- 1Université Paris-Saclay, AgroParisTech, INRAE, UMR PNCA, 91120, Palaiseau, France
- 2Université Sorbonne Paris Nord and Université Paris Cité, Inserm, INRAE, CNAM, Center of Research in Epidemiology and StatisticS (CRESS), Nutritional Epidemiology Research Team (EREN), F-93017, Bobigny, France
Background: Animal and plant protein sources have contrasting relationships with nutrient adequacy and long-term health, and their adequate ratio is highly debated.
Objective: We aimed to explore how the percentage of plant protein in the diet (%PP) relates to nutrient adequacy and long-term health but also to environmental pressures, to determine the adequate and potentially optimal %PP values.
Methods: Observed diets were extracted from the dietary intakes of French adults (INCA3, n = 1,125). Using reference values for nutrients and disease burden risks for foods, we modeled diets with graded %PP values that simultaneously ensure nutrient adequacy, minimize long-term health risks and preserve at best dietary habits. This multi-criteria diet optimization was conducted in a hierarchical manner, giving priority to long-term health over diet proximity, under the constraints of ensuring nutrient adequacy and food cultural acceptability. We explored the tensions between objectives and identified the most critical nutrients and influential constraints by sensitivity analysis. Finally, environmental pressures related to the modeled diets were estimated using the AGRIBALYSE database.
Results: We find that nutrient-adequate diets must fall within the ~15–80% %PP range, a slightly wider range being nevertheless identifiable by waiving the food acceptability constraints. Fully healthy diets, also achieving the minimum-risk exposure levels for both unhealthy and healthy foods, must fall within the 25–70% %PP range. All of these healthy diets were very distant from current typical diet. Those with higher %PP had lower environmental impacts, notably on climate change and land use, while being as far from current diet.
Conclusion: There is no single optimal %PP value when considering only nutrition and health, but high %PP diets are more sustainable. For %PP > 80%, nutrient fortification/supplementation and/or new foods are required.
Introduction
Historical and current nutritional transitions are coupled with changes in the relative contribution of dietary animal and plant proteins. This has been studied from hunter-gatherers to post-agricultural societies (1), from traditional diets that enabled thriving civilizations to diets in post-industrialized countries (2, 3), and more recently, in Western countries with the emerging trend toward more plant-predominant diets.
Changes in plant and animal protein intake raise classic nutritional questions. One in particular concerns the possible risk of some nutrient shortage with diets too low in animal protein, since animal protein foods contribute significantly to the intake of indispensable nutrients like iron, calcium and vitamin B12, whose overt deficiencies have various adverse health consequences (such as anemia and higher risk of osteopotosis) (4, 5). However, plant proteins are also important for the intake of fiber and some indispensable nutrients (like vitamins B9 and C), which modulate short and long-term disease risk, and are also lower in saturated fats that are excessively consumed (6, 7). Beyond the relationship to nutrient adequacy, animal/plant proteins and their packages largely affect the metabolome and the microbiota and physiological functions that are crucial for long-term health (6, 8–10). Accordingly, there have been many contrasting associations reported recently between plant and animal protein intake and mortality, especially regarding cardiovascular diseases (6, 11, 12).
More globally, plant (such as legumes, nuts and whole grains) and animal (such as red and processed meats) protein sources have heterogeneous relationships to nutrient adequacy (13) and to long-term health regarding cardiovascular diseases (9, 14–16) and cancers (17, 18). There is indeed a challenge for food-based dietary guidelines to point out what proportions of plant and animal protein foods should be recommended (19, 20). However, the plant to animal protein ratio remains a poor, summarizing descriptor of dietary patterns, since two diets with the same plant to animal protein ratio can actually be very different (9). There is thus a need to analyze the overall proportion of plant protein in the diet in view of the related dietary profiles and their nutritional adequacy and healthiness.
Furthermore, current interest in the proportions of plant and animal proteins in the diet also stems from their differential association with environmental pressures, in particular greenhouse gas emissions (GHGe) and land use (21–24). Altogether, the plant to animal protein ratio in the diet appears central to the sustainability of the food systems (21, 25). This has implications for dietary guidelines that aim to encompass both human and planetary health (26–28).
Thus, the literature lacks an analysis of what proportion of plant protein in the diet (%PP, the percentage of plant protein in total protein intake) is adequate and, even further, what proportion is optimal from a unified nutrition and health perspective that also considers the impact on other aspects of sustainability. We hypothesized that %PP could be safely increased well beyond its current low level, but will certainly be limited by a too-low level of animal protein. We also hypothesized that, rather than an optimal value, there may be a relatively wide range of %PP values that would be similarly adequate, when considering only human nutrition and health. Here, using advanced diet modeling and optimization, we studied whether an optimal %PP value can be identified when taking into account the reference values for nutrients and the disease burden risks for food categories. We characterized modeled diets that departed as little as possible from prevailing diets at all levels of adequate %PP values for nutrient adequacy and long-term health to identify nutritional issues (i.e., limiting nutrients) and dietary levers (i.e., effective foods). We furthermore estimated the environmental pressures associated with modeled diets along the whole range of adequate %PP values.
Materials and methods
Input of dietary data
The data used for this study were extracted from the French Individual and National Study on Food Consumption Survey 3 (INCA3) conducted in 2014–2015. The INCA3 survey is a representative cross-sectional survey of the French population; its method and design have been fully described elsewhere (29). Males aged 18–64 years (n = 564) and pre-menopausal females aged 18–54 years (n = 561), not identified as under-reporters, were included in the present study; the final sample contained 1,125 adults (Supplementary Figure S1).
Dietary data were collected by professional investigators assisted by a standardized and validated dietary software (GloboDiet) from three unplanned, non-consecutive, 24 h dietary recalls spread over a three-week period (two weekdays and one weekend day). Portion sizes were estimated using validated photographs (29), and the nutrient contents of different food items came from the 2016 food composition database operated by the French Information Centre on Food Quality (CIQUAL) (30). Mixed foods were broken down into ingredients and then gathered into 45 food groups (Supplementary Table S1). For each sex, the nutrient content of each food group was calculated as the mean nutrient content of food items constituting the food group weighted by their mean intake by the sex considered, as previously described (31). All dietary data (food group consumption and nutrient content) relate to the total population of each sex (including non-consumers).
Multi-criteria diet optimization under constraints
Using multi-criteria optimization, we identified modeled diets (i.e., modeled consumptions of the 45 food groups) with a minimal long-term health risk and a minimal departure from the observed diet (taking into account cultural acceptability and inertia), under constraints that would ensure adequate nutrient intakes and remain within current consumption limits. In this context, we investigated the role of %PP to identify its adequate range of variations and to characterize the dietary, nutritional and environmental consequences of these variations.
This non-linear optimization problem was performed using the NLP solver of the OPTMODEL procedure of SAS software version 9.4 (SAS Institute Inc., Cary, NC, USA). Optimization was implemented at the population level but in males and females, separately. The optimized diets of males and females were then averaged to derive optimized diets for the adult population.
Objectives
The main optimization objective was to minimize the long-term health risk of the modeled diet, as assessed by the Health Risk (HR) criterion. The HR criterion was set to target the dietary recommendations from the Global Burden of Diseases (GBD) based on epidemiological studies about the associations between consumption of different food groups and risk of chronic diseases (32). The HR criterion thus aimed to limit the consumption of three unhealthy food groups or categories (red meat, processed meat and sweetened beverages), while promoting that of six healthy food groups or categories (whole grain products, fruits, vegetables, legumes, nuts and seeds, and milk) until their minimum risk exposure levels (TMREL) were reached. According to the most recent (2019) estimates from the GBD, TMREL values were 0 g/d for red meat, processed meat and sweetened beverages, and 150, 325, 300, 95, 14.5 and 430 g/d, respectively, for whole grain products, fruits, vegetables, legumes, nuts and seeds, and milk (32). In our study, the HR criterion was thus expressed and minimized as:
where i denotes the food groups to be decreased (red meat, processed meat and sweetened beverages), j denotes the food groups to be increased (whole grain products, fruits, vegetables, legumes, nuts and seeds, and milk), Opt(i) and Opt(j) are the optimized consumptions of food groups i and j, respectively (in g/d), Max(i) is the upper limit of consumption of food group i (in g/d), TMREL(j) is the TMREL value of food group j (in g/d), DALYs(i) and DALYs(j) are the disability-adjusted life-years (DALYs) associated with excessive or insufficient consumptions of food groups i and j, respectively (in y), and DALYs(all) is the sum of all DALYs(i) and DALYs(j). The Max values used were the maximal recommended consumption of unhealthy foods in line with the French dietary guidelines (33): 71 g/d for red meat, 25 g/d for processed meat and 263 g/d (corresponding to the average portion size) for sweetened beverages intake. The TMREL and DALYs values used were issued from the most recent (2019) estimates from the GBD (32) adapted to our study context (by using sex-specific and French DALYs values, Supplementary Table S2).
