, or unreported factors might have contributed to the study heterogeneity. We did not conduct the subgroup analysis because of small samples. Third, some studies were retrospective studies, and the measurement methods were not described at all (e.g., laboratory assay, medical records, or self-reported). Forth, in view of study factors might not be independent of each other, we should perform the multivariable meta-regression. Having said that, it is possible that our results were substantially influenced by residential location, dietary habits, comorbidity, and population age. But, the majority of the retrieved articles were lack of full clinical information. Finally, publication bias was apparent in the comparison of Mg levels between COVID-19 patients and controls.
Conclusion
In conclusion, our meta-analysis showed that COVID-19 patients had lower circulating Zn, Fe, and Se levels than healthy controls, and their levels were associated with the presence of severity status. Moreover, circulating Fe levels may provide part of the explanation for the unfavorable survival status. We strongly recommend that future studies make efforts to conduct a larger, multi-regional, and representative patient samples in order to uncover the roles of trace elements. Despite some doubts in the benefits of the trace elements, we presumed optimistically that supplements of trace elements might provide an adjutant treatment in the early stages of COVID-19.
Data availability statement
The raw data supporting the conclusions of this article will be made available by the authors, without undue reservation.
Author contributions
BL and YL conceived and designed the experiments and collected literature. YL and WL analyzed the data. BL wrote the drafts of the manuscript. YL checked and revised the manuscript. All authors interpreted the data and reviewed and approved the final version of the manuscript.
Funding
This work was supported by the Research and Development Project of Liaoning Province (2020JH2/10300098).
Acknowledgments
We would like to thank He Qun and Li Yanshu for valuable help in online software usage and data analysis.
Conflict of interest
The authors declare that the research was conducted in the absence of any commercial or financial relationships that could be construed as a potential conflict of interest.
Publisher’s note
All claims expressed in this article are solely those of the authors and do not necessarily represent those of their affiliated organizations, or those of the publisher, the editors and the reviewers. Any product that may be evaluated in this article, or claim that may be made by its manufacturer, is not guaranteed or endorsed by the publisher.
Supplementary material
The Supplementary Material for this article can be found online at: https://www.frontiersin.org/articles/10.3389/fnut.2022.982032/full#supplementary-material
Supplementary Figure 1 | Sensitivity analysis of Zn levels between COVID-19 patients and controls.
Supplementary Figure 2 | Sensitivity analysis of Fe levels between COVID-19 patients and controls.
Supplementary Figure 3 | Sensitivity analysis of Cu levels between COVID-19 patients and controls.
Supplementary Figure 4 | Sensitivity analysis of Mg levels between COVID-19 patients and controls.
Supplementary Figure 5 | Sensitivity analysis of Se levels between COVID-19 patients and controls.
References
1. Capuzzo M, Amaral ACK, Liu VX. Assess COVID-19 prognosis. But be aware of your instrument’s accuracy! Intensive Care Med. (2021) 47:1472–4. doi: 10.1007/s00134-021-06539-3
3. Choi HM, Moon SY, Yang HI, Kim KS. Understanding viral infection mechanisms and patient symptoms for the development of COVID-19 therapeutics. Int J Mol Sci. (2021) 22:1737.
4. Villapol S. Gastrointestinal symptoms associated with COVID-19: impact on the gut microbiome. Transl Res. (2020) 226:57–69.
5. Zhou F, Yu T, Du R, Fan G, Liu Y, Liu Z, et al. Clinical course and risk factors for mortality of adult inpatients with COVID-19 in Wuhan, China: a retrospective cohort study. Lancet. (2020) 395:1054–62. doi: 10.1016/S0140-6736(20)30566-3
6. Fedele D, De Francesco A, Riso S, Collo A. Obesity, malnutrition, and trace element deficiency in the coronavirus disease (COVID-19) pandemic: an overview. Nutrition. (2021) 81:111016. doi: 10.1016/j.nut.2020.111016
7. Srivastava R, Kumar A. Use of aspirin in reduction of mortality of COVID-19 patients: a meta-analysis. Int J Clin Pract. (2021) 75:e14515.
