- 1Institute of Microbiology, Heilongjiang Academy of Sciences, Harbin, China
- 2Key Laboratory of Flexible Electronics, Institute of Advanced Materials, Nanjing Tech University, Nanjing, China
Non-alcoholic fatty acid liver disease (NAFLD) is a reputed global health concern, affecting children and young adults. Accumulating evidence suggests that edible fungi polysaccharides have the potential to relieve NAFLD. Our previous study found that Auricularia cornea var. Li. polysaccharides (ACP) could improve immune by regulating gut microbiota. However, its NAFLD-alleviating potentials have been scarcely reported. This study analyzed the protective effects of Auricularia cornea var. Li. polysaccharides on high-fat diet (HFD)-induced NAFLD and mechanistic actions. We first analyzed the histology and hepatic lipid profile of animals to evaluate this variant’s ameliorating effects on NAFLD. Then, antioxidant and anti-inflammatory potentials of ACP were studied. Finally, we explored changes in the gut microbiome diversity for mechanistic insights from the gut-liver region. Results showed that supplementation with ACP substantially reduced homeostasis model assessment-insulin resistance (HOMA-IR), body fat, liver index rates and weight gain (p < 0.05). This variant also improved HDL-C levels while decreasing triglyceride (TG), total cholesterol (TC), and low-density lipoprotein cholesterol (LDL-C) levels which were initially triggered by HFD. ACP mediation also decreased the serum alanine aminotransferase (ALT) and aspartate aminotransferase (AST) levels considerably with H&E technique indicating that it can reduce liver lipid accumulation, thus lowering liver damages risks (p < 0.05). The antioxidant potentials of ACP were also demonstrated as it decreased the hepatic levels of malondialdehyde (MDA) and increased the activities of superoxide dismutase (SOD), catalase (CAT), and glutathione peroxidase (GSH-PX). Proinflammatory markers like IL-6, IL-1β and TNF-α concentrations were decreased by ACP supplementation, accompanied with increased IL-4 levels. Finally, ACP supplementation regulated the intestinal microbiota to near normal patterns. In all, ACP protects HFD-induced NAFLD by improving liver characteristics and regulating colonic flora composition, our findings assert that ACP can be a promising strategy in NAFLD therapy.
Introduction
Nonalcoholic fatty liver disease (NAFLD), is a highly pervasive liver condition globally (25.24% incidence rate), common among young adults and children (1). In China, it is only second to viral hepatitis in chronic liver disease causes (2). Although, the mechanisms regulating its pathogenesis is still unclear, NAFLD symptoms range from simple steatosis to severe inflammation, with the possibility of cirrhosis (3). Following the ‘multiple hit’ hypothesis, the gut microbiome could be linked to the onset and development of NAFLD (4). Current NAFLD treatments such as medicinal intervention and bariatric surgery have several side effects, including increased weight and osteoporosis (5), raising the need to explore alternative methods for NAFLD alleviation.
Notable biosafety and industrial importance have been identified in polysaccharides from edible fungi (6). Moreso, it has been reported previously that polysaccharides could have immunomodulatory and antioxidant activities. Findings suggested that Auricularia polytricha aqueous extract supplementation showed protective material against NAFLD by attenuating inflammatory response, oxidative stress and lipid deposition (7). It was found that polysaccharides from Auricularia auricula and Auricularia polytricha could inhibit oxidative stress, nuclear factor kappa-B (NF-κB) signaling and proinflammatory cytokine production (8). It has been reported that Auricularia auricula polysaccharides demonstrated the regulatory effects of endogenous metabolism and gut microbiota composition (9). The results of showed that the Auricularia auricula polysaccharide interventions had a potential to improve hyperlipidemia with the modulation of gut microbiota structure (10). Recent studies have shown that dietary supplementation of Auricularia auricula-judae polysaccharides could alleviate nutritional obesity in rats via regulating inflammatory response and lipid metabolism (11). It is thus possible that Auricularia polysaccharides could be useful in NAFLD prevention and treatment.
A genetically-stable variant of Auricularia cornea species known as Auricularia cornea var. Li could be produced in large amounts in China (12). Through several pathways, Wang et al. reported that this variant exerted hepatoprotective effects against the alcoholic liver diseases (13). Auricularia cornea var. Li. polysaccharides also has good antioxidant capacity, antinephritic effects, unique phenotype and potential medicinal properties (14). Our previous study found that Auricularia cornea var. Li. polysaccharides supplementation could improve immune by regulating gut microbiota (15). However, little is known about its NAFLD-alleviating potentials.
The objective of this work to analyze the protective effects of Auricularia cornea var. Li. polysaccharides on HFD-induced NAFLD and its related mechanism. The ameliorative effects of Auricularia cornea var. Li. polysaccharides on hepatic lipid profile and histological alterations, and inflammation cytokines in immunosuppressed rats were studied. In addition, the regulatory effect of Auricularia cornea var. Li. polysaccharides on the gut microbiota was analyzed. These findings will give further understanding regarding NAFLD pathogenesis and a framework exploring the possible application of Auricularia cornea var. Li. Polysaccharides in NAFLD therapy.
Materials and methods
Isolation and purification of Auricularia cornea var. Li. Polysaccharides
Auricularia cornea var. Li. was purchased from the edible fungus base in Heilongjiang province. The extraction methods of AAP are described by (16). Make a few changes. In short, first extract 20 g auriculae powder with 1 L deionized water at 90°C for 2 h. The total extract was concentrated to 1/3 of its original volume under reduced pressure and precipitated overnight with three-volume frozen 95% ethanol at 4°C. The protein content of precipitated solution was removed by Sevag method. The aqueous solution was dialyzed with deionized water for 3 days (7 kD), and the water was changed 3 times a day. Crude polysaccharides are obtained by concentration and freeze-drying. Purification was performed using anion exchange columns to separate the components of the crude polysaccharide, followed by further purification using gel columns and partial lyophilization of the final polysaccharide for further study.
Animals and experimental design
Thirty-six specific-pathogen-free (SPF) male Sprague–Dawley rats (160 ~ 180 g) were supplied by the Shanghai Slack Laboratory Animal Co. LTD [Shanghai, China, SCXK (Shanghai) 2012–0002]. All feeding was done in an animal room (22 ± 0.5°C and 55 ± 5%) with 12 h light/12 h dark cycles. Chow and water were made available ad libitum for the one-week acclimatization period. Afterward, rats were placed in three groups (n = 12 rats per group), including the control, HFD and Auricularia cornea var. Li. polysaccharides (ACP) groups. Rats in the control groups were fed with a regular diet throughout the experiment, while HFD group and ACP group fed with a HFD for 6 weeks. ACP (200 mg kg−1) solution was orally administrated to the rats in the ACP group, while equal volume of distilled water were administrated to the rats in the HFD group. Feed formulas are reported in Supplementary Table S1. Rats were humanely sacrificed after a 12 h fasting period. The blood was placed on ice for 2 h, and then the serum was obtained by centrifugation. The livers, epididymal adipose tissue and perirenal adipose tissue of rats were weighed and recorded, then stored at −80°C. The blood and small intestine were obtained for next experiments. The Ethics Committee of the First Affiliated Hospital of Heilongjiang University of Chinese Medicine approved all animal experiments and protocols (2022060801). Liver index and Body fat rate were calculated using the following formula:
Liver histopathological analysis
Referring to the method of (17) with slight modifications. We placed all liver tissues in paraffin wax and made thin slices 5 μm thick,and then stained with hematoxylin and eosin (H&E). Histology of these sections were observed using a light microscope (Nikon E100, 200 × magnification).
Determination of TC, TG, LDL-C, and HDL-C in the liver
The liver tissues were homogenized in aseptic PBS (1: 9, w/v), succeeded by centrifugation at 10,000 r/min for 15 min at 4°C. The concentration of TC, TG, HDL-C, and LDL-C was detected using Rats ELISA kits (Roche Diagnostics GmbH, Mannheim, German) based on the instructions of the manufacturer.