We also evaluated how the modeled diets deviated from current diets, in order to consider inertia to changes in food consumption, which is one way to account for social/cultural acceptability. The Diet Departure (DD) criterion was defined as the sum of the squares of the differences between observed and optimized food group consumption, standardized by their observed standard deviations, as previously explained (31). DD was thus expressed and minimized as:
where k is the number of food groups (n = 45), Obs(k) and Opt(k) are, respectively, the observed and optimized consumption of food group k (in g/d) and SD(k) is the current standard deviation of the consumption of food group k.
Constraints
During diet optimization, the total energy intake was constrained to stay within ±5% of its observed value. Thirty-five nutritional constraints were applied to ensure adequate nutrient intake in the male and female populations (Supplementary Table S3), based on the most recent reference values from the French Agency for Food, Environmental and Occupational Health & Safety (ANSES) (34). We did not consider any constraints for vitamin D, because its reference value is known to be much too high to be reached by a non-fortified diet alone (31, 33). As the absorption of iron and zinc is dependent on dietary factors, the requirements were based on bioavailable iron and zinc calculated from the dietary intake using equations that predict their absorption (35–37), as detailed in a previous study by our group (31). This previous study had demonstrated that current recommendations regarding bioavailable iron and zinc are very constraining when trying to model healthier diets, these recommendations being much higher than current intakes (e.g., there is a current iron-deficiency anemia prevalence of 4.1% in French women) (31). Therefore, like in this previous study, we used threshold values lower than current reference values. They correspond to a deficiency prevalence of 5%, because such flexibility enables the identification of diets that are apparently healthier overall, with a better balance in DALYs due to less cardiometabolic disease, despite a higher prevalence of iron-deficiency anemia (31). In addition, to take into account the slightly lower digestibility of plant vs animal proteins regarding the nutritional constraint on protein requirement, a 5% penalty was applied to protein intake from plant protein food items, as previously described (38). As the intake of individual amino acids is generally adequate when the protein intake is sufficient in a varied diet (39), only protein requirements were considered in the model constraints, but we have a posteriori verified that modeled diets also contained adequate intakes of indispensable amino acids by using a database of the amino acid composition of food groups (Supplementary Information Text S1).
Moreover, some acceptability constraints were applied to the food group consumption (Supplementary Table S4). Acceptability constraints aimed to keep the food group intakes within the range of observed intakes, by bounding each food group intake between its 5th and 95th percentile of observed consumption in males and females separately. We did not do this for the unhealthy food groups or categories (red meat, processed meat and sweetened beverages), for which a dietary constraint with an upper limit was already defined according to the French dietary guidelines. Another exception was made for some healthy food groups (legumes and milk) that had 95th percentile values slightly lower than TMREL values, and for which the upper limit has thus been raised to their TMREL values.
Optimization strategy
We firstly aimed to determine the range of adequate %PP values in the diet that would ensure nutrient adequacy with a minimal long-term health risk. This first problem of identifying the adequate %PP range was addressed by optimizing the HR criterion under all the nutritional and acceptability constraints, with an additional constraint on %PP that was iteratively parameterized according to a grid search. This grid search constraint forced the %PP value to be equal to x%, with x% varying from 0 to 100% by steps of 5% (or even 1% at the edges of the adequate %PP range). As this problem was often non-uniquely identifiable, leading to different solutions with slightly distinct dietary patterns but similar HR values (especially for the intermediate %PP values that allowed for a variety of food group combinations with a similarly null HR value), we choose to systematically select the dietary solution that was the most acceptable a priori, based on the lowest departure from the current diet. According to the hierarchical method in multi-criteria optimization (40), this second problem of diet selection was addressed in a second stage. This time it was done by optimizing the DD criterion under the constraint that HR was equal to its previously identified minimal value, always under all the nutritional and acceptability constraints, and the grid search constraint on %PP covering its previously identified adequate range.
Limiting nutrients and contribution of food groups to their intake
We conducted a dual value analysis to better characterize the tensions between %PP, nutrient adequacy and long-term health. We reported the dual values associated with the %PP equality constraint and the nutritional constraints during HR optimization (obtained during the first problem solving, as explained above), which represent the potential HR gain if the limiting bound (lower or upper) of the considered constraint was relaxed by one unit. In order to compare the relative influence of nutrients, their dual values were standardized to represent the potential HR gain if the limiting bound was relaxed by 10%, to classify nutrients from the most limiting (higher absolute standardized dual value) to the least limiting (lowest absolute standardized dual value).
For the most limiting nutrients in the different modeled diets (i.e., nutrients with the most active constraints), we studied contributions of different food groups to intake of that particular nutrient in each modeled diet identified for each adequate %PP value (i.e., in the modeled diets resulting from the second problem solving, as explained above).
Sensitivity analysis
We also conducted a sensitivity analysis to assess the influence of some constraints of particular interest. We thus compared the results obtained when requiring the deficiency prevalence to be ≤1% rather than ≤5% (main model) in the nutritional constraints for bioavailable iron and zinc (their alternative threshold values are given in Supplementary Table S3), and when removing or not (main model) all the dietary and acceptability constraints on food group intakes.
Diet environmental impacts
Finally, to assess environmental pressures related to the observed and modeled diets, we used the French agricultural life cycle inventory database AGRIBALYSE® v3.1; its methodological approach (summarized in Supplementary Information Text S2) has been described elsewhere (41–43). In particular, we evaluated the food-related GHGe (in kg CO2eq, with the non-CO2 GHGe included and weighted according to their relative impact on warming), land use (referring to the use and transformation of land, dimensionless), water use (relating to the local scarcity of water, in m3 water deprivation) and fossil resource use (use of non-renewable fossil resources such as coal, oil, and gas, in MJ), together with a single environmental footprint score (dimensionless) that aggregated 16 indicators (44).
Results
Range of adequate %PP values and identified tensions between %PP, nutrient adequacy and long-term health
The adequate %PP range compatible with nutrient adequacy was 16–82% in males and 16–77% in females, and only the 25–70% %PP range was additionally compatible with a minimal health risk (HR criterion) for both sexes (Table 1). In this narrower range, a null HR value was attained by the removal of unhealthy foods (red meat, processed meat and sweetened beverages) and an increase in healthy foods (whole grain products, fruits, vegetables, legumes, nuts and seeds, and milk) up to or above their TMREL values (32).
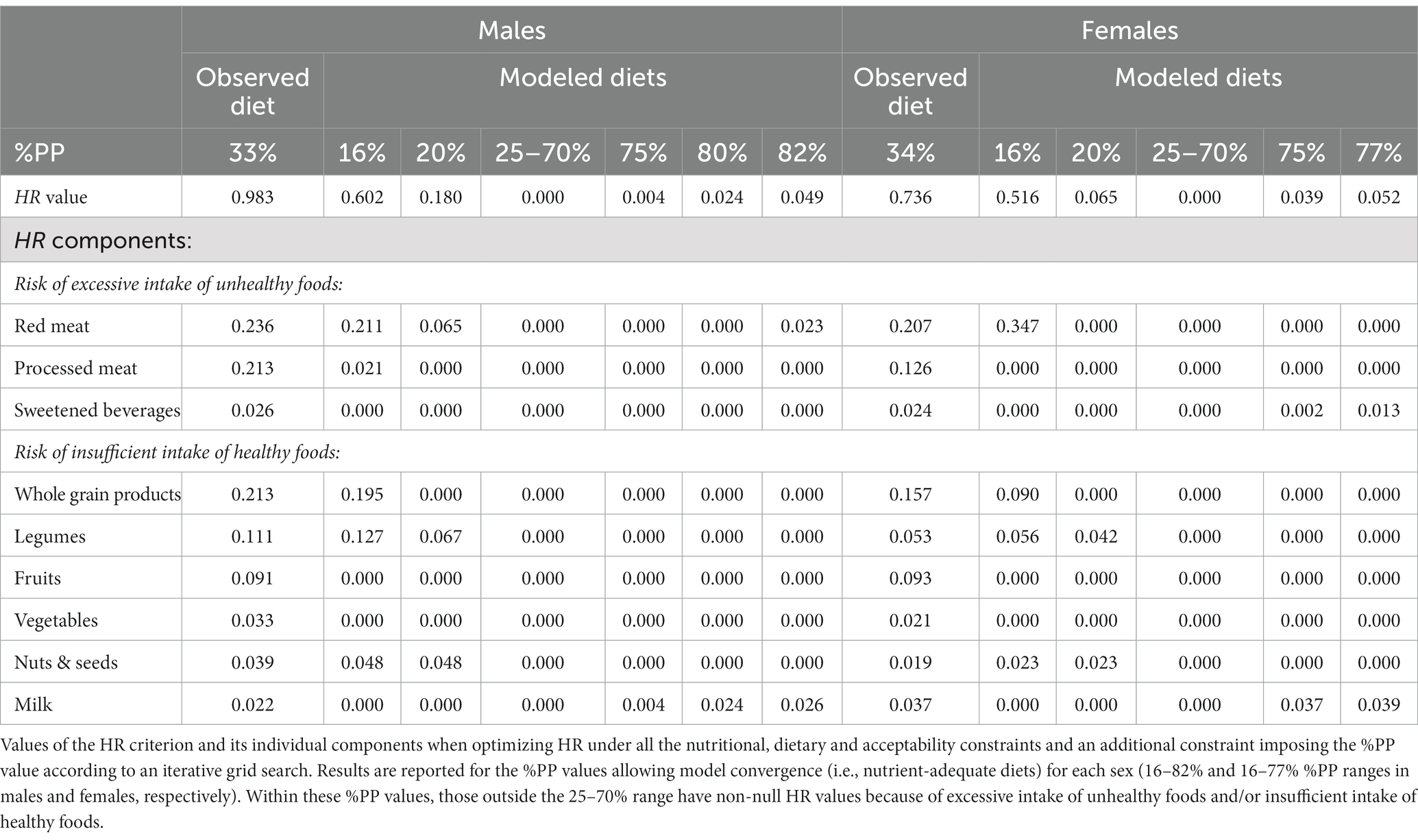
Table 1. Range of adequate values of the percentage of plant protein in the diet (%PP) and corresponding minimal values of long-term health risk (HR criterion) in French males and females.
