- 1School of Health Sciences, College of Health, Massey University, Palmerston North, New Zealand
- 2Riddet Institute, Massey University, Te Ohu Rangahau Kai Facility, Palmerston North, New Zealand
- 3Alpha-Massey Natural Nutraceutical Research Centre, Massey University, Palmerston North, New Zealand
- 4Food Experience and Sensory Testing Laboratory, School of Food and Advanced Technology, Massey University, Palmerston North, New Zealand
- 5Department of Human Nutrition, University of Otago, Dunedin, New Zealand
- 6High-Value Nutrition National Science Challenge, Auckland, New Zealand
Background and aims: Daily kiwifruit (KF) consumption has been associated with improved sleep quality, but underlying physiological mechanisms are unknown. This study examined acute effects of fresh and dried green KF, compared with a water control, on sleep quality, mood, and urinary serotonin and melatonin metabolite concentrations.
Methods: 24 men (age: 29 ± 1 years, body mass index: 24 ± 1 kg/m2) with poor (n = 12) or good (n = 12) sleep quality participated in a randomized, single-blind crossover study. One of three treatments was consumed with a standardized evening meal; (1) the flesh of two fresh green KF, (2) dried green KF powder (including skin; equivalent to dry matter of two fresh KF) mixed with water, or (3) a water control, in their own home. Subjective and objective sleep quality, mood, waking urinary 5-hydroxyindoleacetic acid (5-HIAA), 6-sulfatoxymelatonin (aMT6s), vitamin C and B-vitamin concentrations were determined.
Results: Regardless of sleep quality group, compared to control, morning sleepiness, alertness upon awakening, and vigor were improved (p < 0.05) after dried KF consumption. Compared to control, both fresh and dried KF treatments tended (p < 0.1) toward improved esteem and total mood disturbance. Both KF treatments increased (fresh +1.56 ± 0.4 ng/g, p = 0.001; dried: +1.30 ± 0.4 ng/g, p = 0.004) urinary concentration of the serotonin metabolite 5-HIAA compared to the control (4.32 ± 0.4 ng/g). In poor sleepers, ease of awakening improved by 24% after dried KF consumption (p = 0.005) and tended to improve by 13% after fresh KF intake (p = 0.052) compared to the control. Good sleepers tended toward 9% improved ratings of getting to sleep with fresh KF (p = 0.053) compared to the control. Poor sleepers had lower amounts of some B-vitamins compared to good sleepers (p < 0.05).
Conclusion: Consumption of dried or fresh KF with a standard evening meal, was associated with improved aspects of sleep quality and mood, possibly mediated through changes in serotonin metabolism.
Clinical trial registration: [www.anzctr.org.au], identifier [ACTRN12621000046808].
1. Introduction
Sleep is essential for the cellular repair of the body. Inadequate sleep is associated with increased health risks such as inflammation, diabetes, hypertension, and obesity. (1–5). Insufficient sleep can also lower cognitive performance and cause mood changes (6). One night of sleep disturbance can affect a person’s ability to concentrate; increasing technical errors and their overall mood the following day (7–9). Sleep quality is an assessment of a person’s contentment with their sleep period. This includes measures of one’s sleep duration, latency, efficiency, and waking after sleep. Good sleep quality has positive effects including improved daily function and feeling rested. Poor sleep quality outcomes include increased irritability and fatigue (10).
The circadian clock in the hypothalamus, which is affected by the light and dark cycles, controls sleep–wake cycles in humans. The neurohormone melatonin is secreted from pinealocytes in the pineal gland, and its precursor serotonin plays a vital role in regulating this. The urinary melatonin metabolite 6-sulfatoxymelatonin (aMT6s) is related to subjective and objective sleep quality measures (11) and the urinary concentration of the serotonin metabolite 5-hydroxyindoleacetic acid (5-HIAA) is related to mood (12). Moreover, first-morning urine concentration of aMT6s accurately reflected peak and total nocturnal plasma melatonin secretion (13).
The consumption of fruit and vegetables is known to affect plasma and urinary melatonin and serotonin metabolite concentrations. Consumption of vegetables (sweet corn, bitter gourd, Japanese radish sprout, shumeji and shiitake mushrooms) (14), tomatoes (15), and cherries (16), which are rich in melatonin, increased morning urinary aMT6s concentration (14–16) and improved sleep quality (15–17). Consumption of serotonin-rich fruits, such as kiwifruit (KF) (18) and a Jerte Valley cherry-based product (19) increased urinary levels of 5-HIAA (19, 20) and mood (19). Additionally, vitamin C is a co-factor involved in catecholamine biosynthesis and reducing oxidative stress. Studies have also shown increased urinary levels of vitamin C when consuming vitamin C-rich foods. B-vitamins are also important co-factors involved in catecholamine biosynthesis. Furthermore, daily consumption of two green KF 1 h before bed for 4 weeks was associated with improved sleep quality (21). KF is high in serotonin, vitamin C and B-vitamins (22). However, the relationships between biochemical measures and sleep quality with KF consumption are unknown.
The aim of this study was to determine the effect of consuming the flesh of two fresh green KF (without the skins), whole freeze-dried green KF (with the skins included; equivalent to two green KF), or a water control with a standardized evening meal on urinary concentrations of metabolites of melatonin, serotonin, vitamin C and B-vitamins as well as objective (actigraphy) and subjective sleep quality and mood measures in healthy young men. We hypothesized that sleep quality and mood would improve and urinary metabolite concentrations would increase after the KF interventions compared to the control intervention.
2. Materials and methods
2.1. Participant recruitment and screening
Twenty-four healthy men (eligibility: age 18–35 years, Body Mass Index (BMI) 18.5–30 kg/m2) were recruited by advertisement from the Massey University campus and community in Palmerston North, Manawatu, New Zealand, between January 2021 and May 2021 amid the COVID-19 pandemic. The Pittsburgh Sleep Quality Index (PSQI) was used to determine participants subjective sleep quality. Participants were classified as a ‘good sleeper’ when their global PSQI score was ≤ 5 and as a ‘poor sleeper’ when their global PSQI score was > 5 (23). Exclusion criteria included smoking, excessive alcohol intake of > 21 standard drinks per week, use of prescribed or non-prescribed medications and antibiotics, physically active for more than 2 hours a day, food intolerances and allergies, consuming a vegan/vegetarian diet, gastrointestinal disorders; chronic conditions, such as cardiorespiratory, diabetes mellitus, high cholesterol/blood pressure; psychiatric conditions; diagnosed sleep conditions and working night shift or irregular work hours. In addition, participants who experienced significant weight loss (>5%) 3 months prior to the start of the study or consumed strict diets were excluded.
The study protocol was approved by the Massey University Human Ethics Committee (Massey University HEC: Southern A application-20/52), and the study was conducted according to the guidelines in the Declaration of Helsinki. The study was registered as a clinical trial with the Australian New Zealand Clinical Trial Registry.1 All participants provided written informed consent before the clinical trial and could withdraw at any time for any reason.
2.2. Study design, intervention, and protocol
The intervention was a randomized, single-blind, cross-over study. The study involved two green KF interventions of different forms and a control. The two KF interventions were: (1) flesh of two fresh green KF (Actinidia deliciosa cv. Hayward) (flesh only; approximate 200 g), (2) freeze-dried whole (flesh and skin), green KF (32 g, equivalent to the dry matter of two fresh green KF) with 200 ml of water, or (3) a control of 200 ml of water. The nutritional composition of each treatment is shown in Table 1. The researcher administering and analyzing the data (AK) was blinded to treatment allocation until completion of analyzes, and a separate researcher (CG) was unblinded and responsible for preparing the interventions. The researcher preparing the interventions was not involved in analyzing the data.
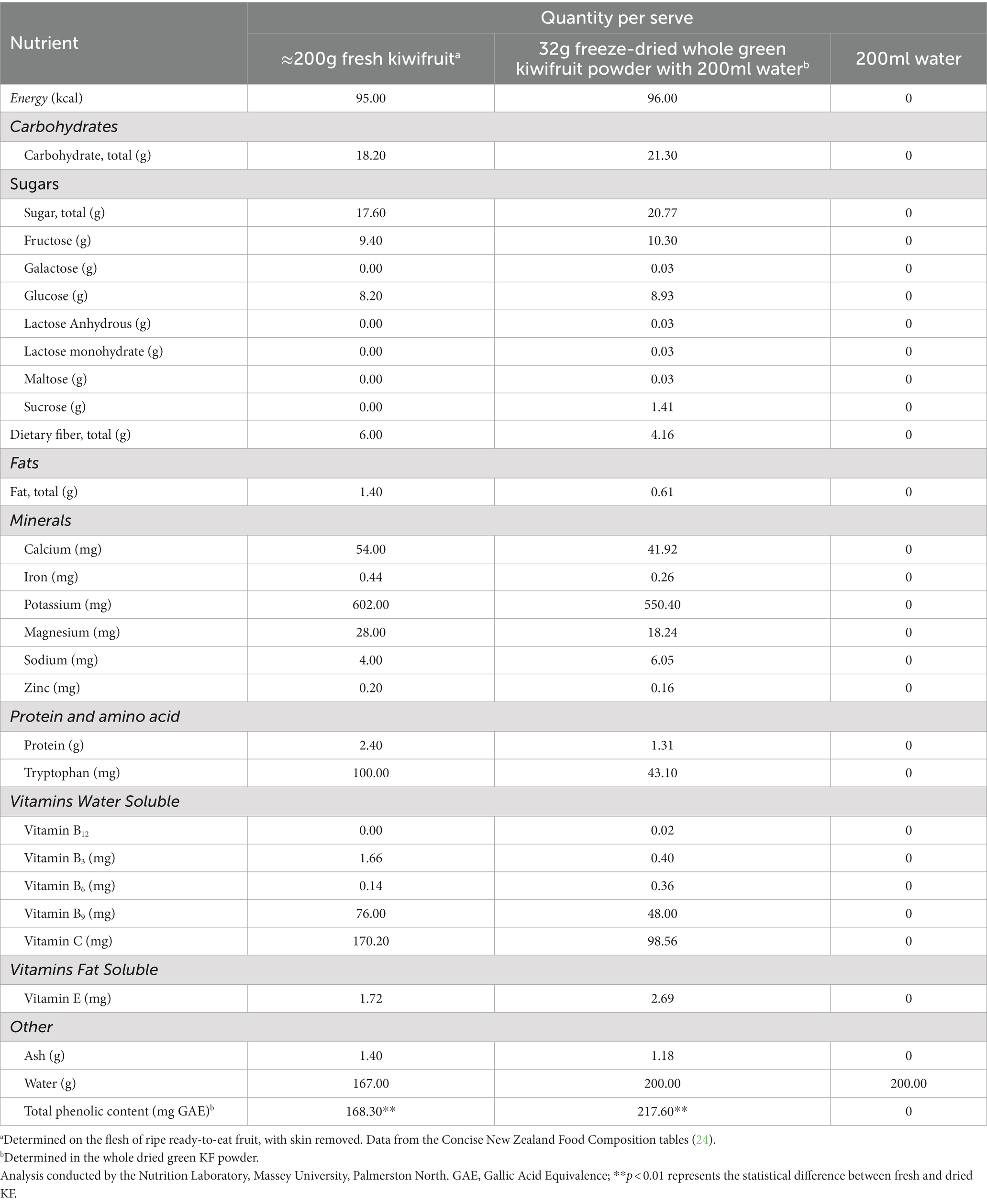
Table 1. Nutritional composition of total daily treatments for the fresh kiwifruit, freeze-dried kiwifruit, and control (water).