Serum ALT, AST, FBG, and FINs determination
Serum ALT, AST, fasting plasma glucose (FBG)and fasting insulin (FINs) levels were obtained using mouse kits (Conodi Creatures, Fujian, China) by following the manufacturer’s directives. HOMA-IR was calculated using the following formula:
Determination of the level of oxidative stress in the liver
Liver oxidative stress in the different groups were assessed by measuring malondialdehyde (MDA), superoxide dismutase (SOD), catalase (CAT), and glutathione peroxidase (GSH-PX) levels using commercial kits obtained from Jiancheng Creatures Co Ltd., (Nanjing, China).
Inflammatory cytokines measurement
Aseptic PBS (1:9 w/v) was used to homogenize liver tissues and centrifuged at 10,000 r/min for 10 min at 4°C. Afterward, the TNF-α, IL-6, IL-4 and IL-1β were detected using ELISA kits obtained from Conodi Creatures Co Ltd., (Fujian, China) by following the manufacturer’s instructions.
DNA extraction and 16S rRNA gene sequencing
Total bacterial genomic DNA was isolated from fecal samples using the Fast DNA SPIN extraction kits (MP Biomedicals, Santa Ana, CA, United States) according to the manufacturer’s instructions. 0.8% agarose gel electrophoresis was used to determine the DNA molecular size, and NanoDrop NC-2000 Spectrophotometer was used to quantify DNA. Afterwards, the bacterial 16S rRNA genes V3–V4 region was amplified by polymerase chain reaction (PCR) with the forward primer 338F (5’-ACTCCTACGGGAGGCAGCA-3′) and the reverse primer 806R (5’-GGACTACHVGGGTWTCTAAT-3′). PCR amplicons were purified with Agencourt AMPure Beads (Beckman Coulter, Indianapolis, IN) and quantified using the PicoGreen dsDNA Assay Kit (Invitrogen, Carlsbad, CA, United States). The TruSeq Nano DNA LT Library Prep Kit (Illumina, United States) was used for library construction. Sequencing was performed using the MiSeq Reagent Kit v3 (Shanghai Personal Biotechnology Co., Ltd.,Shanghai, China) on the Illumina MiSeq platform. Following data unloading, we sieved out low-quality reads (reads containing N bases, 3 ‘end adapter, reads with average quality value ≥20). Data obtained by pair-end sequencing was spliced into long tags using FastQ-Join software. Spliced tags were carefully filtered to obtain effective data (chimeras were also removed as described by (18)). Finally, the QIME software (v1.9.1) and UcLUST method were used to cluster OTU units (19) and align the representative sequence (20, 21).
Statistical analysis
All data were analyzed using SPSS 22.0 software (SPSS Inc., Chicago, IL, United States). Statistical analysis of Duncan’s multiple range tests after one-way analysis of variance (ANOVA). For all analyses, at p < 0.05, the differences were considered significant.
Results
Effects of ACP supplementation on body weight, liver index, body fat rate, and HOMA-IR of HFD-Fed rats
In Figure 1A, we observed that HFD substantially increased the final body weight, which was reversed in the ACP supplementation group. Liver index analysis results indicate that HFD induction gave a marked increase in the measured parameters which were reversed after ACP supplementation (Figure 1B). As expected, the HFD group had heightened body fat rates compared to the control group (p < 0.05), which was again ameliorated in the polysaccharide group (Figure 1C). The HFD group also showed increased HOMA-IR levels (p < 0.05) which was reversed to control group levels after ACP supplementation (Figure 1D). In conclusion, the addition of ACP normalized the increase in body weight, liver index, and body fat percentage in HFD-fed rats. At the same time, HOMA-IR was reduced.
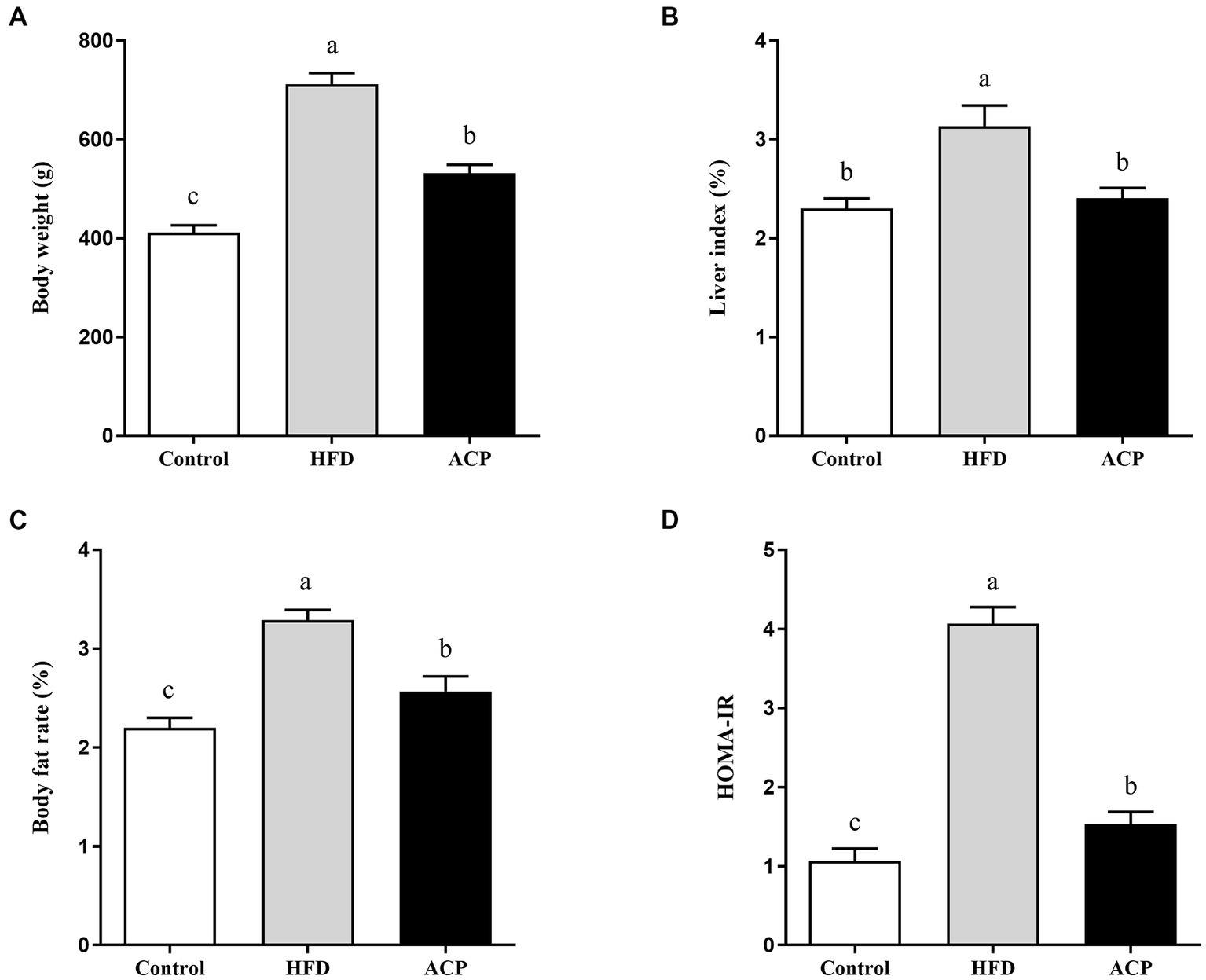
Figure 1. Effect of ACP supplementation on body weight (A), liver index (B), body fat rate (C) and (D) HOMA-IR of HFD-fed rats. Control, control group; HFD, high fat diet group; and ACP, A. cornea var. Li. polysaccharides group. Values are expressed as mean ± SD (n = 12). Different superscript letters indicate significant differences (p < 0.05).
Effects of ACP supplementation on lipid accumulation in the liver
We measured the liver TC, TG, LDL-C, and HDL-C using the ELISA kit. The HFD group showed elevated TG, TC, and LDL-C levels compared to the control group (p < 0.05) (Figures 2A–C). Moreover, the HDL-C levels were lowered (Figure 2D). Compared to the HFD group, LDL-C, TC and TG levels were significantly lower when ACP was administered (p < 0.05), while HDL-CS levels were significantly increased (p < 0.05), returning to similar levels in the control group.