Among the %PP equality constraint and the nutritional constraints, none were found limiting for HR minimization over the 25–70% %PP range (Table 2). The %PP equality constraint was limiting only for %PP values lower than 25% (strongly) and higher than 70% (more moderately, due to the lower HR impact of the milk decrease for the highest %PP values than of the red meat increase and whole grain product decrease for the lowest %PP values). Nutrients identified as increasingly limiting as %PP decreased below 25% were fiber, sugar (excluding lactose), saturated fatty acids and atherogenic fatty acids (lauric, myristic and palmitic acids). As %PP decreased below 25%, it was hence increasingly challenging to maintain sufficient intake of fiber and non-excessive intakes of sugar and fatty acids (as shown by the opposite sign of their dual values), which resulted in dietary solutions of increasingly degraded HR values. Nutrients that were identified as increasingly limiting when %PP increased above 70% were iodine, sodium, vitamin B2, calcium, EPA + DHA, vitamin A and α-linolenic acid in both sexes together with vitamin B12 in males and bioavailable iron in females. As %PP increased above 70%, it was increasingly challenging to maintain sufficient intakes of these nutrients and a non-excessive sodium intake. The other nutrients (n = 20, those not shown in Table 2) were never limiting over the adequate %PP range, including, of note, protein.
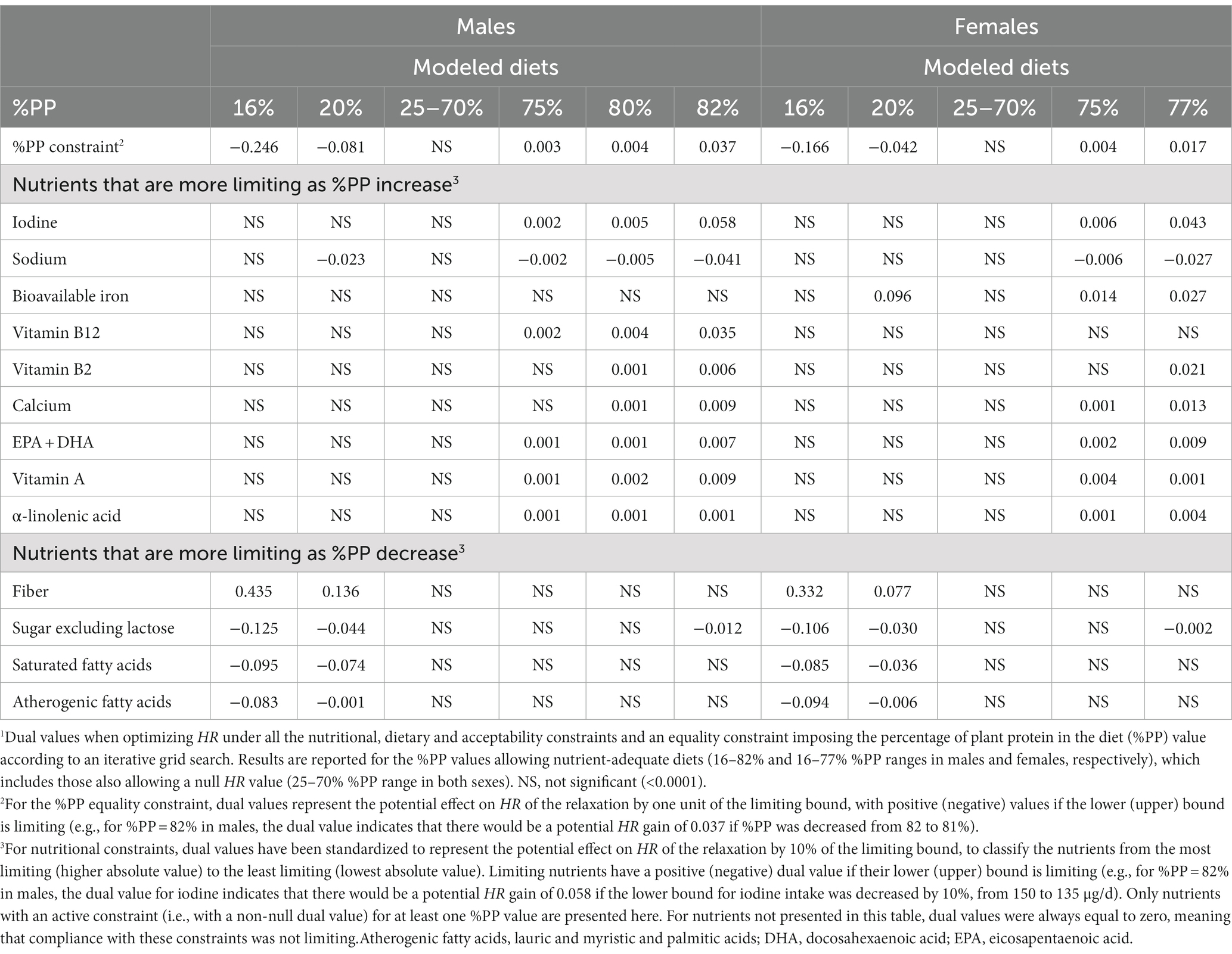
Table 2. Dual values of the active constraints identified during minimization of the long-term health risk (HR criterion) in French males and females1.
Sensitivity analysis showed that being more demanding for bioavailable iron and zinc (i.e., constraining their deficiency prevalence at ≤1% rather than ≤5% as in the main model) resulted in slightly restricting the adequate %PP range on the right (16–79% in males and 16–70% in females), and the %PP range ensuring a null HR value on both sides (30–65% in males and 35–45% in females) (data not shown). Conversely, when suppressing all the food group consumption limits (i.e., all the dietary and acceptability constraints) from the model (Supplementary Table S5), the range of adequate %PP values was expanded on both sides (8–94% in males and 8–92% in females), as was the %PP range ensuring a null HR value (16–86% in males and 16–84% in females), but consistently with the same limiting nutrients as in the main model (in particular, insufficient fiber intake for excessively low %PP values, or insufficient intakes of vitamin B12, iodine and EPA + DHA for excessively high %PP values).
Modeled diets
All the modeled diets identified (Figure 1; Supplementary Figure S2) were very distant from the current typical French diets, with departure values (DD criterion) equal to or greater than twice the standard deviation observed in the population. Furthermore, most of the modeled diets with a null HR value (i.e., in the 25–70% %PP range) were all about equally distant from the observed diets, with close DD values (differing by less than 20%) in the 35–65% %PP range and similar DD values (differing by less than 5%) in the 45–60% %PP range.
