- 1Buchinger Wilhelmi Clinic, Überlingen, Germany
- 2Department of Medical and Molecular Genetics, King’s College London, London, United Kingdom
- 3Buchinger Wilhelmi Clinic, Marbella, Spain
Background: Post-acute sequelae of a severe acute respiratory syndrome coronavirus 2 infection, also known as long COVID, comprises a variety of symptoms that impair the quality of life. This represents a growing public health burden, with millions of individuals worldwide affected.
Case description: We present a case series of 14 COVID-19 patients with post-acute symptoms who underwent medically supervised long-term fasting (6 to 16 days) according to the peer-reviewed Buchinger Wilhelmi protocol. The EQ-5D-5L questionnaire and visual scales were used to evaluate the intensity of the symptoms, retrospectively during the acute phase, and prospectively before and after long-term fasting. Blood tests were also performed before and after fasting. Thirteen patients reported that fasting caused an enhancement in their perceived overall health. Only one patient had no improvement. Both frequent (fatigue, breathlessness, muscle and joint pains) and less frequent (cognitive impairment, smell and taste disorders) sequelae ameliorated. Body weight and other risk factors for cardiometabolic diseases like blood pressure, blood glucose, total cholesterol, low-density-lipoprotein cholesterol, and triglycerides were reduced. No severe side effects occurred.
Discussion: This case series reports beneficial changes in self-perceived symptoms in patients with long COVID after long-term fasting. This highlights the potential of long-term fasting as an effective intervention for managing and treating long COVID.
1. Introduction
Long COVID, also known as “post-acute sequelae of COVID-19 (PASC),” is a condition that manifest after an incomplete recovery from a severe acute respiratory syndrome coronavirus 2 (SARS-CoV-2) infection in at least 10% of individuals (1). Symptoms are highly variable and can affect multiple systems, including respiratory, cardiovascular, neurological, gastrointestinal and musculoskeletal systems as well as mood disorders (2). The most frequent symptoms are fatigue, breathlessness, cognitive impairment and pain such as chest pain or headache (3, 4). Long COVID can develop in two phases depending on the persistence of symptoms. After the acute disease, ongoing symptoms can remain during 4 weeks to 3 months. If the symptoms persist for more than 3 months, the condition is classified as long COVID. According to the World Health Organisation (5), the symptoms of long COVID last for at least 2 months and cannot be explained by an alternative diagnosis. Thereby, the symptoms can either persist with possible fluctuations or emerge despite an initial recovery from an acute COVID-19 episode (5).
The SARS-CoV-2 virus primarily enters the cells through the angiotensin-converting enzyme 2 receptor, that is present in various cell types of the body, leading to an inflammatory response that impairs organ function (2). The underlying mechanisms of tissue damage in COVID-19 involve chronic inflammation (6, 7) and oxidative stress (8, 9), although we do not fully understand the mechanisms by which the infection leads to tissue damage. The subsequent development of long COVID is not fully predictable. Persistent viral antigens, the reactivation of human herpesviruses (e.g., Epstein–Barr), a higher virus load and the activation of autoreactive immunity are associated with immunologic perturbations that can predict the development of long COVID (1). Oxidative stress can lead to mitochondrial dysfunction, which impairs cellular energy homeostasis (8). The burst of reactive oxygen species caused by COVID-19 can lead to endothelial dysfunction. When this is combined with an elevated tendency for clotting, microclots can persist in plasma and block microcapillaries and thus impairing oxygen exchange (9). COVID-19-induced inflammation in the brain can result in dysfunctional neurological signaling and persist in neurological complications (1), including myelin loss (7) and tau hyperphosphorylation like in Alzheimer’s disease (6). Dysbiosis of the gut microbiota can even contribute to the pathogenesis of long COVID (10).
There is no consensus on therapeutic approaches to address long COVID (4). The multiplicity and complexity of symptoms of long COVID is incompatible with common pharmacological interventions, which are based on a one-symptom one-medication model. Non-pharmacological interventions like fasting or nutritional strategies act on multiple metabolic processes, ultimately leading to global changes in metabolic health (11).