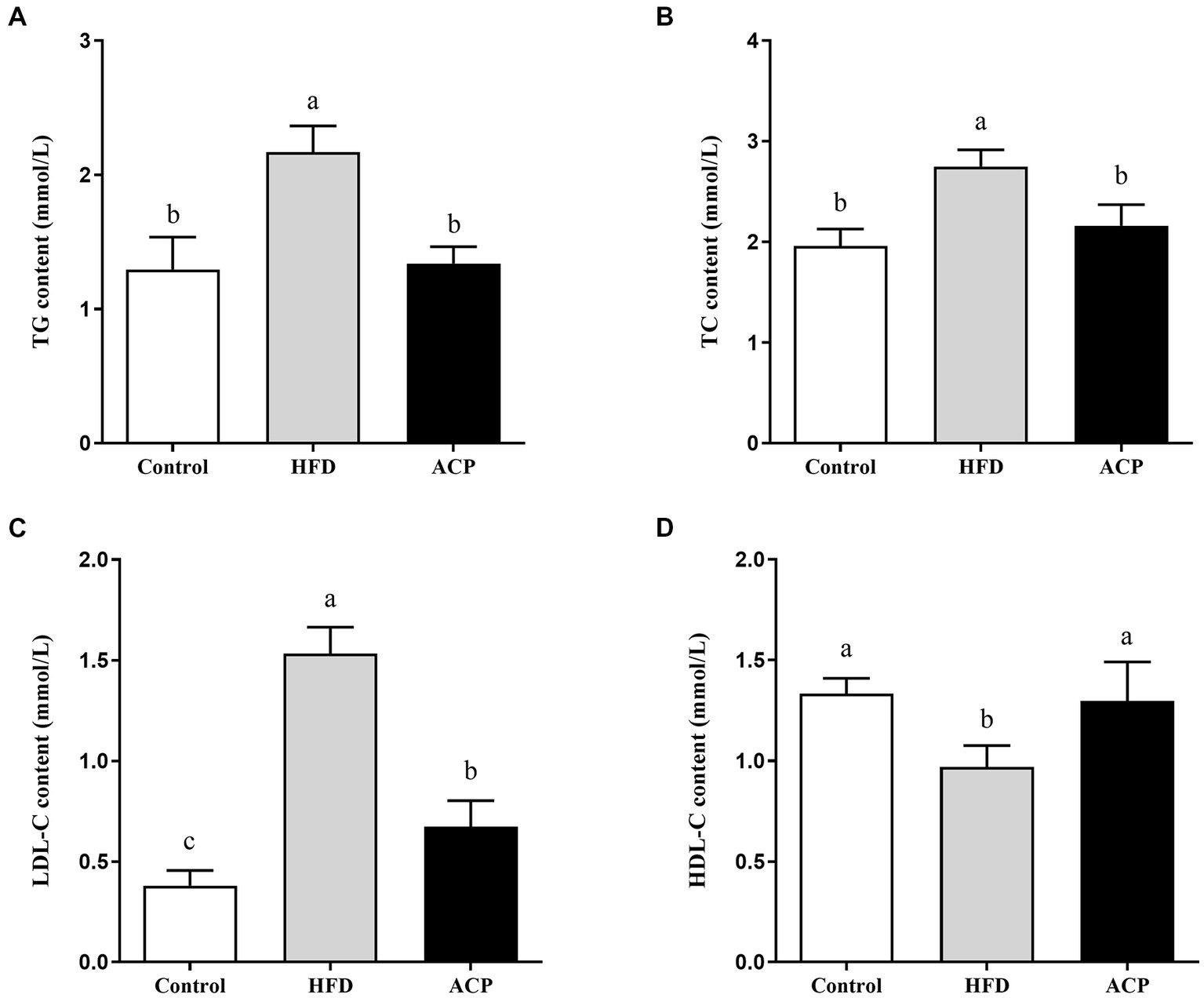
Figure 2. Effects of ACP supplementation on lipid accumulation in the liver in HFD-fed rats. Control, control group; HFD, high fat diet group; and ACP, A. cornea var. Li. polysaccharides group. (A) Hepatic triglyceride (TG) level. (B) Hepatic total cholesterol (TC) level. (C) Hepatic low density lipoprotein cholesterol (HDL-C) level. (D) Hepatic high density lipoprotein cholesterol (LDL-C) level. Values are expressed as mean ± SD (n = 12). Different superscript letters indicate significant differences (p < 0.05).
Effects of ACP supplementation on ALT and AST levels in the serum
We used the ELISA technique to assess liver function by evaluating the ALT and AST levels of rats. ALT and AST activities were significantly increased in the HFD group compared to the control group (p < 0.05) (Figures 3A,B). The increase in HFD-induced ALT and AST activity was significantly suppressed in the ACP supplemented group compared to the HFD group (p < 0.05).
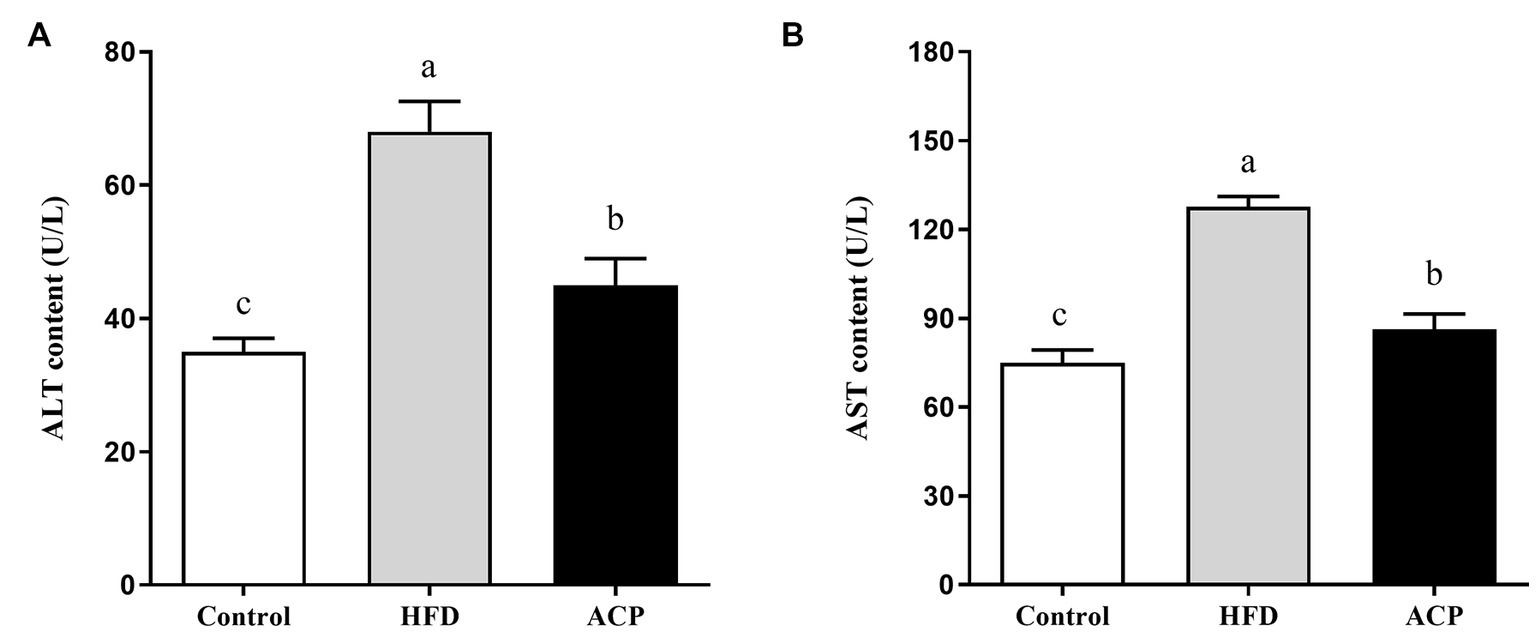
Figure 3. Effects of ACP supplementation on liver function. Control, control group; HFD, high fat diet group; and ACP, A. cornea var. Li. polysaccharides group. (A) Serum alanine aminotransferase (ALT) level. (B) Serum aspartate aminotransferase (AST) level. Values are expressed as mean ± SD (n = 12). Different superscript letters indicate significant differences (p < 0.05).