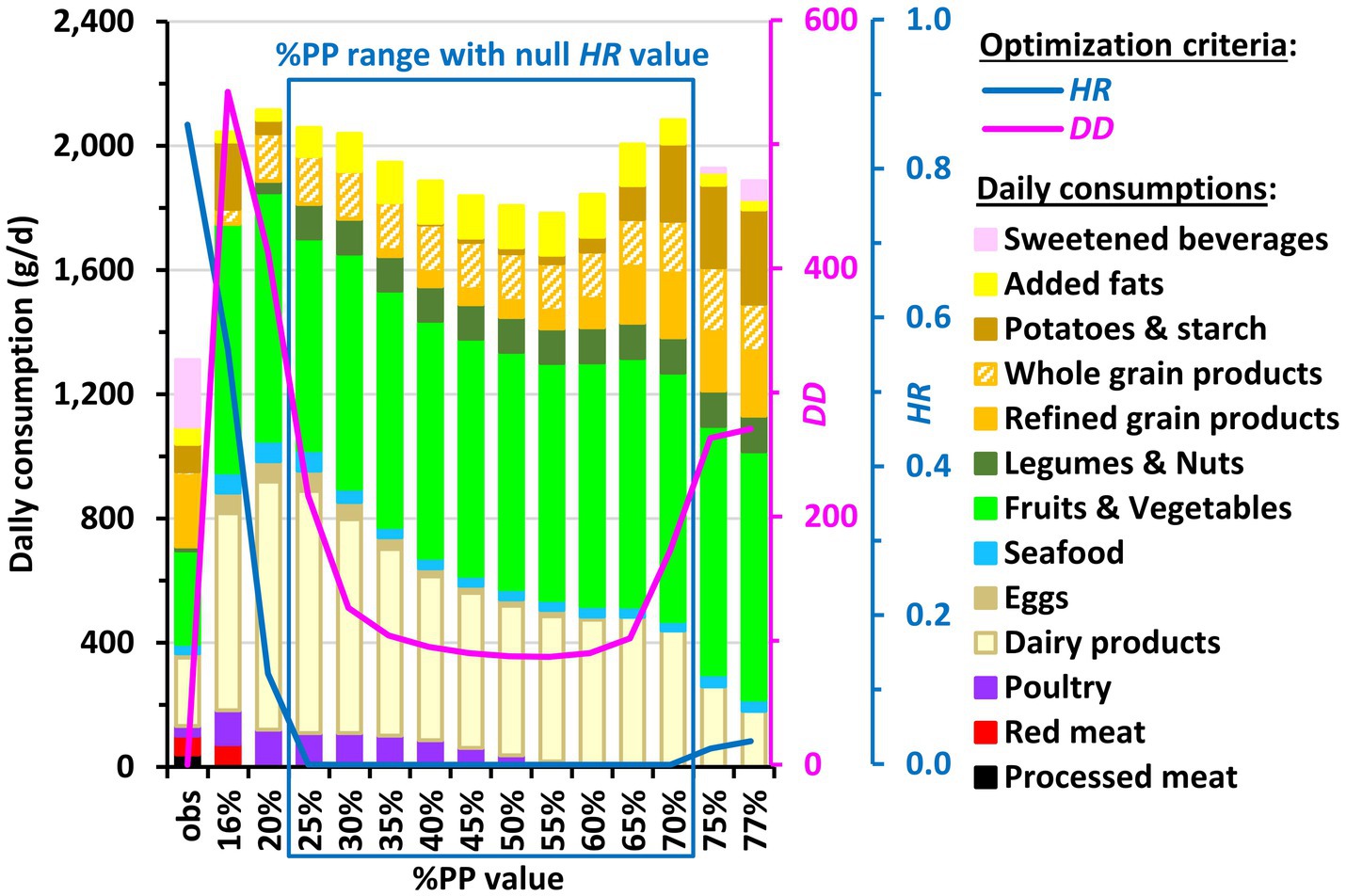
Figure 1. Daily food category consumption in the observed diets (obs) and modeled diets obtained by long-term health risk (HR) and diet departure (DD) minimization under imposed percentage of plant protein in the diet (%PP) in French adults. Results are reported for all the adequate %PP values ensuring nutrient adequacy (16–77%), which includes those also ensuring a null HR value (25–70%). The Bar charts represent the cumulative consumptions of food categories (black axis on the left) and the curves represent the HR and DD values (blue and pink axes on the right, respectively). For clarity, the 45 modeled food groups are not represented here but grouped into broader categories that are included in HR (such as red and processed meats) or represent other protein sources (such as poultry and seafood). Consumption of water, hot beverages, alcohol and miscellaneous foods are not shown for clarity. Details about food grouping and consumptions of food categories not shown here are given in Supplementary Tables S1, S6, respectively.
Although the energy intake remained relatively stable between modeled and observed diets (by construction), the total intakes of both animal-based and plant-based foods were increased in the 25–70% %PP range, notably owing to the important increases in milk, fruits and vegetables up to or above their TMREL values (Supplementary Table S6; Supplementary Figure S3). Regarding plant products, all the modeled diets exhibited dramatic increases in fruits and vegetables, whole grain products and legumes and nuts. Regarding animal products, red and processed meats were readily removed as %PP increased. These meats were replaced by poultry and eggs, which transiently increased, the modeled diets then being meat-free from PP% = 60%. Dairy and seafood were the only remaining animal products at the right end of the adequate %PP range (Supplementary Figure S3).
Over the entire adequate %PP range, including meat-free diets, the intakes of protein and of each indispensable amino acid were always much higher than their 98% safe intake thresholds (Figure 2; Supplementary Table S7; Supplementary Figure S4).
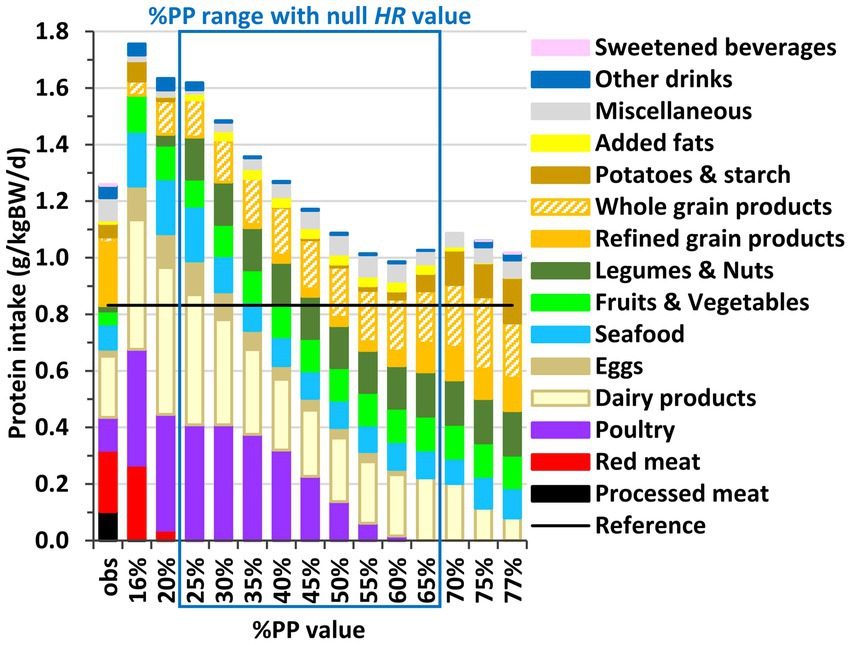
Figure 2. Contribution of food categories to protein intake in the observed diets (obs) and modeled diets obtained by long-term health risk (HR) and diet departure minimization under imposed percentage of plant protein in the diet (%PP) in French adults. Results are reported for all the adequate %PP values ensuring nutrient adequacy (16–77%), which includes those ensuring also a null HR value (25–70%). Sections inside the bars represent the contributions of food categories to protein intake (in g of protein/kg of BW/d). See Supplementary Table S1 for the detailed composition of food categories.
Contributions of food groups to limiting nutrient intakes
Regardless of their %PP value, all the modeled diets were nutrient-adequate, in contrast with observed diets (Supplementary Table S8).
As %PP increased, it was increasingly difficult to maintain sufficient intakes of bioavailable iron, vitamins B12, B2 and A, and iodine and calcium, owing to the decreases in the animal products that were their main contributors (red meat, dairy products and eggs) (Supplementary Figure S5). The EPA + DHA and α-linolenic acid intakes, which are largely insufficient in the observed diets, were made sufficient in all the modeled diets by increases in their main contributors, respectively, seafood and added fats, with difficulties to maintain them sufficient for the highest %PP values (Supplementary Figure S5). The sodium intake, which is dramatically excessive in the observed diets, was reduced to its upper limit in all the modeled diets as a result of removing processed meat and reducing refined grain products, with difficulties to maintain sodium not excessive for the highest %PP values, due to increases in some starch and miscellaneous foods (Supplementary Figure S5). Conversely, as %PP decreased, it was increasingly difficult to maintain a sufficient intake of fiber and non-excessive intakes of sugar and saturated fatty acids, due to the meat and dairy increases (Supplementary Figure S6).
Environmental impacts of modeled diets
Across modeled diets, GHGe gradually decreased as %PP increased until %PP = 70%, where the GHGe were ~ 50% lower than with the observed diet (Figure 3). Similar trends were observed for land use and, to a lesser extent, fossil resource use (Supplementary Figure S7), with 40% and ~ 20% decreases, respectively, from the observed to the modeled diet with %PP = 70%. In contrast, water use was ~25–50% higher for the null-HR modeled diets than for the observed diet, due to their very high levels of fruits and vegetables that were by far the most water-demanding food groups (Supplementary Figure S7). Overall, at the level of the single environmental footprint score that aggregated 16 indicators, the same trend was observed as for GHGe, with a 37% decrease in this aggregated score from the observed to the modeled diet with %PP = 70% (Supplementary Figure S7).