Randomization was conducted using a 6 × 3 Williams Design balanced for the order of presentation and carryover effects. The trial CONSORT flow diagram is shown in Figure 1. The study was conducted in the participant’s home environment. Before starting the study evenings, a familiarization evening occurred one to three days before the first evening. The same procedure described below was followed without providing a standardized evening meal and intervention.
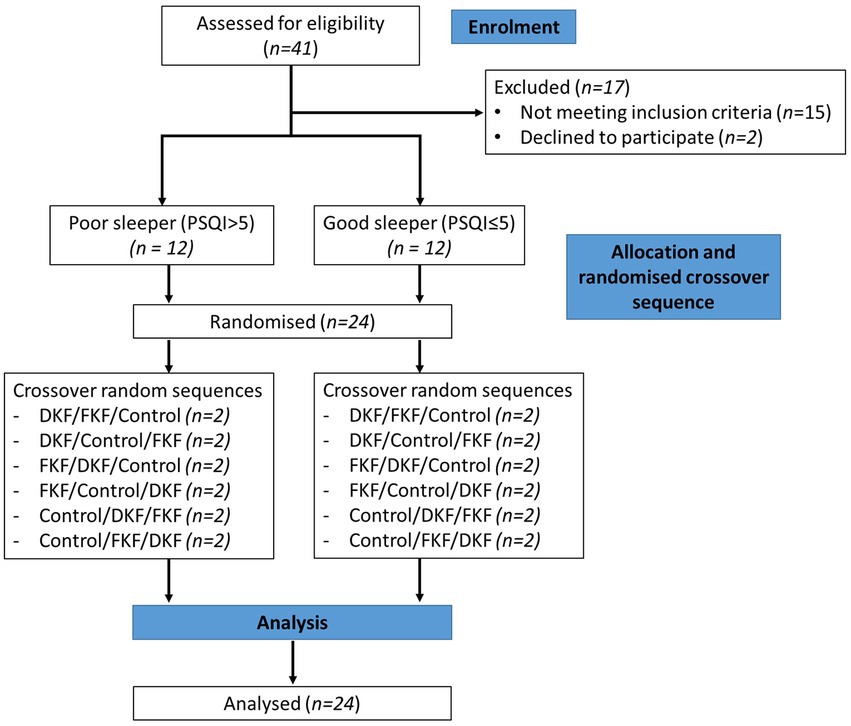
Figure 1. Consolidated Standards of Reporting Trials (CONSORT) flow diagram of the recruitment, enrolment, and random assignment process. DKF (Dried KF—32 g freeze-dried green KF powder), FKF (fresh KF—approx. 200 g fresh green KF).
Participants were studied on three separate occasions, separated by six to eight days. Each participant consumed an intervention with a standardized evening meal [Pub Size Spaghetti and Meatballs (McCain Foods), ∼720 kcal, P 26.5 g, C 75.0 g, F 33.5 g] Enrolled participants were asked to refrain from eating KF from enrolment until the completion of the study period. On the day of the study, participants were asked to collect a data collection pack (Figure 2). The pack contained the evening meal, an intervention they were randomized to receive, an actigraphy watch, a urine sample collection container, and a survey booklet. It was confirmed that the participant refrained from consuming any restricted foods during the two days prior to their study day. The restricted foods included oranges, pineapples, bananas, mangos, papayas, plums, grape, cherries, strawberries, tomatoes, capsicum, pistachios, plantains, mushrooms, chocolate, teas, coffee, and caffeinated beverages as they are known to contain and alter levels of serotonin and melatonin in urine (20, 25, 26).
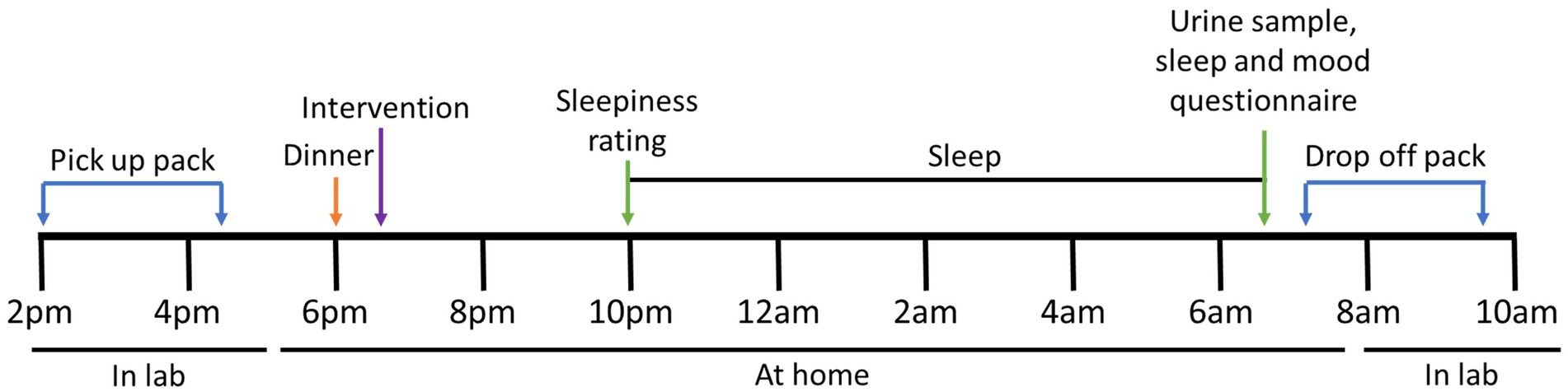
Figure 2. Study protocol for each intervention. At approximately 6 pm, the participant consumed a standardized evening dinner followed by one of three interventions. Before bedtime, at approximately 10–11 pm, the participant was asked to rate their sleepiness. Upon waking the next morning, a urine sample was collected, and the survey booklet was completed. The sample was delivered to the laboratory within 2 h of collection.
The participant consumed the standardized evening meal 4 h before their usual sleep time, followed immediately by the allocated intervention taking note of the time. Participants were asked not to eat any food or drinks, except water, until the following morning. Immediately before going to bed, participants were asked to rate their sleepiness level using the Stanford Sleepiness Scale (SSS). The participant was asked to activate the phase marker on the actigraphy watch when they were in bed and ready to sleep. Upon waking the following day, participants were asked to activate the phase marker, collect the whole first-morning urine sample noting the time, and complete a set of surveys including another SSS, Leeds Sleep Evaluation Questionnaire (LSEQ), and the abbreviated Profile of Mood States (POMS). The urine sample and surveys were delivered to the laboratory within 2 h of waking (Figure 2). Arrangements were made if the participant could not deliver samples within this time.
2.2.1. Subjective measures for sleep quality and mood
Two questionnaires to measure subjective sleep quality were used. The SSS measures sleepiness, consisting of a one-item scale; the participants select one of seven statements that best represent their current sleepiness. For example, a score of one indicates feeling active, vital, and alert, while a score of seven indicates no longer fighting sleep, sleep onset soon (27). The LSEQ is ten Visual Analog Scales (VAS) as a subjective self-measure to assess sleep quality changes throughout psychopharmacological treatment interventions (28). The scale evaluates four domains of sleep: (i) ease of getting to sleep (mean of questions 1, 2 and 3), (ii) quality of sleep (mean of questions 4 and 5), (iii) ease of awakening from sleep (mean of questions 6 and 7), and (iv) alertness upon awakening (mean of questions 8, 9, and 10). A 100 mm VAS scale was used for scoring, and scores were averaged per domain to determine the domain score. Higher scores indicate a better sleep quality domain.
Mood was assessed using the abbreviated POMS questionnaire (29). This form contains 40 mood-related adjectives rated on a 5-point Likert-type scale, ranging from 0 (not at all) to 4 (extremely). The data is then categorized into seven mood scales (maximum scores indicated): (i) tension, (ii) depression, (iii) anger, (iv) fatigue, (v) confusion, (vi) vigor, and (vii) esteem. Finally, a Total Mood Score (TMD) was calculated by adding tension, depression, anger, fatigue, and confusion scores, then subtracting the vigor and esteem scores.
2.2.2. Objective sleep measures
Participants were provided with an Actiwatch Spectrum Plus (Philips Respironics, Murrysville, Pennsylvania, United States) to wear on the wrist of their non-dominant arm during the study evenings. The medium threshold setting was used due to its use in studies using actigraphy to assess sleep (30), and 30 s was selected as the epoch length. The data was downloaded and subject to a mathematical algorithm in Actiware 6.0.9 (Philips Respironics, Murrysville, Pennsylvania, USA) to quantify sleep onset latency, sleep efficiency, total sleep time, wake after sleep onset, number of awakenings and the average length of awakenings.
2.2.3. Biochemical measures
Urine samples were collected in 500 ml containers with 1.0 g of ethylenediaminetetraacetic acid (EDTA). Participants were provided with two containers. Upon arriving at the laboratory, urine samples were weighed, and their volume was recorded. The urine was aliquoted into 1.5 ml Eppendorf tubes and stored at -80°C until analysis of aMT6s and vitamin C. Ten milliliters of urine were acidified with 6 M HCL and aliquoted and stored at -80°C until analysis of 5-HIAA. Urinary aMT6s and 5-HIAA analyzes were analyzed according to the manufacturer’s instructions using an enzyme-linked immunosorbent assay (ELISA) kit (IBL International, Hamburg, Germany). Assays were performed in duplicate and averaged. The average intra-assay coefficient of variance for the aMT6s assay was 5.2%, and the inter-assay was 2.9%. The average intra-assay coefficient of variance for the 5-HIAA assay was 7.5%, and the inter-assay was 3.5%. The ascorbate content of the samples was determined by reverse-phase high-performance liquid chromatography (HPLC) with electrochemical detection (31). B-vitamins and vitamers were measured using high-performance liquid chromatography coupled with mass spectrometry (UHPLC–MS/MS) technique (32). The B-vitamins measured included pantothenic acid, 4-pyridoxic acid, nicotinic acid, nicotinamide, nicotinuric acid, pyridoxal, biotin, riboflavin, folic acid, pyridoxamine and thiamine. The concentrations of measured metabolites and vitamins were corrected to creatinine to adjust for variation in urine dilution. Creatinine was measured using the colorimetric Jaffe method (33) (Nutrition Laboratory, Massey University).
2.3. Data and statistical analysis
A power analysis was performed using ease of getting to sleep VAS from LSEQ as the dependent variable to estimate the required sample size. Estimates of variance components were conducted based on data from a study examining caffeine ingestion’s effect on VAS getting to sleep in healthy subjects (34). When there are three treatments and a sample size of two in each of the six sequences, a 6 × 3 Williams Crossover inequality test of paired differences will have 94.2% power to detect a minimum difference of 20 mm or greater, assuming that the standard deviation of the paired differences is 13 mm (34) at the 5% significance level. The Bonferroni adjustment was used to keep the family-wise error at the specified error level. Thus, with the total number of pairwise comparisons equal to 3, each pairwise test was at the two-sided 1.67% significance level.