8. Tamba BI, Leon MM, Petreus T. Common trace elements alleviate pain in an experimental mouse model. J Neurosci Res. (2013) 91:554–61. doi: 10.1002/jnr.23191
9. Cannas D, Loi E, Serra M, Firinu D, Valera P, Zavattari P. Relevance of essential trace elements in nutrition and drinking water for human health and autoimmune disease risk. Nutrients. (2020) 12:2074. doi: 10.3390/nu12072074
10. Gombart AF, Pierre A, Maggini S. A review of micronutrients and the immune system-working in harmony to reduce the risk of infection. Nutrients. (2020) 12:236.
11. Maggini S, Wintergerst ES, Beveridge S, Hornig DH. Selected vitamins and trace elements support immune function by strengthening epithelial barriers and cellular and humoral immune responses. Br J Nutr. (2007) 98(Suppl. 1):S29–35. doi: 10.1017/S0007114507832971
12. Nedic O, Sunderic M, Robajac D, Miljus G, Cetic D, Penezic A. Major trace elements and their binding proteins in the early phase of Covid-19 infection. J Biol Inorg Chem. (2022) 27:261–9. doi: 10.1007/s00775-022-01931-w
13. Kumar A, Kubota Y, Chernov M, Kasuya H. Potential role of zinc supplementation in prophylaxis and treatment of COVID-19. Med Hypotheses. (2020) 144:109848.
14. Fooladi S, Matin S, Mahmoodpoor A. Copper as a potential adjunct therapy for critically ill COVID-19 patients. Clin Nutr ESPEN. (2020) 40:90–1. doi: 10.1016/j.clnesp.2020.09.022
16. Franklin RB, Costello LC. The important role of the apoptotic effects of zinc in the development of cancers. J Cell Biochem. (2009) 106:750–7.
17. Jarosz M, Olbert M, Wyszogrodzka G, Mlyniec K, Librowski T. Antioxidant and anti-inflammatory effects of zinc. Zinc-dependent NF-kappaB signaling. Inflammopharmacology. (2017) 25:11–24.
18. Himoto T, Masaki T. Associations between zinc deficiency and metabolic abnormalities in patients with chronic liver disease. Nutrients. (2018) 10:88.
19. Wessels I, Rolles B, Slusarenko AJ, Rink L. Zinc deficiency as a possible risk factor for increased susceptibility and severe progression of Corona virus disease 19. Br J Nutr. (2022) 127:214–32. doi: 10.1017/S0007114521000738
20. Ivanova ID, Pal A, Simonelli I, Atanasova B, Ventriglia M, Rongioletti M, et al. Evaluation of zinc, copper, and Cu:Zn ratio in serum, and their implications in the course of COVID-19. J Trace Elem Med Biol. (2022) 71:126944. doi: 10.1016/j.jtemb.2022.126944
21. Girelli D, Marchi G, Busti F, Vianello A. Iron metabolism in infections: focus on COVID-19. Semin Hematol. (2021) 58:182–7.
22. Drakesmith H, Pasricha SR, Cabantchik I, Hershko C, Weiss G, Girelli D, et al. Vaccine efficacy and iron deficiency: an intertwined pair? Lancet Haematol. (2021) 8:e666–9. doi: 10.1016/S2352-3026(21)00201-5
23. Oppenheimer SJ. Iron and its relation to immunity and infectious disease. J Nutr. (2001) 131:616S–33S; discussion 33S–5S.
