- 1Division of Neonatology, Department of Pediatrics, University of Alabama at Birmingham, Birmingham, AL, United States
- 2Division of Pulmonary and Critical Care Medicine, Department of Medicine, University of Alabama at Birmingham, Birmingham, AL, United States
- 3Program in Protease and Matrix Biology, University of Alabama at Birmingham, Birmingham, AL, United States
- 4Atlantia Clinical Trials, Cork, Ireland
Introduction: Dysbiosis of the gut microbiome may augment lung disease via the gut-lung axis. Proteobacteria may contribute to tissue proteolysis followed by neutrophil recruitment, lung tissue injury, and perpetuation of chronic inflammation. To study the effects of probiotics across the gut-lung axis, we sought to determine if a Lactobacillus probiotic and herbal blend was safe and well-tolerated in healthy volunteers and asthmatic patients.
Methods: We conducted a 1-month randomized, open-label clinical trial in Cork, Ireland with healthy and asthmatic patients who took the blend twice a day. The primary endpoint was safety with exploratory endpoints including quality of life, lung function, gut microbiome ecology, and inflammatory biomarkers.
Results: All subjects tolerated the blend without adverse events. Asthmatic subjects who took the blend showed significant improvements in lung function as measured by forced expiratory volume and serum short chain fatty acid levels from baseline to Week 4. The gut microbiome of asthmatic subjects differed significantly from controls, with the most prominent difference in the relative abundance of the proteobacteria Escherichia coli. Administration of the probiotic maintained overall microbial community architecture with the only significant difference being an increase in absolute abundance of the probiotic strains measured by strain-specific PCR.
Conclusion: This study supports the safety and efficacy potential of a Lactobacillus probiotic plus herbal blend to act on the gut-lung axis. However, due to the lack of a control group, a longer blinded, placebo-controlled study will be warranted to confirm the efficacy improvements observed in this trial.
Clinical trial registration: https://clinicaltrials.gov/, identifier NCT05173168.
1. Introduction
The gut-lung axis serves as a powerful means of communication between the microbiome of the gut and the inflammatory and immune microenvironment of the lungs. Patients with respiratory diseases often have gastrointestinal symptoms and show distinct imbalanced gut microbiomes compared to healthy individuals (1–5). Inhaled exposure to environmental toxins such as cigarette smoke and pollution can decrease bacterial diversity and increase inflammation in the gut (6, 7). As such, the state of the intestinal microbiota is deeply connected to lung health and vice versa.
Supplementation with probiotic lactic-acid-producing bacteria has been widely studied. Live Lactobacillus strains have been individually clinically studied for supporting gut and lung health in improving and preventing infections (8, 9). Commensal species L. rhamnosus, L. plantarum, and L. acidophilus support maintaining proper uptake of short chain fatty acids (SCFAs) in both healthy and diseased populations (10–12). SCFAs and other metabolites produced by commensal bacteria travel through systemic circulation and mediate inflammatory and immune responses in distal organs (13). In a double-blind, randomized controlled trial of asthmatic patients, a Lactobacillus blend taken once a day for 8 weeks showed immunomodulatory effects via improvement in Th2 cells-associated IL-4 and lung function via forced expiratory volume and forced vital capacity (14). Other clinical studies of probiotics, primarily conducted in children, show improvements in asthma severity, allergic response, and immune biomarkers (15–18). Adequate SCFA production is also associated with reduced allergic airway inflammation (19, 20).
Bioactive compounds in herbal extracts can further support the anti-inflammatory action of live probiotic strains. Herbal extracts of holy basil leaf, turmeric root, and vasaka leaf can be taken orally to support antioxidant, antitussive, anti-inflammatory, and bronchodilatory effects in the lungs and systemically (21–23). A blend of live Lactobacillus strains with holy basil, turmeric, and vasaka extracts, decreased matrix metalloproteinase 9 (MMP-9) pathway activity, neutrophil recruitment, and pro-inflammatory biomarkers in preclinical in vitro and in vivo models of lung inflammation (24).
Our group has focused on attenuating inflammation and supporting lung function through the gut-lung axis using oral probiotic supplementation. In this clinical study, we hypothesized that a preclinically studied (24) Lactobacillus probiotic and herbal blend would demonstrate safety and preliminary biomarker and clinical improvements in healthy and asthmatic populations in a 1-month course of dosing.
2. Materials and methods
2.1. Trial design
The study was conducted in accordance with the Declaration of Helsinki and approved by the Clinical Research Ethics Committee of the Cork Teaching Hospitals of University College Cork [ECM 4 (n) 7/9/2021 and ECM 5 (7) 10/26/2021 and ECM 3 (lll) 11/16/2021].
This study was an open-label, exploratory pilot study to assess the safety of 4 weeks of dosing a probiotic and herbal blend (resB Lung Support, ResBiotic Nutrition) in healthy and asthmatic participants. Forty participants were screened to identify 22 eligible subjects. The study population consisted of n = 11 healthy participants and n = 11 participants diagnosed with asthma. Within these populations, active smoking status was noted (healthy smokers n = 3, asthmatic smokers n = 4). Informed consent was obtained from all subjects involved in the study. First patient first visit was November 23, 2021, and last patient last visit was January 20, 2022.
The study protocol consisted of four onsite visits over a 6-week period. Participants were pre-screened with an online questionnaire and invited for an onsite screening visit (Visit 1) to confirm their eligibility. A urine drug test was conducted and a urine test for pregnancy was performed for individuals of childbearing potential. No changes were made to the methods after the trial started.
2.1.1. Participants
Male and female participants ages 18–65 were recruited who were either in general good health at the discretion of the investigator or had asthma and were on stable medication for at least 3 months. Exclusion criteria included pregnancy, acute or chronic illness which by the investigator’s judgment precluded them from participating in the study, ≤2 hospital admissions in the past 6 months, and use of antibiotics, probiotics, immunosuppressive medications, or oral steroids (>10 mg/day) for >3 days in the previous 12 weeks. Participants were also excluded if they had made any major dietary changes or changed medications or supplements in the 30 days prior to enrollment.
Participants were enrolled and conducted site visits at a single site: Atlantia Food Trials, Heron House Office, Blackpool Retail Park, in Cork, Ireland.
2.1.2. Interventions
All 22 participants took the supplement twice daily for 4 weeks (28 days). One capsule contains 8.25 × 109 CFU Lactobacillus plantarum RSB11®, 7.9×109 CFU Lactobacillus acidophilus RSB12®, 6.4 × 109 CFU Lactobacillus rhamnosus RSB13®, 48.0 mg vasaka (Adhatoda vasica root) extract, 42.0 mg holy basil (Ocimum sanctum leaf) extract, and 30.0 mg turmeric (Curcuma longa root) extract. Total CFU count at the time of the last patient out was 2.6 × 1010 CFU/capsule. At Visit 1, participants were provided with a 30-day supply plus 2 days of overage. Study product was stored refrigerated on-site, and participants were instructed to store it refrigerated at home as well. Supplementation started after completion of Visit 2, where participants were instructed to consume one capsule twice daily with food and water at home. At Visits 3 and 4, participants returned all unused investigational product so that compliance could be calculated. Participants had to consume at least 75% (45 capsules) of their supply to be deemed compliant.
2.1.3. Outcomes
The primary objective of this trial was to measure safety of the blend in healthy and asthmatic study participants. Safety was measured by number of participants experiencing at least one adverse event (AE); number of AEs including causality, severity, and seriousness assessments; number of participants with discontinuations due to AEs; change in systolic blood pressure, diastolic blood pressure, heart rate, and body temperature from baseline to Week 2 and Week 4; change in blood safety parameters via serum chemistry profile and hematology profile from baseline to Week 2 and Week 4.
Exploratory objectives for this trial included: change in gut microbiota (16 s) sequencing from baseline to Week 4; change in lung function measured by spirometry [Forced Expired Volume in 1 s (FEV1) and Forced Vital Capacity (FVC)] from baseline to Week 4; change in oxygen levels (% pulse oxygen levels) from baseline to Week 4; change in SGRQ score from baseline to Week 4.