Statistical analyzes were performed using SPSS software (version 25; IBM, Armonk, NY, United States). No outliers for any outcome measures were noted by examination of studentized residuals for values greater than ±3. A paired t-test was used to compare nutritional compositional and demographic data. Effects of sleeper type and treatment and their interaction effect were determined using a repeated measures mixed-effects model. An unstructured covariance structure was used to account for the repeated treatments by the subject. Post hoc comparisons, adjusted for multiple comparisons using Bonferroni’s correction, were performed when there were significant main or interaction effects. Residual plots were inspected to confirm that the normality and constant variance model assumptions were met. Statistical significance of the mixed effects models was accepted at a probability inferior to 0.05 (p < 0.05). A trend was noted at a probability lower than 0.10 but higher than 0.05. To assess the within-subject correlations between urinary metabolites and sleep quality and mood measures, a univariate model with sleep and mood measures as the dependent variable, urinary metabolites as the covariate, and subject as the fixed factor were performed (35). Correlations with p < 0.05 and R-value > ± 0.4 were considered significant (36). All data are presented as means ± standard errors of the mean (SEM).
3. Results
3.1. Participants
Twenty-four young men (18–35 years) completed the study: 12 with good sleep quality (age: mean ± SEM: 29 ± 0.9 years; body weight: 79.3 ± 2.8 kg; BMI: 24.4 ± 0.6 kg/m2) and 12 with poor sleep quality (29 ± 1.2 years; 76.4 ± 2.2 kg; 24.3 ± 0.7 kg/m2). The demographics between the groups were similar except for PSQI which was significantly lower in good sleepers (3 ± 1) compared to poor sleepers (8 ± 2, p = 0.005) (Table 2). Main effects of sleeper type were identified for evening sleepiness (SSS) [F(2, 22) = 5.71 p = 0.026], getting to sleep (LSEQ) [F(2, 22) = 4.57 p = 0.044] and quality of sleep (LSEQ) [F(2, 22) = 7.91 p = 0.010]. Regardless of treatment, good sleepers rated themselves sleepier in evening (mean ± SEM across treatments; 4.4 ± 0.3) compared to poor sleepers (3.4 ± 0.3; post hoc p = 0.026). Good sleepers rated themselves as harder getting to sleep and had worse sleep quality (mean ± SEM across treatments; 47.8 ± 2.6, 46.6 ± 2.7 respectively) compared to poor sleepers (55.6 ± 2.6; post hoc p = 0.044, 57.2 ± 2.7; post hoc p = 0.010). All subjects completed and tolerated the study protocol. All participants had not been diagnosed with COVID-19.
3.2. Subjective measures of sleep and mood
3.2.1. Sleep measures
Table 3 shows a summary of subjective sleep measures. Analyzes determined interaction effects of treatment by sleeper type for ease of getting to sleep (LSEQ) [F(2, 22) = 4.81, p = 0.018] and ease of awakening following sleep (LSEQ) [F(2, 22) = 3.85, p = 0.037]. Within good sleepers, ratings of ease of getting to sleep tended to increase after the fresh KF treatment (54.1 ± 4.1) compared to the control (45.1 ± 3.4; post hoc p = 0.053; Figure 3A). Within poor sleepers, ratings of ease of waking were higher after the freeze-dried KF (62.8 ± 5.1; post hoc p = 0.005) and tended to be higher after the fresh KF treatment (51.9 ± 5.0, post hoc p = 0.052), compared to the control (38.6 ± 5.1, Figure 3B).
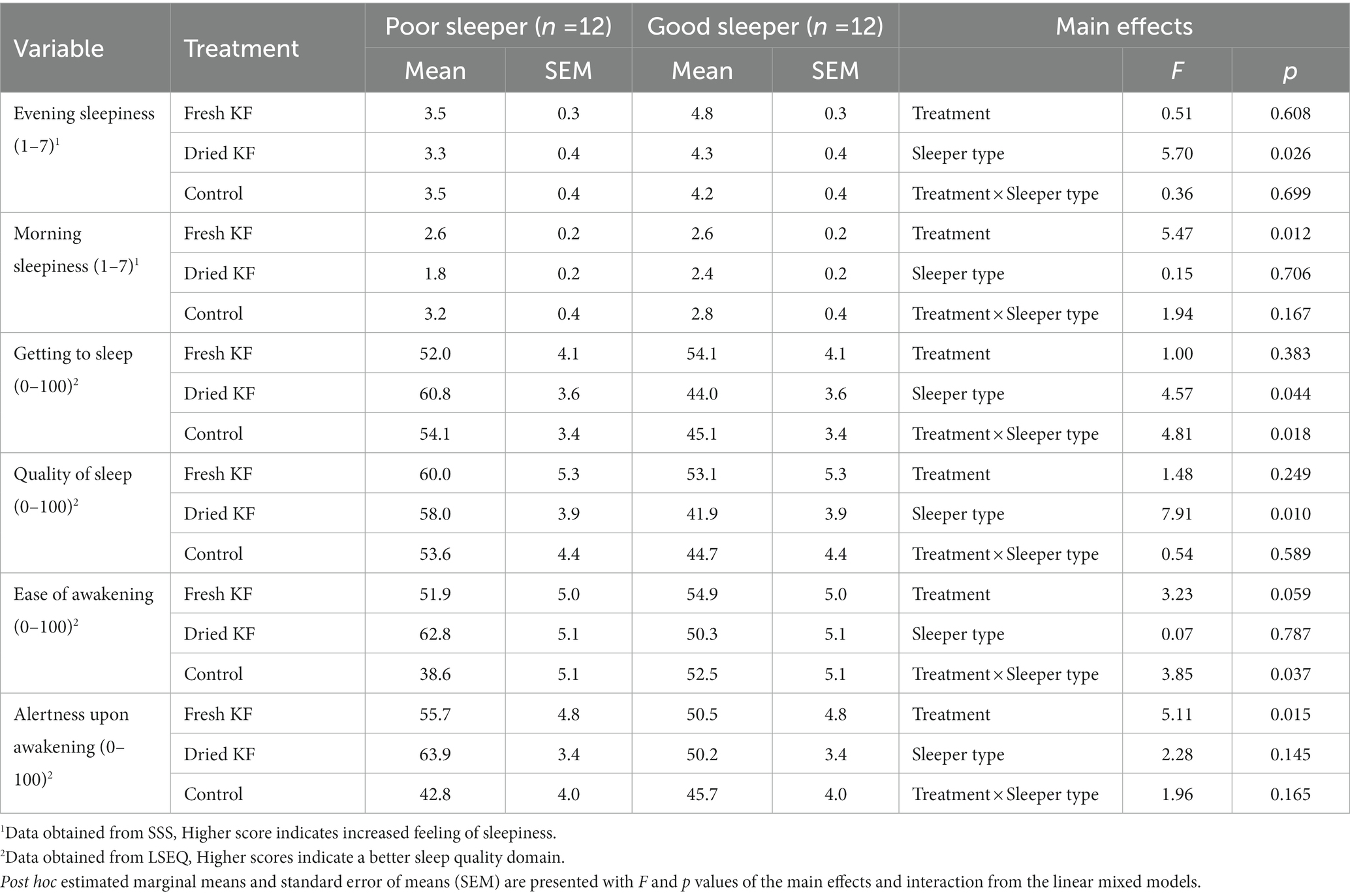
Table 3. Subjective sleep outcomes for the fresh KF, dried KF and water control treatments in poor and good sleepers.
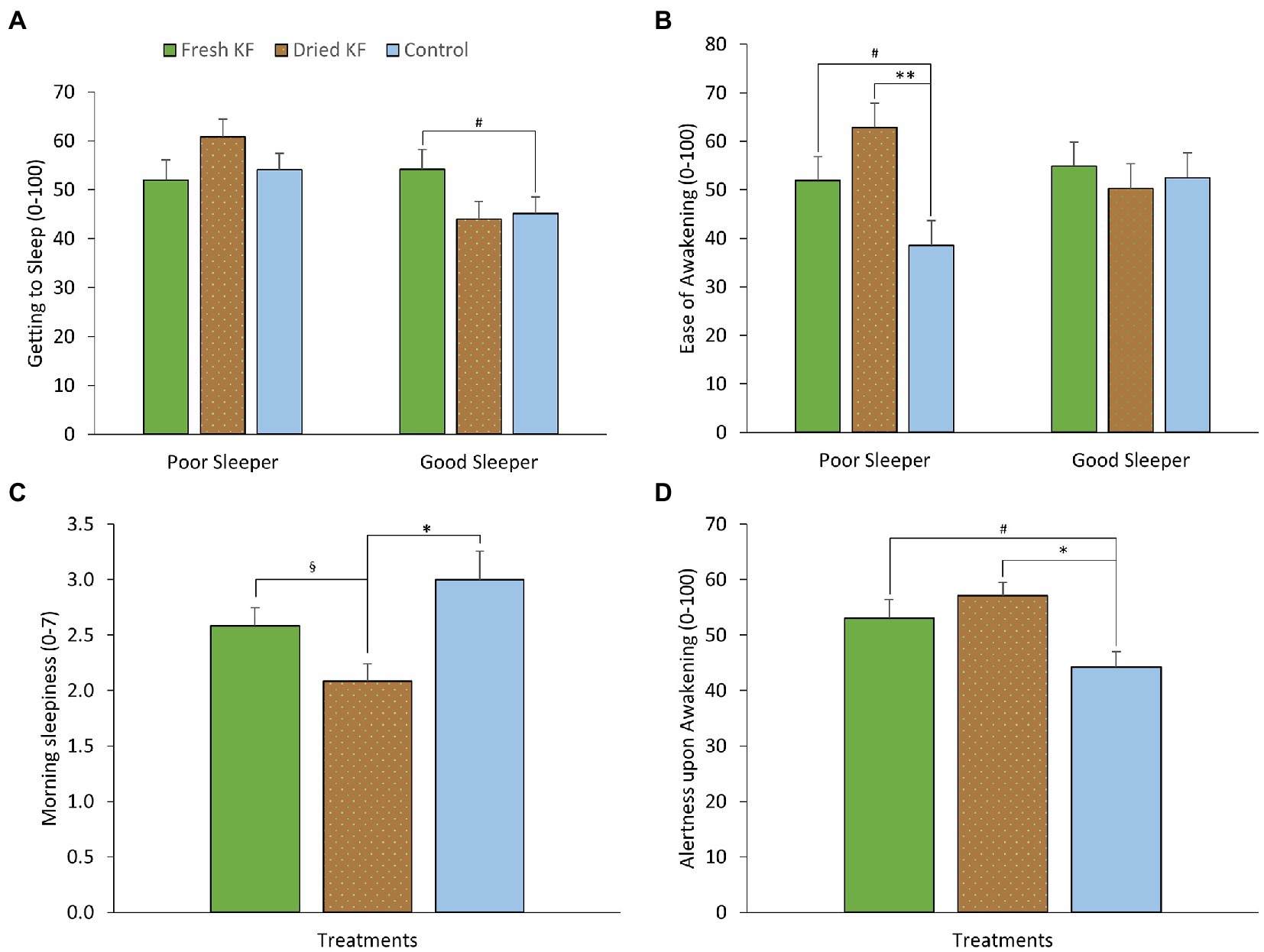
Figure 3. Estimated marginal means and standard error of means (SEM) for post-treatment subjective ratings of morning sleepiness (SSS) (A), getting to sleep (LSEQ) (B), ease of awakening (LSEQ) (C) and alertness upon awakening (LSEQ) (D). *p < 0.05 represents statistical difference from control values. §p < 0.05 represents the statistical difference from Dried KF values. **p < 0.01 represents statistical difference from control values. #p < 0.1 represents statistical difference from control values.