24. Savy M, Edmond K, Fine PE, Hall A, Hennig BJ, Moore SE, et al. Landscape analysis of interactions between nutrition and vaccine responses in children. J Nutr. (2009) 139:2154S–218S. doi: 10.3945/jn.109.105312
25. Trapani V, Rosanoff A, Baniasadi S, Barbagallo M, Castiglioni S, Guerrero-Romero F, et al. The relevance of magnesium homeostasis in COVID-19. Eur J Nutr. (2022) 61:625–36. doi: 10.1007/s00394-021-02704-y
26. Eskander M, Razzaque MS. Can maintaining optimal magnesium balance reduce the disease severity of COVID-19 patients? Front Endocrinol. (2022) 13:843152. doi: 10.3389/fendo.2022.843152
27. Zeng HL, Yang Q, Yuan P, Wang X, Cheng L. Associations of essential and toxic metals/metalloids in whole blood with both disease severity and mortality in patients with COVID-19. FASEB J. (2021) 35:e21392. doi: 10.1096/fj.202002346RR
28. Alamdari NM, Afaghi S, Rahimi FS, Tarki FE, Tavana S, Zali A, et al. Mortality Risk factors among hospitalized COVID-19 patients in a major referral center in Iran. Tohoku J Exp Med. (2020) 252:73–84. doi: 10.1620/tjem.252.73
29. Ma C, Hoffmann PR. Selenoproteins as regulators of T cell proliferation, differentiation, and metabolism. Semin Cell Dev Biol. (2021) 115:54–61.
30. Duntas LH. Selenium and inflammation: underlying anti-inflammatory mechanisms. Horm Metab Res. (2009) 41:443–7.
31. Talaie A, Jafary H, Faraji F, Malekirad AA. The serum oxidative stress biomarkers and selenium levels in a group of migraine patients compared with healthy controls: a case-control study. Biol Trace Elem Res. (2022). doi: 10.1007/s12011-021-03024-2. [Epub ahead of print].
32. Alexander J, Tinkov A, Strand TA, Alehagen U, Skalny A, Aaseth J. Early nutritional interventions with zinc, selenium and vitamin d for raising anti-viral resistance against progressive COVID-19. Nutrients. (2020) 12:2358. doi: 10.3390/nu12082358
33. Huang LJ, Mao XT, Li YY, Liu DD, Fan KQ, Liu RB, et al. Multiomics analyses reveal a critical role of selenium in controlling T cell differentiation in Crohn’s disease. Immunity. (2021) 54:1728–44.e7. doi: 10.1016/j.immuni.2021.07.004
34. Moghaddam A, Heller RA, Sun Q, Seelig J, Cherkezov A, Seibert L, et al. Selenium deficiency is associated with mortality risk from COVID-19. Nutrients. (2020) 12:2098.
35. Im JH, Je YS, Baek J, Chung MH, Kwon HY, Lee JS. Nutritional status of patients with COVID-19. Int J Infect Dis. (2020) 100:390–3.
36. Majeed M, Nagabhushanam K, Gowda S, Mundkur L. An exploratory study of selenium status in healthy individuals and in patients with COVID-19 in a south Indian population: the case for adequate selenium status. Nutrition. (2021) 82:111053. doi: 10.1016/j.nut.2020.111053
37. Muka T, Glisic M, Milic J, Verhoog S, Bohlius J, Bramer W, et al. A 24-step guide on how to design, conduct, and successfully publish a systematic review and meta-analysis in medical research. Eur J Epidemiol. (2020) 35:49–60. doi: 10.1007/s10654-019-00576-5
38. Moher D, Liberati A, Tetzlaff J, Altman DG, Group P. Preferred reporting items for systematic reviews and meta-analyses: the PRISMA statement. PLoS Med. (2009) 6:e1000097. doi: 10.1371/journal.pmed.1000097
39. Al-Saleh I, Alrushud N, Alnuwaysir H, Elkhatib R, Shoukri M, Aldayel F, et al. Essential metals, vitamins and antioxidant enzyme activities in COVID-19 patients and their potential associations with the disease severity. Biometals. (2022) 35:125–45. doi: 10.1007/s10534-021-00355-4
40. Beigmohammadi MT, Bitarafan S, Abdollahi A, Amoozadeh L, Salahshour F, Mahmoodi Ali Abadi M, et al. The association between serum levels of micronutrients and the severity of disease in patients with COVID-19. Nutrition. (2021) 91–2:111400.
41. Elham AS, Azam K, Azam J, Mostafa L, Nasrin B, Marzieh N. Serum vitamin D, calcium, and zinc levels in patients with COVID-19. Clin Nutr ESPEN. (2021) 43:276–82.