At Baseline and Week 4, participants had blood drawn for biomarker analysis, spirometry measured, and completed the Saint George’s Respiratory Questionnaire (SGRQ). Participants supplied a stool sample at Visit 2 and Visit 4. Vitals, safety blood parameters, and adverse events/severe adverse events (AE/SAE) were monitored at each visit. Participants completed an end-of-study product questionnaire at Visit 4. There were no changes to the study protocol after the study initiated.
2.1.4. Blinding
This was an open label study where study personnel and the participants were aware of the product they were taking; blinding did not apply.
2.1.5. Statistical methods
The primary method of analysis was descriptive statistics. Summary statistics for continuous measures were provided for the actual measurements at each visit and change from baseline to each visit. These tables included sample size, minimum and maximum statistics, mean, median, quartiles, and standard deviations. Key variables were categorized into clinical ranges. In the summary tables, counts and percentages were used in the frequency tables.
Inferential statistics were run to provide information on the trends within the data. D’Agostino and Pearson test of normality was run to determine if the data was normally distributed. Post-hoc paired t-tests were conducted to assess within group changes from baseline to Week 4 in the healthy and asthmatic populations separately. Participants were further segmented into smokers vs. non-smokers in assessing the changes from baseline to Week 4 in serum SCFA using paired t-tests. All analyses requiring significance testing were two-sided at a 5% significance level. Results were viewed as statistically significant if the value of p was less than or equal to 0.05.
2.2. Microbiome amplicon sequencing and analysis
Microbial DNA was extracted from human stool samples and 16S amplicon sequencing was performed using the Illumina MiSeq platform at the University of Alabama at Birmingham Microbiome Resource Core Facility under the direction of Dr. Casey Morrow. Sequencing data quality control, alignment and demultiplexing were perform by the using a custom script built using MOTHUR and QIIME 2 with amplicon sequence variants (ASVs) identified using SILVA. Processed ASV tables were imported into MicrobiomeAnalyst for further analysis and data visualization (25, 26). ASVs that appeared in less than two samples, were prevalent in 10% of samples and less than 5% of the inter quartile range were removed, leading to the removal of 504 low abundance features. Cumulative sum scaling was performed but not rarefication or transformation. Alpha diversity was quantified with the Shannon and Chao1 Indices. We visualized beta diversity with principal coordinates analysis of Bray–Curtis dissimilarity matrices and performed significance testing using permutational multivariate analysis of variance (PERMANOVA) and permutational multivariate analysis of dispersion (PERMDISP). Feature selection was performed using MetagenomeSeq and DESeq2.
2.3. Qualitative PCR
DNA was re-extracted from same fecal material used in MiSeq with Zymobiomics DNA Mini-Prep kit (Zymo Research). PCR (Qiagen Fast Cycling PCR kit) was performed on samples with at least 3 ng/μL total DNA by pico green quantification (Quant-It dsDNA Assay Kit, ThermoFisher). A common 16S gene-based forward primer was utilized with strain-specific reverse primers at a similar site (0.4 μM), in a 25 μL reaction with 30 ng DNA template, using SYBR Green dye (LTi) to monitor in real time. Analysis of PCR reactions was performed on 2% agarose gels. Densitometry (% and ng values) was calculated from the imaged PCR gels using a GS-900 densitometer and Image Lab software (Version 6.1, Bio-Rad). Bands were gel extracted (Qiagen Qiaquick Gel Extaction Kit) and sequenced to confirm species identity. ZymoBiomics Gut Microbiome Standard (negative control) was used to demonstrate lack of nonspecific amplification.
2.4. Biomarker analysis
Serum SCFA levels were measured by GC–MS at Creative Proteomics (Shirley, NY). The following SCFAs were analyzed: acetic acid (C2:0), propionic acid (C3:0), butyric acid (C4:0), isobutyric acid (C4:0i), valeric acid (C5:0); isovaleric acid (C5:0i), hexanoic acid (C6:0). Free short chain fatty acids were derivatized using methyl chloroformate in 1-propanol yielding propyl esters before subsequent liquid–liquid extraction into hexane and analysis on a SLB-5 ms (30 × 0.25 × 1.0 μm) (Supelco) column and detection using GC-EI-MS in SIM-mode. Instrumental analysis was performed on an Agilent 7890 GC coupled to an Agilent 5977 MSD (Agilent Technologies). Quantification was performed against a 5-point calibration curve.
3. Results
3.1. Oral supplementation with probiotic blend is safe for human consumption
Forty total participants were screened to randomize 22 participants with asthma (n = 11) or were healthy (n = 11) who met study eligibility criteria (Table 1 and Figure 1). Smoking status was noted (healthy smokers n = 3, asthmatic smokers n = 4) because of its well-documented impact on lung health and susceptibility to disease. Three asthmatic participants also reported having a history of allergic rhinitis. Participants who met the eligibility criteria and successfully completed the Screening Visit were enrolled in the trial. Subjects took the probiotic blend twice a day for 4 weeks, and assessments were conducted at Baseline and the end of Week 2 and Week 4.
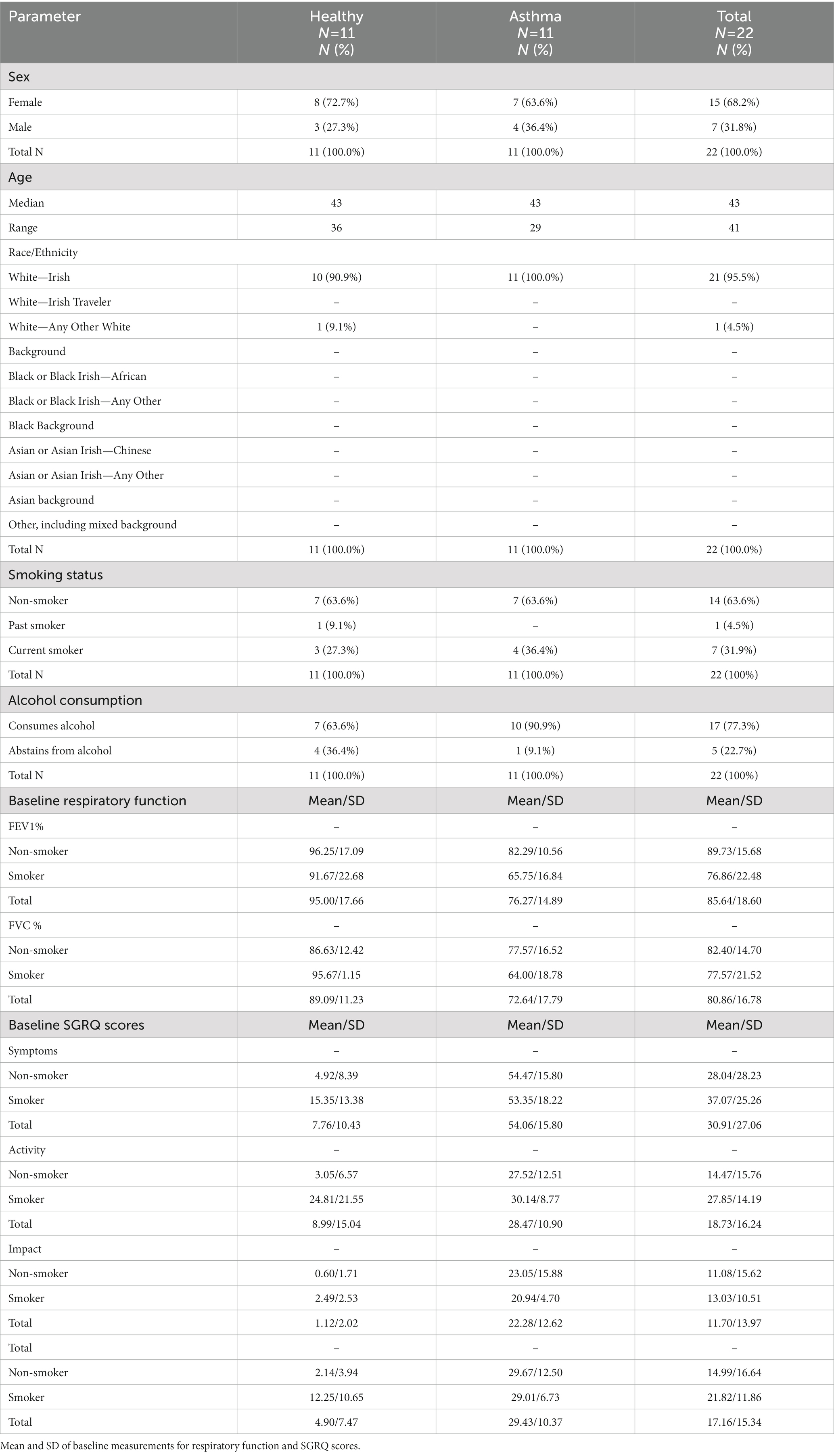
Table 1. Frequency table of participant demographics collected at baseline in the safety population.