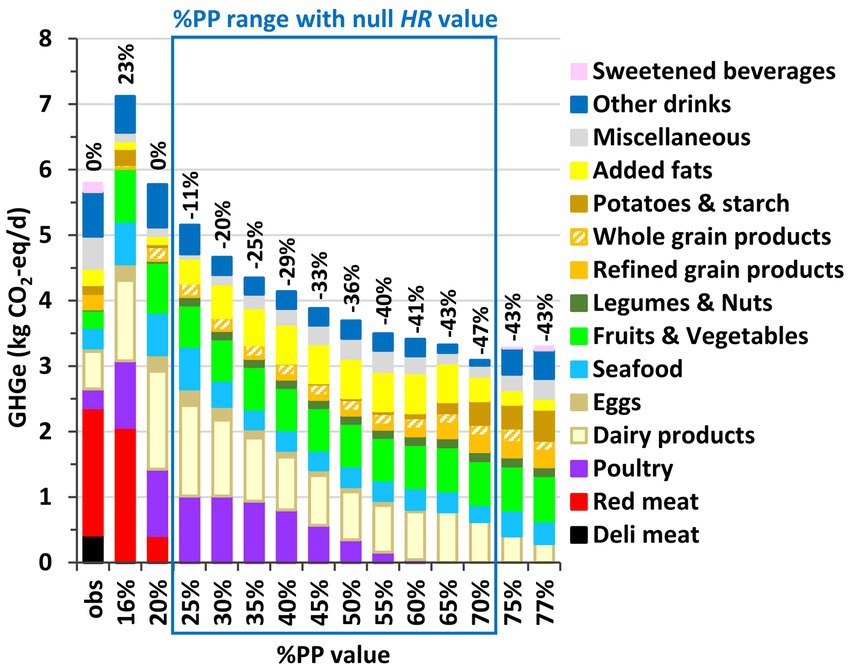
Figure 3. Greenhouse gas emissions (GHGe) associated with the observed (obs) and modeled diets obtained by long-term health risk (HR) and diet departure minimization under imposed percentage of plant protein in the diet (%PP) in French adults. Results are reported for all the adequate %PP values ensuring nutrient adequacy (16–77%), which includes those ensuring also a null HR value (25–70%). Sections inside the bars represent the contributions of food categories to GHGe (in kg CO2-eq/d), and values above the bars represent the relative deviation in GHGe from its observed value (in %). See Supplementary Table S1 for the detailed composition of food categories.
Discussion
Gathering all nutritional information over a large spectrum that covered nutrient reference values and long-term health risks, our study formally establishes ranges of plant protein proportion (%PP) for nutrient-adequate and healthy diets. One major finding is that there is no optimal %PP value, as we found a spectrum of similarly healthy diets over the 25–70% range. However, diets in the upper end were associated with substantially lower GHGe and overall environmental impact.
A wide dietary %PP range, from ~15 to 80%, was found compatible with providing all nutrients in adequate amounts. Our results do not agree with those of Vieux et al., who recently argued that %PP must be <50% to ensure nutritional adequacy (45). However, in this diet optimization study, solutions with %PP >50% were rejected not because of their true intrinsic inability to meet nutrient requirements but because of an incorrect problem formulation, as we recently pointed out (46). Furthermore, by not analyzing how the constraints considered affected the results and by not identifying the limiting nutrients, this work was not informative about the nutritional barriers to increasing %PP, which was our concern here along with its other health and environmental impacts. In our study, as shown by sensitivity analysis, the wide %PP range identified as compatible with nutritional adequacy was slightly restrained by the considered constraints for food acceptability, whereas the nutritional issues identified remained broadly the same with or without these constraints. From a nutritional viewpoint, no diet with %PP < ~15% was able to provide enough fiber and non-excessive amounts of saturated fatty acids, while also satisfying all constraints for nutrient intakes and food acceptability. In these too-low %PP diets, inadequate fiber intake was due to insufficient consumption of whole grains, legumes, and nuts, which were the most critical plant protein sources with intakes below their minimum-risk exposure levels. More interestingly, given the ongoing dietary transition, we could not find diets with %PP > ~80% that would provide sufficient amounts of a large set of nutrients, particularly iodine, vitamin B12 (in males), bioavailable iron (in females), calcium and EPA + DHA. These nutrients are considered to be at issue in vegetarian diets (except calcium and iodine in lacto-ovo-vegetarian), notably calcium and B12 in predominantly plant-based diets (47, 48). From a dietary viewpoint, as shown when approaching the critical value of %PP = 80%, dairy appeared to be key to preventing iodine and calcium shortages. Seafood, meanwhile, appeared critical to providing EPA + DHA (with oily fishes as the main source) as well as iodine and B12. Milk and seafood were the last remaining animal products at the highest %PP values, confirming their importance as healthy, nutrient-dense protein sources (49). Healthy plant protein sources such as legumes and nuts apparently could not replace milk or seafood. This is because they actually reached their upper allowed intake very early (as soon as %PP = 25% for legumes), which indicates that they constitute an effective dietary lever. However, even when removing all food intake limits (in sensitivity analysis), it remained impossible to obtain 100% plant-based diets because of the same nutritional issues (insufficient intakes of vitamin B12, iodine and EPA + DHA). Our findings do not indicate that vegetarian (without seafood) or vegan diets (without seafood and dairy) cannot be nutritionally adequate. It means that solutions for diets that are entirely or almost entirely plant-based should rely on additional food products than those presently consumed by the general population, including fortified foods (50–52). This warrants further studies about the potential of new foods to extend the limit of the %PP range identified as adequate here.
Within the wide range of nutrient-adequate %PP values, we did not find a single optimal diet, but a large range of diets with %PP from 25 to 70%. These diets were all optimal when considering their health value, because their food consumptions complied with minimum-risk exposure levels. These consisted of no red meat and high levels of fruits and vegetables, whole grains, legumes, nuts and milk, in line with dietary guidelines (53). Modeled healthy diets were variations of this pattern, which explains why they were also similarly distant from current diets. Within this healthy pattern spectrum, the increase in %PP was predominantly related to the decrease in total and animal proteins. This occurred mostly in poultry and eggs and, to a lesser extent, dairy. This finding aligns well with the current spectrum of observed diets, with plant-based diets being higher in plant protein but especially low in total and animal protein (54, 55). This could simply be ascribed to the higher protein density in animal protein sources compared to plant protein sources. Also, the nutrients identified as limiting at the borders of the healthy %PP range appear to be related to the nutrient density of animal vs. plant protein sources when expressed relative to protein density. However, the dietary protein amount was never limiting, even at the highest %PP levels. There is a growing consensus that the protein package and not the protein per se are important to the question of plant to animal protein ratio in the diet (6, 9). Likewise, indispensable amino acid amounts were well above reference values based on requirements. It is usually considered that dietary proteins, and in particular plant proteins tend to complement each other, because dietary proteins are not low in the same amino acids (56, 57). Lysine, which is the most critical amino acid, and is specifically low in grains is not limiting in the diet if grains are not the main source of protein in the diet (39). In real diets, composed of a mix of different types of proteins that complement each other, sufficient amounts of protein appear to guarantee sufficient amounts of amino acids (22, 55, 58).
Distance from the prevailing diets is often used in diet modeling to take into account so-called cultural acceptability (59–61), also referred to as dietary inertia (62). In this study, healthy diets in the 35–65% %PP range departed rather similarly from the prevailing diets, which are still at ~35% %PP. This confirms that the plant to animal protein ratio is, by itself, a poor descriptor of diet characteristics, and so blanket statements about the right %PP are not warranted. Given that modeled healthy diets ranging from 35% %PP (the level of the current diets in Western countries) to 65% %PP were all very distant from current diets, our study also shows that overcoming dietary inertia is required for healthy diets, irrespective of the plant to animal protein target ratio (63).
The GHGe and overall composite score for environmental pressures were lower for healthy modeled diets than observed diets, and all the more as %PP increased. A large body of literature has reported that diets which are more plant-based are associated with lower environmental pressure, and vice versa, whether diets were modeled (48, 61, 64, 65), observed (66–68) or composite (25). However, until our study, this relationship had not yet been shown according to %PP in healthy diets. In our setting, %PP was strongly associated with the environmental impact of healthy diets. As compared to the prevailing diets, lower GHGe and composite score are firstly explained by the removal of total red meat in all healthy diets, red meat accounting for ~1/3 of the pressure in prevailing diets. This is in line with the literature that points to red meat and associated sustainability concerns (65, 69). Finally, we found that other environmental pressures (land use and fossil resource use), except water use, had similar patterns of change, in line with the literature (21, 48). The general relationship between %PP and environmental pressure can mostly be ascribed to the fact that animal sources are rich in protein, and that livestock breeding is associated with higher resource use, higher land use, and higher GHGe (23, 70–72). Nevertheless, further investigation of the relationship between %PP and environmental impacts would require prioritizing the minimization of environmental impacts over that of diet departure. Therefore, we cannot rule out the possibility that moderate %PP diets, if well-designed, may have as low environmental impacts as high %PP diets, at the cost of a larger diet departure.
