Phenome-wide Mendelian randomization study evaluating the association of circulating vitamin D with complex diseases
- 1Guangdong Provincial Key Laboratory of Food, Nutrition and Health, Sun Yat-sen University (North Campus), Guangzhou, Guangdong, China
- 2Department of Epidemiology, School of Public Health, Sun Yat-sen University (North Campus), Guangzhou, Guangdong, China
- 3Department of Hepatobiliary Surgery, Jingdezhen No.1 People's Hospital, Jingdezhen, Jiangxi, China
- 4Department of Cardiology, The First Affiliated Hospital of Jiangxi Medical College, Shangrao, Jiangxi, China
- 5Department of Clinical Medicine, Jiangxi Medical College, Shangrao, Jiangxi, China
Background: Circulating vitamin D has been associated with multiple clinical diseases in observational studies, but the association was inconsistent due to the presence of confounders. We conducted a bidirectional Mendelian randomization (MR) study to explore the healthy atlas of vitamin D in many clinical traits and evaluate their causal association.
Methods: Based on a large-scale genome-wide association study (GWAS), the single nucleotide polymorphism (SNPs) instruments of circulating 25-hydroxyvitamin D (25OHD) from 443,734 Europeans and the corresponding effects of 10 clinical diseases and 42 clinical traits in the European population were recruited to conduct a bidirectional two-sample Mendelian randomization study. Under the network of Mendelian randomization analysis, inverse-variance weighting (IVW), weighted median, weighted mode, and Mendelian randomization (MR)–Egger regression were performed to explore the causal effects and pleiotropy. Mendelian randomization pleiotropy RESidual Sum and Outlier (MR-PRESSO) was conducted to uncover and exclude pleiotropic SNPs.
Results: The results revealed that genetically decreased vitamin D was inversely related to the estimated BMD (β = −0.029 g/cm2, p = 0.027), TC (β = −0.269 mmol/L, p = 0.006), TG (β = −0.208 mmol/L, p = 0.002), and pulse pressure (β = −0.241 mmHg, p = 0.043), while positively associated with lymphocyte count (β = 0.037%, p = 0.015). The results did not reveal any causal association of vitamin D with clinical diseases. On the contrary, genetically protected CKD was significantly associated with increased vitamin D (β = 0.056, p = 2.361 × 10−26).
Conclusion: The putative causal effects of circulating vitamin D on estimated bone mass, plasma triglyceride, and total cholesterol were uncovered, but not on clinical diseases. Vitamin D may be linked to clinical disease by affecting health-related metabolic markers.
Introduction
Vitamin D is an essential fat-soluble nutrition from cholecalciferol and steroid pro-hormone, which is predominately obtained from sunlight, dietary sources, and supplementation (1, 2). Vitamin D deficiency is common worldwide, with nearly one billion people experiencing vitamin D deficiency in 2019 (3). In this study, it is reported that 69.2% of the Asian population suffer from vitamin D deficiency, with 61% of postmenopausal women deficient in vitamin D , and the prevalence of vitamin D insufficiency among children is 56.2% (4). The deficiency in vitamin D is critical and is a contributor to the risk of metabolic diseases (5–7), cancers (8, 9), and all-cause mortality (10, 11).
Lowering 25-hydroxyvitamin D (25(OH)D) may act as a trigger for the inflammatory response to disturb the composition and function of circulating cytokines and growth factors (12), influencing endothelial cells (13), hence, increasing the risk of neurodegenerative disorders (14), cardiovascular disease (15, 16), musculoskeletal lesions (17), and mortality (11). The large-scale meta-analysis included 50 randomized controlled trials (RCTs) with a total of 74,655 participants and revealed that vitamin D supplementation statistically significantly reduced the risk of cancer death (RR: 0.85, 0.74–0.97) in adults compared with placebo (18). Moreover, vitamin D supplementation significantly reduced total cancer mortality in an updated meta-analysis of RCTs with 1,591 deaths (19). The meta-analysis comparing the highest with the lowest circulating 25(OH)D concentrations showed a 39% lower risk between levels of total 25(OH)D and colorectal cancer (CRC) risk (OR: 0.61, 0.52–0.71) with 11 case–control studies, and a 20% reduced CRC risk (HR: 0.80, 0.66–0.97) with six prospective cohort studies (20). Moreover, the effects of vitamin D supplementation on decreasing the LDL-c level (21) and releasing insulin resistance (22) were uncovered.
Inversely, the results from an RCT of vitamin D3 (cholecalciferol) at a dose of 2,000 IU per day during a median follow-up of 5.3 years found that supplementation with vitamin D3 did not result in a lower incidence of invasive cancer or cardiovascular events than placebo (23). The negative results were observed in another RCT with 5,108 older adult participants (65.9 years) during a median follow-up of 3.3 years, in which monthly high-dose vitamin D3 (200,000 IU) supplementation did not prevent CVD (24). Meanwhile, the studies did not illuminate the benefits of vitamin D3 supplementation for relapse-free survival of digestive tract cancer at 5 years (25, 26). Although numerous studies explored the association of vitamin D with many clinical diseases, inconsistent results were produced, and the potential confounders may contribute to the unobvious benefit of vitamin D.
Recently, single nucleotide polymorphism (SNP) that was identified by genome-wide association studies (GWASs) was recruited as an instrument in Mendelian randomization (MR) analyses to explore the causal effects of exposures on outcomes (6, 27–30). The MR analysis demonstrated that genetically decreased 25(OH)D was associated with the risk of multiple sclerosis (MS) and provided strong evidence for the causal role of vitamin D in MS susceptibility (8). Moreover, a significant association between 25(OH)D levels and T2DM was found in a European-descent MR with SNP instruments of vitamin D synthesis (31). Inversely, no evidence for the effects of 25(OH)D on T2DM was observed in another MR study with four instrumental SNPs of 25(OH)D concentrations (27). Meanwhile, the delicate associations between genetically predicted 25(OH)D and multiple outcomes were observed, such as in inflammatory bowel disease (IBD) (28), bone mineral density (BMD) (32), non-alcoholic fatty liver disease (NAFLD) (29), and hypertension (33). The genetic instruments of 25(OH)D recruited in a previous MR analysis were limited to four or six variants involved in vitamin D synthesis (DHCR7/NADSYN1, rs12785878 and CYP2R1, rs10741657), transportation (GC, rs3755967), and degradation (CYP24A1, rs17216707), as well as two novel vitamin D metabolism pathways, such as SEC23A (Sec23 homolog A, coat protein complex II component, rs8018720) and AMDHD1 (amidohydrolase domain containing 1, rs10745742). The overall estimate of heritability of 25(OH)D attributable to statistically significant GWAS common SNPs was 2.84% (34). The limited efficiency of SNP instruments may lead to inconsistent results.
In the current study, we conducted a systematic literature review of previous MR studies on 25(OH)D and performed a bidirectional MR analysis to examine the causal association between genetic determinants of 25(OH)D and cardiovascular diseases (CAD), fracture, rheumatoid arthritis (RA), Alzheimer's dementia (AD), inflammatory bowel disease (IBD), chronic kidney disease (CKD), and related clinical risk factors using summary data from large-scale genome-wide association studies.
Methods
Study design
The GWAS summary statistics about 10 clinical diseases (including chronic kidney disease, stroke, type-2 diabetes, coronary artery disease, CAD with diabetes, Crohn's disease, ulcerative colitis, fracture, rheumatoid arthritis, and Alzheimer's dementia) and 42 clinical traits (including the anthropometric index, glycemic traits, serum lipids, cardiovascular measurements, kidney function, musculoskeletal health, bone mineral density, and blood cell and plasma cytokine) were collected from publicly available data sources and analyzed to estimate the genetic causal relationship of serum 25(OH)D with multifarious clinical outcomes through bidirectional two-sample Mendelian randomization (MR) (35). The MR procedure consists of three assumptions (36): (1) the genetic instrumental variables are strongly associated with exposures; (2) the genetic instrumental variables are not associated with any known or unmeasured confounders: influencing the association between genetic variants and outcomes; (3) the genetic variants are associated with outcomes only through exposures: variants causing significant effects on outcomes not through other pathways, no horizontal pleiotropy. The framework of the MR study is presented in Supplementary Figure 1. Ethics approval was not required for this study.
Identification of SNPs associated with 25(OH)D and clinical outcomes
Based on the large-scale GWAS, which consisted of 443,734 participants from the UK Biobank, the 138 conditionally independent SNPs for 25(OH)D, mapped to 69 distinct loci, among which 63 were previously not reported, were selected at the genome-wide significance level (6.6 × 10−9) after adjusting for age, sex, and season of 25(OH)D measurement (37). Of these conditionally independent SNPs, 53 (38%) had a minor allele frequency (MAF) of <5%, and 85 (62%) were common (MAF ≥ 5%). The 53 SNPs with a MAF of <5% conferred an average absolute effect of 0.23 standard deviations on standardized log transformed 25(OH)D levels per effect allele, compared to 0.03 standard deviations of the 85 SNPs with a MAF of ≥5%. The average absolute effect on 25(OH)D of the 53 low frequency and rare variants was at least 7 times larger than the average effect of the 85 common SNPs. The known vitamin D loci (CYP2R1, DHCR7, GC, CYP24A1, AMDHD1, and SEC23A) were replicated in the study (Supplementary Table 1; Supplementary Figure 2). Serum 25(OH)D in this study was measured by liquid chromatography-tandem mass spectrometry.