No SAEs or withdrawals due to AEs occurred during this study in the healthy and asthmatic populations, successfully satisfying our primary endpoint (Table 2). There was only one mild gastrointestinal complaint of bloating, which required no action, reported during the trial (Supplementary Table S1). Individual results for vital signs and safety blood parameters were clinically reviewed by the medical doctor and deemed to be safe at all timepoints across all participants (Supplementary Tables S2, S3). No participants reported changes in their alcohol habits during the course of the study.
3.2. Probiotic blend improves lung function in asthmatic subjects
Spirometry measurements were taken at Baseline and Week 4 to assess changes in lung function. Data was normally distributed as measured by D’Agostino and Pearson test of normality: FEV1 (K2 = 1.097, p = 0.5778), FVC (K2 = 0.4601, p = 0.7945). In asthmatic participants, average FEV1% increased significantly (p = 0.018) and FVC trended up (p = 0.082) from Baseline to Week 4 (Table 3). The healthy population did not see a change in FEV1% (p = 0.099) or FVC (p = 0.387), and neither group had a significant improvement in FEV/FVC ratio (healthy p = 0.113, asthma p = 0.284). Three patients showed a decrease in average FEV1 or FVC, but the change was <10% (Supplementary Table S4).
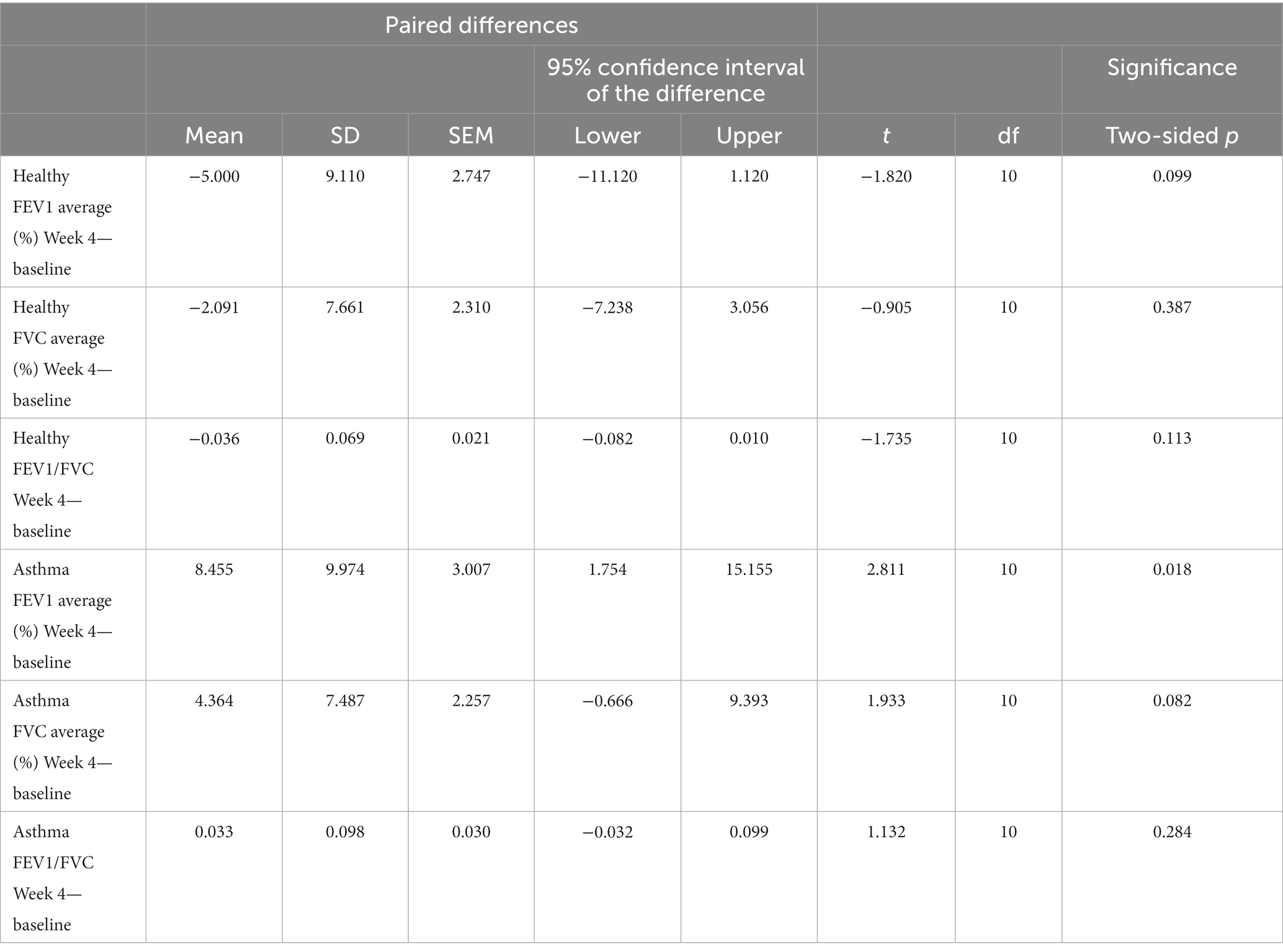
Table 3. Paired samples test to assess within group change in lung function parameters from baseline to Week 4 in the healthy (n = 11) and asthma (n = 11) populations.
3.3. Probiotic blend improves quality of life scores as measured by SGRQ
Participants responded to the Saint George’s Respiratory Questionnaire (SGRQ) at Baseline, Week 2, and Week 4 to evaluate the supplement’s effect on quality of life as measured through symptoms, impact, and total score (Supplementary Table S5). Across total score and the subcategories of symptoms, activity, and impact there was no significant change from baseline to Week 4 in either the healthy or asthmatic participants (Table 4). However, impact scores in asthmatic participants trended toward significance (p = 0.065). Of the participants that smoke, have asthma, or have asthma and smoke, 36% noted an improvement in their overall health, 43% noted less frequent coughing, 43% noted fewer instances of feeling short of breath, and 29% noted fewer cough or breathing-related sleep disturbances.