42. Ghanei E, Baghani M, Moravvej H, Talebi A, Bahmanjahromi A, Abdollahimajd F. Low serum levels of zinc and 25-hydroxyvitmain D as potential risk factors for COVID-19 susceptibility: a pilot case-control study. Eur J Clin Nutr. (2022). doi: 10.1038/s41430-022-01095-5. [Epub ahead of print].
43. Golabi S, Adelipour M, Mobarak S, Piri M, Seyedtabib M, Bagheri R, et al. The association between vitamin D and zinc status and the progression of clinical symptoms among outpatients infected with SARS-CoV-2 and potentially non-infected participants: a cross-sectional study. Nutrients. (2021) 13:3368. doi: 10.3390/nu13103368
44. Razeghi Jahromi S, Moradi Tabriz H, Togha M, Ariyanfar S, Ghorbani Z, Naeeni S, et al. The correlation between serum selenium, zinc, and COVID-19 severity: an observational study. BMC Infect Dis. (2021) 21:899. doi: 10.1186/s12879-021-06617-3
45. Jothimani D, Kailasam E, Danielraj S, Nallathambi B, Ramachandran H, Sekar P, et al. COVID-19: poor outcomes in patients with zinc deficiency. Int J Infect Dis. (2020) 100:343–9.
46. Joulaei H, Keshani P, Foroozanfar Z, Zamanian D, Hassani A, Parvizi F, et al. Serum zinc associated with immunity and inflammatory markers in Covid-19. Open Med (Wars). (2022) 17:702–11.
47. Kocak OF, Ozgeris FB, Parlak E, Kadioglu Y, Yuce N, Yaman ME, et al. Evaluation of serum trace element levels and biochemical parameters of COVID-19 patients according to disease severity. Biol Trace Elem Res. (2021) 200:3138–3146. doi: 10.1007/s12011-021-02946-1
48. Maares M, Hackler J, Haupt A, Heller RA, Bachmann M, Diegmann J, et al. Free zinc as a predictive marker for COVID-19 mortality risk. Nutrients. (2022) 14:1407. doi: 10.3390/nu14071407
49. Bagher Pour O, Yahyavi Y, Karimi A, Khamaneh AM, Milani M, Khalili M, et al. Serum trace elements levels and clinical outcomes among Iranian COVID-19 patients. Int J Infect Dis. (2021) 111:164–8. doi: 10.1016/j.ijid.2021.08.053
50. Pvsn KK, Tomo S, Purohit P, Sankanagoudar S, Charan J, Purohit A, et al. Comparative analysis of serum zinc, copper and magnesium level and their relations in association with severity and mortality in SARS-CoV-2 patients. Biol Trace Elem Res. (2022). doi: 10.1007/s12011-022-03124-7. [Epub ahead of print].
51. Skalny AV, Timashev PS, Aschner M, Aaseth J, Chernova LN, Belyaev VE, et al. Serum zinc, copper, and other biometals are associated with COVID-19 severity markers. Metabolites. (2021) 11:244.
52. Verschelden G, Noeparast M, Noparast M, Goossens MC, Lauwers M, Cotton F, et al. Plasma zinc status and hyperinflammatory syndrome in hospitalized COVID-19 patients: an observational study. Int Immunopharmacol. (2021) 100:108163. doi: 10.1016/j.intimp.2021.108163
53. Yasui Y, Yasui H, Suzuki K, Saitou T, Yamamoto Y, Ishizaka T, et al. Analysis of the predictive factors for a critical illness of COVID-19 during treatment relationship between serum zinc level and critical illness of COVID-19. Int J Infect Dis. (2020) 100:230–6. doi: 10.1016/j.ijid.2020.09.008
54. Bastin A, Shiri H, Zanganeh S, Fooladi S, Momeni Moghaddam MA, Mehrabani M, et al. Iron chelator or iron supplement consumption in COVID-19? The role of iron with severity infection. Biol Trace Elem Res. (2021). doi: 10.1007/s12011-021-03048-8. [Epub ahead of print].