Effects of ACP supplementation on histological alterations of the liver tissues
As shown in Figure 4, in the Control group, the structure of the hepatic lobule was clear, the hepatic cords was arranged neatly, no obvious expansion or compression of the hepatic sinuses was observed, and no obvious inflammation was observed. A large number of hepatocyte balloon degeneration, cell swelling, nuclei centered, cytoplasmic vacuolation (black arrow), multiple focal lymphocyte infiltration (yellow arrow), rare hepatocyte necrosis, and nuclear fragmentation (red arrow) were found in the tissue of HFD-fed rats. However, administration of ACP was effective in reversing this HFD-induced trend, suggesting that ACP acts as a positive hepatoprotective agent.
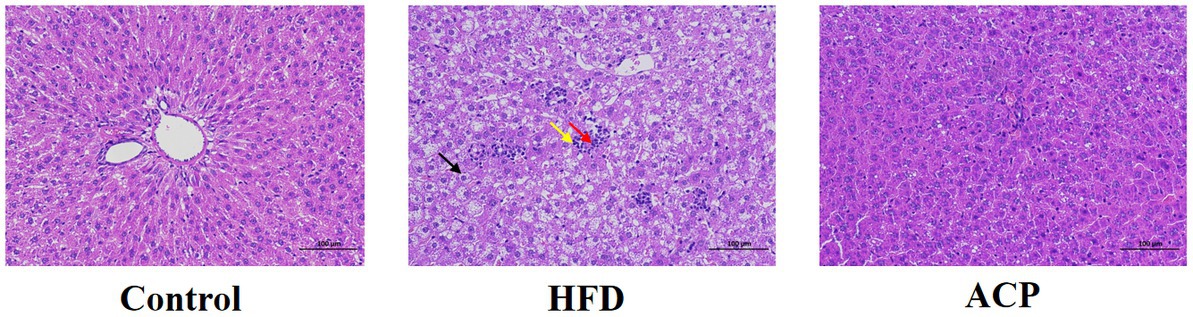
Figure 4. Effects of ACP supplementation on histological alterations of liver. Control, control group; HFD, high fat diet group; and ACP, A. cornea var. Li. polysaccharides group. Values are expressed as mean ± SD (n = 12). Different superscript letters indicate significant differences (p < 0.05).
Effects of ACP supplementation on the oxidative stress
In this study, we measured selected oxidative stress indicators to evaluate the effect of ACP supplementation on NAFLD. As presented in Figure 5, compared with the control group, the rats in the HFD group showed higher MDA levels (p < 0.05), with much reduced SOD, CAT, and GSH-PX in the liver tissues (p < 0.05). However, MDA levels were significantly lower in rats receiving ACP intervention compared to the HFD group (p < 0.05). Meanwhile, the levels of SOD, CAT, and GSH-PXs were significantly increased (p < 0.05). All these results suggest that ACP supplementation can effectively inhibit HFD-induced oxidative stress damage in NAFLD rats.
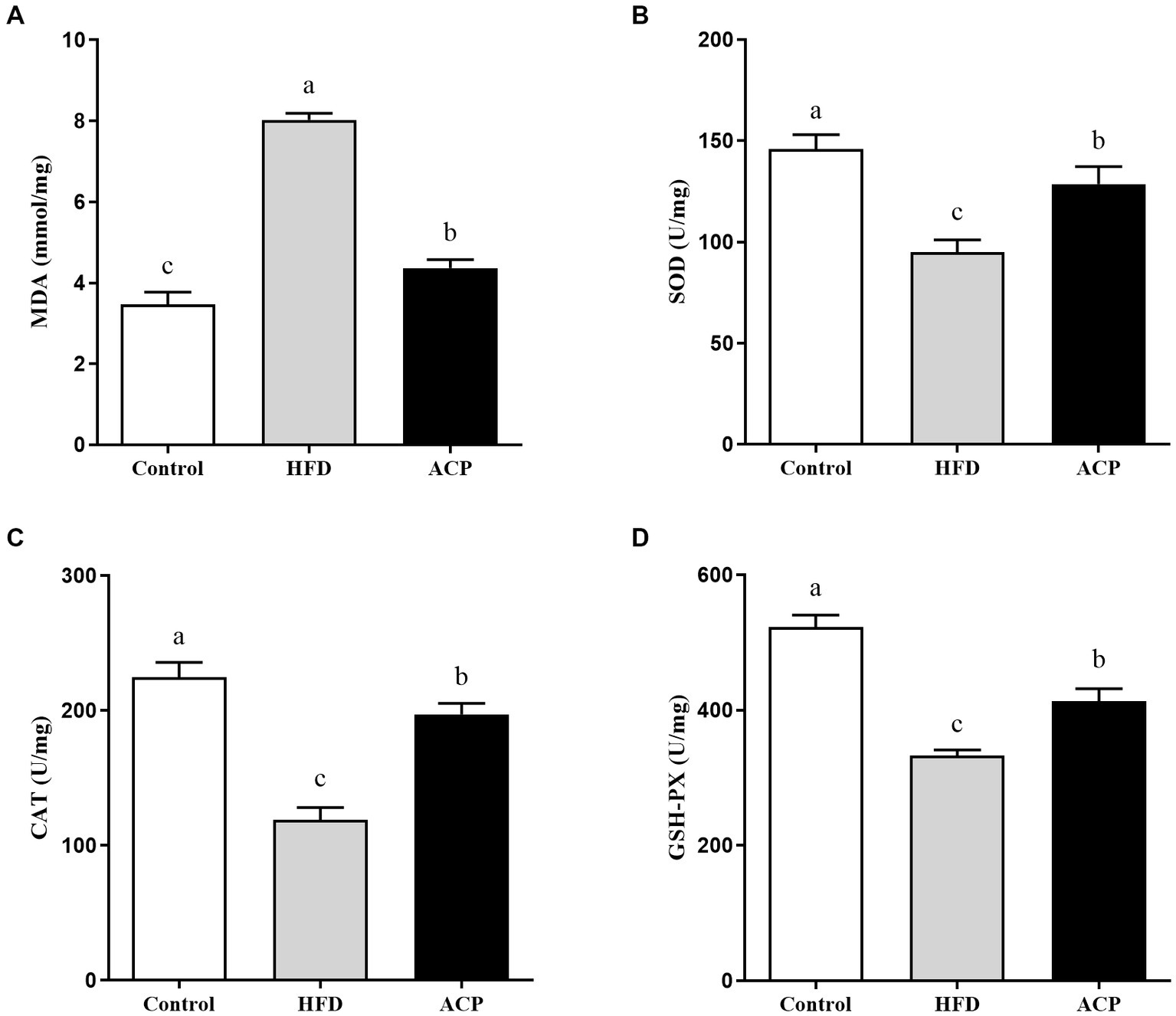
Figure 5. Effects of ACP supplementation on the oxidative stress. Control, control group; HFD, high fat diet group; and ACP, A. cornea var. Li. polysaccharides group. (A) MDA, (B) SOD, (C) CAT, and (D) GSH-PX. Values are expressed as mean ± SD (n = 12). Different superscript letters indicate significant differences (p < 0.05).
Effects of ACP supplementation on cytokine contents in the liver
Long-term use of HFD can cause persistent low-grade inflammation and contribute to NAFLD. Following analyses of selected cytokines, our results (Figure 6) showed that HFD rats exhibited heightened levels of IL-6, IL-1β and TNF-α concentrations compared to the control group (p < 0.05). Furthermore, IL-4 levels decreased. Compared with the HFD group, IL-6, IL-1β and TNF-α levels were significantly lower and IL-4 levels were significantly higher after ACP supplementation (p < 0.05), converging to the control group.