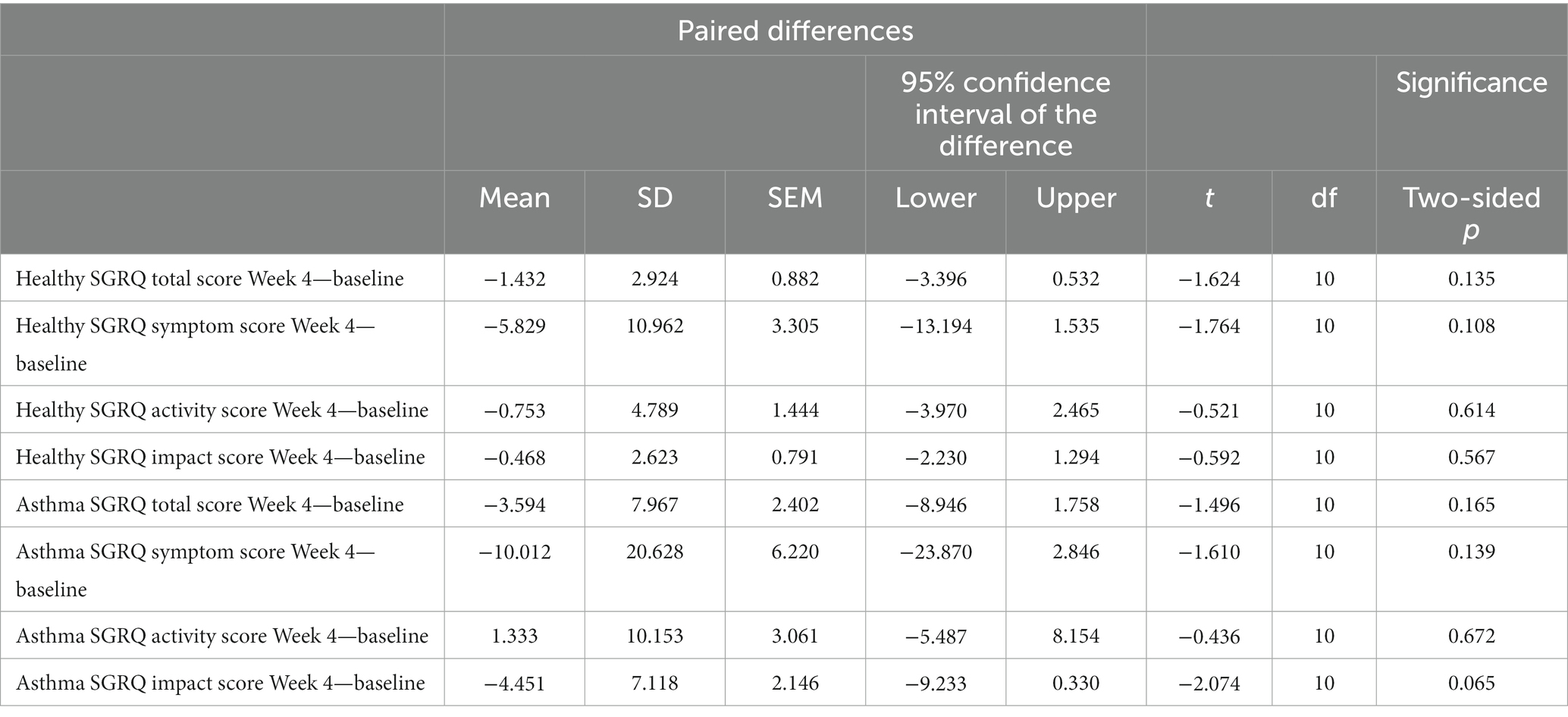
Table 4. Paired samples test to assess within group change in SGRQ total score and subscales from Baseline to Week 4 in the healthy (n = 11) and asthma (n = 11) populations.
3.4. Majority of participants would recommend probiotic and herbal blend
In an end of trial survey, 100% of participants who smoke would recommend the probiotic and herbal blend to friends and family. Ninety percent of asthmatic participants and 82% of healthy participants would recommend the same.
3.5. Probiotic blend improves serum short chain fatty acid levels in asthmatic subjects
Serum was analyzed for changes in short chain fatty acid (SCFA) levels between baseline and Week 4. Participants with asthma showed significant changes in serum SCFA levels. Propionic acid increased across all asthmatics (Figure 2A), propionic acid and isovaleric acid increased significantly in asthmatic non-smokers (Figure 2B), and acetic acid and butyric acid significantly increased in subjects with asthma who smoked (Figure 2C). Across all groups, isobutyric acid and valeric acid did not show a significant change, and hexanoic acid levels were too low for detection.
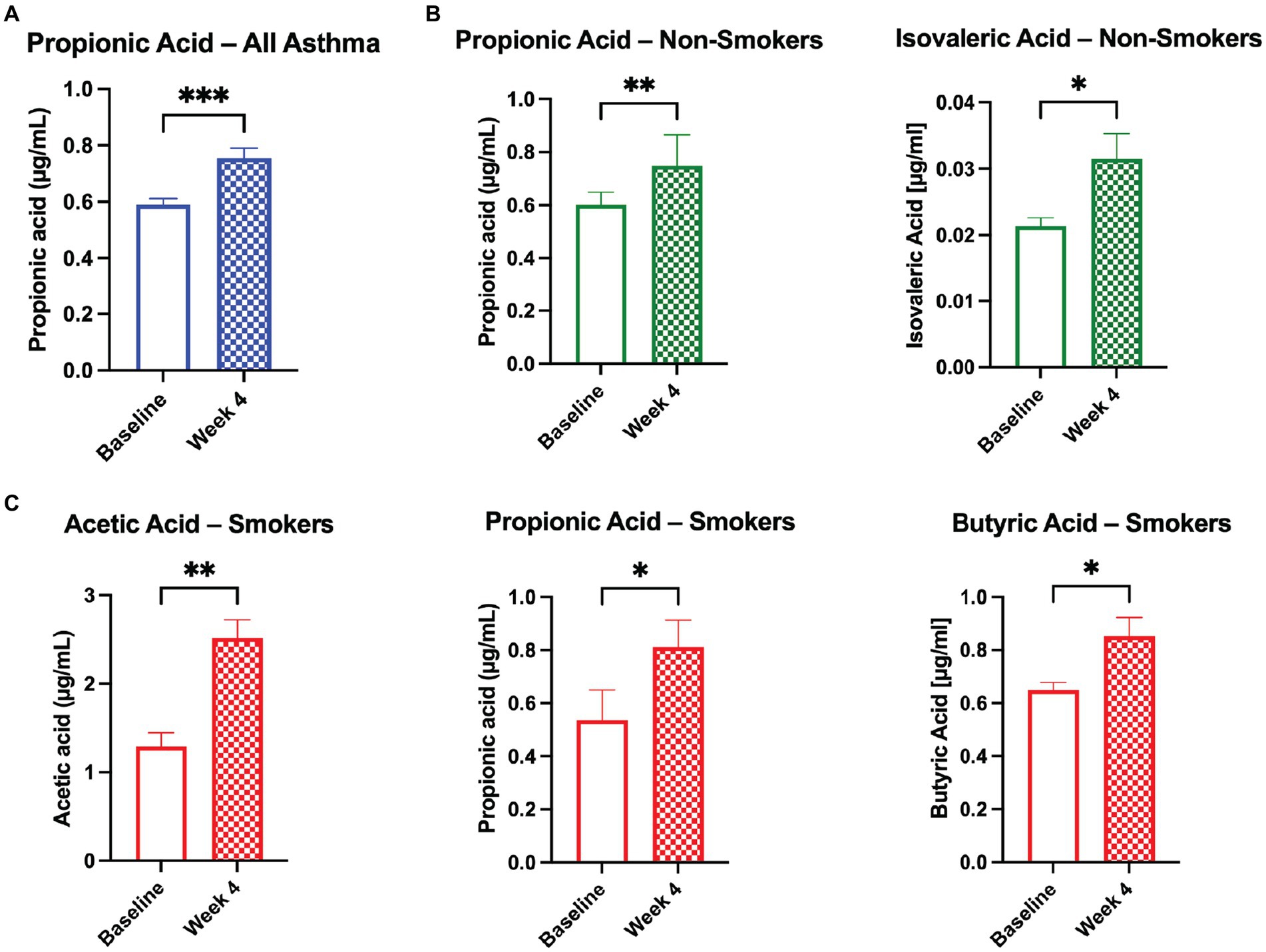
Figure 2. Probiotic and herbal blend improves serum SCFA levels in the asthmatic population. Significant increases in serum short chain fatty acid (SCFA) levels as broken down by (A) all asthmatic participants, (B) asthmatic non-smokers, and (C) asthmatic smokers. *p < 0.05, **p < 0.01, ***p < 0.001.
3.6. Gut microbiome profile differs in asthmatic subjects
To examine the effects of the probiotic on the intestinal microbiome for potentially adverse signals, we collected stool samples at baseline and Week 4 and analyzed for participants’ gut microbiome signatures. The overall alpha (intra-sample) and beta (inter-sample) diversity was not significantly altered between baseline and after 4 weeks of probiotic administration (Shannon Diversity, p = 0.644, t-test; Chao1 Richness Index, p = 0.665, t-test, Figure 3A, p = 1, R2 = 0.007, PERMANOVA; p = 0.279, PERMDISP, Figure 3B), with only modest differences in colonization at the genus level (Supplementary Table S6).