Main effects of treatment were identified for morning sleepiness (SSS) [F (2, 22) = 5.47, p = 0.012] and alertness upon awakening (LSEQ) [F(2, 22) = 5.11, p = 0.015]. Irrespective of sleeper type, morning sleepiness ratings were higher after the control (3.00 ± 0.26; post hoc p = 0.013) and fresh KF (2.6 ± 0.2; post hoc p = 0.034) interventions compared to the freeze-dried KF treatment (2.1 ± 0.2; Figure 3C). Ratings of alertness upon awakening were higher after the freeze-dried KF (57.1 ± 2.4; post hoc p = 0.018) and tended to be higher following the fresh KF treatment (53.1 ± 3.4; post hoc p = 0.082) compared to the control (44.2 ± 2.8, Figure 3D).
3.2.2. Mood measures
A summary of subjective mood measures is presented in Table 4. Main significant effects of treatment for esteem-related affect were identified [F(2, 22) = 4.56, p = 0.022], vigor [F(2, 22) = 4.34, p = 0.026], and total mood disturbance [F(2, 22) = 3.82, p = 0.038]. Furthermore, main trend effects of treatment for fatigue [F(2, 22) = 3.17, p = 0.062] and confusion [F(2, 22) = 3.37, p = 0.053] were identified. Irrespective of sleeper type, ratings for esteem-related affect tended to be higher following freeze-dried KF (12.6 ± 0.8; p = 0.060) and fresh KF (12.3 ± 0.6; post hoc p = 0.054) compared to the control (10.8 ± 0.5; Figure 4A). Ratings for vigor were higher following freeze-dried KF (6.58 ± 0.91; post hoc p = 0.030), but not fresh KF (4.9 ± 0.7; post hoc p = 0.487) treatments compared to the control (3.9 ± 0.6; Figure 4A). Ratings for total mood disturbance tended to be lower following the freeze-dried KF (86.9 ± 2.5; post hoc p = 0.054) and fresh KF (90.3 ± 1.8; post hoc p = 0.063) treatments compared to the control (96.4 ± 2.1, Figure 4B). No main effects for sleeper type or interactions were identified for any mood measures.
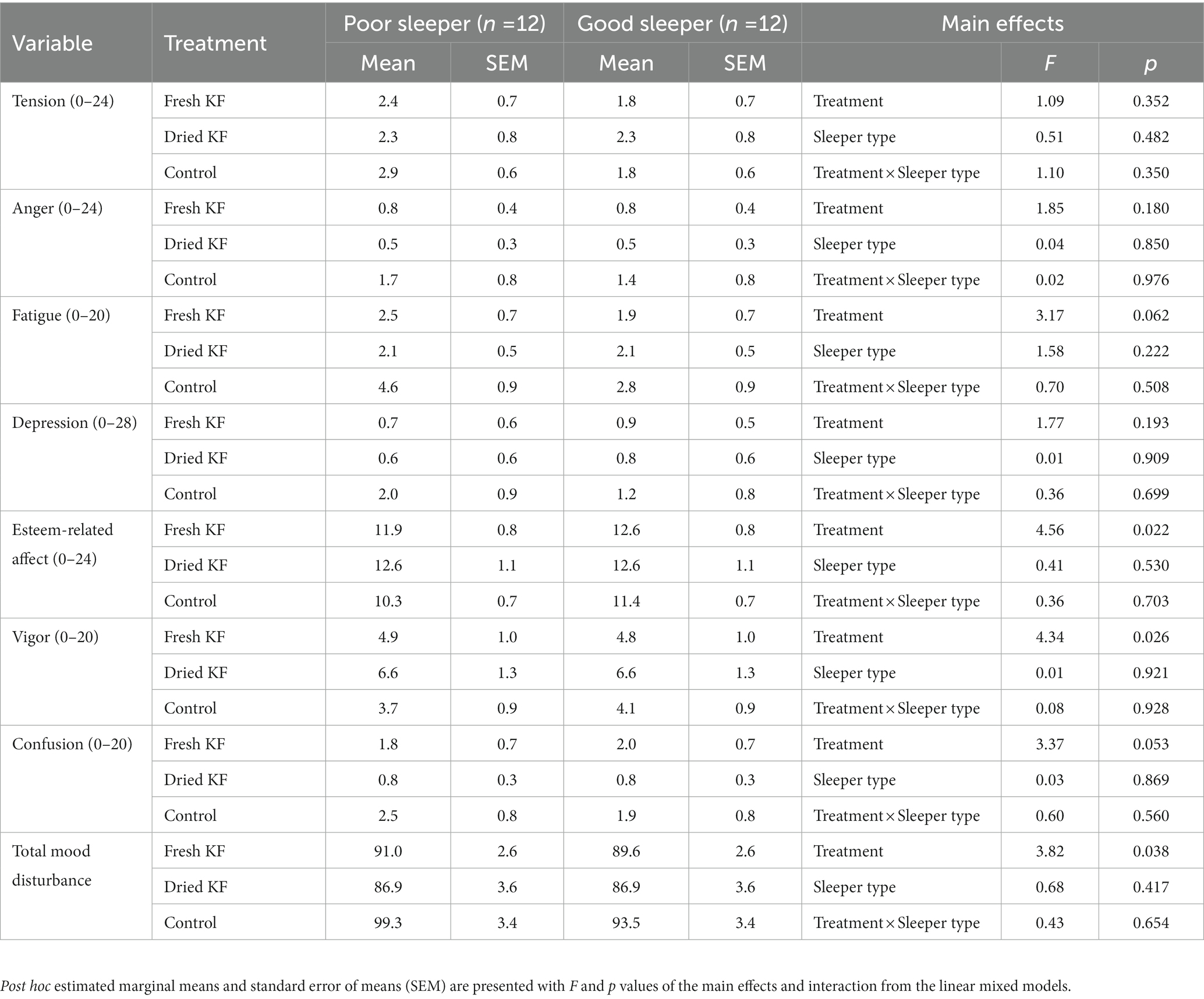
Table 4. Subjective mood outcomes for the fresh KF, dried KF and water control treatments in poor and good sleepers.
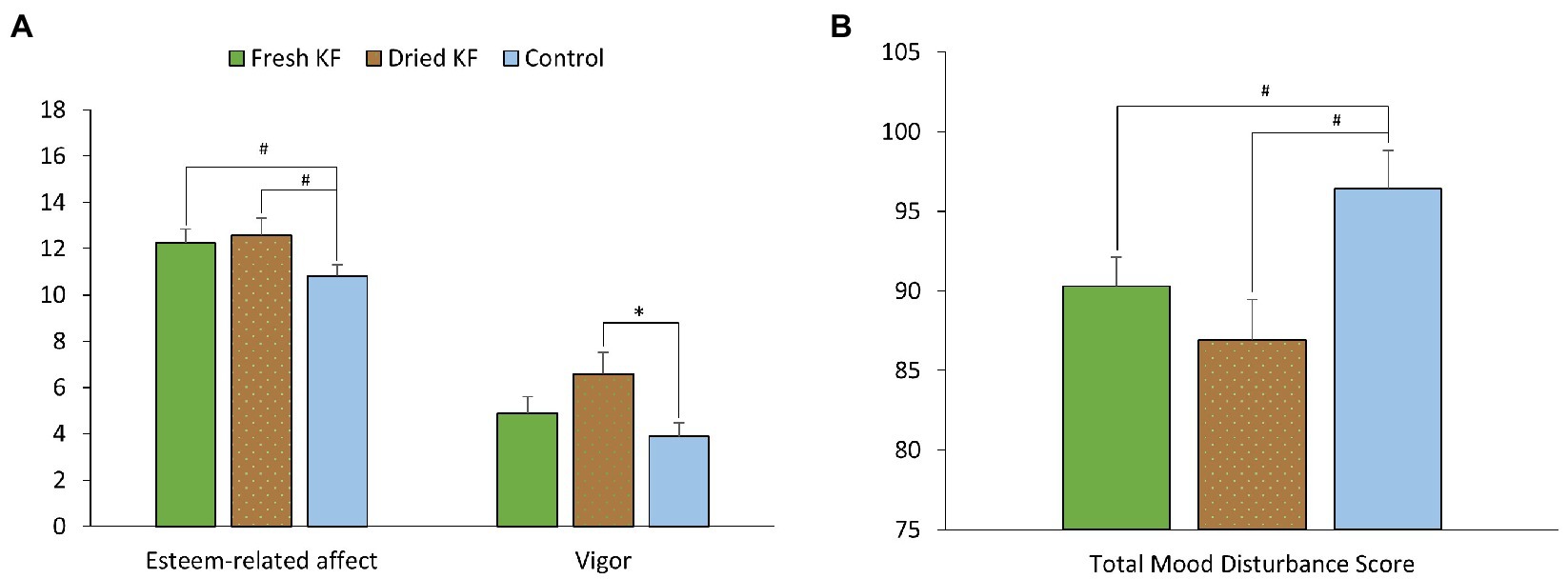
Figure 4. Estimated marginal means and standard error of means (SEM) for post-treatment subjective mood ratings (abbreviated Profile of Mood States) of esteem and vigor (A) and total mood disturbance (B). *p < 0.05 represents statistical difference from control values. #p < 0.1 represents statistical difference from control values.
3.3. Objective measures of sleep quality
A summary of objective sleep measures is presented in Table 5. There was an interaction effect between treatment and sleeper type for the number of awakenings [F(2, 22) = 4.06, p = 0.032]. Within poor sleepers, the number of awakenings tended to be lower after the fresh KF treatment (35.9 ± 2.76; p = 0.099) than the control (43.4 ± 3.5, Figure 5B). Conversely, among good sleepers, the number of awakenings tended to increase after the freeze-dried KF treatment (42.8 ± 3.81; post hoc p = 0.080) compared to the control (33.3 ± 3.54, Figure 5B).