Long-term fasting (LF) is defined as the voluntary renouncement of food intake lasting from 5 days to several weeks, depending on the individual physical condition (12). The Buchinger Wilhelmi fasting programme is minimally supplemented with up to 250 kcal through fruit juice and honey (13). This therapeutic approach has shown multiple effects on pathological mechanisms involved in COVID-19 infections, such as decreasing inflammation (14, 15) or reducing oxidative stress (16, 17). Furthermore, LF has been shown to reduce risk factors associated with severe COVID-19 courses. This includes weight loss, reduction in waist circumference (13), normalization of blood pressure (18), as well as glucose and lipid levels (13, 19) which are linked to COVID-19 comorbidities like obesity, hypertension, diabetes or dyslipidemia (2). Moreover, LF has been shown to affect gut microbiota (20) and improved well-being (13).
Up to now, clinical trials investigating the effects of LF on patients with long COVID are lacking. This case series provides the first documented improvements of self-reported symptoms and blood parameters before and after LF in patients with long COVID.
2. Methods
Regular patients of the Buchinger Wilhelmi clinics in Marbella (Spain) and Überlingen (Germany) with PSCA and confirmed positive PCR or SARS-CoV-2 rapid antigen test were included. All patients gave their written informed consent. Medical doctors conducted comprehensive medical history assessments, physical examinations before the fasting and documented height and waist circumference. Trained nurses measured every morning resting blood pressure, pulse, and body weight. Blood samples were collected before and after fasting. Adverse events were monitored continuously. For detailed descriptions, refer to (13), and Supplementary Figure S1 provides a visualization of the data collection.
The patients reported retrospectively about the acute phase of the COVID-19 infection. The severity level of the symptoms [asymptomatic, mild, moderate, severe, critical (life threatening)] and of the disease and level of care were determined.
Patients self-reported 19 symptoms that are potentially associated with COVID-19 on a visual analogue scale between 0 (none) and 10 (maximum) either retrospectively for the acute phase or prospectively before and after LF.
The EQ-5D-5L questionnaire was also applied for the acute phase, before and after LF. The questionnaire consists of five dimensions: mobility, self-care, usual activities, pain and anxiety/depression. Each dimension has five response levels: no problems, slight problems, moderate problems, severe problems, unable to /extreme problems. The index is calculated by applying a scoring algorithm (21). A value of 1 reflects full health, a value of 0 reflects worst health. The EQ-5D visual scale with the two endpoints “the best (100) and worst (0) health you can imagine” provides a quantitative measure of the perceived overall health (22).
Moreover, our intention was to ascertain which therapies or activities patients subjectively attributed the most to the improvement in their self-reported symptoms. Patients were provided with the option to denote their preferences by marking the categories of “fasting,” “diet,” “physiotherapy,” and “psychotherapy” or by providing individual comments under the “others” category. Two routine blood analysis were performed, one at the beginning and one at the end of fasting (13).
LF was performed according to the Buchinger Wilhelmi fasting program as described in details by Wilhelmi de Toledo et al. (13) and in line with the guidelines of fasting therapy (23).
3. Case description
Fourteen patients (5 women and 9 men) with persistent post-COVID-19 symptoms were admitted. Table 1 shows patients’ baseline characteristics and acute infection details. Age ranged from 33 (No. 9) to 74 years (No. 14). One patient (No. 11) was asymptomatic during the acute phase, while three had mild symptoms, five had moderate, and five had severe symptoms. The perception of acute symptoms varied widely from 1 day to 1.5 years. Five patients were able to perform their usual activities, three were unable to do so, and four were hospitalized during the acute phase. Three of them (Nos. 2, 12, 14) receiving oxygen treatment. The length of the inpatient stays was 3, 4, 5 and 10 days. Drugs were taken by five patients [corticosteroids (n = 4); antivirals (n = 3); antibiotics (n = 3)]. The period between acute infection and start of the fasting intervention varied from 5 to 22 months.
The patients had several pre-existing conditions that were diagnosed at the time of the fasting intervention. The most common diagnoses were obesity (n = 7), dyslipidemia (n = 4), digestive disorders (n = 4), and heart disease (n = 4). A table with all diagnoses per patient can be found in the supplements.
The patients stayed at the clinic for 10–21 days and underwent a fasting therapy for more than 6 days up to 16 days with a subsequent food reintroduction period of 3–5 days most patients (Table 1).
LF induced marked weight loss in all patients, as shown in Table 2. Systolic blood pressure decreased or remained unchanged, with patient No. 12, who had the highest initial value, experiencing the greatest reduction by −55 mmHg. Patient No. 9, who had the lowest initial value, showed no relevant change (−2 mmHg). Diastolic blood pressure changes followed a similar pattern, albeit less pronounced.