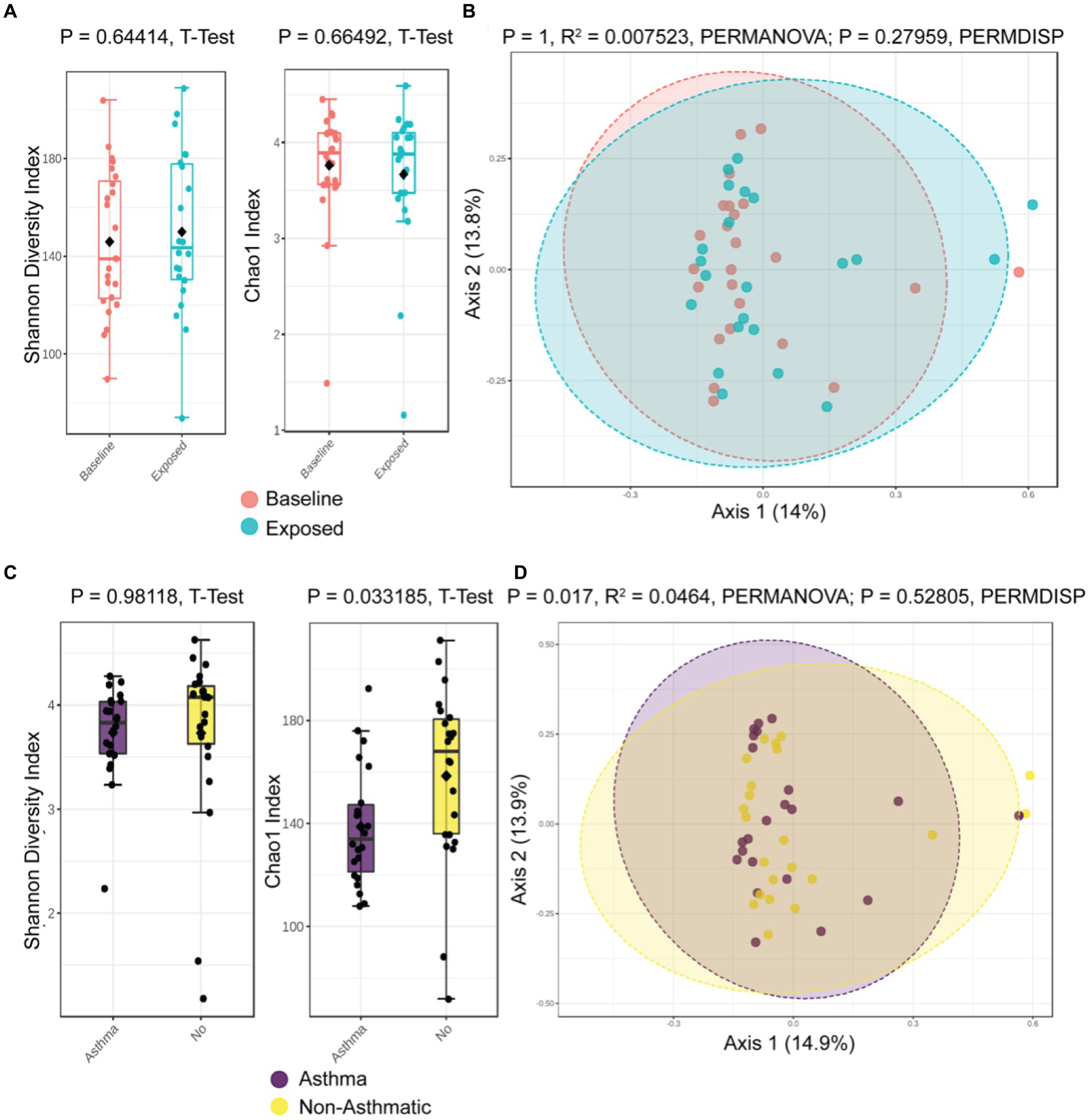
Figure 3. Global microbiome community composition not disrupted by probiotic administration, but asthmatics have a different gut microbiome. (A) Alpha diversity is unaltered by probiotic administration. (B) Beta diversity remains similar following probiotic administration. Asthmatic patients have different alpha diversity (C) and beta diversity (D).
However, the patient’s asthma status did associate with differences in alpha and beta diversity (Chao1, p = 0.033 Figure 3C, and p = 0.017, R2 = 0.0464, PERMANOVA; p = 0.52805, PERMDISP, Figure 3D). The most prominent differences at the genus level were higher relative abundance of E. coli (Log2FC 26.6), Bacteroidetes dorei (Log2FC 24.2), and B. ovatus (Log2FC 21.7) (Supplementary Table S7).
3.7. Probiotic Lactobacillus strains detected in stool
We performed qPCR using primers validated for strain-specificity. Most samples were not colonized with L. plantarum at baseline (19%), but this signature was augmented in most samples by administration of the probiotic and herbal blend (81%, χ2, 12.5, p = 0.0004). L. acidophilus colonization was more common at baseline (37%) and was detected in most of the post-exposure samples (62%, χ2, 2, p = 0.157). Similarly, L. rhamnosus was detected in most individuals before and after probiotic administration (62% vs. 81%, χ2, 1.39, p = 0.238).
In healthy participants, the concentration of L. plantarum increased significantly and L. acidophilus and L. rhamnosus trended upward from baseline to Week 4 after taking the probiotic and herbal blend (Figure 4A). Percent densitometry was calculated from PCR product bands of gels and of L. plantarum and L. acidophilus increased from baseline to Week 4 with L. rhamnosus trending up (Figure 4B). In asthmatic participants, the concentration of all three Lactobacillus strains increased significantly from baseline to Week 4 (Figure 4C). Percent densitometry of L. plantarum and L. acidophilus increased from baseline to Week 4 and L. rhamnosus trended upward (Figure 4D).