55. Bianconi V, Mannarino MR, Figorilli F, Cosentini E, Batori G, Marini E, et al. The detrimental impact of elevated Ferritin to Iron ratio on in-hospital prognosis of patients with COVID-19. Expert Rev Mol Diagn. (2022) 22:469–78. doi: 10.1080/14737159.2022.2052047
56. Chakurkar V, Rajapurkar M, Lele S, Mukhopadhyay B, Lobo V, Injarapu R, et al. Increased serum catalytic iron may mediate tissue injury and death in patients with COVID-19. Sci Rep. (2021) 11:19618. doi: 10.1038/s41598-021-99142-x
57. Kilercik M, Ucal Y, Serdar M, Serteser M, Ozpinar A, Schweigert FJ. Zinc protoporphyrin levels in COVID-19 are indicative of iron deficiency and potential predictor of disease severity. PLoS One. (2022) 17:e0262487. doi: 10.1371/journal.pone.0262487
58. Lanser L, Burkert FR, Bellmann-Weiler R, Schroll A, Wildner S, Fritsche G, et al. Dynamics in anemia development and dysregulation of iron homeostasis in hospitalized patients with COVID-19. Metabolites. (2021) 11:653. doi: 10.3390/metabo11100653
59. Lv Y, Chen L, Liang X, Liu X, Gao M, Wang Q, et al. Association between iron status and the risk of adverse outcomes in COVID-19. Clin Nutr. (2021) 40:3462–9.
60. Nai A, Lore NI, Pagani A, De Lorenzo R, Di Modica S, Saliu F, et al. Hepcidin levels predict Covid-19 severity and mortality in a cohort of hospitalized Italian patients. Am J Hematol. (2021) 96:E32–5. doi: 10.1002/ajh.26027
61. Sonnweber T, Boehm A, Sahanic S, Pizzini A, Aichner M, Sonnweber B, et al. Persisting alterations of iron homeostasis in COVID-19 are associated with non-resolving lung pathologies and poor patients’ performance: a prospective observational cohort study. Respir Res. (2020) 21:276. doi: 10.1186/s12931-020-01546-2
62. Tojo K, Sugawara Y, Oi Y, Ogawa F, Higurashi T, Yoshimura Y, et al. The U-shaped association of serum iron level with disease severity in adult hospitalized patients with COVID-19. Sci Rep. (2021) 11:13431.
63. Yagci S, Serin E, Acicbe O, Zeren MI, Odabasi MS. The relationship between serum erythropoietin, hepcidin, and haptoglobin levels with disease severity and other biochemical values in patients with COVID-19. Int J Lab Hematol. (2021) 43(Suppl. 1):142–51. doi: 10.1111/ijlh.13479
64. Zhao K, Huang J, Dai D, Feng Y, Liu L, Nie S. Serum iron level as a potential predictor of coronavirus disease 2019 severity and mortality: a retrospective study. Open Forum Infect Dis. (2020) 7:ofaa250. doi: 10.1093/ofid/ofaa250
65. Hackler J, Heller RA, Sun Q, Schwarzer M, Diegmann J, Bachmann M, et al. Relation of serum copper status to survival in COVID-19. Nutrients. (2021) 13:1898.
66. Al-Jassas HK, Al-Hakeim HK, Maes M. Intersections between pneumonia, lowered oxygen saturation percentage and immune activation mediate depression, anxiety, and chronic fatigue syndrome-like symptoms due to COVID-19: a nomothetic network approach. J Affect Disord. (2022) 297:233–45. doi: 10.1016/j.jad.2021.10.039
67. Bonakdaran S, Layegh P, Hasani S, Afkhamizadeh M, Khorasani ZM, Mohebbi M, et al. The prognostic role of metabolic and endocrine parameters for the clinical severity of COVID-19. Dis Markers. (2022) 2022:5106342. doi: 10.1155/2022/5106342
68. Gunay S, Caliskan S, Sigirli D. Relationship of magnesemia with myocardial damage and mortality in patients with COVID-19. Magnes Res. (2021) 34:93–102. doi: 10.1684/mrh.2021.0485
69. Quilliot D, Bonsack O, Jaussaud R, Mazur A. Dysmagnesemia in Covid-19 cohort patients: prevalence and associated factors. Magnes Res. (2020) 33:114–22. doi: 10.1684/mrh.2021.0476
70. Zhu L, Bao X, Bi J, Lin Y, Shan C, Fan X, et al. Serum magnesium in patients with severe acute respiratory syndrome coronavirus 2 from Wuhan, China. Magnes Res. (2021) 34:103–13.