This study has some limitations. We modeled diets according to changes in intakes of food groups, based on the present food repertoire and current intake levels in the population. Food grouping is critical in diet modeling (33), and food diversity and composition can change rapidly in Western countries, as seen by recent changes (73). A similar limitation applies to the assessment of a diet’s environmental impacts, for which also we did not consider variations related to food production systems (74, 75). Nevertheless, we used a classical food grouping, which helps represent dietary patterns at an appropriately high level of detail. We also believe that using standard/traditional foods in modeling provides a good starting point to evaluate the situation before considering changes in the food offer or food composition. Our study uses sources of information as background parameters, including references/targets for nutrients and food categories. Clearly, there are many uncertainties in this regard (33). Nonetheless, we believe that a strength of our study is our use of a conceptual framework that aggregates most of the state of the art knowledge in nutrition.
To conclude, we identified that the range of equally optimal %PP values for nutrition and health is wide (25–70%), and that all of these healthy diets deviate greatly from prevailing diets. From a public health perspective, there is no unique, optimal %PP value when considering nutrition and health alone. However, significant changes in current eating habits are nonetheless required to achieve healthier diets. The focus should therefore shift from protein per se to what is carried with protein (i.e., the nutrient package), the overall health value of the food groups that convey protein, as well as the efforts needed to move away from current Western dietary patterns (22). Moreover, in the higher end of the adequate %PP range, modeled healthy diets have a lower environmental impact and are thus more sustainable than other healthy diets. Thus, in current and future dietary transitions, environmental pressures appear to be a more direct determinant than health objectives to justify increasing %PP levels. At %PP > ~80%, changes in food repertoire diversity, food composition, nutrient enrichment or nutrient supplementation are required for fully nutrient-adequate diets. Finally, the adequate %PP range may be narrower in some populations, such as the elderly, who may have higher protein requirements than the general adult population, and this would deserve further study.
Preprint
A manuscript was deposited as a preprint, to MedRxiv (10.1101/2022.05.20.22275349). The copyright holder for this preprint is the author, with all rights reserved and no reuse allowed without permission.
Data availability statement
The raw data supporting the conclusions of this article will be made available by the authors, without undue reservation.
Author contributions
HF and FM designed the research, wrote the first draft of the manuscript, and had primary responsibility for the final content. HF conducted the research and analyzed the data. AD, EP, FM, JW, J-FH, and EK-G provided methodological support and help with interpretation of the results. All authors provided critical comments on the manuscript, and read and approved the final manuscript.
Funding
This research did not receive any specific grant from funding agencies in the public, commercial, or not-for-profit sectors.
Acknowledgments
The authors thank Vincent Colomb and Mélissa Cornélius (Ademe) for their important support in the use of the AGRIBALYSE database.
Conflict of interest
HF has received a research grant by INRAE from Roquette; FM has received research grants as PhD fellowships under his direction by AgroParisTech and INRAE from Terres Univia and Ecotone foundation, under the aegis of Fondation de France.
The remaining authors declare that the research was conducted in the absence of any commercial or financial relationships that could be construed as a potential conflict of interest.
Publisher’s note
All claims expressed in this article are solely those of the authors and do not necessarily represent those of their affiliated organizations, or those of the publisher, the editors and the reviewers. Any product that may be evaluated in this article, or claim that may be made by its manufacturer, is not guaranteed or endorsed by the publisher.
Supplementary material
The Supplementary material for this article can be found online at: https://www.frontiersin.org/articles/10.3389/fnut.2023.1178121/full#supplementary-material
Abbreviations
ANSES, French Agency for Food, Environmental and Occupational Health and Safety; CIQUAL, French Information Centre on Food Quality; DALYs, Disability-adjusted life-years; DD, Diet departure criterion; GBD, Global Burden of Diseases; GHGe, greenhouse gas emissions; HR, Health risk criterion; INCA3, Third Individual and National Study on Food Consumption French Survey; %PP, Percentage of plant protein in the diet; TMREL, Theoretical minimum risk exposure level.
References
1. Styring, AK, Fraser, RA, Arbogast, R-M, Halstead, P, Isaakidou, V, Pearson, JA, et al. Refining human palaeodietary reconstruction using amino acid δ15N values of plants, animals and humans. J Archaeol Sci. (2015) 53:504–15. doi: 10.1016/j.jas.2014.11.009
2. World Health Organization (WHO). Diet, nutrition and the prevention of chronic diseases. 3. Global and regional food consumption patterns and trends. 3rd, (2003):1–149.
3. Gerbens-Leenes, PW. Dietary transition: longterm trends, animal versus plant energy intake, and sustainability issues In: F Mariotti, editor. Vegetarian and plant-based diets in health and disease prevention. London: Academic Press (2017). 117–34.
4. Phillips, SM, Fulgoni, VL 3rd, Heaney, RP, Nicklas, TA, Slavin, JL, and Weaver, CM. Commonly consumed protein foods contribute to nutrient intake, diet quality, and nutrient adequacy. Am J Clin Nutr. (2015) 101:1346S–52S. doi: 10.3945/ajcn.114.084079
5. Salome, M, Kesse-Guyot, E, Fouillet, H, Touvier, M, Hercberg, S, Huneau, JF, et al. Development and evaluation of a new dietary index assessing nutrient security by aggregating probabilistic estimates of the risk of nutrient deficiency in two French adult populations. Br J Nutr. (2021) 126:1225–36. doi: 10.1017/S0007114520005115
6. Mariotti, F. Animal and plant protein sources and cardiometabolic health. Adv Nutr. (2019) 10:S351–66. doi: 10.1093/advances/nmy110
7. Paivarinta, E, Itkonen, ST, Pellinen, T, Lehtovirta, M, Erkkola, M, and Pajari, AM. Replacing animal-based proteins with plant-based proteins changes the composition of a whole nordic diet-a randomised clinical trial in healthy Finnish adults. Nutrients. (2020) 12:943–59. doi: 10.3390/nu12040943
8. Lepine, G, Fouillet, H, Remond, D, Huneau, JF, Mariotti, F, and Polakof, S. A scoping review: metabolomics signatures associated with animal and plant protein intake and their potential relation with cardiometabolic risk. Adv Nutr. (2021) 12:2112–31. doi: 10.1093/advances/nmab073
9. Richter, CK, Skulas-Ray, AC, Champagne, CM, and Kris-Etherton, PM. Plant protein and animal proteins: do they differentially affect cardiovascular disease risk? Adv Nutr. (2015) 6:712–28. doi: 10.3945/an.115.009654
10. Dietrich, S, Trefflich, I, Ueland, PM, Menzel, J, Penczynski, KJ, Abraham, K, et al. Amino acid intake and plasma concentrations and their interplay with gut microbiota in vegans and omnivores in Germany. Eur J Nutr. (2022) 61:2103–14. doi: 10.1007/s00394-021-02790-y
11. Naghshi, S, Sadeghi, O, Willett, WC, and Esmaillzadeh, A. Dietary intake of total, animal, and plant proteins and risk of all cause, cardiovascular, and cancer mortality: systematic review and dose-response meta-analysis of prospective cohort studies. BMJ. (2020) 370:m2412. doi: 10.1136/bmj.m2412
12. Zhong, VW, Allen, NB, Greenland, P, Carnethon, MR, Ning, H, Wilkins, JT, et al. Protein foods from animal sources, incident cardiovascular disease and all-cause mortality: a substitution analysis. Int J Epidemiol. (2021) 50:223–33. doi: 10.1093/ije/dyaa205
13. Salomé, M, de Gavelle, E, Dufour, A, Dubuisson, C, Volatier, JL, Fouillet, H, et al. Plant-protein diversity is critical to ensuring the nutritional adequacy of diets when replacing animal with plant protein: observed and modeled diets of French adults (INCA3). J Nutr. (2020) 150:536–45. doi: 10.1093/jn/nxz252
14. Malik, VS, Li, Y, Tobias, DK, Pan, A, and Hu, FB. Dietary protein intake and risk of type 2 diabetes in US men and women. Am J Epidemiol. (2016) 183:715–28. doi: 10.1093/aje/kwv268