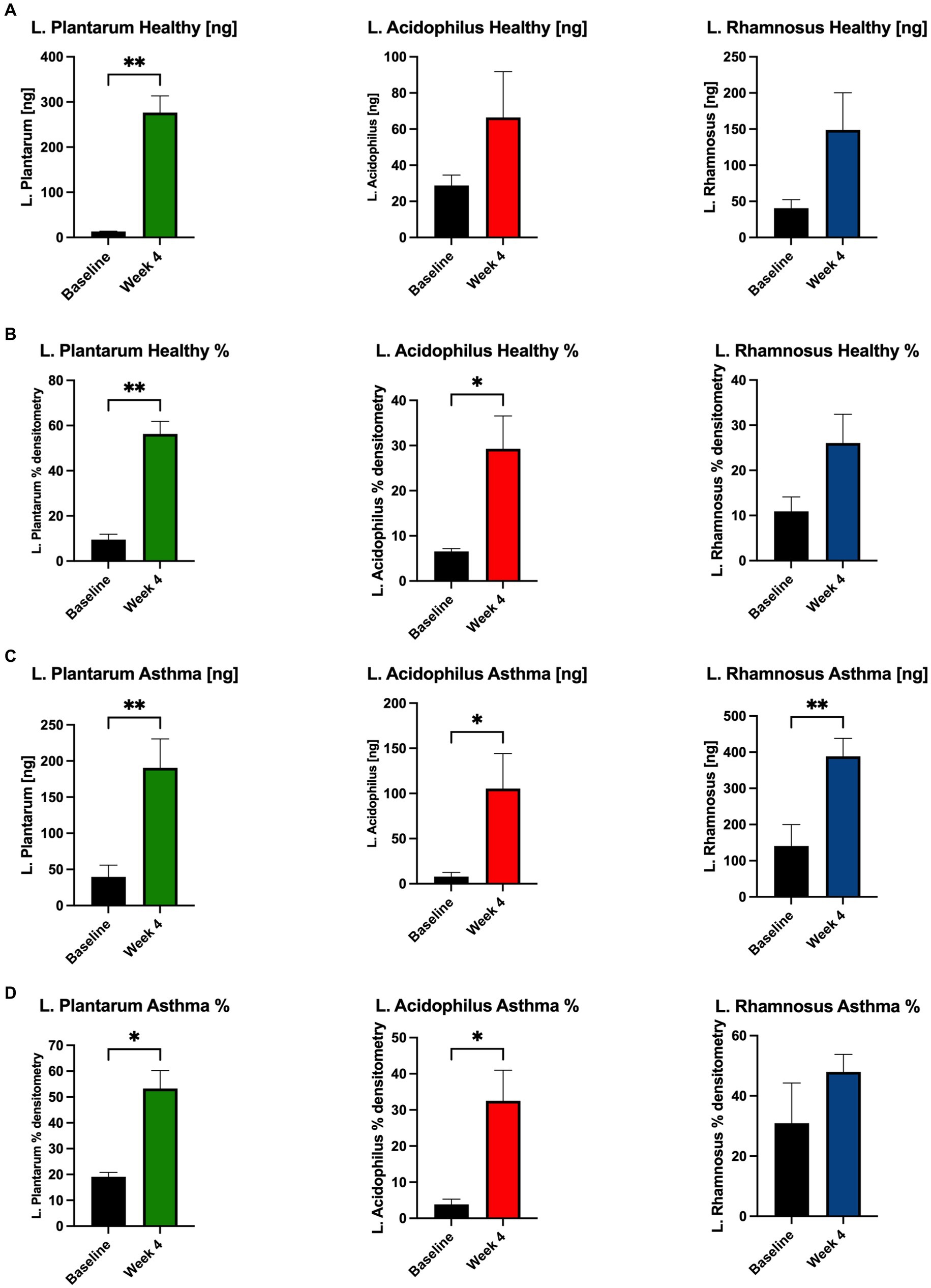
Figure 4. Abundance of probiotic Lactobacillus strains increases in stool of participants. In healthy participants, the concentration [ng] of (A) L. plantarum increased significantly and L. acidophilus and L. rhamnosus trended upward from baseline to Week 4 after supplementation. (B) % densitometry of L. plantarum and L. acidophilus increased significantly and L. rhamnosus trended upward from baseline to Week 4. In asthmatic participants, the concentration [ng] of (C) all three Lactobacillus strains increased significantly from baseline to Week 4 after supplementation. (D) % densitometry of L. plantarum and L. acidophilus increased significantly and L. rhamnosus trended upward from baseline to Week 4. *p < 0.05, **p < 0.01.
4. Discussion
In our 1-month clinical trial dosing a Lactobacillus probiotic blend to healthy volunteers and asthmatic participants, we found that the supplement was safe and well-tolerated among all subjects, fulfilling our primary endpoint. This was anticipated because prior to this clinical trial, all three probiotic strains have been independently clinically validated and the herbs have a long history of use in preclinical research and traditional medicine (8, 9, 27–30).
Although a majority of the participants did show a modest improvement in quality-of-life scores, the changes were not significant in the healthy or asthmatic participants. In the healthy participants, their SGRQ scores were generally good at baseline which meant that any change was incremental. Even the asthmatic population that was more likely to start from a lower baseline (higher score) did not show a significant change. The dosing period of the trial was only 4 weeks which did not allow for enough time to capture changes in perceived symptoms as a result of taking the blend.
There were slight decreases in average FEV1 and/or FVC in 3 asthmatic patients: 2 smokers and 1 non-smoker. However, the differences from baseline to Week 4 were small, with increases <10% of the lower Week 4 value. Additionally, it was noted that 10 patients had a > 10% difference between their two FEV or FVC readings which could have skewed the average value. Several asthma patients did report increases in SGRQ scores from baseline to Week 4; however, two of the participants with large increases (AN2 and AN4) both contracted lower respiratory tract infections unrelated to taking study product during the course of the trial which may have affected the results of the questionnaire. A third asthma patient with an increase in SGRQ scores has allergic rhinitis, which may have played a role, although they reported no AE or SAEs. Across all participants, there were no related respiratory AEs reported and no SAEs of any kind reported.
SCFA levels were not significantly changed in the healthy population from baseline to Visit 4. However, SCFA levels were significantly upregulated in the serum of the asthmatic participants, suggesting a pre-existing deficiency that was addressed through supplementation. SCFAs are known to affect immune cell function and a variety of inflammatory pathways including TNF-α, IL-2, IL-6, and IL-10 (31). We observed distinct increases in serum SCFAs acetic acid, propionic acid, and butyric acid in subjects with asthma who smoked, and propionic acid and isovaleric acid in subjects with asthma who did not smoke. Gut dysbiosis diminishes metabolism of anti-inflammatory SCFAs, impairing the body’s ability to regulate systemic inflammation and exacerbating allergic lung inflammation (19, 20, 32, 33).
An interesting finding was the significant improvement in lung function in the asthmatic group as measured by FEV1%. This change may have been due to the significant uptick in serum SCFAs which traveled through systemic circulation and reduced inflammation in the lungs. Nevertheless, the sample size in this study is too small to determine a direct correlation, and larger studies would be necessary to confirm this correlation. Previous studies have shown an association between Lactobacillus administration and immune regulation of Th1/Th2 response, reducing allergic inflammation characteristic of asthma (14, 34). The effect of the herbal extracts can also not be discounted, as turmeric, holy basil, and vasaka have antioxidant and anti-inflammatory properties (22, 35). Although FVC values were not statistically significant, a longer study with more subjects may reveal a larger impact over time.
As anticipated, the probiotic induced no significant alterations in the global gut microbiome community composition of the human participants (36). This agrees with prior literature that demonstrates a limited long-term alteration in the gut microbiota from probiotics in healthy adults, that stems from colonization resistance from the microbiota and host factors that perform ecology maintenance (36–40). Alternatively, prior studies of probiotic administration have suggested that the administration of a Lactobacillus probiotic can alter the prevalence of other Lactobacillus strains without altering overall community structure (36). We verified by strain-specific PCR that the three Lactobacillus strains contained in the probiotic blend increased from baseline to Week 4, of note significantly in the asthmatic population.
We identified robust differences in the microbiota of asthmatic patients in our cohort, confirming prior observations that community composition was associated with altered microbiota (41, 42). Specifically, an increase in proteobacteria E. coli was particularly notable, since proteobacteria have been linked with increased risk of respiratory disease (43). These differences are interesting and deserving of further validation in a larger cohort of asthmatic individuals.
There are several limitations to this study, as its primary focus was safety. The study was unblinded and did not have a placebo group. It is difficult to credit the observed improvements to specific components of the blend. We also did not collect efficacy data at Week 2 (Visit 3), although it is unlikely that an effect would have been observed after 2 weeks. The magnitude of change in SCFAs required to affect biomarkers is unknown, as we did not assess asthma-specific markers of inflammation in serum such as eosinophils, leukotrienes, or IL-5. Future trials will include a placebo arm and a longer administration period in order to be able to draw stronger conclusions about clinical efficacy endpoints.
Information on the type or severity of asthma was not collected. If a participant had intermittent or non-seasonal asthma, their resolution of symptoms or improvement in lung function could have been reflective of the regular course of the disease. In regard to allergic asthma, three of the asthmatic participants reported having allergic rhinitis; however, none reported allergic symptoms during the trial. If a participant did have an allergic asthma attack, it could have skewed the SGRQ or lung function results, providing either a false improvement or false worsening result. However, due to the season in which the trial was conducted, a seasonal attack was less likely. The study was conducted in its entirety between November 23, 2021, and January 20, 2022, a time of the year during which seasonal allergies are rare and do not begin until mid-late January in Ireland.
In summary, the Lactobacillus probiotic and herbal blend was found to be safe in healthy and asthmatic subjects, and improvements in lung function were accompanied by potentially beneficial increases in SCFA in asthmatic subjects. Based on the results of this preliminary clinical study, we propose that this blend may improve lung function and inflammation by supplementing microbes in the gut to increase SCFA production in systemic circulation. Clinical relevance of these findings affects both patients with and without existing respiratory conditions who are seeking a means to support their lung health.