The characteristics of selected instrumental SNPs for specific clinical diseases and clinical traits are presented in Table 1. Contributing studies received ethical approval from their respective institutional review boards. Informed consent was obtained from all participants of contributing studies. The GWAS summary statistics of 10 clinical diseases (Supplementary Tables 2, 3) and 42 clinical traits (Supplementary Table 4) were collected from publicly available resources. We conducted a comprehensive literature review to test the horizontal pleiotropy in selected SNPs and evaluate whether any of the SNPs were influenced by linkage disequilibrium (LD). To examine assumptions 2 and 3, we chose the variant with the lowest p-value for association with clinical outcomes.
Characteristics of selected data sources
To comprehensively explore the effective atlas of vitamin D on numerous healthy outcomes (clinical diseases and clinical traits), the specific genetic SNPs were selected from public sources (Table 1). For clinical diseases, the GWAS summary-level data were extracted from the Chronic Kidney Disease Consortium (CKDGen; 561,055 controls and 64,164 cases) for CKD; the Diabetes Genetics Replication and Meta-analysis (DIAGRAM) Consortium for T2DM (824,006 controls and 74,124 cases); the International Stroke Genetics Consortium (ISGC) for stroke (454,450 controls and 67,162 cases); the coronary artery disease genome-wide replication and meta-analysis (CARDIoGRAM) plus the Coronary Artery Disease (C4D) Genetics (CARDIoGRAMplusC4D) Consortium for CAD (123,504 controls and 60,801 cases), and CAD with diabetes (11,698 controls and 3,968 cases); the International Inflammatory Bowel Disease Genetics Consortium (IIBDGC) for IBD (48,950 for ulcerative colitis and 51,109 for Crohn's disease); the Psychiatric Genomics Consortium (PGC-ALZ), the Alzheimer's Disease Sequencing Project (ADSP), and the International Genomics of Alzheimer's Project (IGAP) for AD (383,378 controls and 71,880 cases); the GEnetic Factors for OSteoporosis consortium (GEFOS) for fracture (227,116 controls and 37,857 cases); and Genetics and Allied research in Rheumatic diseases Networking (GARNET) and Rheumatoid Arthritis Consortium International (RACI) for RA (73,758 controls and 29,880 cases).
For clinical traits, 20 GWAS summary datasets for genetic determinants were available. Genome-wide association analyses have been published for adiposity (BMI, WHR adjusted BMI, and obesity) by the Genetic Investigation of Anthropometric Traits (GIANT) Consortium; glycemic traits [hemoglobin A1c (HbA1c), fasting glucose, fasting insulin, and fasting proinsulin] by the Meta-Analyses of Glucose and Insulin-Related Traits Consortium (MAGIC), plasma lipids (HDL-C, LDL-C, TC, and TG) by the Global Lipids Genetics Consortium (GLGC), bone mineral density (estimated BMD, total body BMD, forearm, femoral neck, and lumbar spine BMD), musculoskeletal measurements (lean body mass, hand grip strength, and gait speed), blood pressure (systolic BP, diastolic BP, and pulse pressure), and heart rate variability by the GEFOS and the genome-wide repository of associations between SNPs and phenotypes (GRASP). Meanwhile, the circulating cytokines and growth factors for inflammation and urinary biomarkers for kidney function, such as the urinary albumin to creatinine ratio (UACR), the urinary potassium to creatinine ratio (UK/UCr), the urinary sodium to creatinine ratio (UNa/UCr), and the urinary sodium to potassium ratio (UNa/UK), were explored.
The definitions of clinical disease and clinical traits are explained in Supplementary material. All clinical diseases were diagnosed using ICD-10 (international classification of diseases, 10th revisions) codes. The ICD-10 codes of chronic kidney disease, stroke, type-2 diabetes, coronary artery disease, CAD with diabetes, Crohn's disease, ulcerative colitis, fracture, rheumatoid arthritis, and Alzheimer's dementia are presented in Supplementary Table 7.
Statistical analysis
The summary datasets consist of effect sizes and standard errors of outcomes and exposures. However, the effect/non-effect alleles must be harmonized between outcome and exposure, when the effect allele was flipped (effect/non-effect alleles were G/T for the exposure and T/G for the outcome). Meanwhile, the alleles of outcome were matched with exposure alleles and effect alleles were aligned, when the strand of SNPs was flipped (the effect/non-effect alleles were G/T for the exposure and C/A for the outcome). Finally, we eliminated the incompatible SNPs (effect/non-effect alleles were A/G for the exposure and A/T for the outcome).
All the SNPs that independently (linkage disequilibrium r2 < 0.01) and strongly associated with the exposures at the genome-wide significant levels were extracted to verify the horizontal pleiotropy. The associations of curated SNPs with traits were assessed online (GWAS Catalog, https://www.ebi.ac.uk/gwas; ClinicalTrials. gov, https://clinicaltrials.gov; PhenoScanner, http://www.phenoscanner.medschl.cam.ac.uk) through Mendelian randomization analysis. Moreover, the Mendelian Randomization Pleiotropy RESidual Sum and Outlier (MR-PRESSO) was employed to identify and remove pleiotropic SNPs by assessing outliers among the included SNPs contributing to the Mendelian randomization estimate. We repeated the analyses after excluding potentially pleiotropic SNPs. Then, inverse-variance weighting (IVW), weighted median, weighted mode, and Mendelian randomization (MR)–Egger regression were applied to explore the causal effect of genetically decreased vitamin D on a wide spectrum of phenotypes (Supplementary material). For sensitivity analysis, bidirectional Mendelian randomization was conducted to validate the causal effects of genetic determinants of clinical diseases on vitamin D. All analyses were performed using R Version 4.0.2 with the R package Mendelian Randomization, MR-PRESSO.
Results
Associations of genetic 25(OH)D with the risk of clinical diseases
The results failed to reveal any effect of 25(OH)D on many clinical diseases (All p > 0.05) in IVW analysis (Table 2, Figure 1). Similar results were obtained in the weighted median and weighted mode statistics (p > 0.05; Supplementary Table 5). There was no significant horizontal pleiotropy and heterogeneity in all selected SNPs after excluded pleiotropic variants by MR-PRESSO (p_Het > 0.05, intercept p > 0.05, global test p > 0.05; Table 2, Figure 1, Supplementary Table 5, Supplementary Figures 3a–j).
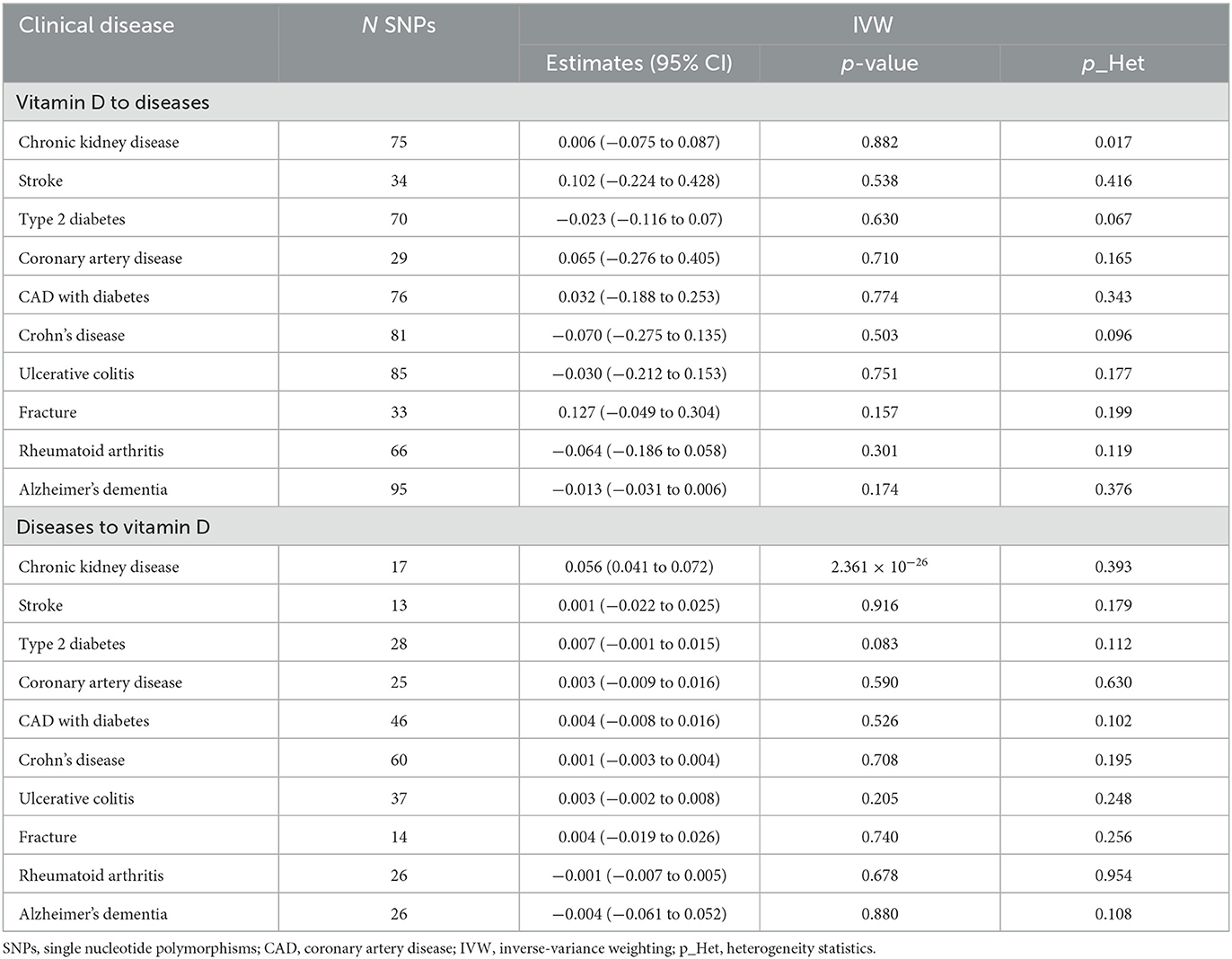
Table 2. Association of vitamin D with clinical diseases using inverse-variance weighting Mendelian randomization study.