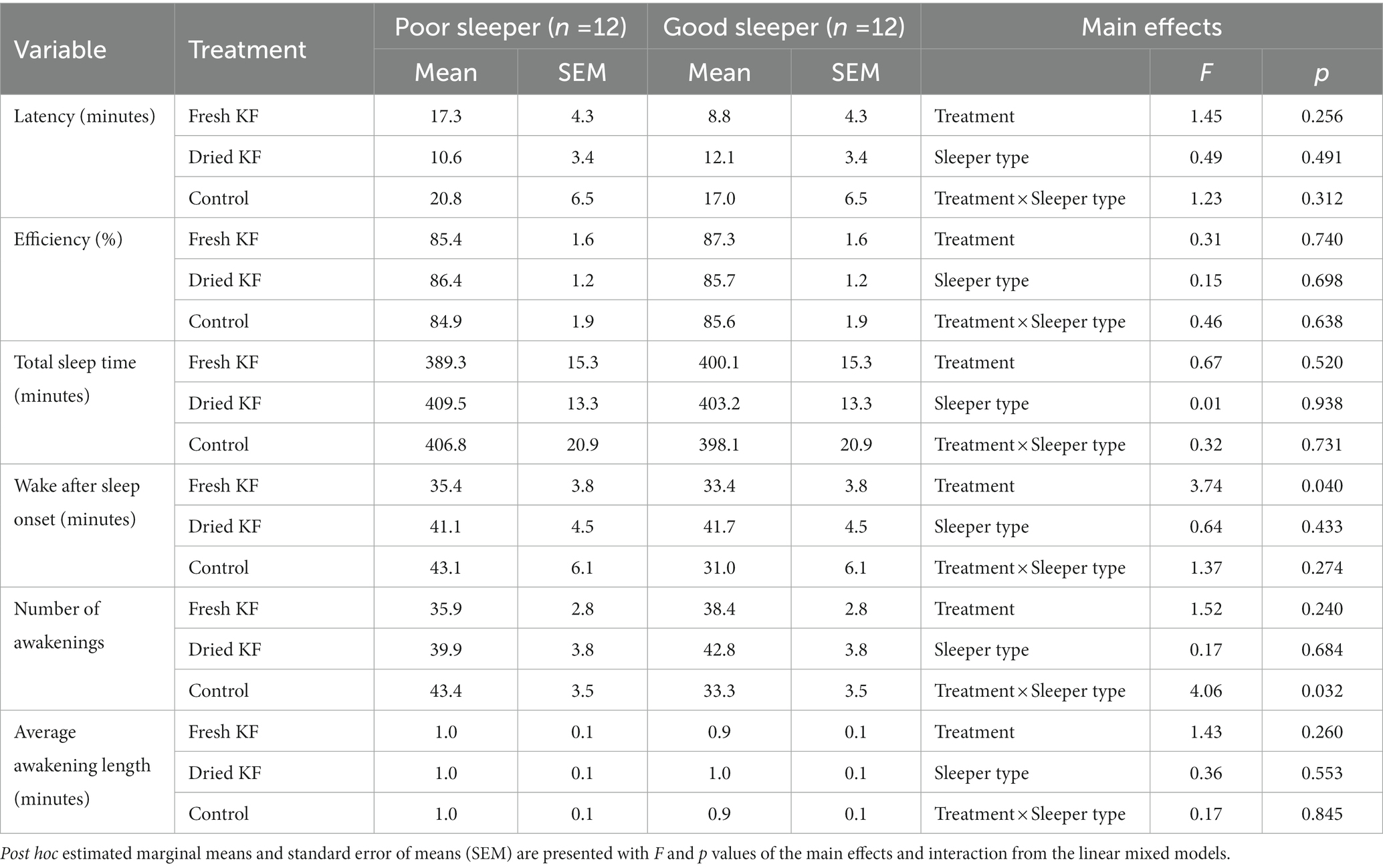
Table 5. Objective sleep outcomes for the fresh KF, dried KF and water control treatments in poor and good sleepers.
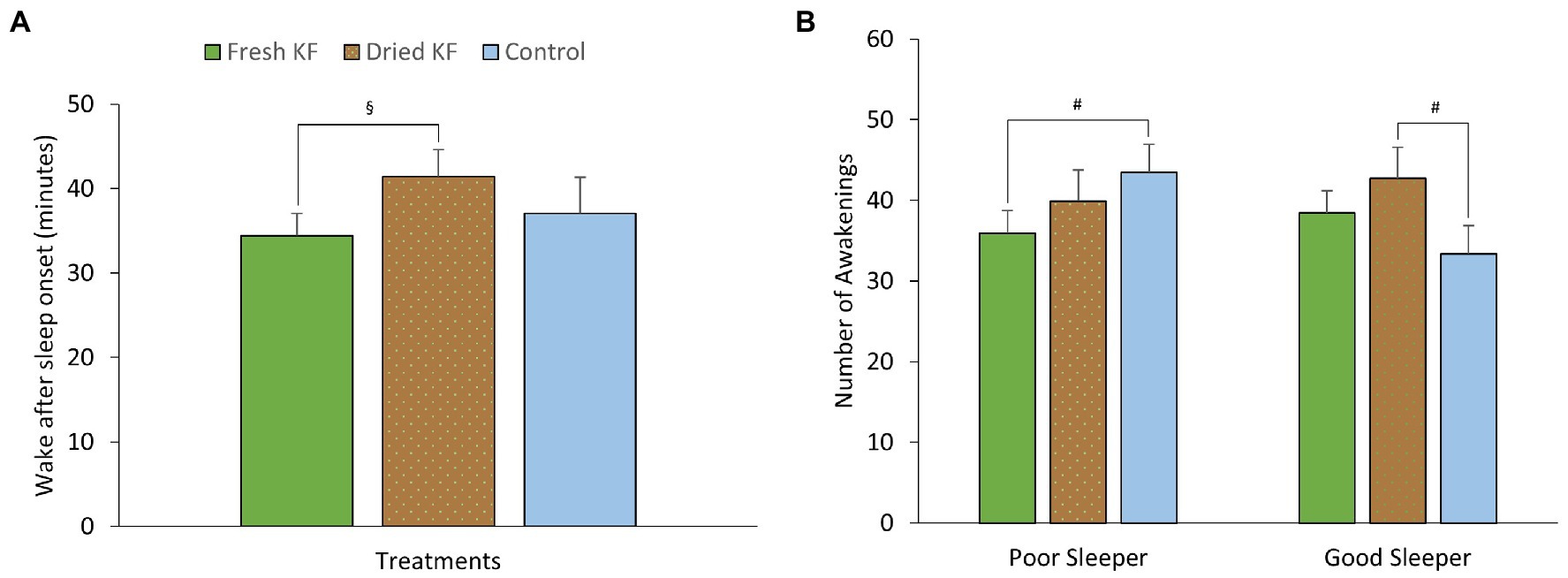
Figure 5. Estimated marginal means and standard error of means (SEM) for post-treatment objective sleep (actigraphy) measures of wake after sleep onset (A) and the number of awakening (B). §p < 0.05 represents the statistical difference from Dried KF values. #p < 0.1 represents statistical difference from control values.
There were main effects of treatment for wake after sleep onset [F(2, 22) = 3.74, p = 0.040]. Irrespective of sleeper type, the number of awakenings was higher after the freeze-dried KF treatment (41.4 ± 3.2; p = 0.045) but not the fresh KF treatment (34.4 ± 2.7; p = 0.888) compared to the control (37.0 ± 4.3) (Figure 5A). No main effects of treatment for sleeper type were identified for any objective sleep measures.
3.4. Urinary metabolites
A summary of urinary measures is in Table 6. A significant main effect of treatment for urinary 5-HIAA concentration was identified [F(2, 22) = 19.15, p < 0.001] with post hoc comparisons identifying freeze-dried KF (6.2 ± 0.4 mg/g creatinine; p < 0.001) and fresh KF (6.6 ± 0.4 mg/g creatinine; p = 0.001) treatments as showing significantly higher 5-HIAA concentration, as compared to the control (4.9 ± 0.4 mg/g creatinine) (Figure 6A). A significant main effect of sleeper type for urinary B-vitamin concentration was identified for nicotinamide [F(2, 22) = 6.11, p = 0.022], biotin [F(2, 22) = 5.77, p = 0.025], riboflavin [F(2, 22) = 6.85, p = 0.016], pyridoxamine [F(2, 22) = 7.71, p = 0.011], thiamine [F(2, 22) = 7.58, p = 0.012] and was near significant for pyridoxal [F(2, 22) = 4.28, p = 0.051]. The post hoc comparison revealed that good sleepers had significantly higher concentrations of all measured B-vitamins than poor sleepers (Figure 6B). No interaction effects were identified for any urinary metabolite measures. Additionally, the urinary concentration of 5-HIAA and vitamin C in good sleepers was negatively related to total mood disturbance (5-HIAA; r = −0.41, p = 0.04) and latency (vitamin C; r = −0.72, p < 0.005). A full within correlation table is presented in Supplementary Table S1.
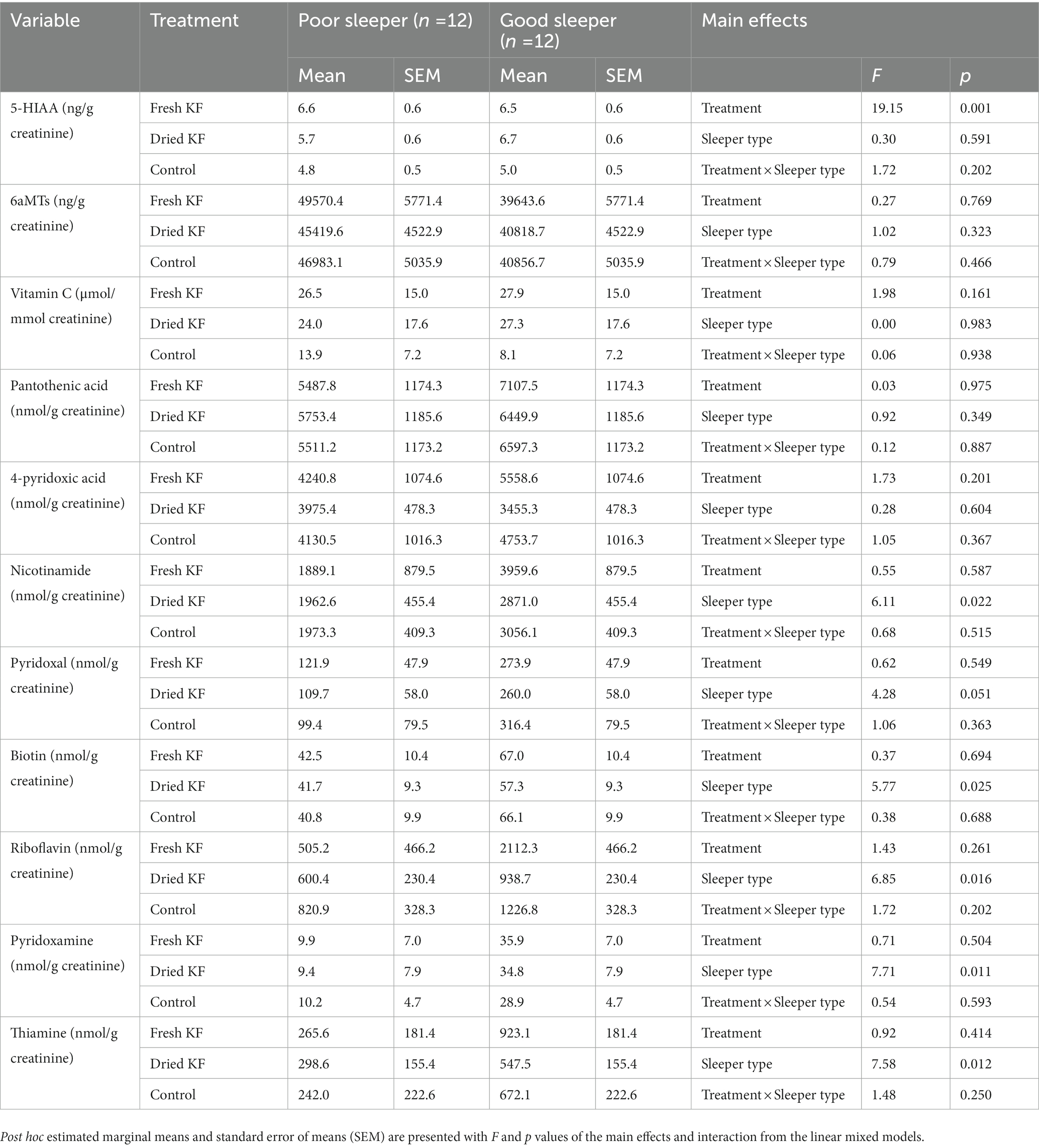
Table 6. Urinary 5-HIAA, aMT6s, vitamin C and B-vitamins for the fresh KF, dried KF and water control treatments in poor and good sleepers.