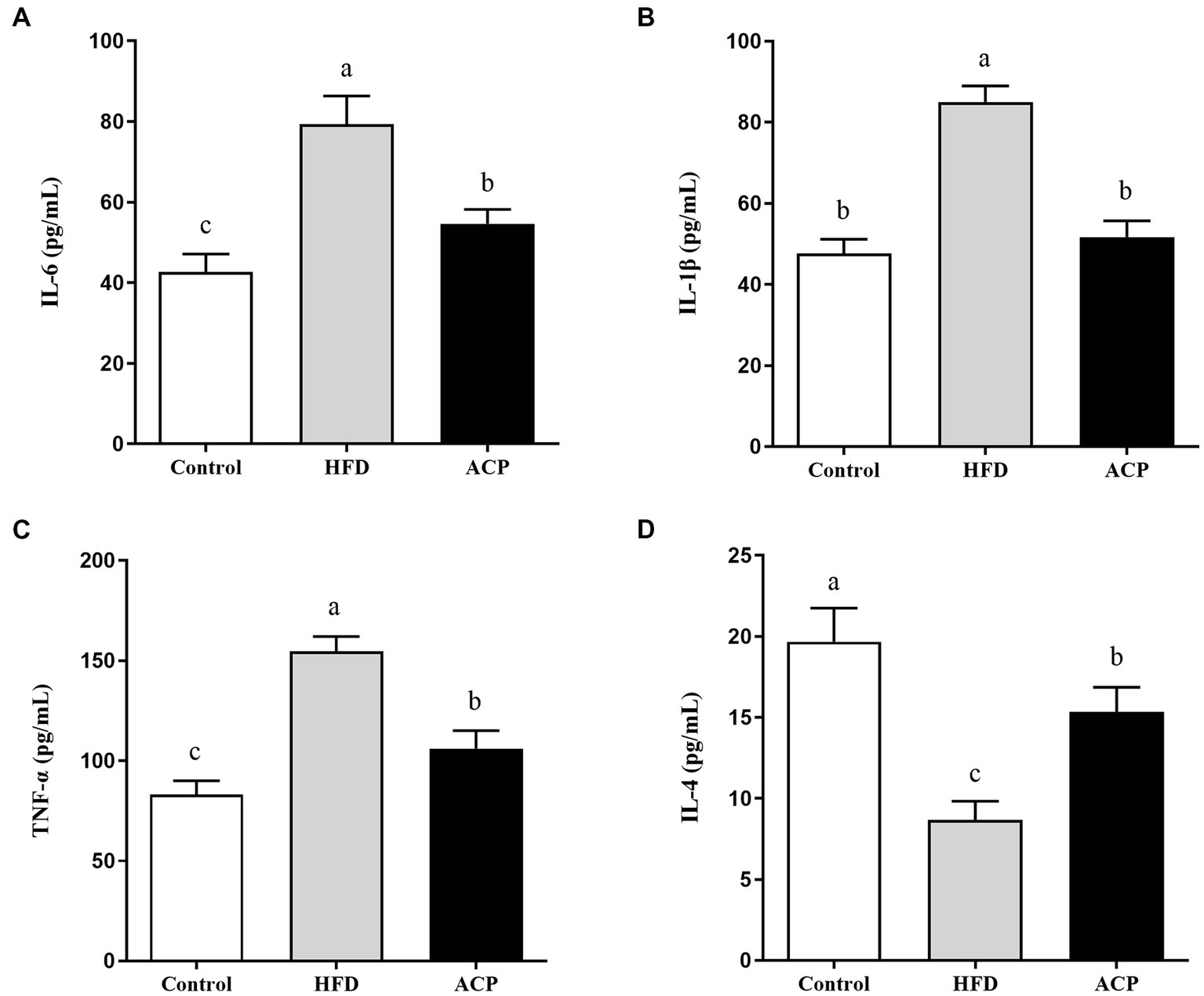
Figure 6. Effects of ACP supplementation on cytokine contents. Control, control group; HFD, high fat diet group; and ACP, A. cornea var. Li. polysaccharides group. (A) IL-6; (B) IL-1β; (C) TNF-α; and (D) IL-4. Values are expressed as mean ± SD (n = 12). Different superscript letters indicate significant differences (p < 0.05).
Effects of ACP supplementation on gut microbiota
To investigate whether mixed lactobacilli have an important role in the bacterial communities of NAFLD-induced rats, the gut microbiota of rats was analyzed by sequencing the 16S rDNA variable region V3–V4. The phylum levels of the gut microbiome samples are shown as Figure 7A. Firmicutes and Bacteroidota were the dominant phyla with a total abundance of more than 70%, followed by Proteobacteria and Deferribacteres. The relative abundance of Firmicutes was increased and the relative abundance of Bacteroidota were decreased in the HFD group. In reversing this trend, ACP supplementation lowered Firmicutes abundance level, raised Bacteroidetes diversity gave a decreased ratio of Firmicutes: Bacteroidetes. The genus levels were shown in Figure 7B. In the HFD group, the relative abundances of Bifidobacterium, Bacteroides, Odoribacter, Alloprevotella, Rikenellaceae RC9 gut group and Blautia were decreased compared with the control group. In contrast, the abundance of these genuses in the ACP supplementation were increased in the compare with the HFD group. Moreover, the relative abundances of Parabacteroides, Lachnoclostridium, Lachnospiraceae NK4A136 group and Roseburia were increased, which was decreased when compared to the HFD group.
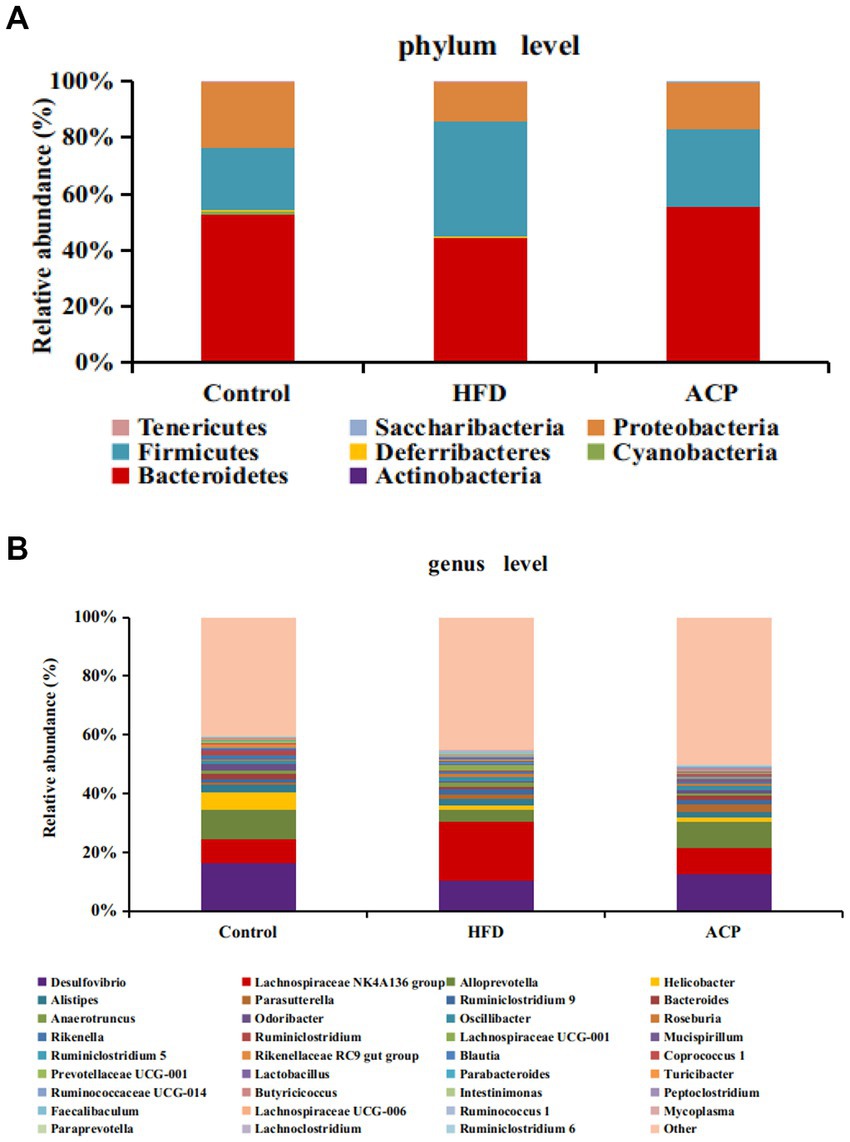
Figure 7. Changes of the gut microbiota at the phylum (A) and genus (B) level after ACP supplementation. Control, control group; HFD, high fat diet group; and ACP, A. cornea var. Li. polysaccharides group.