15. Tharrey, M, Mariotti, F, Mashchak, A, Barbillon, P, Delattre, M, and Fraser, GE. Patterns of plant and animal protein intake are strongly associated with cardiovascular mortality: the Adventist Health Study-2 cohort. Int J Epidemiol. (2018) 47:1603–12. doi: 10.1093/ije/dyy030
16. Guasch-Ferre, M, Satija, A, Blondin, SA, Janiszewski, M, Emlen, E, O'Connor, LE, et al. Meta-analysis of randomized controlled trials of red meat consumption in comparison with various comparison diets on cardiovascular risk factors. Circulation. (2019) 139:1828–45. doi: 10.1161/CIRCULATIONAHA.118.035225
17. Farvid, MS, Sidahmed, E, Spence, ND, Mante Angua, K, Rosner, BA, and Barnett, JB. Consumption of red meat and processed meat and cancer incidence: a systematic review and Meta-analysis of prospective studies. Eur J Epidemiol. (2021) 36:937–51. doi: 10.1007/s10654-021-00741-9
18. Huang, Y, Cao, D, Chen, Z, Chen, B, Li, J, Guo, J, et al. Red and processed meat consumption and cancer outcomes: Umbrella review. Food Chem. (2021) 356:129697. doi: 10.1016/j.foodchem.2021.129697
19. Havemeier, S, Erickson, J, and Slavin, J. Dietary guidance for pulses: the challenge and opportunity to be part of both the vegetable and protein food groups. Ann N Y Acad Sci. (2017) 1392:58–66. doi: 10.1111/nyas.13308
20. Comerford, KB, Miller, GD, Reinhardt Kapsak, W, and Brown, KA. The complementary roles for plant-source and animal-source foods in sustainable healthy diets. Nutrients. (2021) 13:3469. doi: 10.3390/nu13103469
21. Clark, MA, Springmann, M, Hill, J, and Tilman, D. Multiple health and environmental impacts of foods. Proc Natl Acad Sci U S A. (2019) 116:23357–62. doi: 10.1073/pnas.1906908116
22. Katz, DL, Doughty, KN, Geagan, K, Jenkins, DA, and Gardner, CD. Perspective: the public health case for modernizing the definition of protein quality. Adv Nutr. (2019) 10:755–64. doi: 10.1093/advances/nmz023
23. Xu, XM, Sharma, P, Shu, SJ, Lin, TS, Ciais, P, Tubiello, FN, et al. Global greenhouse gas emissions from animal-based foods are twice those of plant-based foods. Nat Food. (2021) 2:724–32. doi: 10.1038/s43016-021-00358-x
24. Jarmul, S, Dangour, AD, Green, R, Liew, Z, Haines, A, and Scheelbeek, PFD. Climate change mitigation through dietary change: a systematic review of empirical and modelling studies on the environmental footprints and health effects of ‘sustainable diets’. Envir Res Lett. (2020) 15:123014. doi: 10.1088/1748-9326/abc2f7
25. Reinhardt, SL, Boehm, R, Blackstone, NT, El-Abbadi, NH, McNally Brandow, JS, Taylor, SF, et al. Systematic review of dietary patterns and sustainability in the United States. Adv Nutr. (2020) 11:1016–31. doi: 10.1093/advances/nmaa026
26. Kesse-Guyot, E, Rebouillat, P, Brunin, J, Langevin, B, Alles, B, Touvier, M, et al. Environmental and nutritional analysis of the EAT-Lancet diet at the individual level: insights from the NutriNet-Sante study. J Clean Prod. (2021) 296:126555. doi: 10.1016/j.jclepro.2021.126555
27. Willett, W, Rockstrom, J, Loken, B, Springmann, M, Lang, T, Vermeulen, S, et al. Food in the anthropocene: the EAT-Lancet Commission on healthy diets from sustainable food systems. Lancet. (2019) 393:447–92. doi: 10.1016/S0140-6736(18)31788-4
28. Tuomisto, HL. Importance of considering environmental sustainability in dietary guidelines. Lancet Planet Health. (2018) 2:e331–2. doi: 10.1016/S2542-5196(18)30174-8
29. Dubuisson, C, Dufour, A, Carrillo, S, Drouillet-Pinard, P, Havard, S, and Volatier, JL. The Third French Individual and National Food Consumption (INCA3) Survey 2014-2015: method, design and participation rate in the framework of a European harmonization process. Public Health Nutr. (2019) 22:584–600. doi: 10.1017/S1368980018002896
30. Ciqual. The French Agency for Food Environmental and Occupational Health & Safety (ANSES). ANSES-CIQUAL French food composition table. Version 2016 (2016). Available at: https://ciqual.anses.fr/
31. Dussiot, A, Fouillet, H, Wang, J, Salome, M, Huneau, JF, Kesse-Guyot, E, et al. Modeled healthy eating patterns are largely constrained by currently estimated requirements for bioavailable iron and zinc-a diet optimization study in French adults. Am J Clin Nutr. (2022) 115:958–69. doi: 10.1093/ajcn/nqab373
32. GBD Risk Factors Collaborators. Global burden of 87 risk factors in 204 countries and territories, 1990-2019: a systematic analysis for the Global Burden of Disease Study 2019. Lancet. (2020) 396:1223–49. doi: 10.1016/S0140-6736(20)30752-2
33. Mariotti, F, Havard, S, Morise, A, Nadaud, P, Sirot, V, Wetzler, S, et al. Perspective: modeling healthy eating patterns for food-based dietary guidelines-scientific concepts, methodological processes, limitations, and lessons. Adv Nutr. (2021) 12:590–9. doi: 10.1093/advances/nmaa176
34. ANSES. The French Agency for Food Environmental and Occupational Health & Safety (ANSES). Avis de l’ANSES relatif à l'Actualisation des références nutritionnelles françaises en vitamines et minéraux. Saisine n°2018-SA-0238, saisine liée n°2012-SA-0103. Maisons-Alfort. (2021). Available at: https://www.anses.fr/fr/system/files/NUT2018SA0238Ra.pdf
35. Armah, SM, Carriquiry, A, Sullivan, D, Cook, JD, and Reddy, MB. A complete diet-based algorithm for predicting nonheme iron absorption in adults. J Nutr. (2013) 143:1136–40. doi: 10.3945/jn.112.169904
36. Hallberg, L, and Hulthen, L. Prediction of dietary iron absorption: an algorithm for calculating absorption and bioavailability of dietary iron. Am J Clin Nutr. (2000) 71:1147–60. doi: 10.1093/ajcn/71.5.1147
37. Miller, LV, Krebs, NF, and Hambidge, KM. Mathematical model of zinc absorption: effects of dietary calcium, protein and iron on zinc absorption. Br J Nutr. (2013) 109:695–700. doi: 10.1017/S000711451200195X
38. de Gavelle, E, Huneau, JF, and Mariotti, F. Patterns of protein food intake are associated with nutrient adequacy in the general French adult population. Nutrients. (2018) 10:226. doi: 10.3390/nu10020226
39. de Gavelle, E, Huneau, JF, Bianchi, CM, Verger, EO, and Mariotti, F. Protein adequacy is primarily a matter of protein quantity, not quality: modeling an increase in plant: animal protein ratio in French adults. Nutrients. (2017) 9:1333. doi: 10.3390/nu9121333
40. Mausser, H, Grodzevich, O, and Romanko, O. Normalization and other topics in multi-objective optimization. Proceedings of the fields–MITACS industrial problems workshop, (2006) 89–101.
41. ADEME. Agribalyse data v3.1 ADEME (2022) Available at: https://doc.agribalyse.fr/documentation-en/.
42. Colomb, V, Amar, SA, Mens, CB, Gac, A, Gaillard, G, Koch, P, et al. AGRIBALYSE (R), the French LCI Database for agricultural products: high quality data for producers and environmental labelling. OCL-Oilseeds Fats Crops Lipids. (2015) 22:D104. doi: 10.1051/ocl/20140047
43. Koch, P, and Salou, T. AGRIBALYSE®: Rapport Méthodologique – Volet Agriculture – Version 3.1. Angers, France. ADEME. (2022). Available at: https://3613321239-files.gitbook.io/~/files/v0/b/gitbook-x-prod.appspot.com/o/spaces%2F-LpO7Agg1DbhEBNAvmHP%2Fuploads%2FQN0ySICvr4UDCrC3JbmE%2FM%C3%A9thodologie%20AGB%203.1_Production%20agricole.pdf?alt=media&token=4da12058-cd06-4948-9d20-d7fa7f371835
44. European Commission. Guidance for the development of Product Environmental Footprint Category Rules (PEFCRs). version 6.3. (2018). Available at: https://ec.europa.eu/environment/eussd/smgp/pdf/PEFCR_guidance_v6.3.pdf
45. Vieux, F, Remond, D, Peyraud, JL, and Darmon, N. Approximately half of total protein intake by adults must be animal-based to meet non-protein, nutrient-based recommendations, with variations due to age and sex. J Nutr. (2022) 152:2514–25. doi: 10.1093/jn/nxac150
46. Mariotti, F, Huneau, JF, and Fouillet, H. No nutritional lessons can be learned from a misspecified and overrestricted model with no sensitivity analysis. J Nutr. (2023) 153:911–12. doi: 10.1016/j.tjnut.2022.12.021
47. Bakaloudi, DR, Halloran, A, Rippin, HL, Oikonomidou, AC, Dardavesis, TI, Williams, J, et al. Intake and adequacy of the vegan diet. A systematic review of the evidence. Clin Nutr. (2021) 40:3503–21. doi: 10.1016/j.clnu.2020.11.035
48. Springmann, M, Wiebe, K, Mason-D'Croz, D, Sulser, TB, Rayner, M, and Scarborough, P. Health and nutritional aspects of sustainable diet strategies and their association with environmental impacts: a global modelling analysis with country-level detail. Lancet Planet Health. (2018) 2:e451–61. doi: 10.1016/S2542-5196(18)30206-7
49. Cifelli, CJ, Houchins, JA, Demmer, E, and Fulgoni, VL. Increasing plant based foods or dairy foods differentially affects nutrient intakes: dietary scenarios using NHANES 2007-2010. Nutrients. (2016) 8:422. doi: 10.3390/nu8070422
50. Mangano, KM, and Tucker, KL. Bone health and vegan diets In: F Mariotti, editor. Vegetarian and plant-based diets in health and disease prevention. London: Academic Press (2017). 315–31.