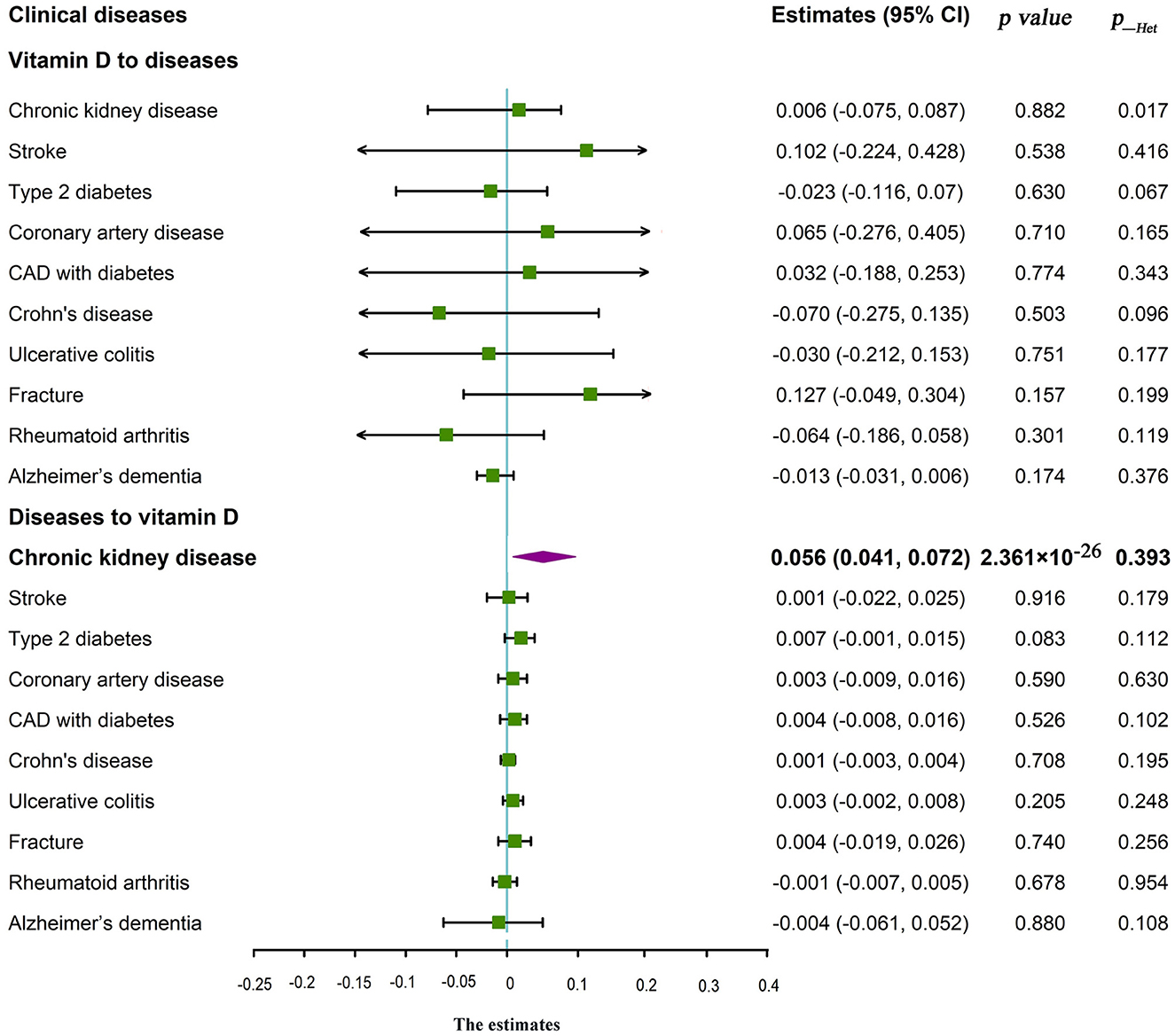
Figure 1. Forest plot of the association between circulating 25(OH)D and clinical diseases in bidirectional Mendelian randomization analysis. CAD, coronary artery disease; p_Het, heterogeneity statistics.
The inversed association between increased risk of CKD with 25(OH)D (β = 0.056, 95% CI: 0.04–0.072, p = 2.361 × 10−26, p_Het = 0.393) was found in the IVW analysis (Table 2, Figure 1). Similarly, significant associations were found in the weighted median (β = 0.057, 95% CI: 0.035–0.080, p = 3.128 × 10−25) and weighted mode (β = 0.056, 95% CI: 0.021–0.091, p = 0.002). There was no heterogeneity and horizontal pleiotropy with MR–Egger regression (Intercept p = 0.335) after excluded pleiotropic variants using the restrictive MR pleiotropy residual sum and outlier test (MR-PRESSO) method (Global test p = 0.469; Supplementary Table 5). In addition, no evidence supported the effects of clinical diseases on 25(OH)D, except CKD in reversed MR analysis (Table 2, Figure 1, Supplementary Table 5, Supplementary Figures 4a–j).
Association of genetic 25(OH)D with clinical traits
Mendelian randomization analyses were conducted to assess the association of plasma 25(OH)D with 42 clinical traits. The results revealed that genetically decreased 25(OH)D was strongly correlated with estimated BMD (g/cm2), TC (mmol/L), TG (mmol/L), and PP (mmHg), and negatively associated with lymphocyte count (%; All p < 0.05, p_Het > 0.05) in IVW (Table 3, Figure 2). There was no evidence for the association of genetically decreased vitamin D with the anthropometric index, glycemic traits, kidney function, and musculoskeletal health (Table 3, Figure 2, Supplementary Table 6). The intercepts in MR–Egger test were tightly centered around the null, which revealed the statistic effects of genetic instruments in Mendelian randomization analyses did not be influenced by pleiotropy.