Discussion
The pervasive effects of NAFLD on a global scale is a public health concern that has warranted continued research into its mitigation (22). Using environment-friendly approaches such as edible fungus that secrete biologically-active compounds can be a promising technique for its therapy (23, 24). Our previous study found that polysaccharides supplementation with Auricularia cornea var. Li. (ACP) could improve immune by regulating gut microbiota (15), but its role in alleviating NAFLD has been poorly studied. The objective of this work to analyze the protective effects of Auricularia cornea var. Li. polysaccharides on HFD-induced NAFLD and its related mechanism. In this study, HFD significantly increased the body weight gain, liver index, body fat rate and HOMA-IR, but this was restored to normalcy by supplementation with ACP, in agreement with Naudrin et al. (25), who opined that supplementation with this polysaccharide could be protective against NAFLD induced by HFD. It is known that HFD-induced NAFLD is featured by increased TC, TG, and LDL levels and decreased HDL levels (26). Interestingly, it has also been reported that when these NAFLD biomarker levels are lowered, the condition is reversed (27, 28). We observed that the hepatic levels of TC, TG, and LDL and lowered the HDL levels were induced by HFD. This trend was reversed by supplementation with ACP, which was in line with the effects of polysaccharides from Enteromorpha prolifera in previous study (29). HFD also caused liver injury via significant fat accumulation, but this was lowered after ACP administration in NAFLD rats. Lipid deposition and toxicity levels which indicate inflammations and liver injury, are typically measured by serum AST and ALT levels (30). In our study, the HFD rats group should considerably high serum AST and ALT levels, which was reversed by ACP supplementation. These indicate that mediation with this polysaccharide could attenuate HFD-induced liver injury and protect liver function. The biomarkers used to measure liver oxidative stress (lipid peroxidation) include MDA, SOD, CAT and T-AOC. SOD breaks down superoxide anion, which is required as a catalyst in reducing O2▪− to H2O2, this is then converted to water by CAT (31). MDA can be a reliable in vivo biomarker of oxidative stress (32). Zhu et al. reported that chicory polysaccharide could improve the NAFLD in a rat model (33). Our results showed that ACP supplementation significantly decreased the hepatic levels of MDA and increased the activities of SOD, CAT, and GSH-PX, indicating that ACP supplementation could alleviate the NAFLD through elevating antioxidant capacity. In addition to immune response regulations, cytokines also play a role in repair of damaged tissues. Microbes in the intestine are known to directly or indirectly trigger immune responses and inflammations via cytokines like IL-6, IL-1β and TNF-α, with possible NAFLD-ameliorating effects (34). In this study, we observed that while IL-6, IL-1β and TNF-α levels were significantly raised by induced HFD conditions while IL-4 levels were lowered. This scenario was reversed by ACP mediation, suggesting that it could be strategic in NAFLD therapy via cytokine level regulation.
There is increasing evidence that NAFLD onset could be mitigated or aggravated by microbes in the gut (35). Our results showed that in the HFD group, the relative abundance of Bacteroidetes decreased substantially, noting an unusual increase in Firmicutes levels, aggreging with earlier studies that report high Firmicutes: Bacteroidetes ratio (36–38). The Firmicutes phylum have been reported to raise calories absorption which triggers obesity biomarkers with suppressed Bacteroidetes levels (39, 40). Following this scenario, ACP supplementation reversed this trend by raising Bacteroidetes abundance and also lowered the Firmicutes: Bacteroidetes ratio. Other reports demonstrated that the presence of Bifidobacterium (41), Bacteroides (42, 43), and Alloprevotella (44) were negatively correlated with obesity. As a non-pathogenic gut microbe, Bifidobacterium contributes to gut and intestinal health by secreting pathogen-inhibiting organic acids and lowers intestinal environment pH (45). Blautia is well-known butyrate producers, which is associated with a wide range of health benefits, including improved body composition and weight loss (46, 47). It has been reported that orally administered Odoribacter laneus could improve glucose control and inflammatory profile in obese rats by depleting circulating succinate (48). Alloprevotella belonging to the Bacteroidetes phylum has shown anti-inflammatory effects (49).
LPS is secreted by abundances of Parabacteroides (50, 51), Lachnoclostridium (52), Lachnospiraceae NK4A136 group (53) and Romboutsia (51), triggering proinflammatory cytokines, obesity, and insulin resistance. Again, organic acid-producing bacteria can be substantially suppressed by Lachnoclostridium, Lachnospiraceae NK4A136 group and Romboutsia from the Lachnospiraceae phylum (54). This has been correlated with cancers and metabolic syndrome (55, 56) but interestingly, this was normalized after ACP mediation. ACP further alleviates NAFLD by regulating the ecological imbalance of intestinal microbiota caused by a high-fat diet.
Conclusion
The current study showed that parameters like HOMA-IR, body fat rate, liver index, and body weight gain were significantly lowered by ACP supplementation. In addition, HDL levels were improved with a corresponding decrease in hepatic levels of TC, TG, and LDL and ALT and AST levels. ACP supplementation could alleviate oxidative stress by decreasing the hepatic levels of MDA and increasing the activities of SOD, CAT, and GSH-PX. Immunomodulatory biomarkers like IL-6, IL-1β, IL-4 and TNF-α concentrations were also significantly lowered. Interestingly, gut microbial biodiversity was restored, suggesting that this procedure could be promising in alleviating NAFLD symptoms.
Data availability statement
The datasets presented in this study can be found in online repositories. The names of the repository/repositories and accession number(s) can be found in the article/Supplementary material.
Ethics statement
The animal study was reviewed and approved by the Ethics Committee of the First Affiliated Hospital of Heilongjiang University of Chinese Medicine.
Author contributions
HX and XC designed the study. WJ and YP performed the experiments. TZ wrote the manuscript. XM and JH analyzed the data. All authors contributed to the article and approved the submitted version.
Funding
Present research work was financially supported by grants from the Natural Science Foundation of Heilongjiang Province (ZD2020C010).
Conflict of interest
The authors declare that the research was conducted in the absence of any commercial or financial relationships that could be construed as a potential conflict of interest.
Publisher’s note
All claims expressed in this article are solely those of the authors and do not necessarily represent those of their affiliated organizations, or those of the publisher, the editors and the reviewers. Any product that may be evaluated in this article, or claim that may be made by its manufacturer, is not guaranteed or endorsed by the publisher.