71. Skesters A, Kustovs D, Lece A, Moreino E, Petrosina E, Rainsford KD. Selenium, selenoprotein P, and oxidative stress levels in SARS-CoV-2 patients during illness and recovery. Inflammopharmacology. (2022) 30:499–503. doi: 10.1007/s10787-022-00925-z
72. Younesian O, Khodabakhshi B, Abdolahi N, Norouzi A, Behnampour N, Hosseinzadeh S, et al. Decreased serum selenium levels of COVID-19 patients in comparison with healthy individuals. Biol Trace Elem Res. (2022) 200:1562–7. doi: 10.1007/s12011-021-02797-w
73. Maret W, Sandstead HH. Zinc requirements and the risks and benefits of zinc supplementation. J Trace Elem Med Biol. (2006) 20:3–18.
74. Asl SH, Nikfarjam S, Majidi Zolbanin N, Nassiri R, Jafari R. Immunopharmacological perspective on zinc in SARS-CoV-2 infection. Int Immunopharmacol. (2021) 96:107630. doi: 10.1016/j.intimp.2021.107630
75. Wessels I, Fischer HJ, Rink L. Dietary and physiological effects of zinc on the immune system. Annu Rev Nutr. (2021) 41:133–75.
76. Foster M, Samman S. Zinc and regulation of inflammatory cytokines: implications for cardiometabolic disease. Nutrients. (2012) 4:676–94. doi: 10.3390/nu4070676
77. Brocard A, Dreno B. Innate immunity: a crucial target for zinc in the treatment of inflammatory dermatosis. J Eur Acad Dermatol Venereol. (2011) 25:1146–52. doi: 10.1111/j.1468-3083.2010.03934.x
78. Wessels I, Maywald M, Rink L. Zinc as a gatekeeper of immune function. Nutrients. (2017) 9:1286.
79. Djoko KY, Ong CL, Walker MJ, McEwan AG. The role of copper and zinc toxicity in innate immune defense against bacterial pathogens. J Biol Chem. (2015) 290:18954–61.
80. Rani I, Goyal A, Bhatnagar M, Manhas S, Goel P, Pal A, et al. Potential molecular mechanisms of zinc- and copper-mediated antiviral activity on COVID-19. Nutr Res. (2021) 92:109–28. doi: 10.1016/j.nutres.2021.05.008
81. Lee MC, Chen YK, Tsai-Wu JJ, Hsu YJ, Lin BR. Zinc supplementation augments the suppressive effects of repurposed NF-kappaB inhibitors on ACE2 expression in human lung cell lines. Life Sci. (2021) 280:119752. doi: 10.1016/j.lfs.2021.119752
82. Frontera JA, Rahimian JO, Yaghi S, Liu M, Lewis A, de Havenon A, et al. Treatment with zinc is associated with reduced in-hospital mortality among COVID-19 patients: a multi-center cohort study. Res Sq. (2020). doi: 10.21203/rs.3.rs-94509/v1. [Epub ahead of print].
83. Ali N, Fariha KA, Islam F, Mohanto NC, Ahmad I, Hosen MJ, et al. Assessment of the role of zinc in the prevention of COVID-19 infections and mortality: a retrospective study in the Asian and European population. J Med Virol. (2021) 93:4326–33. doi: 10.1002/jmv.26932
84. Haschka D, Hoffmann A, Weiss G. Iron in immune cell function and host defense. Semin Cell Dev Biol. (2021) 115:27–36.
85. von Haehling S, Jankowska EA, van Veldhuisen DJ, Ponikowski P, Anker SD. Iron deficiency and cardiovascular disease. Nat Rev Cardiol. (2015) 12: 659–69.