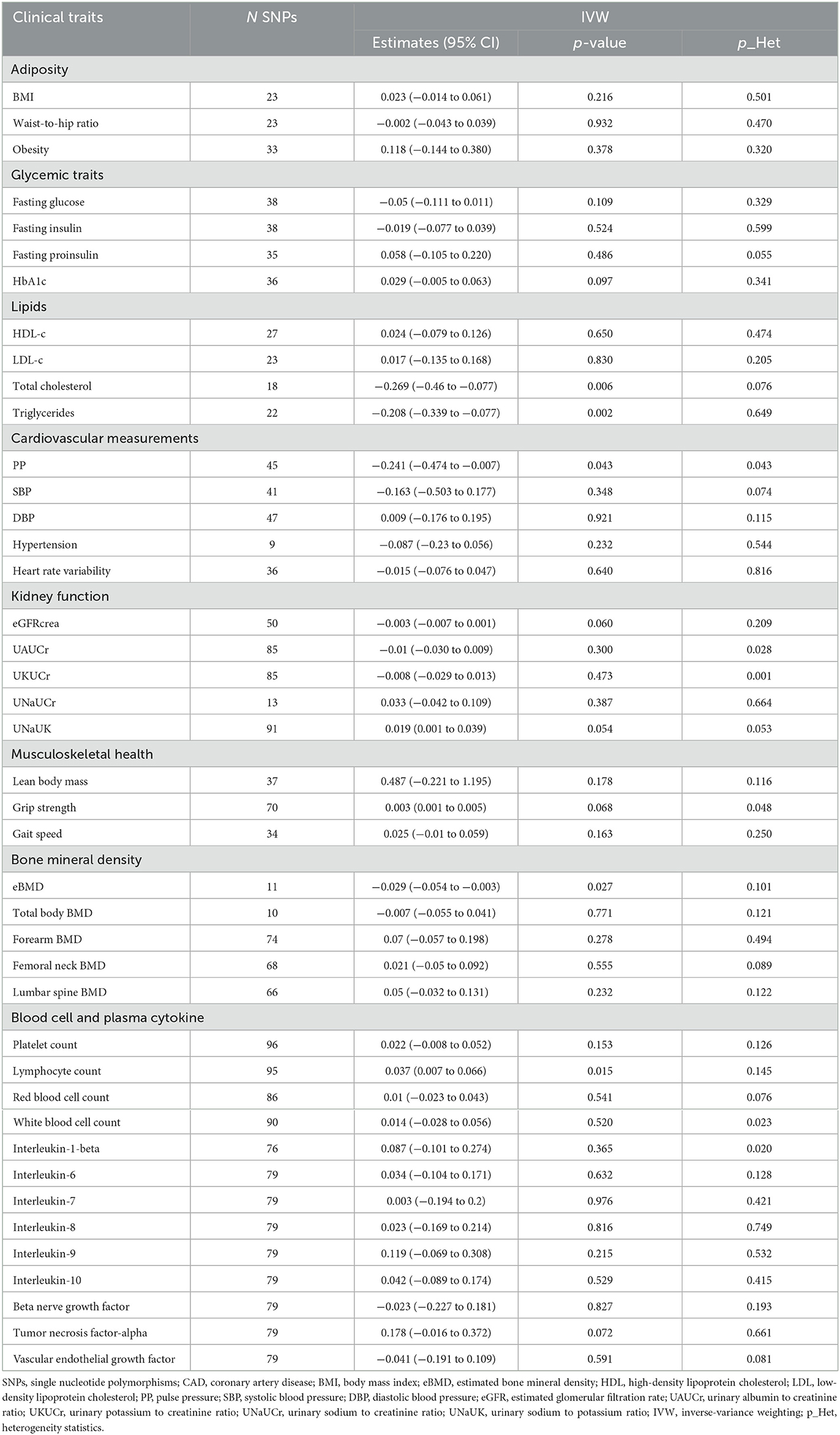
Table 3. Association of vitamin D with clinical traits using inverse-variance weighting Mendelian randomization.
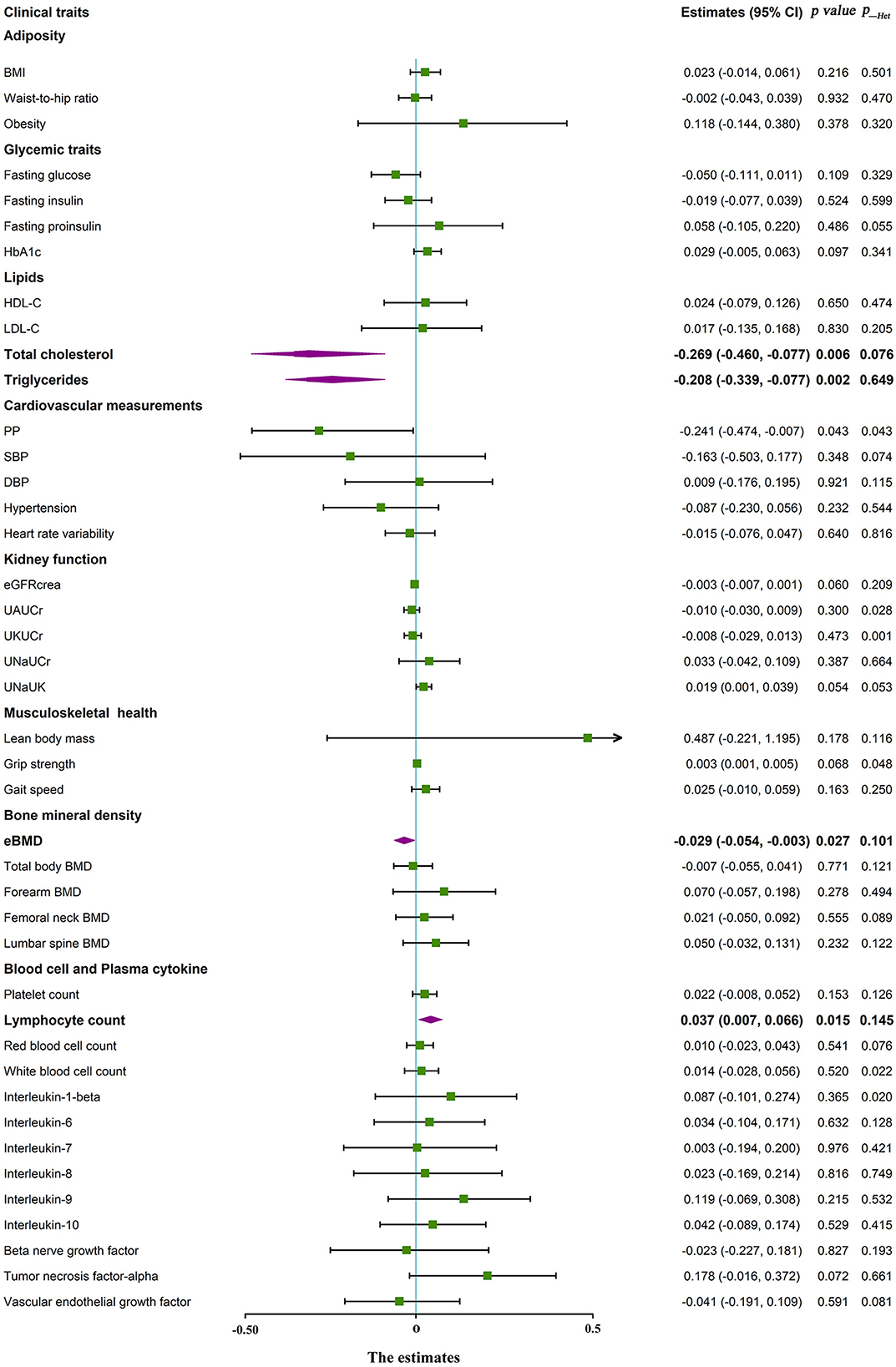
Figure 2. Forest plot about the association between circulating 25(OH)D and clinical traits in Mendelian randomization analysis. BMI, body mass index; eBMD, estimated bone mineral density; HDL, high density lipoprotein cholesterol; LDL, low density lipoprotein cholesterol; PP, pulse pressure; SBP, systolic blood pressure; DBP, diastolic blood pressure; eGFR, estimated glomerular filtration rate; UAUCr, urinary albumin to creatinine ratio; UKUCr, urinary potassium to creatinine ratio; UNaUCr, urinary sodium to creatinine ratio; UNaUK, urinary sodium to potassium ratio; p_Het, heterogeneity statistics.
Discussion
In the present Mendelian randomization (MR) study, our findings did not support the putative causal effects of 25(OH)D on multiple clinical diseases. Genetically decreased 25(OH)D was significantly associated with the estimated bone mineral density (eBMD), plasma cholesterol, pulse pressure, and elevated lymphocyte count. A bidirectional MR study did not reveal the significant effects of CVD, IBD, T2DM, AD, and musculoskeletal disorders on 25(OH)D concentration. However, chronic kidney disease was positively associated with decreased 25(OH)D.
Dietary and skin-derived vitamin D will play biological roles in multiple organs after hydroxylating in the liver and kidney (67). Patients with chronic kidney disease will decrease the biosynthesis of circulating 25(OH)D by reducing the production of hydroxylase (68). Vitamin D exerts a biological role by binding the vitamin D receptor (VDR) (69), which is commonly found in musculoskeletal cells and various extracellular tissues, such as parathyroid tissue, intestinal tissue, and kidneys (70). Vitamin D deficiency not only increased the risk of rickets, osteomalacia, and osteoporotic fractures (71) but also contributed to extra-skeletal disorders (72) in epidemiological studies. However, no significant associations of 25(OH)D with the risk of clinical diseases were found in our study. Meanwhile, the reversed MR analysis did not reveal any impact of multiple chronic diseases on serum 25(OH)D, except for CKD. The relationship between vitamin D and clinical disease may not be linear, and a linear analysis may yield negative results. A non-linear association of 25(OH)D deficiency with the risk of cardiovascular disease was discovered in a non-linear MR analysis (73), and the non-linear dose–response relationships between 25(OH)D concentrations and coronary heart disease, stroke, and mortality outcomes were discovered in a stratified MR analysis (74).