Supplementary material
The Supplementary material for this article can be found online at: https://www.frontiersin.org/articles/10.3389/fnut.2023.1161537/full#supplementary-material
References
1. Zhang, X, Wu, M, Liu, Z, Yuan, H, Wu, X, Shi, T, et al. Increasing prevalence of NAFLD/NASH among children, adolescents and young adults from 1990 to 2017: a population-based observational study. BMJ Open. (2021) 11:e042843. doi: 10.1136/bmjopen-2020-042843
2. Zhongjie, H, and Jing, Z. Current status of research on nonalcoholic fatty liver disease in China. J. Clin. Hepatol. (2016) 32:552–6. doi: 10.3969/j.issn.1001-5256.2016.03.034
3. Gong, Z, Tas, E, Yakar, S, and Muzumdar, R. Hepatic lipid metabolism and non-alcoholic fatty liver disease in aging. Mol Cell Endocrinol. (2017) 455:115–30. doi: 10.1016/j.mce.2016.12.022
4. Buzzetti, E, Pinzani, M, and Tsochatzis, EA. The multiple-hit pathogenesis of non-alcoholic fatty liver disease (NAFLD). Metabolism. (2016) 65:1038–48. doi: 10.1016/j.metabol.2015.12.012
5. Radaelli, M, Martucci, F, Perra, S, Accornero, S, Castoldi, G, Lattuada, G, et al. NAFLD/NASH in patients with type 2 diabetes and related treatment options. J Endocrinol Investig. (2018) 41:509–21. doi: 10.1007/s40618-017-0799-3
6. Yin, Z, Liang, Z, Li, C, Wang, J, Ma, C, and Kang, W. Immunomodulatory effects of polysaccharides from edible fungus: a review. Food Sci Human Wellness. (2021) 10:393–400. doi: 10.1016/j.fshw.2021.04.001
7. Chiu, W-C, Yang, H-H, Chiang, S-C, Chou, Y-X, and Yang, H-T. Auricularia polytricha aqueous extract supplementation decreases hepatic lipid accumulation and improves antioxidative status in animal model of nonalcoholic fatty liver. Biomedicine. (2014) 4:1–10. doi: 10.7603/s40681-014-0012-3
8. Xiang, H, Sun-Waterhouse, D, and Cui, C. Hypoglycemic polysaccharides from Auricularia auricula and Auricularia polytricha inhibit oxidative stress, NF-κB signaling and proinflammatory cytokine production in streptozotocin-induced diabetic mice. Food Sci Human Wellness. (2021) 10:87–93. doi: 10.1016/j.fshw.2020.06.001
9. Liu, Q, An, X, Chen, Y, Deng, Y, Niu, H, Ma, R, et al. Effects of Auricularia auricula polysaccharides on gut microbiota and metabolic phenotype in mice. Foods. (2022) 11:2700. doi: 10.3390/foods11172700
10. Zhang, T, Zhao, W, Xie, B, and Liu, H. Effects of Auricularia auricula and its polysaccharide on diet-induced hyperlipidemia rats by modulating gut microbiota. J Funct Foods. (2020) 72:104038. doi: 10.1016/j.jff.2020.104038
11. Liu, Q, Ma, R, Li, S, Fei, Y, Lei, J, Li, R, et al. Dietary supplementation of auricularia auricula-judae polysaccharides alleviate nutritional obesity in mice via regulating inflammatory response and lipid metabolism. Foods. (2022) 11:942. doi: 10.3390/foods11070942
12. Xiao, L, and Kuotan, Z. A review of the advances of the research on a white variant strain in genus Auricularia. Edible Med Mushrooms. (2016) 24:230–3.
13. Wang, X, Lan, Y, Zhu, Y, Li, S, Liu, M, Song, X, et al. Hepatoprotective effects of Auricularia cornea var. Li. Polysaccharides against the alcoholic liver diseases through different metabolic pathways. Sci Rep. (2018) 8:7574. doi: 10.1038/s41598-018-25830-w
14. Wang, D, Jiang, X, Teng, S, Zhang, Y, Liu, Y, Li, X, et al. The antidiabetic and antinephritic activities of Auricularia cornea (an albino mutant strain) via modulation of oxidative stress in the db/db mice. Front Immunol. (2019) 10:1039. doi: 10.3389/fimmu.2019.01039
15. Zhao, M, Shi, W, Chen, X, Liu, Y, Yang, Y, and Kong, X. Regulatory effects of Auricularia cornea var. Li. Polysaccharides on immune system and gut microbiota in cyclophosphamide-induced mice. Front Microbiol. (2022) 13:1056410. doi: 10.3389/fmicb.2022.1056410
16. Kong, X, Duan, W, Li, D, Tang, X, and Duan, Z. Effects of polysaccharides from Auricularia auricula on the immuno-stimulatory activity and gut microbiota in immunosuppressed mice induced by cyclophosphamide. Front Immunol. (2020) 11:595700. doi: 10.3389/fimmu.2020.595700
17. Wang, G, Jiao, T, Xu, Y, Li, D, Si, Q, Hao, J, et al. Bifidobacterium adolescentis and Lactobacillus rhamnosus alleviate non-alcoholic fatty liver disease induced by a high-fat, high-cholesterol diet through modulation of different gut microbiota-dependent pathways. Food Funct. (2020) 11:6115–27. doi: 10.1039/c9fo02905b
18. Edgar, RC. UPARSE: highly accurate OTU sequences from microbial amplicon reads. Nat Methods. (2013) 10:996–8. doi: 10.1038/nmeth.2604
19. Caporaso, JG, Kuczynski, J, Stombaugh, J, Bittinger, K, Bushman, FD, Costello, EK, et al. QIIME allows analysis of high-throughput community sequencing data. Nat Methods. (2010) 7:335–6. doi: 10.1038/nmeth.f.303
20. Desantis, TZ, Hugenholtz, P, Larsen, N, Rojas, M, Brodie, EL, Keller, K, et al. Greengenes, a chimera-checked 16S rRNA gene database and workbench compatible with ARB. Appl Environ Microbiol. (2006) 72:5069–72. doi: 10.1128/AEM.03006-05
21. Edgar, RC. Search and clustering orders of magnitude faster than BLAST. Bioinformatics. (2010) 26:2460–1. doi: 10.1093/bioinformatics/btq461
22. Zhong, M, Yan, Y, Yuan, H, Rong, A, Xu, G, Cai, F, et al. Astragalus mongholicus polysaccharides ameliorate hepatic lipid accumulation and inflammation as well as modulate gut microbiota in NAFLD rats. Food Funct. (2022) 13:7287–301. doi: 10.1039/D2FO01009G
23. Liang, J, Zhang, M, Wang, X, Ren, Y, Yue, T, Wang, Z, et al. Edible fungal polysaccharides, the gut microbiota, and host health. Carbohydr Polym. (2021) 273:118558. doi: 10.1016/j.carbpol.2021.118558
24. Yin, C, Noratto, GD, Fan, X, Chen, Z, Yao, F, Shi, D, et al. The impact of mushroom polysaccharides on gut microbiota and its beneficial effects to host: a review. Carbohydr Polym. (2020) 250:116942. doi: 10.1016/j.carbpol.2020.116942
25. Naudin, CR, Maner-Smith, K, Owens, JA, Wynn, GM, Robinson, BS, Matthews, JD, et al. Lactococcus lactis subspecies cremoris elicits protection against metabolic changes induced by a western-style diet. Gastroenterology. (2020) 159:639–651.e5. doi: 10.1053/j.gastro.2020.03.010
26. Nabavi, S, Rafraf, M, Somi, M, Homayouni-Rad, A, and Asghari-Jafarabadi, M. Effects of probiotic yogurt consumption on metabolic factors in individuals with nonalcoholic fatty liver disease. J Dairy Sci. (2014) 97:7386–93. doi: 10.3168/jds.2014-8500
27. Ye, H, Li, Q, Zhang, Z, Sun, M, Zhao, C, and Zhang, T. Effect of a novel potential probiotic Lactobacillus paracasei Jlus66 isolated from fermented milk on nonalcoholic fatty liver in rats. Food Funct. (2017) 8:4539–46. doi: 10.1039/C7FO01108C
28. Zhao, Z, Wang, C, Zhang, L, Zhao, Y, Duan, C, Zhang, X, et al. Lactobacillus plantarum NA136 improves the non-alcoholic fatty liver disease by modulating the AMPK/Nrf2 pathway. Appl Microbiol Biotechnol. (2019) 103:5843–50. doi: 10.1007/s00253-019-09703-4
29. Ren, R, Gong, J, Zhao, Y, Zhuang, X, Ye, Y, and Lin, W. Sulfated polysaccharides from Enteromorpha prolifera suppress SREBP-2 and HMG-CoA reductase expression and attenuate non-alcoholic fatty liver disease induced by a high-fat diet. Food Funct. (2017) 8:1899–904. doi: 10.1039/C7FO00103G