51. Thorpe, DL, Beeson, WL, Knutsen, R, Fraser, GE, and Knutsen, SF. Dietary patterns and hip fracture in the Adventist Health Study 2: combined vitamin D and calcium supplementation mitigate increased hip fracture risk among vegans. Am J Clin Nutr. (2021) 114:488–95. doi: 10.1093/ajcn/nqab095
52. Craig, WJ, Mangels, AR, Fresan, U, Marsh, K, Miles, FL, Saunders, AV, et al. The safe and effective use of plant-based diets with guidelines for health professionals. Nutrients. (2021) 13:4144. doi: 10.3390/nu13114144
53. Herforth, A, Arimond, M, Alvarez-Sanchez, C, Coates, J, Christianson, K, and Muehlhoff, E. A global review of food-based dietary guidelines. Adv Nutr. (2019) 10:590–605. doi: 10.1093/advances/nmy130
54. Halkjaer, J, Olsen, A, Bjerregaard, LJ, Deharveng, G, Tjonneland, A, Welch, AA, et al. Intake of total, animal and plant proteins, and their food sources in 10 countries in the European Prospective Investigation into Cancer and Nutrition. Eur J Clin Nutr. (2009) 63:S16–36. doi: 10.1038/ejcn.2009.73
55. Mariotti, F, and Gardner, CD. Dietary protein and amino acids in vegetarian diets-A review. Nutrients. (2019) 11:2661. doi: 10.3390/nu11112661
56. Mariotti, F. Plant protein, animal protein, and protein quality In: F Mariotti, editor. Vegetarian and plant-based diets in health and disease prevention. London: Academic Press (2017). 621–42.
57. Dimina, L, Remond, D, Huneau, JF, and Mariotti, F. Combining plant proteins to achieve amino acid profiles adapted to various nutritional objectives-an exploratory analysis using linear programming. Front Nutr. (2021) 8:809685. doi: 10.3389/fnut.2021.809685
58. Young, VR, and Pellett, PL. Plant proteins in relation to human protein and amino acid nutrition. Am J Clin Nutr. (1994) 59:1203S–12S. doi: 10.1093/ajcn/59.5.1203S
59. Gazan, R, Brouzes, CMC, Vieux, F, Maillot, M, Lluch, A, and Darmon, N. Mathematical optimization to explore tomorrow's sustainable diets: a narrative review. Adv Nutr. (2018) 9:602–16. doi: 10.1093/advances/nmy049
60. Macdiarmid, JI, Kyle, J, Horgan, GW, Loe, J, Fyfe, C, Johnstone, A, et al. Sustainable diets for the future: can we contribute to reducing greenhouse gas emissions by eating a healthy diet? Am J Clin Nutr. (2012) 96:632–9. doi: 10.3945/ajcn.112.038729
61. Seconda, L, Fouillet, H, Huneau, JF, Pointereau, P, Baudry, J, Langevin, B, et al. Conservative to disruptive diets for optimizing nutrition, environmental impacts and cost in French adults from the NutriNet-Sante cohort. Nat Food. (2021) 2:174–82. doi: 10.1038/s43016-021-00227-7
62. Webb, D, and Byrd-Bredbenner, C. Overcoming consumer inertia to dietary guidance. Adv Nutr. (2015) 6:391–6. doi: 10.3945/an.115.008441
63. Petersen, KS, Flock, MR, Richter, CK, Mukherjea, R, Slavin, JL, and Kris-Etherton, PM. Healthy dietary patterns for preventing cardiometabolic disease: the role of plant-based foods and animal products. Curr Dev Nutr. (2017) 1:cdn.117.001289. doi: 10.3945/cdn.117.001289
64. Springmann, M, Godfray, HC, Rayner, M, and Scarborough, P. Analysis and valuation of the health and climate change cobenefits of dietary change. Proc Natl Acad Sci U S A. (2016) 113:4146–51. doi: 10.1073/pnas.1523119113
65. Wilson, N, Cleghorn, CL, Cobiac, LJ, Mizdrak, A, and Nghiem, N. Achieving healthy and sustainable diets: a review of the results of recent mathematical optimization studies. Adv Nutr. (2019) 10:S389–403. doi: 10.1093/advances/nmz037
66. Kesse-Guyot, E, Chaltiel, D, Wang, J, Pointereau, P, Langevin, B, Allès, B, et al. Sustainability analysis of French dietary guidelines using multiple criteria. Nat Sustain. (2020) 3:377–85. doi: 10.1038/s41893-020-0495-8
67. Segovia-Siapco, G, and Sabate, J. Health and sustainability outcomes of vegetarian dietary patterns: a revisit of the EPIC-Oxford and the Adventist Health Study-2 cohorts. Eur J Clin Nutr. (2019) 72:60–70. doi: 10.1038/s41430-018-0310-z
68. Baudry, J, Pointereau, P, Seconda, L, Vidal, R, Taupier-Letage, B, Langevin, B, et al. Improvement of diet sustainability with increased level of organic food in the diet: findings from the BioNutriNet cohort. Am J Clin Nutr. (2019) 109:1173–88. doi: 10.1093/ajcn/nqy361
69. Nelson, ME, Hamm, MW, Hu, FB, Abrams, SA, and Griffin, TS. Alignment of healthy dietary patterns and environmental sustainability: a systematic review. Adv Nutr. (2016) 7:1005–25. doi: 10.3945/an.116.012567
70. Crippa, M, Solazzo, E, Guizzardi, D, Monforti-Ferrario, F, Tubiello, FN, and Leip, A. Food systems are responsible for a third of global anthropogenic GHG emissions. Nat Food. (2021) 2:198–209. doi: 10.1038/s43016-021-00225-9
71. Poore, J, and Nemecek, T. Reducing food's environmental impacts through producers and consumers. Science. (2018) 360:987–92. doi: 10.1126/science.aaq0216
72. Godfray, HCJ, Aveyard, P, Garnett, T, Hall, JW, Key, TJ, Lorimer, J, et al. Meat consumption, health, and the environment. Science. (2018) 361. doi: 10.1126/science.aam5324
73. Wickramasinghe, K, Breda, J, Berdzuli, N, Rippin, H, Farrand, C, and Halloran, A. The shift to plant-based diets: are we missing the point? Glob Food Sec. (2021) 29:100530. doi: 10.1016/j.gfs.2021.100530
74. Reganold, JP, and Wachter, JM. Organic agriculture in the twenty-first century. Nat Plants. (2016) 2:15221. doi: 10.1038/NPLANTS.2015.221
Keywords: healthy dietary patterns, nutrient adequacy, environmental footprints, diet optimization, plant-based diets
Citation: Fouillet H, Dussiot A, Perraud E, Wang J, Huneau J-F, Kesse-Guyot E and Mariotti F (2023) Plant to animal protein ratio in the diet: nutrient adequacy, long-term health and environmental pressure. Front. Nutr. 10:1178121. doi: 10.3389/fnut.2023.1178121
Edited by:
Rui Poínhos, University of Porto, PortugalReviewed by:
Anne Pihlanto, Natural Resources Institute Finland (Luke), FinlandAmanda Gomes Almeida Sá, Federal University of Santa Catarina, Brazil
Copyright © 2023 Fouillet, Dussiot, Perraud, Wang, Huneau, Kesse-Guyot and Mariotti. This is an open-access article distributed under the terms of the Creative Commons Attribution License (CC BY). The use, distribution or reproduction in other forums is permitted, provided the original author(s) and the copyright owner(s) are credited and that the original publication in this journal is cited, in accordance with accepted academic practice. No use, distribution or reproduction is permitted which does not comply with these terms.
*Correspondence: Hélène Fouillet, aGVsZW5lLmZvdWlsbGV0QGFncm9wYXJpc3RlY2guZnI=; François Mariotti, ZnJhbmNvaXMubWFyaW90dGlAYWdyb3BhcmlzdGVjaC5mcg==