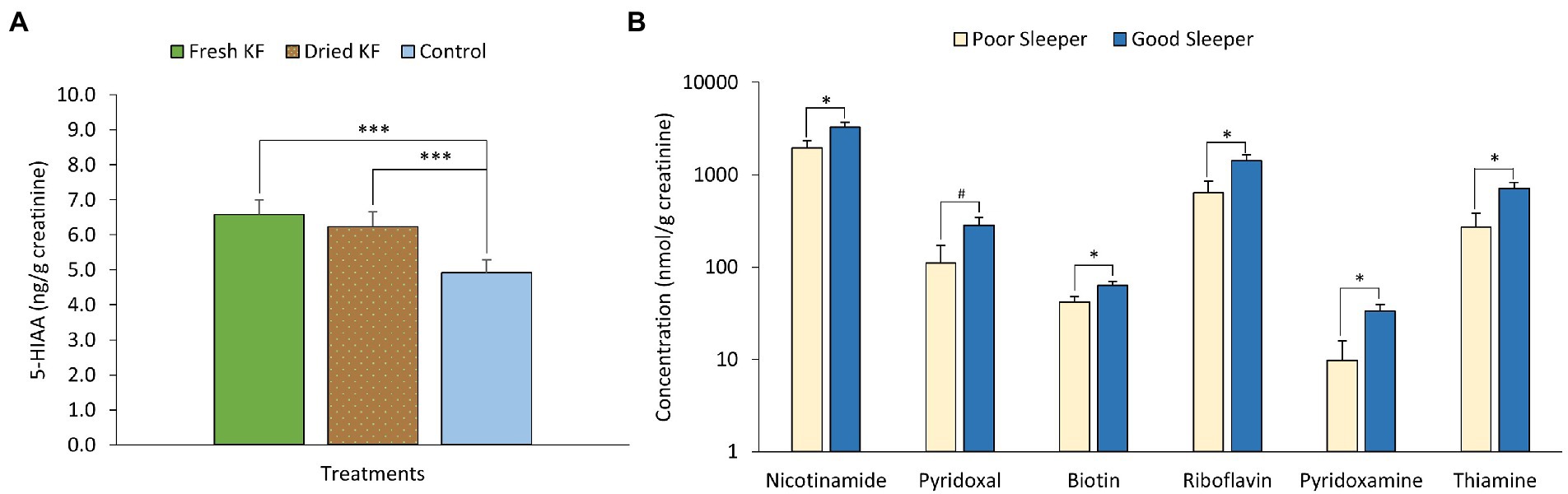
Figure 6. Estimated marginal means and standard error of means (SEM) for post-treatment urinary 5-HIAA (A) and urinary B-vitamins (B). #p < 0.1 represents statistical difference between sleeper type. *p < 0.05 represents the statistical difference between sleeper types. ***p < 0.005 represents statistical difference from control concentrations.
4. Discussion
The current study shows that irrespective of the sleep quality participant experience, acutely supplemented freeze-dried KF resulted in feeling less sleepy and more alert. Both fresh and freeze-dried KF increased urinary excretion of 5-HIAA. Furthermore, poor sleepers felt it was easier to wake up in the morning after consuming freeze-dried KF in the evening and this was also moderately felt when consuming the fresh KF. Good sleepers rated themselves as getting to sleep easier when consuming the fresh KF. Interestingly, poor sleepers tended to wake more often in the night after consuming freeze-dried KF with the evening meal, while good sleepers woke less often during the night after consuming fresh KF with the evening meal.
The beneficial impacts observed when consuming KF on aspects of sleep quality are consistent with other KF sleep intervention studies that showed improvement in subjective daytime function ratings (37) and sleep quality measures (21). In addition, in a mouse model fed KF skin extracts sleep onset latency was reduced (38), thus providing further evidence supporting the beneficial impact of KF on sleep. Sleep is a complex process controlled by internal and external factors. There are potentially at least three explanations that could explain the observed outcomes. Firstly, it is known that KF is rich in phenylalanine, tyrosine, tryptophan, glutamic acid (22) and serotonin (39) that are further metabolized to produce dopaminergic, serotoninergic, and GABAergic neurochemicals. These neurotransmitters are vital in the regulation of sleep/wake cycles. Secondly, green KF contains the enzyme actinidin, which has been shown to cause an earlier peak increase in plasma circulating amino acids in adults when compared to kiwifruit without actinidin (40). Therefore, potentially increasing the concentration of bioavailable amino acids to be further metabolized into neurochemicals. Lastly, KF may act on improving sleep via potentiating the bioaminergic responses. This has been demonstrated in in vitro studies (41). Given this, KF may impact sleep through the supply and modulation of peripheral neurochemical responses, which may impact systemic concentration modifications throughout the rest of the body. Further studies are needed to determine which postprandial biomarkers are increased in sleep and upon waking the following morning to elucidate how KF may impact sleep and subsequent mood.
The current study also shows that irrespective of sleep quality participant experience, acutely supplemented freeze-dried KF resulted in improved vigor compared to the control. Likewise, fresh, and freeze-dried KF treatments tended to improve esteem-related affects, and freeze-dried KF tended to decrease total mood disturbance score compared to the control. The impacts of consuming KF on aspects of waking mood in this study are consistent with earlier KF mood studies that showed improvement in mood (42, 43).
Similarly, the various underlying regulatory mechanisms of mood are complex. Firstly, vitamin C is a potent water-soluble antioxidant with distinct roles in the body, including reducing systemic inflammation and as co-factors in production of neurotransmitters. Consumption of KF reduces oxidative stress and inflammatory markers (44). Additionally, higher vitamin C status has been correlated with decreased total mood disturbances (45), and regular consumption of KF increases vitamin C in the body (46). Secondly, neurochemicals, such as serotonin, provided by KF, may also enhance mood (47). Lastly, KF is rich in other vitamins and polyphenols, which may have facilitated these mood improvements by improving oxidative stress and metabolism. Given this, KF may have impacted mood via the supply of vitamin C and other nutrients, which altered the metabolism of neurochemicals and may also have affected oxidative stress. One cannot rule out that improved sleep might have led to improved mood outcomes and vice versa (48).
No differences between KF treatments were observed for urinary aMT6s and vitamin C. The correlation analysis of urinary metabolites with sleep and mood measures indicated that urinary aMT6s and vitamin C morning excretion were negatively related to sleep latency. Waking urinary aMT6s can be used to predict nocturnal plasma melatonin (13) and lower urinary aMT6s excretion has been associated with lowered alertness (11). The results of aMT6s can be interpreted in two ways; first, the lack of treatment effects on excreted aMT6s may be due to insufficient samples collected or only one sample collected upon waking. Studies have shown that a 24–48-h collection period may better reflect these changes in urinary aMT6s (49). Dim light melatonin onset, a circadian phase marker measured over a 5–7 h window in plasma or saliva before bed, could be measured to assess whether KF may alter the circadian phase. Second, the finding may suggest that another mechanism not related to melatonin may facilitate the acute improvements in sleep quality and mood by KF. However, regardless of sleep quality, consumption of fresh and dried KF treatments increased urinary excretion of 5-HIAA compared to the control, which is consistent with data on the acute effects of KF consumption on urinary 5-HIAA (20). Comparably, in a different study, participants consuming tart cherries increased morning urinary 5-HIAA excretion and improved mood (19). 5-HIAA is the primary metabolite of serotonin and is involved with mood regulation (47). The results here suggested that consumption of fresh or freeze-dried KF may have increased circulating and brain levels of serotonin, which impacted mood.
Concentrations of excreted B-vitamins were greater for good sleepers than for poor sleepers, regardless of the treatment received. Excretion of B-Vitamins can be used as an indicator of baseline B-vitamin status, with higher excretion suggesting saturation and lowered amounts indicating deficiency (50). The lower amount of B-vitamins may be explained by a lower background dietary intake of B-vitamins, however this is speculation and should be interpreted with caution as no dietary records were collected. It may be suggested that poor sleepers utilize more B-vitamins for cellular metabolic processes. For example, nicotinamide (a form of B3), pyridoxine and pyridoxamine (forms of B6) are required as co-factors in metabolizing neurotransmitters (51). Additionally, low levels of B-vitamins have also been associated with low-grade inflammation (52) and poor sleep caused increased circulating inflammatory markers (53). However, this study did not measure any inflammatory markers, suggesting that inflammatory status is worth exploring in future studies. Other factors that were not assessed that may also impact B-vitamins include exercise status, which is known to increase the requirements for B-vitamins (54).
Irrespective of sleep quality participant experience, acutely supplemented freeze-dried KF improved morning sleepiness compared to both fresh KF treatment and control. This finding could be explained by the structural and compositional differences between KF interventions; a KF drink that contained the skin (liquid) or KF flesh eaten fresh without the skin (solid). Including skin in the freeze-dried powder altered the polyphenolic content, and as mentioned previously, KF skin extracts potentiate sleep induction in a pentobarbital-induced sleep mouse model (38). Thus, the freeze-dried powder may be improving sleep onset, thus causing better morning alertness. The results on sleep onset suggested that this may occur in poor sleepers. Furthermore, the added polyphenols may be impacting the body by affecting the expression of clock genes (55), improving cerebral blood flow (thus, improving mood (56)), altering the permeability of the blood–brain barrier and/or altering neurotransmissions (57).
Nevertheless, it is worth noting that the food structure may influence stomach emptying rate and satiety and cause differential interactions with other food matrices, consequently impacting gut comfort. For example, consumption of a carbohydrate-rich meal with KF lowered postprandial hunger compared to consumption of a carbohydrate meal only (58). Also, consuming fresh green actinidin-containing KF has improved gastric comfort compared to KF without actinidin (59). These findings suggest that improvements in sleep quality may be due to reduced feelings of bloating and hunger; however, these parameters were not measured in this study.
Contrary to the previous KF and sleep quality studies, this study was the first to assess the acute and separate effects of a fresh KF or dried (containing skin) KF treatment on sleep quality and mood in a healthy male cohort with good or poor-quality sleep. The urinary 5-HIAA and aMT6s concentrations suggest a novel potential mechanism underpinning the relationship between KF and sleep. Additionally, the results provide evidence for the repurposing of KF skin and lower-quality fruit, which would otherwise be wasted, into products that can easily be stored with extended shelf-life.
This study is not without limitations. One of the limitations was the low subject numbers, the study was powered using a different intervention to this (34), as this was the first study to assess acute impact of KF on LSEQ. Secondly, due to the nature of the intervention, it was impossible to blind participants to their treatment. This is evident in the ratings for getting to sleep and quality of sleep in good sleepers. Fresh KF was on average higher than both the control and freeze-dried KF, suggesting that some participants may have preconceived notions about one treatment over another. Thirdly, because the study was conducted only on men, it is difficult to interpret the results for women. Fourthly, the control used was not iso-calorically matched, suggesting participants may have rated themselves poorly on the control intervention due to other factors such as appetite and hunger, which were not measured. Furthermore, urine samples were limited to morning samples, with no other timepoints collected. Collecting samples other than urine at different time points may have allowed a better understanding of the postprandial changes that have occurred. Collection of plasma and saliva pre and post intervention and up to 5 h before bedtime may have provided a better understanding of melatonin circulation, which may have influenced sleep (49). In addition, saliva could have been collected upon waking and used to quantify cortisol awakening response (CAR), which could provide a simple measure of the reactive capacity of the hypothalamic–pituitary–adrenal (HPA) axis.