A weak correlation of 25(OH)D with estimated BMD of heel was found, but not with femoral neck BMD (FN-BMD) or lumbar spine BMD (LS-BMD) in our study. Meta-analysis of RCTs revealed that vitamin D supplementation was not associated with a lower risk of fractures in older adults (75). The MR study showed that the SNP instruments (rs2282679, rs117913124, rs10741657, rs12785878, and rs727479) were not associated with femoral neck BMD (FN-BMD), lumbar spine BMD (LS-BMD), or estimated BMD (eBMD), but rs6013897 near CYP24A1 was associated with FN-BMD at borderline statistical significance (p = 0.01). High 25(OH)D concentration was not associated with higher FN-BMD (p = 0.37) or LS-BMD (p = 0.49) in the inverse-variance weighted analysis or in sensitivity analyses but was associated with estimated BMD (p = 0.02) (76). The differences in the biological pathways of 25(OH)D-SNPs may be the critical factor causing the contradictory result. Mendelian randomization analyses that combine all SNP effects may mask the role of individual SNPs. Moreover, the beneficial effects of vitamin D on many diseases may be largely due to undetected confounders in an epidemiologic study.
In the present study, no significant associations of genetic 25(OH)D with the risk of CAD, stroke, and T2DM were uncovered. The putative causal effects of circulating vitamin D on plasma triglyceride and total cholesterol were uncovered. Previous observational studies revealed that circulating 25(OH)D was inversely associated with blood pressure and the risk of type-2 diabetes (77), but positively correlated with blood lipids (triglycerides, HDL-c, LDL-c, and total cholesterol) (16). Moreover, vitamin D supplementation will increase LDL-cholesterol concentrations (78). The genetic SNPs were not only related to circulating 25-hydroxyvitamin D but also plasma LDL-cholesterol and triglyceride levels. Pleiotropy-associated confounding cannot be completely ruled out (79).
The SNPs used in the previous two-stage and two-sample Mendelian randomization studies were mainly obtained from the SUNLIGHT consortium (study of underlying genetic determinants of Vitamin D and highly related traits) GWAS, which consisted of 31 cohorts from Europe, Canada, and the USA with atotal of 79,366 samples. The statistical effects of all SNPs were calculated by fixed-effect inverse-variance weighted meta-analysis. The joint test with multiple cohorts will easily induce an undetected bias due to the disparate measurements for 25(OH)D and the adjusted covariates. Meanwhile, we were unable to estimate the interactions between genetic variants and dietary intake of vitamin D as well as sunlight exposure as a source of vitamin D production in the skin. In the current study, the adequate instrumental SNPs involved in vitamin D synthesis (DHCR7/NADSYN1 and CYP2R1), transportation (GC), and degradation (CYP24A1), as well as novel vitamin D metabolism pathways, such as SEC23A (Sec23 homolog A, coat protein complex II component) and amidohydrolase domain containing 1 (AMDHD1), were recruited from the UK Biobank study with 401,460 white British participants. Moreover, the association between SNPs and 25(OH)D was estimated by linear mixed-model and the interactions of age, sex, and season with 25(OH)D were evaluated. The subjects in the GWAS analysis investigating the relationship between genetic loci and clinical features were of European and Caucasian descent, which is congruent with the population in UK Biobank. A comprehensive network of Mendelian randomization analysis, inverse-variance weighting (IVW), weighted median, weighted mode, and Mendelian randomization (MR)–Egger regression were performed to explore the causal effects and pleiotropy, as well as avoid bias. Mendelian randomization pleiotropy RESidual Sum and Outlier (MR-PRESSO) was conducted to uncover and exclude pleiotropic SNPs. The methods in our study provided a credible result for the large-scale study.
Here, several strengths of our study should be mentioned. First, the panoptic atlas of 25(OH)D in many clinical diseases and healthy traits were explored in this MR analysis, which will help us to have a more comprehensive understanding of the relationship between 25(OH)D and health. In addition, the bidirectional two-sample MR study was conducted to estimate the influence of diseases on 25(OH)D concentrations. Second, the SNP biomarkers and SNP estimates were obtained in mostly European studies, thus minimizing the possibility of population stratification bias. Third, the genetic SNPs for vitamin D were derived from a recent large-scale GWAS study (n = 443,734) rather than an earlier SUNLIGHT study, which may be more representative of the genetic instruments used to explore the genetic correlation of vitamin D.
However, there are some potential limitations worth noting in the current study.
Although we performed tests to prevent pleiotropy, some genetic SNPs are not only related to circulating 25-hydroxyvitamin D but also to traits, such as plasma LDL-cholesterol and triglyceride levels, and the potential pleiotropy cannot be definitively excluded. Moreover, the non-linear association between 25(OH)D and clinical outcomes could not be assessed by our two-sample MR analysis with a summary dataset. A non-linear MR analysis using raw data is needed in future. Meanwhile, 25(OH)D was recruited as the symbol of serum vitamin D concentration. In addition, the biological roles of active metabolite 1,25-dihydroxy vitamin D in clinical outcomes were not explored. Meanwhile, we still failed to study the biological roles of 24, 25-(OH)2-D3, 1, 24, 25-(OH)3-D3, and 25, 26-(OH)2-D3 metabolites and epimer of vitamin D in health (80). In addition, our results were not likely biased by pleiotropy due to the fact that 25OHD-associated genetic variants were not associated with other lifestyles, which influenced clinical outcomes, such as drinking, physical activity, or smoking. However, we cannot exclude the possibility that such an association may have a genetic basis rather than a causal relationship.
Hence, a large-scale genome-wide scan for genetic variants of vitamin D and further investigation to understand the potential role of vitamin D in the development of clinical outcomes are required. Meanwhile, a long-term and multicentric RCT study that can avoid the interference of numerous known and unpredictable confounders on the results, such as diet, exercise, sleep, geographic latitude, and climate, is seriously needed.
Conclusion
Our study suggested that there was no evidence of the causal effect of 25(OH)D on numerous clinical diseases. Genetically decreased serum vitamin D was associated with estimated bone mineral density evaluated by ultrasound of the heel, plasma cholesterol, pulse pressure, and elevated lymphocyte count. Chronic kidney disease was inversely related to serum 25(OH)D concentration. The putative causal effects of vitamin D on multiple clinical diseases was not supported.
Author contributions
K-qL: concept and design. J-jX: data collection, statistics, and writing the manuscript. X-bZ and TY: data collection. W-tT: data interpretation. K-qL and J-jX: study supervision. All authors critically revised the manuscript and approved the submitted version.
Acknowledgments
The authors thank all the staff of the Departments of Medicine, Human Genetics, Epidemiology and Biostatistics, McGill University, Montreal, Canada.
Conflict of interest
The authors declare that the research was conducted in the absence of any commercial or financial relationships that could be construed as a potential conflict of interest.
Publisher's note
All claims expressed in this article are solely those of the authors and do not necessarily represent those of their affiliated organizations, or those of the publisher, the editors and the reviewers. Any product that may be evaluated in this article, or claim that may be made by its manufacturer, is not guaranteed or endorsed by the publisher.
Supplementary material
The Supplementary Material for this article can be found online at: https://www.frontiersin.org/articles/10.3389/fnut.2023.1108477/full#supplementary-material
Supplementary Table 1. Characterics of 138 conditionally independent SNPs that reach genome-wide significance in the UKBB GWAS for 25OHD.
Supplementary Table 2. The effect estimates of the instrumental variables for 25(OH)D (exposure) and clinical diseases.
Supplementary Table 3. The effect estimates of the instrumental variables for clinical diseases (exposure) and 25(OH)D.
Supplementary Table 4. The effect estimates of the instrumental variables for 25(OH)D (exposure) and clinical traits.
Supplementary Table 5. Association of vitamin D with clinical diseases using other mendelian randomization studies in addition to inverse variance weighting.
Supplementary Table 6. Association of vitamin D with clinical traits using other mendelian randomization studies in addition to inverse variance weighting.
Supplementary Table 7. The International Classification of Diseases (ICD) codes for included clinical diseases.
Supplementary Figure 1. Assumptions of bidirectional Mendelian randomization analysis. (a) Main assumption of Mendelian randomization analysis. (b) Reversed assumption of Mendelian randomization analysis. (1) The genetic instrumental variables are strongly associated with exposures; (2) the genetic instrumental variables are not associated with any known or unmeasured confounders: influencing the association between genetic variants and outcomes; (3) the genetic variants are associated with outcomes only through exposures: variants causing significant effects on outcomes not through other pathways, no horizontal pleiotropy.
Supplementary Figure 2. Biological metabolic pathways of vitamin D.
Supplementary Figure 3. Scatter plot of the IVW MR study investigating the effect of 25(OH)D on clinical diseases. (a) Effect of vitamin D on chronic kidney disease. (b) Effect of vitamin D on stroke. (c) Effect of vitamin D on type-2 diabetes. (d) Effect of vitamin D on coronary artery disease. (e) Effect of vitamin D on coronary artery disease with diabetes. (f) Effect of vitamin D on Crohn's disease. (g) Effect of vitamin D on ulcerative colitis. (h) Effect of vitamin D on fracture. (i) Effect of vitamin D on rheumatoid arthritis. (j) Effect of vitamin D on Alzheimer's dementia. The x-axis represents the genetic association with 25OHD levels; the y-axis represents the genetic association with the risk of clinical diseases. The line represents the IVW method. 25OHD, 25-hydroxyvitamin D (vertical and horizontal blue lines around points show a 95% confidence interval for each polymorphism).