Data availability statement
The datasets presented in this study can be found in online repositories. The names of the repository/repositories and accession number(s) can be found: https://www.ncbi.nlm.nih.gov/bioproject/PRJNA922968, PRJNA922968.
Ethics statement
The studies involving human participants were reviewed and approved by the Clinical Research Ethics Committee of the Cork Teaching Hospitals of University College Cork. The patients/participants provided their written informed consent to participate in this study.
Author contributions
CL: study conception and strategy. NW, LQ, TN, ZN, XX, AG, and CL: study design. NW, TN, KW, NA, AG, CL, and BH: manuscript preparation and interpretation. NW, TD, AG, and CL: clinical trial design and execution. TN, ZN, LQ, GD, CK, AG, CL, BH, KT, ME, and IM: clinical data analysis. All authors reviewed, advised, and approved the final version submitted for publication and agree to be accountable for the work.
Funding
Research reported in this article was supported by the National Heart, Lung and Blood Institute of the National Institutes of Health under award number K08 HL141652 (CL) and K08 HL151907 (KW). resB Lung Support for clinical trial use was gifted by ResBiotic Nutrition, Inc.
Acknowledgments
A preprint of this manuscript has been published online at medRxiv.
Conflict of interest
ResBiotic Nutrition Inc. is a university startup out of the University of Alabama at Birmingham of which CL is the Founder, AG is the Chief Medical Officer, and NA and KW are Advisors.
The remaining authors declare that the research was conducted in the absence of any commercial or financial relationships that could be construed as a potential conflict of interest.
Publisher’s note
All claims expressed in this article are solely those of the authors and do not necessarily represent those of their affiliated organizations, or those of the publisher, the editors and the reviewers. Any product that may be evaluated in this article, or claim that may be made by its manufacturer, is not guaranteed or endorsed by the publisher.
Supplementary material
The Supplementary material for this article can be found online at: https://www.frontiersin.org/articles/10.3389/fnut.2023.1168582/full#supplementary-material
References
1. Bowerman, KL, Rehman, SF, Vaughan, A, Lachner, N, Budden, KF, Kim, RY, et al. Disease-associated gut microbiome and metabolome changes in patients with chronic obstructive pulmonary disease. Nat Commun. (2020) 11:5886. doi: 10.1038/s41467-020-19701-0
2. Yeoh, YK, Zuo, T, Lui, GC-Y, Zhang, F, Liu, Q, Li, AY, et al. Gut microbiota composition reflects disease severity and dysfunctional immune responses in patients with COVID-19. Gut. (2021) 70:698–706. doi: 10.1136/gutjnl-2020-323020
3. Yazar, A, Atis, S, Konca, K, Pata, C, Akbay, E, Calikoglu, M, et al. Respiratory symptoms and pulmonary functional changes in patients with irritable bowel syndrome. Am J Gastroenterol. (2001) 96:1511–6. doi: 10.1111/j.1572-0241.2001.03748.x
4. Hilty, M, Burke, C, Pedro, H, Cardenas, P, Bush, A, Bossley, C, et al. Disordered microbial communities in asthmatic airways. PLoS One. (2010) 5:e8578. doi: 10.1371/journal.pone.0008578
5. Marri, PR, Stern, DA, Wright, AL, Billheimer, D, and Martinez, FD. Asthma-associated differences in microbial composition of induced sputum. J Allergy Clin Immunol. (2013) 131:346–352.e3. doi: 10.1016/j.jaci.2012.11.013
6. Yan, S, Ma, Z, Jiao, M, Wang, Y, Li, A, and Ding, S. Effects of smoking on inflammatory markers in a healthy population as analyzed via the gut microbiota. Front Cell Infect Microbiol. (2021) 11:633242. doi: 10.3389/fcimb.2021.633242
7. Allais, L, Kerckhof, FM, Verschuere, S, Bracke, KR, De Smet, R, Laukens, D, et al. Chronic cigarette smoke exposure induces microbial and inflammatory shifts and mucin changes in the murine gut. Environ Microbiol. (2016) 18:1352–63. doi: 10.1111/1462-2920.12934
8. Chong, H-X, Yusoff, NAA, Hor, Y-Y, Lew, L-C, Jaafar, MH, Choi, S-B, et al. Lactobacillus plantarum DR7 improved upper respiratory tract infections via enhancing immune and inflammatory parameters: a randomized, double-blind, placebo-controlled study. J Dairy Sci. (2019) 102:4783–97. doi: 10.3168/jds.2018-16103
9. Hojsak, I, Abdović, S, Szajewska, H, Milosević, M, Krznarić, Z, and Kolacek, S. Lactobacillus GG in the prevention of nosocomial gastrointestinal and respiratory tract infections. Pediatrics. (2010) 125:e1171–7. doi: 10.1542/peds.2009-2568
10. Kumar, A, Alrefai, WA, Borthakur, A, and Dudeja, PK. Lactobacillus acidophilus counteracts enteropathogenic E. coli-induced inhibition of butyrate uptake in intestinal epithelial cells. Am J Physiol Gastrointest Liver Physiol. (2015) 309:G602–7. doi: 10.1152/ajpgi.00186.2015
11. Wullt, M, Johansson Hagslatt, ML, Odenholt, I, and Berggren, A. Lactobacillus plantarum 299v enhances the concentrations of fecal short-chain fatty acids in patients with recurrent clostridium difficile-associated diarrhea. Dig Dis Sci. (2007) 52:2082–6. doi: 10.1007/s10620-006-9123-3
12. Moens, F, Duysburgh, C, van den Abbeele, P, Morera, M, and Marzorati, M. Lactobacillus rhamnosus GG and Saccharomyces cerevisiae boulardii exert synergistic antipathogenic activity in vitro against enterotoxigenic Escherichia coli. Benef Microbes. (2019) 10:923–35. doi: 10.3920/BM2019.0064
13. Arpaia, N, Campbell, C, Fan, X, Dikiy, S, van der Veeken, J, deRoos, P, et al. Metabolites produced by commensal bacteria promote peripheral regulatory T-cell generation. Nature. (2013) 504:451–5. doi: 10.1038/nature12726
14. Sadrifar, S, Abbasi-Dokht, T, Forouzandeh, S, Malek, F, Yousefi, B, Salek Farrokhi, A, et al. Immunomodulatory effects of probiotic supplementation in patients with asthma: a randomized, double-blind, placebo-controlled trial. Allergy, Asthma Clin Immunol. (2023) 19:1. doi: 10.1186/s13223-022-00753-4
15. Huang, CF, Chie, WC, and Wang, IJ. Efficacy of Lactobacillus administration in school-age children with asthma: a randomized, placebo-controlled trial. Nutrients. (2018) 10:1678. doi: 10.3390/nu10111678
16. Liu, A, Ma, T, Xu, N, Jin, H, Zhao, F, Kwok, LY, et al. Adjunctive probiotics alleviates asthmatic symptoms via modulating the gut microbiome and serum metabolome. Microbiol Spectr. (2021) 9:e0085921. doi: 10.1128/Spectrum.00859-21
17. Chen, YS, Jan, RL, Lin, YL, Chen, HH, and Wang, JY. Randomized placebo-controlled trial of lactobacillus on asthmatic children with allergic rhinitis. Pediatr Pulmonol. (2010) 45:1111–20. doi: 10.1002/ppul.21296
18. Wickens, K, Barthow, C, Mitchell, EA, Kang, J, van Zyl, N, Purdie, G, et al. Effects of Lactobacillus rhamnosus HN001 in early life on the cumulative prevalence of allergic disease to 11 years. Pediatr Allergy Immunol. (2018) 29:808–14. doi: 10.1111/pai.12982