86. van Swelm RPL, Wetzels JFM, Swinkels DW. The multifaceted role of iron in renal health and disease. Nat Rev Nephrol. (2020) 16:77–98. doi: 10.1038/s41581-019-0197-5
87. Jiang X, Stockwell BR, Conrad M. Ferroptosis: mechanisms, biology and role in disease. Nat Rev Mol Cell Biol. (2021) 22:266–82.
88. Moreira AC, Teles MJ, Silva T, Bento CM, Alves IS, Pereira L, et al. Iron related biomarkers predict disease severity in a cohort of portuguese adult patients during COVID-19 acute infection. Viruses. (2021) 13:2482. doi: 10.3390/v13122482
89. Habib HM, Ibrahim S, Zaim A, Ibrahim WH. The role of iron in the pathogenesis of COVID-19 and possible treatment with lactoferrin and other iron chelators. Biomed Pharmacother. (2021) 136:111228. doi: 10.1016/j.biopha.2021.111228
90. Gorini F, Sabatino L, Pingitore A, Vassalle C. Selenium: an element of life essential for thyroid function. Molecules. (2021) 26:7084.
91. Hiffler L, Rakotoambinina B. Selenium and RNA virus interactions: potential implications for SARS-CoV-2 infection (COVID-19). Front Nutr. (2020) 7:164. doi: 10.3389/fnut.2020.00164
92. Zhang J, Taylor EW, Bennett K, Saad R, Rayman MP. Association between regional selenium status and reported outcome of COVID-19 cases in China. Am J Clin Nutr. (2020) 111:1297–9.
93. Sobczyk MK, Gaunt TR. The effect of circulating zinc, selenium, copper and vitamin K1 on COVID-19 outcomes: a Mendelian randomization study. Nutrients. (2022) 14:233.
94. Demircan K, Chillon TS, Sun Q, Heller RA, Klingenberg GJ, Hirschbil-Bremer IM, et al. Humoral immune response to COVID-19 mRNA vaccination in relation to selenium status. Redox Biol. (2022) 50:102242. doi: 10.1016/j.redox.2022.102242
95. Raha S, Mallick R, Basak S, Duttaroy AK. Is copper beneficial for COVID-19 patients? Med Hypotheses. (2020) 142:109814.
96. Anuk AT, Polat N, Akdas S, Erol SA, Tanacan A, Biriken D, et al. The relation between trace element status (zinc, copper, magnesium) and clinical outcomes in COVID-19 infection during pregnancy. Biol Trace Elem Res. (2021) 199:3608–17. doi: 10.1007/s12011-020-02496-y
97. Tian J, Tang L, Liu X, Li Y, Chen J, Huang W, et al. Populations in low-magnesium areas were associated with higher risk of infection in COVID-19’s early transmission: a nationwide retrospective cohort study in the United States. Nutrients. (2022) 14:909. doi: 10.3390/nu14040909
Keywords: SARS-CoV-2, COVID-19, trace element, serum, prognosis, meta-analysis
Citation: Li Y, Luo W and Liang B (2022) Circulating trace elements status in COVID-19 disease: A meta-analysis. Front. Nutr. 9:982032. doi: 10.3389/fnut.2022.982032
Received: 13 July 2022; Accepted: 27 July 2022;
Published: 12 August 2022.
Edited by:
Ronan Lordan, University of Pennsylvania, United StatesReviewed by:
Amruta Naik, University of Pennsylvania, United StatesAshish Kothari, All India Institute of Medical Sciences, Rishikesh, India
Anoop Kumar, Delhi Pharmaceutical Sciences and Research University, India
Copyright © 2022 Li, Luo and Liang. This is an open-access article distributed under the terms of the Creative Commons Attribution License (CC BY). The use, distribution or reproduction in other forums is permitted, provided the original author(s) and the copyright owner(s) are credited and that the original publication in this journal is cited, in accordance with accepted academic practice. No use, distribution or reproduction is permitted which does not comply with these terms.
*Correspondence: Bin Liang, bliang@cmu.edu.cn