Although actigraphy was used here and in other acute settings (60), results should be interpreted with caution. For instance, a person lying still while awake may be recorded as asleep due to immobility. Actigraphy does not account for these events. Using polysomnography was not feasible in this study due to the in-home setting, but would have provided a better understanding of the actual acute impacts of KF and sleep quality and architecture (61). Future studies could incorporate a larger cohort with participants of mixed cohort of males and females consuming differing doses of the freeze-dried KF. Furthermore, future studies could use an energy matched placebo with the same amounts of vitamin C and B-vitamins. Additionally, other cohorts worth exploring include older adults due to age-related sleep disturbances (62), University students under high stress (63), or inpatients in planned care services (64).
5. Conclusion
Overall, this study is the first to demonstrate that a single evening meal with KF, whether fresh or freeze-dried, improved sleep quality and mood in males with good or poor-quality sleep. This effect may have been mediated through increased serotonin metabolism. Further studies should be conducted to elucidate the impact of freeze-dried KF on those who experience poor sleep and the potential mechanisms by measuring other biomarkers in urine, plasma, and saliva. Nonetheless, as beneficial effects of sleep were identified following supplementation with KF in young men, these data help provide additional evidence for the role of KF in facilitating healthy sleep regulation.
Data availability statement
The original contributions presented in the study are included in the article/Supplementary material, further inquiries can be directed to the corresponding author.
Ethics statement
The study protocol was approved by the Massey University Human Ethics Committee study (Massey University HEC: Southern A application-20/52), and the trial has been registered at the Australian New Zealand Clinical Trials Registry (ANZCTR) (ANZCTR12620000411943). Participants provided written, informed consent for participation.
Author contributions
AK, CG, NR, WM, and SH conceptualized the study and contributed to editing the manuscript. AK designed and conducted the study and prepared the manuscript with input from the other authors. All authors contributed to the article and approved the submitted version.
Funding
This study was funded by the Riddet Institute, a Centre of Research Excellence (CoRE) funded by the NZ Tertiary Education Commission, and the High-Value Nutrition National Science Challenge (UOAX1902) with in-kind support from the partner organization: Zespri International and Alpha Group Holdings. The funders had no role in the design of this study and did not have any role during its execution, analyses, interpretation of the data, or decision to submit results. AK was supported by a PhD stipend from the Riddet Institute.
Acknowledgments
The authors would like to thank the participants that took part in this trial. The authors would like to thank Zespri for the provision of fresh KF. The authors also acknowledge the support of the Riddet Institute and Massey University staff in conducting the clinical study.
Conflict of interest
The authors declare that the research was conducted in the absence of any commercial or financial relationships that could be construed as a potential conflict of interest.
Publisher’s note
All claims expressed in this article are solely those of the authors and do not necessarily represent those of their affiliated organizations, or those of the publisher, the editors and the reviewers. Any product that may be evaluated in this article, or claim that may be made by its manufacturer, is not guaranteed or endorsed by the publisher.
Supplementary material
The Supplementary material for this article can be found online at: https://www.frontiersin.org/articles/10.3389/fnut.2023.1079609/full#supplementary-material
Abbreviations
KF, kiwifruit; 5-HIAA, 5-hydroxyindoleacetic acid; aMT6s, 6-sulfatoxymelatonin; LSEQ, Leeds Sleep Evaluation Questionnaire; SSS, Stanford Sleepiness Scale; BMI, Body Mass Index; PSQI, Pittsburgh Sleep Quality Index; POMS, Profile of Mood States; VAS, Visual Analogue Scales; TMD, Total Mood Score; HPLC, high-performance liquid chromatography; CAR, cortisol awakening response; HPA, hypothalamic–pituitary–adrenal.
Footnotes
1. ^www.anzctr.org.au, ACTRN12621000046808.
References
1. Meier-Ewert, HK, Ridker, PM, Rifai, N, Regan, MM, Price, NJ, Dinges, DF, et al. Effect of sleep loss on C-reactive protein, an inflammatory marker of cardiovascular risk. J Am Coll Cardiol. (2004) 43:678–83. doi: 10.1016/j.jacc.2003.07.050
2. Fang, J, Wheaton, AG, Keenan, NL, Greenlund, KJ, Perry, GS, and Croft, JB. Association of sleep duration and hypertension among US adults varies by age and sex. Am J Hypertens. (2012) 25:335–41. doi: 10.1038/ajh.2011.201
3. Gottlieb, DJ, Redline, S, Nieto, FJ, Baldwin, CM, Newman, AB, Resnick, HE, et al. Association of usual sleep duration with hypertension: the sleep heart health study. Sleep. (2006) 29:1009–14. doi: 10.1093/sleep/29.8.1009
4. Gangwisch, JE, Heymsfield, SB, Boden-Albala, B, Buijs, RM, Kreier, F, Pickering, TG, et al. Sleep duration as a risk factor for diabetes incidence in a large U.S. sample. Sleep. (2007) 30:1667–73. doi: 10.1093/sleep/30.12.1667
5. Cappuccio, FP, Taggart, FM, Kandala, NB, Currie, A, Peile, E, Stranges, S, et al. Meta-analysis of short sleep duration and obesity in children and adults. Sleep. (2008) 31:619–26. doi: 10.1093/sleep/31.5.619
6. Anderson, JR, Carroll, I, Azcarate-Peril, MA, Rochette, AD, Heinberg, LJ, Peat, C, et al. A preliminary examination of gut microbiota, sleep, and cognitive flexibility in healthy older adults. Sleep Med. (2017) 38:104–7. doi: 10.1016/j.sleep.2017.07.018
7. Chellappa, SL, Morris, CJ, and Scheer, F. Daily circadian misalignment impairs human cognitive performance task-dependently. Sci Rep. (2018) 8:3041. doi: 10.1038/s41598-018-20707-4
8. Eastridge, BJ, Hamilton, EC, O’Keefe, GE, Rege, RV, Valentine, RJ, Jones, DJ, et al. Effect of sleep deprivation on the performance of simulated laparoscopic surgical skill. Am J Surg. (2003) 186:169–74. doi: 10.1016/S0002-9610(03)00183-1
9. Babson, KA, Trainor, CD, Feldner, MT, and Blumenthal, H. A test of the effects of acute sleep deprivation on general and specific self-reported anxiety and depressive symptoms: an experimental extension. J Behav Ther Exp Psychiatry. (2010) 41:297–303. doi: 10.1016/j.jbtep.2010.02.008
10. Nelson, KL, Davis, JE, and Corbett, CF. Sleep quality: an evolutionary concept analysis. Nurs Forum. (2022) 57:144–51. doi: 10.1111/nuf.12659
11. Saksvik-Lehouillier, I, Harrison, SL, Marshall, LM, Tranah, GJ, Ensrud, K, Ancoli-Israel, S, et al. Association of Urinary 6-Sulfatoxymelatonin (aMT6s) levels and objective and subjective sleep measures in older men: the MrOS sleep study. J Gerontol A Biol Sci Med Sci. (2015) 70:1569–77. doi: 10.1093/gerona/glv088
12. Chojnacki, C, Poplawski, T, Chojnacki, J, Fila, M, Konrad, P, and Blasiak, J. Tryptophan intake and metabolism in older adults with mood disorders. Nutrients. (2020) 12:3183. doi: 10.3390/nu12103183
13. Graham, C, Cook, MR, Kavet, R, Sastre, A, and Smith, DK. Prediction of nocturnal plasma melatonin from morning urinary measures. J Pineal Res. (1998) 24:230–8. doi: 10.1111/j.1600-079X.1998.tb00538.x
14. Oba, S, Nakamura, K, Sahashi, Y, Hattori, A, and Nagata, C. Consumption of vegetables alters morning urinary 6-sulfatoxymelatonin concentration. J Pineal Res. (2008) 45:17–23. doi: 10.1111/j.1600-079X.2007.00549.x
15. Yang, TH, Chen, YC, Ou, TH, and Chien, YW. Dietary supplement of tomato can accelerate urinary aMT6s level and improve sleep quality in obese postmenopausal women. Clin Nutr. (2019) 39:291–7. doi: 10.1016/j.clnu.2019.02.009
16. Garrido, M, Paredes, SD, Cubero, J, Lozano, M, Toribio-Delgado, AF, Munoz, JL, et al. Jerte Valley cherry-enriched diets improve nocturnal rest and increase 6-sulfatoxymelatonin and total antioxidant capacity in the urine of middle-aged and elderly humans. J Gerontol A Biol Sci Med Sci. (2010) 65:909–14. doi: 10.1093/gerona/glq099
17. Garrido, M, Gonzalez-Gomez, D, Lozano, M, Barriga, C, Paredes, SD, and Rodriguez, AB. A Jerte valley cherry product provides beneficial effects on sleep quality. Influence on aging. J Nutr Health Aging. (2013) 17:553–60. doi: 10.1007/s12603-013-0029-4
18. Briguglio, M, Dell'Osso, B, Panzica, G, Malgaroli, A, Banfi, G, Zanaboni Dina, C, et al. Dietary neurotransmitters: a narrative review on current knowledge. Nutrients. (2018) 10:591. doi: 10.3390/nu10050591
19. Garrido, M, Espino, J, Gonzalez-Gomez, D, Lozano, M, Barriga, C, Paredes, SD, et al. The consumption of a Jerte Valley cherry product in humans enhances mood, and increases 5-hydroxyindoleacetic acid but reduces cortisol levels in urine. Exp Gerontol. (2012) 47:573–80. doi: 10.1016/j.exger.2012.05.003
20. Feldman, JM, and Lee, EM. Serotonin content of foods: effect on urinary excretion of 5-hydroxyindoleacetic acid. Am J Clin Nutr. (1985) 42:639–43. doi: 10.1093/ajcn/42.4.639
21. Lin, HH, Tsai, PS, Fang, SC, and Liu, JF. Effect of kiwifruit consumption on sleep quality in adults with sleep problems. Asia Pac J Clin Nutr. (2011) 20:169–74.