19. Trompette, A, Gollwitzer, ES, Yadava, K, Sichelstiel, AK, Sprenger, N, Ngom-Bru, C, et al. Gut microbiota metabolism of dietary fiber influences allergic airway disease and hematopoiesis. Nat Med. (2014) 20:159–66. doi: 10.1038/nm.3444
20. Cait, A, Hughes, MR, Antignano, F, Cait, J, Dimitriu, PA, Maas, KR, et al. Microbiome-driven allergic lung inflammation is ameliorated by short-chain fatty acids. Mucosal Immunol. (2018) 11:785–95. doi: 10.1038/mi.2017.75
21. Amala, R, and Sujatha, S. Presence of pyrroloquinazoline alkaloid in Adhatoda vasica attenuates inflammatory response through the downregulation of pro-inflammatory mediators in LPS stimulated RAW 264.7 macrophages. Bioimpacts. (2021) 11:15–22. doi: 10.34172/bi.2021.03
22. Manarin, G, Anderson, D, Silva, JME, Coppede, JDS, Roxo-Junior, P, Pereira, AMS, et al. Curcuma longa L. ameliorates asthma control in children and adolescents: a randomized, double-blind, controlled trial. J Ethnopharmacol. (2019) 238:111882. doi: 10.1016/j.jep.2019.111882
23. Kelm, MA, Nair, MG, Strasburg, GM, and DeWitt, DL. Antioxidant and cyclooxygenase inhibitory phenolic compounds from Ocimum sanctum Linn. Phytomedicine. (2000) 7:7–13. doi: 10.1016/S0944-7113(00)80015-X
24. Wenger, NM, Qiao, L, Nicola, T, Nizami, Z, Xu, X, Willis, KA, et al. Efficacy of a probiotic and herbal supplement in models of lung inflammation. Microorganisms. (2022) 10:2136. doi: 10.3390/microorganisms10112136
25. Chong, J, Liu, P, Zhou, G, and Xia, J. Using MicrobiomeAnalyst for comprehensive statistical, functional, and meta-analysis of microbiome data. Nat Protoc. (2020) 15:799–821. doi: 10.1038/s41596-019-0264-1
26. Dhariwal, A, Chong, J, Habib, S, King, IL, Agellon, LB, and Xia, J. MicrobiomeAnalyst: a web-based tool for comprehensive statistical, visual and meta-analysis of microbiome data. Nucleic Acids Res. (2017) 45:W180–8. doi: 10.1093/nar/gkx295
27. Dhuley, JN . Antitussive effect of Adhatoda vasica extract on mechanical or chemical stimulation-induced coughing in animals. J Ethnopharmacol. (1999) 67:361–5. doi: 10.1016/S0378-8741(99)00074-4
28. Maheshwari, RK, Singh, AK, Gaddipati, J, and Srimal, RC. Multiple biological activities of curcumin: a short review. Life Sci. (2006) 78:2081–7. doi: 10.1016/j.lfs.2005.12.007
29. Mondal, S, Varma, S, Bamola, VD, Naik, SN, Mirdha, BR, Padhi, MM, et al. Double-blinded randomized controlled trial for immunomodulatory effects of Tulsi (Ocimum sanctum Linn.) leaf extract on healthy volunteers. J Ethnopharmacol. (2011) 136:452–6. doi: 10.1016/j.jep.2011.05.012
30. Lyra, A, Hillilä, M, Huttunen, T, Männikkö, S, Taalikka, M, Tennilä, J, et al. Irritable bowel syndrome symptom severity improves equally with probiotic and placebo. World J Gastroenterol. (2016) 22:10631–42. doi: 10.3748/wjg.v22.i48.10631
31. Vinolo, MA, Rodrigues, HG, Nachbar, RT, and Curi, R. Regulation of inflammation by short chain fatty acids. Nutrients. (2011) 3:858–76. doi: 10.3390/nu3100858
32. Shin, N-R, Whon, TW, and Bae, J-W. Proteobacteria: microbial signature of dysbiosis in gut microbiota. Trends Biotechnol. (2015) 33:496–503. doi: 10.1016/j.tibtech.2015.06.011
33. Saint-Criq, V, Lugo-Villarino, G, and Thomas, M. Dysbiosis, malnutrition and enhanced gut-lung axis contribute to age-related respiratory diseases. Ageing Res Rev. (2021) 66:101235. doi: 10.1016/j.arr.2020.101235
34. Torii, A, Torii, S, Fujiwara, S, Tanaka, H, Inagaki, N, and Nagai, H. Lactobacillus Acidophilus strain L-92 regulates the production of Th1 cytokine as well as Th2 cytokines. Allergol Int. (2007) 56:293–301. doi: 10.2332/allergolint.O-06-459
35. Jamshidi, N, and Cohen, MM. The clinical efficacy and safety of Tulsi in humans: a systematic review of the literature. Evid Based Complement Alternat Med. (2017) 2017:1–13. doi: 10.1155/2017/9217567
36. Fuentes, S, Egert, M, Jiménez-Valera, M, Ramos-Cormenzana, A, Ruiz-Bravo, A, Smidt, H, et al. Administration of Lactobacillus casei and Lactobacillus plantarum affects the diversity of murine intestinal lactobacilli, but not the overall bacterial community structure. Res Microbiol. (2008) 159:237–43. doi: 10.1016/j.resmic.2008.02.005
37. Heilig, HGHJ, Zoetendal, EG, Vaughan, EE, Marteau, P, Akkermans, ADL, and Vos, WM. Molecular diversity of Lactobacillus spp. and other lactic acid Bacteria in the human intestine as determined by specific amplification of 16S ribosomal DNA. Appl Environ Microbiol. (2002) 68:114–23. doi: 10.1128/AEM.68.1.114-123.2002
38. Zmora, N, Zilberman-Schapira, G, Suez, J, Mor, U, Dori-Bachash, M, Bashiardes, S, et al. Personalized gut mucosal colonization resistance to empiric probiotics is associated with unique host and microbiome features. Cells. (2018) 174:1388–405.e21. doi: 10.1016/j.cell.2018.08.041
39. Suez, J, Zmora, N, Zilberman-Schapira, G, Mor, U, Dori-Bachash, M, Bashiardes, S, et al. Post-antibiotic gut mucosal microbiome reconstitution is impaired by probiotics and improved by autologous FMT. Cells. (2018) 174:1406–23.e16. doi: 10.1016/j.cell.2018.08.047
40. Liou, MJ, Miller, BM, Litvak, Y, Nguyen, H, Natwick, DE, Savage, HP, et al. Host cells subdivide nutrient niches into discrete biogeographical microhabitats for gut microbes. Cell Host Microbe. (2022) 30:836–47.e6. doi: 10.1016/j.chom.2022.04.012
41. Wang, Z, Lai, Z, Zhang, X, Huang, P, Xie, J, Jiang, Q, et al. Altered gut microbiome compositions are associated with the severity of asthma. J Thorac Dis. (2021) 13:4322–38. doi: 10.21037/jtd-20-2189
42. Frati, F, Salvatori, C, Incorvaia, C, Bellucci, A, Di Cara, G, Marcucci, F, et al. The role of the microbiome in asthma: the gut–lung Axis. Int J Mol Sci. (2019) 20:123. doi: 10.3390/ijms20010123
Keywords: microbiome, gut-lung axis, probiotic, Lactobacillus , short-chain fatty acids, asthma
Citation: Wenger NM, Qiao L, Nicola T, Nizami Z, Martin I, Halloran BA, Tanaka K, Evans M, Xu X, Dinan TG, Kakilla C, DunnGalvin G, Ambalavanan N, Willis KA, Gaggar A and Lal CV (2023) Clinical trial of a probiotic and herbal supplement for lung health. Front. Nutr. 10:1168582. doi: 10.3389/fnut.2023.1168582
Edited by:
Nabil Bosco, Lesaffre Group, FranceCopyright © 2023 Wenger, Qiao, Nicola, Nizami, Martin, Halloran, Tanaka, Evans, Xu, Dinan, Kakilla, DunnGalvin, Ambalavanan, Willis, Gaggar and Lal. This is an open-access article distributed under the terms of the Creative Commons Attribution License (CC BY). The use, distribution or reproduction in other forums is permitted, provided the original author(s) and the copyright owner(s) are credited and that the original publication in this journal is cited, in accordance with accepted academic practice. No use, distribution or reproduction is permitted which does not comply with these terms.
*Correspondence: Charitharth Vivek Lal, Y2xhbEB1YWJtYy5lZHU=
†These authors have contributed equally to this work and share first authorship