Supplementary Figure 4. Scatter plot of the IVW method in bidirectional Mendelian randomization analysis investigating the effect of clinical diseases on 25(OH)D. (a) Effect of chronic kidney disease on vitamin D. (b) Effect of stroke on vitamin D. (c) Effect of type-2 diabetes on vitamin D. (d) Effect of coronary artery disease on vitamin D. (e) Effect of coronary artery disease with diabetes on vitamin D. (f) Effect of Crohn's disease on vitamin D. (g) Effect of ulcerative colitis on vitamin D. (h) Effect of fracture on vitamin D. (i) Effect of rheumatoid arthritis on vitamin D. (j) Effect of Alzheimer's dementia on vitamin D. The x-axis represents the genetic association with the risk of clinical diseases; the y-axis represents the genetic association with 25OHD levels; The line represents the IVW method. 25OHD, 25-hydroxyvitamin D (vertical and horizontal blue lines around points show a 95% confidence interval for each polymorphism).
Abbreviations
BMD, bone mineral density; BMI, body mass index; HDL, high density lipoprotein cholesterol; LDL, low density lipoprotein cholesterol; PP, pulse pressure; SBP, systolic blood pressure; DBP, diastolic blood pressure; eGFR, estimated glomerular filtration rate; UAUCr, urinary albumin to creatinine ratio; UKUCr, urinary potassium to creatinine ratio; UNaUCr, urinary sodium to creatinine ratio; UNaUK, urinary sodium to potassium ratio; CAD, coronary artery disease; CD, Crohn's disease; UC, ulcerative colitis; LD, linkage disequilibrium; GWAS, genome-wide association study; MAF, minor allele frequency; MR, Mendelian randomization; IVW, inverse-variance weighting; MRPRESSO, MR-Pleiotropy RESidual Sum and Outlier method; GRASP, genome-wide repository of associations between SNPs and phenotypes; GIANT, genetic investigation of anthropometric traits; MAGIC, The Meta-Analyses of Glucose and Insulin-Related Traits Consortium; GLGC, The Global Lipids Genetics Consortium; CKDGen, The Chronic Kidney Disease Consortium; GEFOS, The GEnetic Factors for OSteoporosis consortium; ISGC, International Stroke Genetics Consortium; DIAGRAM, diabetes genetics replication and meta-analysis; CARDIoGRAMplusC4D, coronary artery disease genome-wide replication and meta-analysis plus the coronary artery disease (C4D) genetics; IBDGenetics, International Inflammatory Bowel Disease Genetics Consortium.
References
1. Duchow EG, Cooke NE, Seeman J, Plum LA, DeLuca HF. Vitamin D binding protein is required to utilize skin-generated vitamin D. Proc Natl Acad Sci U S A. (2019) 116:201915442. doi: 10.1073/pnas.1915442116
2. Banerjee A, Khemka VK, Roy D, Poddar J, Roy TKS, Karnam SA. Role of serum adiponectin and vitamin D in prediabetes and diabetes mellitus. Can J Diabetes. (2017) 41:259–65. doi: 10.1016/j.jcjd.2016.10.006
3. Kahwati LC, LeBlanc E, Weber RP, Giger K, Clark R, Suvada K, et al. Screening for vitamin D deficiency in adults: updated evidence report and systematic review for the US Preventive Services Task Force. JAMA. (2021) 325:1443–63. doi: 10.1001/jama.2020.26498
4. Jiang Z, Pu R, Li N, Chen C, Li J, Dai W, et al. High prevalence of vitamin D deficiency in Asia: a systematic review and meta-analysis. Crit Rev Food Sci Nutr. (2021) 1−10. doi: 10.1080/10408398.2021.1990850
5. Pilz S, Verheyen N, Grubler MR, Tomaschitz A, Marz W. Vitamin D and cardiovascular disease prevention. Nat Rev Cardiol. (2016) 13:404–17. doi: 10.1038/nrcardio.2016.73
6. Vimaleswaran KS, Berry DJ, Lu C, Tikkanen E, Pilz S, Hiraki LT, et al. Causal relationship between obesity and vitamin D status: bi-directional Mendelian randomization analysis of multiple cohorts. PLoS Med. (2013) 10:e1001383. doi: 10.1371/journal.pmed.1001383
7. Pittas AG, Dawson-Hughes B, Sheehan P, Ware JH, Knowler WC, Aroda VR, et al. Vitamin D supplementation and prevention of type 2 diabetes. N Engl J Med. (2019) 381:520–30. doi: 10.1056/NEJMoa1900906
8. Mokry LE, Ross S, Ahmad OS, Forgetta V, Smith GD, Goltzman D, et al. Vitamin D and risk of multiple sclerosis: a Mendelian randomization study. PLoS Med. (2015) 12:e1001866. doi: 10.1371/journal.pmed.1001866
9. Agmon-Levin N, Theodor E, Segal RM, Shoenfeld Y. Vitamin D in systemic and organ-specific autoimmune diseases. Clin Rev Allergy Immunol. (2013) 45:256–66. doi: 10.1007/s12016-012-8342-y
10. Zhang P, Guo D, Xu B, Huang C, Yang S, Wang W, et al. Association of serum 25-hydroxyvitamin D with cardiovascular outcomes and all-cause mortality in individuals with prediabetes and diabetes: results from the UK Biobank Prospective Cohort Study. Diabetes Care. (2022) 45:1219–29. doi: 10.2337/dc21-2193
11. Wan Z, Guo J, Pan A, Chen C, Liu L, Liu G. Association of serum 25-hydroxyvitamin D concentrations with all-cause and cause-specific mortality among individuals with diabetes. Diabetes Care. (2021) 44:350–7. doi: 10.2337/dc20-1485
12. Manousaki D, Paternoster L, Standl M, Moffatt MF, Farrall M, Bouzigon E, et al. Vitamin D levels and susceptibility to asthma, elevated immunoglobulin E levels, and atopic dermatitis: a Mendelian randomization study. PLoS Med. (2017) 14:e1002294. doi: 10.1371/journal.pmed.1002294
13. Brahmbhatt S, Mikhail M, Islam S, Aloia JF. Vitamin D and abdominal aortic calcification in older African American women, the PODA clinical trial. Nutrients. (2020) 12:861. doi: 10.3390/nu12030861
14. Jayedi A, Rashidy-Pour A, Shab-Bidar S. Vitamin D status and risk of dementia and Alzheimer's disease: a meta-analysis of dose-response (dagger). Nutr Neurosci. (2019) 22:750–9. doi: 10.1080/1028415X.2018.1436639
15. Manson JE, Bassuk S, Cook NR, Lee IM, Mora S, Albert CM, et al. Vitamin D, marine n-3 fatty acids, and primary prevention of cardiovascular disease current evidence. Circ Res. (2020) 126:112–28. doi: 10.1161/CIRCRESAHA.119.314541
16. Durazo-Arvizu RA, Pacheco-Dominguez RL, Sempos CT, Kramer H, Hoofnagle AN, Pirzada A, et al. The association between cardiovascular disease risk factors and 25-hydroxivitamin D and related analytes among Hispanic/Latino adults: a pilot study. Nutrients. (2019) 11:1959. doi: 10.3390/nu11081959
17. Burt LA, Billington EO, Rose MS, Raymond DA, Hanley DA, Boyd SK. Effect of high-dose vitamin D supplementation on volumetric bone density and bone strength: a randomized clinical trial. JAMA. (2019) 322:736–45. doi: 10.1001/jama.2019.11889
18. Zhang Y, Fang F, Tang J, Jia L, Feng Y, Xu P, et al. Association between vitamin D supplementation and mortality: systematic review and meta-analysis. BMJ. (2019) 366:l4673. doi: 10.1136/bmj.l4673
19. Keum N, Lee DH, Greenwood DC, Manson JE, Giovannucci E. Vitamin D supplementation and total cancer incidence and mortality: a meta-analysis of randomized controlled trials. Ann Oncol. (2019) 30:733–43. doi: 10.1093/annonc/mdz059
20. Hernández-Alonso P, Boughanem H, Canudas S, Becerra-Tomás N, Fernández de la Puente M, Babio N, et al. Circulating vitamin D levels and colorectal cancer risk: a meta-analysis and systematic review of case-control and prospective cohort studies. Crit Rev Food Sci Nutr. (2023) 63:1–17. doi: 10.1080/10408398.2021.1939649
21. Swart KM, Lips P, Brouwer IA, Jorde R, Heymans MW, Grimnes G, et al. Effects of vitamin D supplementation on markers for cardiovascular disease and type 2 diabetes: an individual participant data meta-analysis of randomized controlled trials. Am J Clin Nutr. (2018) 107:1043–53. doi: 10.1093/ajcn/nqy078
22. Li X, Liu Y, Zheng Y, Wang P, Zhang Y. The effect of vitamin D supplementation on glycemic control in type 2 diabetes patients: a systematic review and meta-analysis. Nutrients. (2018) 10:375. doi: 10.3390/nu10030375