22. Sivakumaran, S, Huffman, L, Sivakumaran, S, and Drummond, L. The nutritional composition of Zespri (R) sun gold kiwifruit and Zespri (R) sweet green kiwifruit. Food Chem. (2018) 238:195–202. doi: 10.1016/j.foodchem.2016.08.118
23. Buysse, DJ, Reynolds, CF, Monk, TH, Berman, SR, and Kupfer, DJ. The Pittsburgh sleep quality index: a new instrument for psychiatric practice and research. Psychiatry Res. (1989) 28:193–213. doi: 10.1016/0165-1781(89)90047-4
24. The Concise New Zealand Food Composition Tables [Internet]. Ministry of Health. (2018). Available from: http://www.foodcomposition.co.nz/concise-tables/
25. Meng, X, Li, Y, Li, S, Zhou, Y, Gan, RY, Xu, DP, et al. Dietary sources and bioactivities of melatonin. Nutrients. (2017) 9:367. doi: 10.3390/nu9040367
26. Johns, NP, Johns, J, Porasuphatana, S, Plaimee, P, and Sae-Teaw, M. Dietary intake of melatonin from tropical fruit altered urinary excretion of 6-sulfatoxymelatonin in healthy volunteers. J Agric Food Chem. (2013) 61:913–9. doi: 10.1021/jf300359a
27. Hoddes, E, Zarcone, V, Smythe, H, Phillips, R, and Dement, WC. Quantification of sleepiness: a new approach. Psychophysiology. (1973) 10:431–6. doi: 10.1111/j.1469-8986.1973.tb00801.x
28. Zisapel, N, and Laudon, M. Subjective assessment of the effects of CNS-active drugs on sleep by the Leeds sleep evaluation questionnaire: a review. Hum Psychopharmacol. (2003) 18:1–20. doi: 10.1002/hup.455
29. Grove, R, and Prapavessis, H. Preliminary evidence for the reliability and validity of an abbreviated profile of mood states. Int J Sport Psychol. (1992) 23:93–109.
30. Chae, KY, Kripke, DF, Poceta, JS, Shadan, F, Jamil, SM, Cronin, JW, et al. Evaluation of immobility time for sleep latency in actigraphy. Sleep Med. (2009) 10:621–5. doi: 10.1016/j.sleep.2008.07.009
31. Pullar, JM, Bayer, S, and Carr, AC. Appropriate handling, processing and analysis of blood samples is essential to avoid oxidation of vitamin C to dehydroascorbic acid. Antioxidants (Basel). (2018) 7:29. doi: 10.3390/antiox7020029
32. Sharma, P, Han, SM, Gillies, N, Thorstensen, EB, Goy, M, Barnett, MPG, et al. Circulatory and urinary B-vitamin responses to multivitamin supplement ingestion differ between older and younger adults. Nutrients. (2020) 12:3529. doi: 10.3390/nu12113529
33. Jaffe, M. Ueber den Niederschlag, welchen Pikrinsäure in normalem Harn erzeugt und über eine neue Reaction des Kreatinins. bchm. (1886) 10:391–400. doi: 10.1515/bchm1.1886.10.5.391
34. Hindmarch, I, Rigney, U, Stanley, N, Quinlan, P, Rycroft, J, and Lane, J. A naturalistic investigation of the effects of day-long consumption of tea, coffee and water on alertness, sleep onset and sleep quality. Psychopharmacology. (2000) 149:203–16. doi: 10.1007/s002130000383
35. Bland, JM, and Altman, DG. Calculating correlation coefficients with repeated observations: part 1--correlation within subjects. BMJ. (1995) 310:446. doi: 10.1136/bmj.310.6977.446
36. Khamis, H. Measures of association: how to choose? J Diagn Med Sonogr. (2008) 24:155–62. doi: 10.1177/8756479308317006
37. Nødtvedt, ØO, Hansen, AL, Bjorvatn, B, and Pallesen, S. The effects of kiwi fruit consumption in students with chronic insomnia symptoms: a randomized controlled trial. Sleep Biol Rhythms. (2017) 15:159–66. doi: 10.1007/s41105-017-0095-9
38. Yang, H, Lee, YC, Han, KS, Singh, H, Yoon, M, Park, JH, et al. Green and gold kiwifruit peel ethanol extracts potentiate pentobarbital-induced sleep in mice via a GABAergic mechanism. Food Chem. (2013) 136:160–3. doi: 10.1016/j.foodchem.2012.07.111
39. Herraiz, T, and Galisteo, J. Tetrahydro-beta-carboline alkaloids occur in fruits and fruit juices. Activity as antioxidants and radical scavengers. J Agric Food Chem. (2003) 51:7156–61. doi: 10.1021/jf030324h
40. Park, S, Church, DD, Starck, C, Schutzler, SE, Azhar, G, Kim, IY, et al. The impact of Hayward green kiwifruit on dietary protein digestion and protein metabolism. Eur J Nutr. (2021) 60:1141–8. doi: 10.1007/s00394-020-02363-5
41. Parkar, SG, Jobsis, CMH, Trower, TM, Cooney, JM, Hedderley, DI, and Bentley-Hewitt, KL. Bioaminergic responses in an in vitro system studying human gut microbiota–kiwifruit interactions. Microorganisms. (2020) 8:1582. doi: 10.3390/microorganisms8101582
42. Carr, AC, Bozonet, SM, Pullar, JM, and Vissers, MC. Mood improvement in young adult males following supplementation with gold kiwifruit, a high-vitamin C food. J Nutr Sci. (2013) 2:e24. doi: 10.1017/jns.2013.12
43. Conner, TS, Fletcher, BD, Pullar, JM, Spencer, E, Mainvil, LA, and Vissers, MCM. Kiwi C for vitality: results of a randomized placebo-controlled trial testing the effects of kiwifruit or vitamin C tablets on vitality in adults with low vitamin C levels. Nutrients. (2020) 12:2898. doi: 10.3390/nu12092898
44. Stonehouse, W, Gammon, CS, Beck, KL, Conlon, CA, von Hurst, PR, and Kruger, R. Kiwifruit: our daily prescription for health. Can J Physiol Pharmacol. (2013) 91:442–7. doi: 10.1139/cjpp-2012-0303
45. Pullar, JM, Carr, AC, Bozonet, SM, and Vissers, MCM. High vitamin C status is associated with elevated mood in male tertiary students. Antioxidants. (2018) 7:91. doi: 10.3390/antiox7070091
46. Bozonet, SM, Carr, AC, Pullar, JM, and Vissers, MC. Enhanced human neutrophil vitamin C status, chemotaxis and oxidant generation following dietary supplementation with vitamin C-rich sun gold kiwifruit. Nutrients. (2015) 7:2574–88. doi: 10.3390/nu7042574
47. Jenkins, TA, Nguyen, JC, Polglaze, KE, and Bertrand, PP. Influence of tryptophan and serotonin on mood and cognition with a possible role of the gut-brain Axis. Nutrients. (2016) 8:56. doi: 10.3390/nu8010056
48. Kahn, M, Sheppes, G, and Sadeh, A. Sleep and emotions: bidirectional links and underlying mechanisms. Int J Psychophysiol. (2013) 89:218–28. doi: 10.1016/j.ijpsycho.2013.05.010
49. Kennaway, DJ. A critical review of melatonin assays: past and present. J Pineal Res. (2019) 67:e12572. doi: 10.1111/jpi.12572
50. Schuster, K, Bailey, LB, Cerda, JJ, and Gregory, JF 3rd. Urinary 4-pyridoxic acid excretion in 24-hour versus random urine samples as a measurement of vitamin B6 status in humans. Am J Clin Nutr. (1984) 39:466–70. doi: 10.1093/ajcn/39.3.466
51. Kennedy, DO. B vitamins and the brain: mechanisms, dose and efficacy—a review. Nutrients. (2016) 8:68. doi: 10.3390/nu8020068
52. Shen, J, Lai, CQ, Mattei, J, Ordovas, JM, and Tucker, KL. Association of vitamin B-6 status with inflammation, oxidative stress, and chronic inflammatory conditions: the Boston Puerto Rican health study. Am J Clin Nutr. (2010) 91:337–42. doi: 10.3945/ajcn.2009.28571
53. Mullington, JM, Simpson, NS, Meier-Ewert, HK, and Haack, M. Sleep loss and inflammation. Best Pract Res Clin Endocrinol Metab. (2010) 24:775–84. doi: 10.1016/j.beem.2010.08.014
54. Manore, MM. Effect of physical activity on thiamine, riboflavin, and vitamin B-6 requirements. Am J Clin Nutr. (2000) 72:598S–606S. doi: 10.1093/ajcn/72.2.598S
55. Noorwali, E, Hardie, L, and Cade, J. Bridging the reciprocal gap between sleep and fruit and vegetable consumption: a review of the evidence, potential mechanisms, implications, and directions for future work. Nutrients. (2019) 11:1382. doi: 10.3390/nu11061382
56. Schaffer, S, and Halliwell, B. Do polyphenols enter the brain and does it matter? Some theoretical and practical considerations. Genes Nutr. (2012) 7:99–109. doi: 10.1007/s12263-011-0255-5
57. Vauzour, D. Dietary polyphenols as modulators of brain functions: biological actions and molecular mechanisms underpinning their beneficial effects. Oxidative Med Cell Longev. (2012) 2012:914273:1–16. doi: 10.1155/2012/914273
58. Mishra, S, Edwards, H, Hedderley, D, Podd, J, and Monro, J. Kiwifruit non-sugar components reduce glycaemic response to co-ingested cereal in humans. Nutrients. (2017) 9:1195. doi: 10.3390/nu9111195
59. Wallace, A, Eady, S, Drummond, L, Hedderley, D, Ansell, J, and Gearry, R. A pilot randomized cross-over trial to examine the effect of kiwifruit on satiety and measures of gastric comfort in healthy adult males. Nutrients. (2017) 9:639. doi: 10.3390/nu9070639
60. Ancoli-Israel, S, Cole, R, Alessi, C, Chambers, M, Moorcroft, W, and Pollak, CP. The role of actigraphy in the study of sleep and circadian rhythms. Sleep. (2003) 26:342–92. doi: 10.1093/sleep/26.3.342
61. Van de Water ATHolmes, A, and Hurley, DA. Objective measurements of sleep for non-laboratory settings as alternatives to polysomnography--a systematic review. J Sleep Res. (2011) 20:183–200. doi: 10.1111/j.1365-2869.2009.00814.x
62. Hughes, RJ, Sack, RL, and Lewy, AJ. The role of melatonin and circadian phase in age-related sleep-maintenance insomnia: assessment in a clinical trial of melatonin replacement. Sleep. (1998) 21:52–68.
63. Almojali, AI, Almalki, SA, Alothman, AS, Masuadi, EM, and Alaqeel, MK. The prevalence and association of stress with sleep quality among medical students. J Epidemiol Glob Health. (2017) 7:169–74. doi: 10.1016/j.jegh.2017.04.005
Keywords: sleep, mood, kiwifruit, melatonin (6-sulfatoxymelatonin), serotonin, alertness
Citation: Kanon AP, Giezenaar C, Roy NC, McNabb WC and Henare SJ (2023) Acute effects of fresh versus dried Hayward green kiwifruit on sleep quality, mood, and sleep-related urinary metabolites in healthy young men with good and poor sleep quality. Front. Nutr. 10:1079609. doi: 10.3389/fnut.2023.1079609
Edited by:
Andrew Scholey, Monash University, AustraliaReviewed by:
Reza Rastmanesh, The Nutrition Society, United KingdomMarie-Pierre St-Onge, Columbia University, United States
Copyright © 2023 Kanon, Giezenaar, Roy, McNabb and Henare. This is an open-access article distributed under the terms of the Creative Commons Attribution License (CC BY). The use, distribution or reproduction in other forums is permitted, provided the original author(s) and the copyright owner(s) are credited and that the original publication in this journal is cited, in accordance with accepted academic practice. No use, distribution or reproduction is permitted which does not comply with these terms.
*Correspondence: Sharon J. Henare, Uy5KLkhlbmFyZUBtYXNzZXkuYWMubno=