30. Zhao, L, Jiang, Y, Ni, Y, Zhang, T, Duan, C, Huang, C, et al. Protective effects of Lactobacillus plantarum C88 on chronic ethanol-induced liver injury in mice. J Funct Foods. (2017) 35:97–104. doi: 10.1016/j.jff.2017.05.017
31. Li, Y-N, Guo, Y, Xi, M-M, Yang, P, Zhou, X-Y, Yin, S, et al. Saponins from Aralia taibaiensis attenuate D-galactose-induced aging in rats by activating FOXO3a and Nrf2 pathways. Oxidative Med Cell Longev. (2014). doi: 10.1155/2014/320513
32. Mateos, R, Lecumberri, E, Ramos, S, Goya, L, and Bravo, L. Determination of malondialdehyde (MDA) by high-performance liquid chromatography in serum and liver as a biomarker for oxidative stress: application to a rat model for hypercholesterolemia and evaluation of the effect of diets rich in phenolic antioxidants from fruits. J Chromatogr B. (2005) 827:76–82. doi: 10.1016/j.jchromb.2005.06.035
33. Zhu, H, Wang, Z, Wu, Y, Jiang, H, Zhou, F, Xie, X, et al. Untargeted metabonomics reveals intervention effects of chicory polysaccharide in a rat model of non-alcoholic fatty liver disease. Int J Biol Macromol. (2019) 128:363–75. doi: 10.1016/j.ijbiomac.2019.01.141
34. Decicco, LA, Rikans, LE, Tutor, CG, and Hornbrook, KR. Serum and liver concentrations of tumor necrosis factor α and interleukin-1β following administration of carbon tetrachloride to male rats. Toxicol Lett. (1998) 98:115–21. doi: 10.1016/S0378-4274(98)00110-6
35. Walker, AW, and Julian, P. Microbiology. Fighting obesity with bacteria. Science. (2013) 341:1069–70. doi: 10.1126/science.1243787
36. Chang, C-J, Lin, C-S, Lu, C-C, Martel, J, Ko, Y-F, Ojcius, DM, et al. Ganoderma lucidum reduces obesity in mice by modulating the composition of the gut microbiota. Nat Commun. (2015) 6:7489. doi: 10.1038/ncomms8489
37. Ilseung, C, Shingo, Y, Laura, C, Methé, BA, Jiri, Z, Kelvin, L, et al. Antibiotics in early life alter the murine colonic microbiome and adiposity. Nature. (2012) 488:621–6. doi: 10.1038/nature11400
38. Zhang, X, Zhang, M, Ho, C-T, Guo, X, Wu, Z, Weng, P, et al. Metagenomics analysis of gut microbiota modulatory effect of green tea polyphenols by high fat diet-induced obesity mice model. J Funct Foods. (2018) 46:268–77. doi: 10.1016/j.jff.2018.05.003
39. Komaroff, AL. The microbiome and risk for obesity and diabetes. JAMA. (2017) 317:355–6. doi: 10.1001/jama.2016.20099
40. Turnbaugh, PJ, Ley, RE, Mahowald, MA, Vincent, M, Mardis, ER, and Gordon, JI. An obesity-associated gut microbiome with increased capacity for energy harvest. Nature. (2006) 444:1027–31. doi: 10.1038/nature05414
41. Zhu, Z, Zhu, B, Sun, Y, Ai, C, Wang, L, Wen, C, et al. Sulfated polysaccharide from sea cucumber and its depolymerized derivative prevent obesity in association with modification of gut microbiota in high-fat diet-fed mice. Mol Nutr Food Res. (2018) 62:1800446. doi: 10.1002/mnfr.201800446
42. Zhang, X, Zhao, Y, Xu, J, Xue, Z, Zhang, M, Pang, X, et al. Modulation of gut microbiota by berberine and metformin during the treatment of high-fat diet-induced obesity in rats. Sci Rep. (2015) 5:14405. doi: 10.1038/srep14405
43. Martinez, KA 2nd, Devlin, JC, Lacher, CR, Yin, Y, Cai, Y, Wang, J, et al. Increased weight gain by C-section: functional significance of the primordial microbiome. Sci Adv. (2017) 3:eaao1874. doi: 10.1126/sciadv.aao1874
44. Wang, J, Wang, P, Li, D, Hu, X, and Chen, F. Beneficial effects of ginger on prevention of obesity through modulation of gut microbiota in mice. Eur J Nutr. (2019) 59:1–20. doi: 10.1007/s00394-019-01938-1
45. Wang, R, Li, H, Yang, X, Xue, X, Deng, L, Shen, J, et al. Genetically obese human gut microbiota induces liver steatosis in germ-free mice fed on normal diet. Front Microbiol. (2018) 9:1602. doi: 10.3389/fmicb.2018.01602
46. Byrne, C, Chambers, E, Morrison, D, and Frost, G. The role of short chain fatty acids in appetite regulation and energy homeostasis. Int J Obes. (2015) 39:1331–8. doi: 10.1038/ijo.2015.84
47. Schwiertz, A, Taras, D, Schäfer, K, Beijer, S, Bos, NA, Donus, C, et al. Microbiota and SCFA in lean and overweight healthy subjects. Obesity. (2010) 18:190–5. doi: 10.1038/oby.2009.167
48. Huber-Ruano, I, Calvo, E, Mayneris-Perxachs, J, Rodríguez-Peña, M-M, Ceperuelo-Mallafré, V, Cedó, L, et al. Orally administered Odoribacter laneus improves glucose control and inflammatory profile in obese mice by depleting circulating succinate. Microbiome. (2022) 10:135. doi: 10.1186/s40168-022-01306-y
49. Ning, K, Lu, K, Chen, Q, Guo, Z, Du, X, Riaz, F, et al. Epigallocatechin Gallate protects mice against methionine–choline-deficient-diet-induced nonalcoholic Steatohepatitis by improving gut microbiota to attenuate hepatic injury and regulate metabolism. ACS Omega. (2020) 5:20800–9. doi: 10.1021/acsomega.0c01689
50. Lieber, AD, Beier, UH, Xiao, H, Wilkins, BJ, Jiao, J, Li, XS, et al. Loss of HDAC6 alters gut microbiota and worsens obesity. FASEB J. (2018) 33:1098–109. doi: 10.1096/fj.201701586R
51. Zhao, L, Chen, Y, Xia, F, Abudukerimu, B, Zhang, W, Guo, Y, et al. A glucagon-like Peptide-1 receptor agonist lowers weight by modulating the structure of gut microbiota. Front Endocrinol. (2018) 9:233. doi: 10.3389/fendo.2018.00233
52. Zhao, L, Zhang, Q, Ma, W, Tian, F, Shen, H, and Zhou, M. A combination of quercetin and resveratrol reduces obesity in high-fat diet-fed rats by modulation of gut microbiota. Food Funct. (2017) 8:4644–56. doi: 10.1039/C7FO01383C
53. Kang, Y, Li, Y, Du, Y, Guo, L, Chen, M, Huang, X, et al. Konjaku flour reduces obesity in mice by modulating the composition of the gut microbiota. Int J Obes. (2019) 43:1631–43. doi: 10.1038/s41366-018-0187-x
54. Duncan, SH, Adela, B, Stewart, CS, Pryde, SE, and Flint, HJ. Acetate utilization and butyryl coenzyme a (CoA):acetate-CoA transferase in butyrate-producing bacteria from the human large intestine. Appl Environ Microbiol. (2002) 68:5186–90. doi: 10.1128/AEM.68.10.5186-5190.2002
55. Keishi, K, and Kikuji, I. Intestinal colonization by a Lachnospiraceae bacterium contributes to the development of diabetes in obese mice. Microbes Environ. (2014) 29:427–30. doi: 10.1264/jsme2.ME14054
Keywords: Auricularia cornea var. Li., polysaccharides, high-fat diet, non-alcoholic fatty liver disease, gut microbiota
Citation: Zhou T, Mao X, Jiang W, Pan Y, Chen X, Hu J, Kong X and Xia H (2023) Assessment of Auricularia cornea var. Li. polysaccharides potential to improve hepatic, antioxidation and intestinal microecology in rats with non-alcoholic fatty liver disease. Front. Nutr. 10:1161537. doi: 10.3389/fnut.2023.1161537
Edited by:
Daxi Ren, Zhejiang University, ChinaReviewed by:
Lidong Guo, Heilongjiang University of Chinese Medicine, ChinaSong Wang, Northeast Agricultural University, China
Copyright © 2023 Zhou, Mao, Jiang, Pan, Chen, Hu, Kong and Xia. This is an open-access article distributed under the terms of the Creative Commons Attribution License (CC BY). The use, distribution or reproduction in other forums is permitted, provided the original author(s) and the copyright owner(s) are credited and that the original publication in this journal is cited, in accordance with accepted academic practice. No use, distribution or reproduction is permitted which does not comply with these terms.
*Correspondence: Xianghui Kong, a3hoMjlAMTI2LmNvbQ==; Haihua Xia, aGFpaHVheGlhQHNpbmEuY29t