23. Manson JE, Cook NR, Lee IM, Christen W, Bassuk S, Mora S, et al. Vitamin D supplements and prevention of cancer and cardiovascular disease. N Engl J Med. (2019) 380:33–44. doi: 10.1056/NEJMoa1809944
24. Scragg R, Stewart AW, Waayer D, Lawes CM, Toop L, Sluyter J, et al. Effect of monthly high-dose vitamin d supplementation on cardiovascular disease in the vitamin D assessment study: a randomized clinical trial. JAMA Cardiol. (2017) 2:608–16. doi: 10.1001/jamacardio.2017.0175
25. Urashima M, Ohdaira H, Akutsu T, Okada S, Yoshida M, Kitajima M, et al. Effect of vitamin D supplementation on relapse-free survival among patients with digestive tract cancers: the AMATERASU Randomized Clinical Trial. JAMA. (2019) 321:1361–9. doi: 10.1001/jama.2019.2210
26. Ng K, Nimeiri HS, McCleary NJ, Abrams TA, Yurgelun MB, Cleary JM, et al. Effect of high-dose vs. standard-dose vitamin D3 supplementation on progression-free survival among patients with advanced or metastatic colorectal cancer: the sunshine randomized clinical trial. JAMA. (2019) 321:1370–9. doi: 10.1001/jama.2019.2402
27. Ye Z, Sharp SJ, Burgess S, Scott RA, Imamura F, Langenberg C, et al. Association between circulating 25-hydroxyvitamin D and incident type 2 diabetes: a Mendelian randomisation study. Lancet Diabetes Endocrinol. (2015) 3:35–42. doi: 10.1016/S2213-8587(14)70184-6
28. Lund-Nielsen J, Vedel-Krogh S, Kobylecki CJ, Brynskov J, Afzal S, Nordestgaard BG. Vitamin D and inflammatory bowel disease: Mendelian randomization analyses in the copenhagen studies and UK Biobank. J Clin Endocrinol Metab. (2018) 103:3267–77. doi: 10.1210/jc.2018-00250
29. Wang N, Chen C, Zhao L, Chen Y, Han B, Xia F, et al. Vitamin D and nonalcoholic fatty liver disease: bi-directional mendelian randomization analysis. EBioMedicine. (2018) 28:187–93. doi: 10.1016/j.ebiom.2017.12.027
30. He Y, Timofeeva M, Farrington SM, Vaughan-Shaw P, Svinti V, Walker M, et al. Exploring causality in the association between circulating 25-hydroxyvitamin D and colorectal cancer risk: a large Mendelian randomisation study. BMC Med. (2018) 16:142. doi: 10.1186/s12916-018-1119-2
31. Yuan S, Jiang X, Michaelsson K, Larsson SC. Genetic Prediction of serum 25-hydroxyvitamin D, calcium, and parathyroid hormone levels in relation to development of type 2 diabetes: a Mendelian randomization study. Diabetes Care. (2019) 42:2197–203. doi: 10.2337/dc19-1247
32. Sun JY, Zhao M, Hou Y, Zhang C, Oh J, Sun Z, et al. Circulating serum vitamin D levels and total body bone mineral density: a Mendelian randomization study. J Cell Mol Med. (2019) 23:2268–71. doi: 10.1111/jcmm.14153
33. Vimaleswaran KS, Cavadino A, Berry DJ, Jorde R, Dieffenbach AK, Lu C, et al. Association of vitamin D status with arterial blood pressure and hypertension risk: a mendelian randomisation study. Lancet Diabetes Endocrinol. (2014) 2:719–29. doi: 10.1016/S2213-8587(14)70113-5
34. Jiang X, O'Reilly PF, Aschard H, Hsu Y-H, Richards JB, Dupuis J, et al. Genome-wide association study in 79,366 European-ancestry individuals informs the genetic architecture of 25-hydroxyvitamin D levels. Nat Commun. (2018) 9:260. doi: 10.1038/s41467-017-02662-2
35. Bowden J, Spiller W, Del Greco MF, Sheehan N, Thompson J, Minelli C, et al. Improving the visualization, interpretation and analysis of two-sample summary data Mendelian randomization via the radial plot and radial regression. Int J Epidemiol. (2018) 47:1264–78. doi: 10.1093/ije/dyy101
36. Didelez V, Sheehan N. Mendelian randomization as an instrumental variable approach to causal inference. Stat Methods Med Res. (2007) 16:309–30. doi: 10.1177/0962280206077743
37. Manousaki D, Mitchell R, Dudding T, Haworth S, Harroud A, Forgetta V, et al. Genome-wide association study for vitamin D levels reveals 69 independent loci. Am J Hum Genet. (2020) 106:327–37. doi: 10.1016/j.ajhg.2020.01.017
38. Turcot V, Lu Y, Highland HM, Schurmann C, Justice AE, Fine RS, et al. Protein-altering variants associated with body mass index implicate pathways that control energy intake and expenditure in obesity. Nat Genet. (2018) 50:26–41. doi: 10.1038/s41588-017-0011-x
39. Pulit SL, Stoneman C, Morris AP, Wood AR, Glastonbury CA, Tyrrell J, et al. Meta-analysis of genome-wide association studies for body fat distribution in 694 649 individuals of European ancestry. Hum Mol Genet. (2019) 28:166–74. doi: 10.1093/hmg/ddy327
40. Berndt SI, Gustafsson S, Magi R, Ganna A, Wheeler E, Feitosa MF, et al. Genome-wide meta-analysis identifies 11 new loci for anthropometric traits and provides insights into genetic architecture. Nat Genet. (2013) 45:501–12. doi: 10.1038/ng.2606
41. Manning AK, Hivert MF, Scott RA, Grimsby JL, Bouatia-Naji N, Chen H, et al. A genome-wide approach accounting for body mass index identifies genetic variants influencing fasting glycemic traits and insulin resistance. Nat Genet. (2012) 44:659–69. doi: 10.1038/ng.2274
42. Strawbridge RJ, Dupuis J, Prokopenko I, Barker A, Ahlqvist E, Rybin D, et al. Genome-wide association identifies nine common variants associated with fasting proinsulin levels and provides new insights into the pathophysiology of type 2 diabetes. Diabetes. (2011) 60:2624–34. doi: 10.2337/db11-0415
43. Soranzo N, Sanna S, Wheeler E, Gieger C, Radke D, Dupuis J, et al. Common variants at 10 genomic loci influence hemoglobin A1C levels via glycemic and nonglycemic pathways. Diabetes. (2010) 59:3229–39. doi: 10.2337/db10-0502
44. Willer C, Schmidt EM, Sengupta S, Peloso GM, Gustafsson S, Kanoni S, et al. Discovery and refinement of loci associated with lipid levels. Nat Genet. (2013) 45:1274–83. doi: 10.1038/ng.2797
45. Evangelou E, Warren HR, Mosen-Ansorena D, Mifsud B, Pazoki R, Gao H, et al. Genetic analysis of over 1 million people identifies 535 new loci associated with blood pressure traits. Nat Genet. (2018) 50:1412–25. doi: 10.1038/s41588-018-0205-x
46. Liu C, Kraja AT, Smith JA, Brody JA, Franceschini N, Bis JC, et al. Meta-analysis identifies common and rare variants influencing blood pressure and overlapping with metabolic trait loci. Nat Genet. (2016) 48:1162–70. doi: 10.1038/ng.3660
47. Nolte IM, Munoz ML, Tragante V, Amare AT, Jansen R, Vaez A, et al. Genetic loci associated with heart rate variability and their effects on cardiac disease risk. Nat Commun. (2017) 8:15805. doi: 10.1038/ncomms15805
48. Wuttke M, Li Y, Li M, Sieber KB, Pattaro C. A catalog of genetic loci associated with kidney function from analyses of a million individuals. Nat Genet. (2019) 51:957–72. doi: 10.1038/s41588-019-0407-x
49. Zanetti D, Rao A, Gustafsson S, Assimes TL, Montgomery SB, Ingelsson E. Identification of 22 novel loci associated with urinary biomarkers of albumin, sodium, and potassium excretion. Kidney Int. (2019) 95:1197–208. doi: 10.1016/j.kint.2018.12.017
50. Zillikens MC, Demissie S, Hsu Y-H, Yerges-Armstrong LM, Chou W-C, Stolk L, et al. Erratum: Large meta-analysis of genome-wide association studies identifies five loci for lean body mass. Nat Commun. (2017) 8:1414. doi: 10.1038/s41467-017-01008-2
51. Willems SM, Wright DJ, Day FR, Trajanoska K, Joshi PK, Morris JA, et al. Large-scale GWAS identifies multiple loci for hand grip strength providing biological insights into muscular fitness. Nat Commun. (2017) 8:16015. doi: 10.1038/ncomms16015
52. Ben-Avraham D, Karasik D, Verghese J, Lunetta KL, Smith JA, Eicher JD, et al. Correction: The complex genetics of gait speed: genome-wide meta-analysis approach. Aging. (2017) 9:1844–6. doi: 10.18632/aging.101260
53. Kemp JP, Morris JA, Medina-Gomez C, Forgetta V, Warrington NM, Youlten SE, et al. Identification of 153 new loci associated with heel bone mineral density and functional involvement of GPC6 in osteoporosis. Nat Genet. (2017) 49:1468–75. doi: 10.1038/ng.3949
54. Medina-Gomez C, Kemp JP, Trajanoska K, Luan J, Chesi A, Ahluwalia TS, et al. Life-course genome-wide association study meta-analysis of total body BMD and assessment of age-specific effects. Am J Hum Genet. (2018) 102:88–102. doi: 10.1016/j.ajhg.2017.12.005
55. Zheng HF, Forgetta V, Hsu YH, Estrada K, Rosello-Diez A, Leo PJ, et al. Whole-genome sequencing identifies EN1 as a determinant of bone density and fracture. Nature. (2015) 526:112–7. doi: 10.1038/nature14878
56. Astle WJ, Elding H, Jiang T, Allen D, Ruklisa D, Mann AL, et al. The allelic landscape of human blood cell trait variation and links to common complex disease. Cell. (2016) 167:1415–29. doi: 10.1016/j.cell.2016.10.042
57. Ahola-Olli AV, Wurtz P, Havulinna AS, Aalto K, Pitkanen N, Lehtimaki T, et al. Genome-wide association study identifies 27 loci influencing concentrations of circulating cytokines and growth factors. Am J Hum Genet. (2017) 100:40–50. doi: 10.1016/j.ajhg.2016.11.007
58. Malik R, Chauhan G, Traylor M, Sargurupremraj M, Okada Y, Mishra A, et al. Multiancestry genome-wide association study of 520,000 subjects identifies 32 loci associated with stroke and stroke subtypes. Nat Genet. (2018) 50:524–37. doi: 10.1038/s41588-018-0058-3
59. Mahajan A, Taliun D, Thurner M, Robertson NR, Torres JM, Rayner NW, et al. Fine-mapping type 2 diabetes loci to single-variant resolution using high-density imputation and islet-specific epigenome maps. Nat Genet. (2018) 50:1505–13. doi: 10.1038/s41588-018-0241-6
60. Nikpay M, Goel A, Won H, Hall LM, Willenborg C, Kanoni S, et al. A comprehensive 1,000 Genomes-based genome-wide association meta-analysis of coronary artery disease. Nat Genet. (2015) 47:1121–30. doi: 10.1038/ng.3396
61. Fall T, Gustafsson S, Orho-Melander M, Ingelsson E. Genome-wide association study of coronary artery disease among individuals with diabetes: the UK Biobank. Diabetologia. (2018) 61:2174–9. doi: 10.1007/s00125-018-4686-z
62. Franke A, McGovern DPB, Barrett JC, Wang K, Radford-Smith GL, Ahmad T, et al. Genome-wide meta-analysis increases to 71 the number of confirmed Crohn's disease susceptibility loci. Nat Genet. (2010) 42:1118–25. doi: 10.1038/ng.717
63. Anderson CA, Boucher G, Lees CW, Franke A, D'Amato M, Taylor KD, et al. Meta-analysis identifies 29 additional ulcerative colitis risk loci, increasing the number of confirmed associations to 47. Nat Genet. (2011) 43:246–52. doi: 10.1038/ng.764
64. Trajanoska K, Morris JA, Oei L, Zheng HF, Evans DM, Kiel DP, et al. Assessment of the genetic and clinical determinants of fracture risk: genome wide association and Mendelian randomisation study. BMJ. (2018) 362:k3225. doi: 10.1136/bmj.k3225
65. Okada Y, Wu D, Trynka G, Raj T, Terao C, Ikari K, et al. Genetics of rheumatoid arthritis contributes to biology and drug discovery. Nature. (2014) 506:376–81. doi: 10.1038/nature12873
66. White LR, Sealock J, Almdahl IS, Jansen IE, Steinberg S, Stefansson H, et al. Genome-wide meta-analysis identifies new loci and functional pathways influencing Alzheimer's disease risk. Nat Genet. (2019) 51:404–13. doi: 10.1038/s41588-018-0311-9
67. Bikle D, Christakos S. New aspects of vitamin D metabolism and action - addressing the skin as source and target. Nat Rev Endocrinol. (2020) 16:234–52. doi: 10.1038/s41574-019-0312-5
68. Zittermann A, Ernst JB, Birschmann I, Dittrich M. Effect of vitamin D or activated vitamin D on circulating 1,25-dihydroxyvitamin D concentrations: a systematic review and metaanalysis of randomized controlled trials. Clin Chem. (2015) 61:1484–94. doi: 10.1373/clinchem.2015.244913
69. Haussler MR, Jurutka PW, Mizwicki M, Norman AW. Vitamin D receptor (VDR)-mediated actions of 1α,25(OH)2vitamin D3: genomic and non-genomic mechanisms. Best Pract Res Clin Endocrinol Metab. (2011) 25:543–59. doi: 10.1016/j.beem.2011.05.010
70. Yang S, Li A, Wang J, Liu J, Han Y, Zhang W, et al. Vitamin D receptor: a novel therapeutic target for kidney diseases. Curr Med Chem. (2018) 25:3256–71. doi: 10.2174/0929867325666180214122352
71. Reid IR, Bolland MJ, Grey A. Effects of vitamin D supplements on bone mineral density: a systematic review and meta-analysis. Lancet. (2014) 383:146–55. doi: 10.1016/S0140-6736(13)61647-5
72. Jeon SM, Shin EA. Exploring vitamin D metabolism and function in cancer. Exp Mol Med. (2018) 50:1–14. doi: 10.1038/s12276-018-0038-9
73. Emerging Risk Factors Collaboration/EPIC-CVD/Vitamin D Studies Collaboration. Estimating dose-response relationships for vitamin D with coronary heart disease, stroke, and all-cause mortality: observational and Mendelian randomisation analyses. Lancet Diabetes Endocrinol. (2021) 9:837–46. doi: 10.1016/S2213-8587(21)00263-1
74. Zhou A, Selvanayagam JB, Hyppönen E. Non-linear Mendelian randomization analyses support a role for vitamin D deficiency in cardiovascular disease risk. Eur Heart J. (2022) 43:1731–9. doi: 10.1093/eurheartj/ehab809
75. Zhao JG, Zeng XT, Wang J, Liu L. Association between calcium or vitamin D supplementation and fracture incidence in community-dwelling older adults: a systematic review and meta-analysis. JAMA. (2017) 318:2466–82. doi: 10.1001/jama.2017.19344
76. Larsson SC, Melhus H, Michaëlsson K. Circulating serum 25-hydroxyvitamin D levels and bone mineral density: Mendelian randomization study. J Bone Miner Res. (2018) 33:840–4. doi: 10.1002/jbmr.3389
77. Zheng J-S, Imamura F, Sharp SJ, van der Schouw YT, Sluijs I, Gundersen TE, et al. Association of plasma vitamin D metabolites with incident type 2 diabetes: EPIC-InterAct case-cohort study. J Clin Endocrinol Metab. (2019) 104:1293–303. doi: 10.1210/jc.2018-01522
78. Zittermann A, Frisch S, Berthold HK, Götting C, Kuhn J, Kleesiek K, et al. Vitamin D supplementation enhances the beneficial effects of weight loss on cardiovascular disease risk markers. Am J Clin Nutr. (2009) 89:1321–7. doi: 10.3945/ajcn.2008.27004
79. Burgess S, Gill D. Genetic evidence for vitamin D and cardiovascular disease: choice of variants is critical. Eur Heart J. (2022) 43:1740–2. doi: 10.1093/eurheartj/ehab870
Keywords: circulating vitamin D, complex diseases, association, Mendelian randomization (MR) analysis, phenome wide association studies
Citation: Xu J-j, Zhang X-b, Tong W-t, Ying T and Liu K-q (2023) Phenome-wide Mendelian randomization study evaluating the association of circulating vitamin D with complex diseases. Front. Nutr. 10:1108477. doi: 10.3389/fnut.2023.1108477
Received: 26 November 2022; Accepted: 01 March 2023;
Published: 29 March 2023.
Edited by:
Shuang Song, Dalian Polytechnic University, ChinaReviewed by:
Armin Zittermann, Heart and Diabetes Center North Rhine-Westphalia, GermanySisi Cao, San Diego State University, United States
Copyright © 2023 Xu, Zhang, Tong, Ying and Liu. This is an open-access article distributed under the terms of the Creative Commons Attribution License (CC BY). The use, distribution or reproduction in other forums is permitted, provided the original author(s) and the copyright owner(s) are credited and that the original publication in this journal is cited, in accordance with accepted academic practice. No use, distribution or reproduction is permitted which does not comply with these terms.
*Correspondence: Ke-qi Liu, lkq2550598@126.com
†These authors have contributed equally to this work