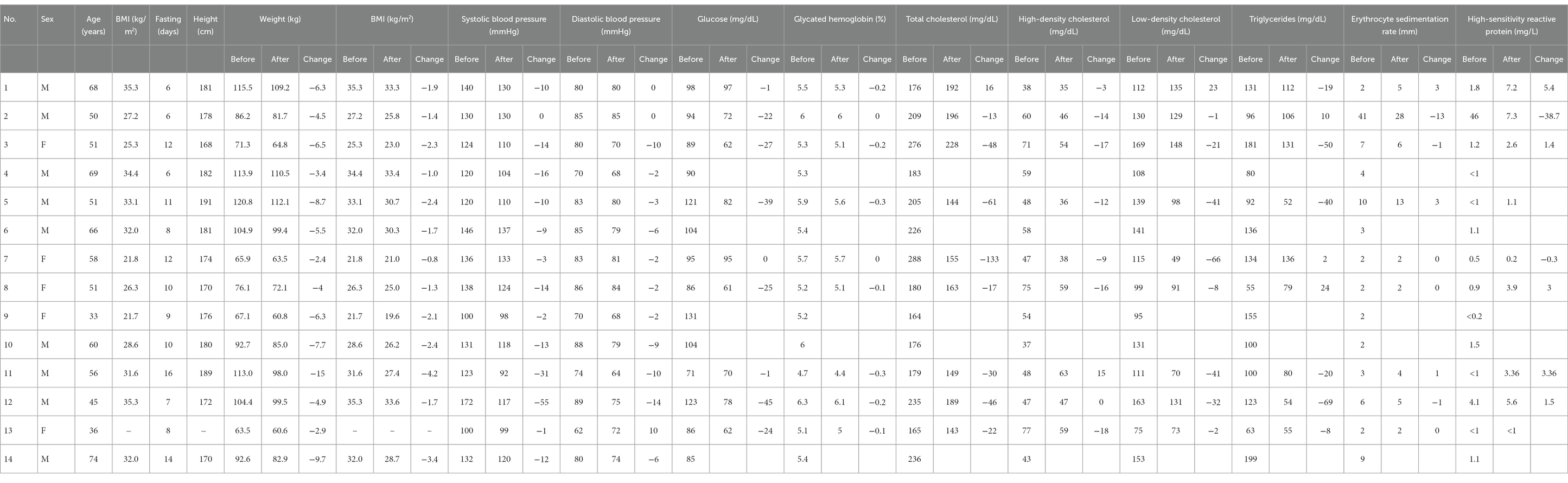
Table 2. Changes in body weight, BMI and blood pressure as well as glucose, lipid and inflammatory parameters before and after long-term fasting as well as calculated changes.
Blood test results before and after LF revealed a reduction in blood glucose levels (Table 2). However, glycated hemoglobin remained unchanged (Table 2). Lipid parameters were predominantly reduced (Table 2). Patients with high initial total cholesterol values had the strongest reduction (No. 7: −133 mg/dL; No. 5: −61 mg/dL). Inflammatory conditions were assessed using erythrocyte sedimentation rate (ESR), reflecting an indirect measurement of the inflammatory level in the body and high-sensitive C-reactive protein (hs CRP), reflecting a direct measurement of the inflammatory response. Changes in ESR were inconsistent. Three patients (No. 2, 3, 12), who had higher baseline levels, showed a reduction. Three patients (No. 1, 5, 11) had increased ESR levels after fasting and three patients with initial low values had no change. Hs-CRP level was reduced by −38,7 mg/L in patient No. 2 who had high initial values and in a second patient (No. 7). Five patients had increased hs-CRP levels after fasting. Additional results on blood count, blood markers, kidney and liver parameters as well as electrolytes are available in the Supplementary material.
Participants’ health perception based on the five dimensions mobility, self-care, usual activities, pain and anxiety/depression increased in most cases after the acute infection. Generally, the fasting intervention improved the health status. This is shown by the results of the EQ-5D index score and supported by the patients reported overall health on a visual analogue scale, that endpoints are labelled by “the best (100) and worst (0) health you can imagine” (Figure 1). In total, 13 patients indicated an improvement.
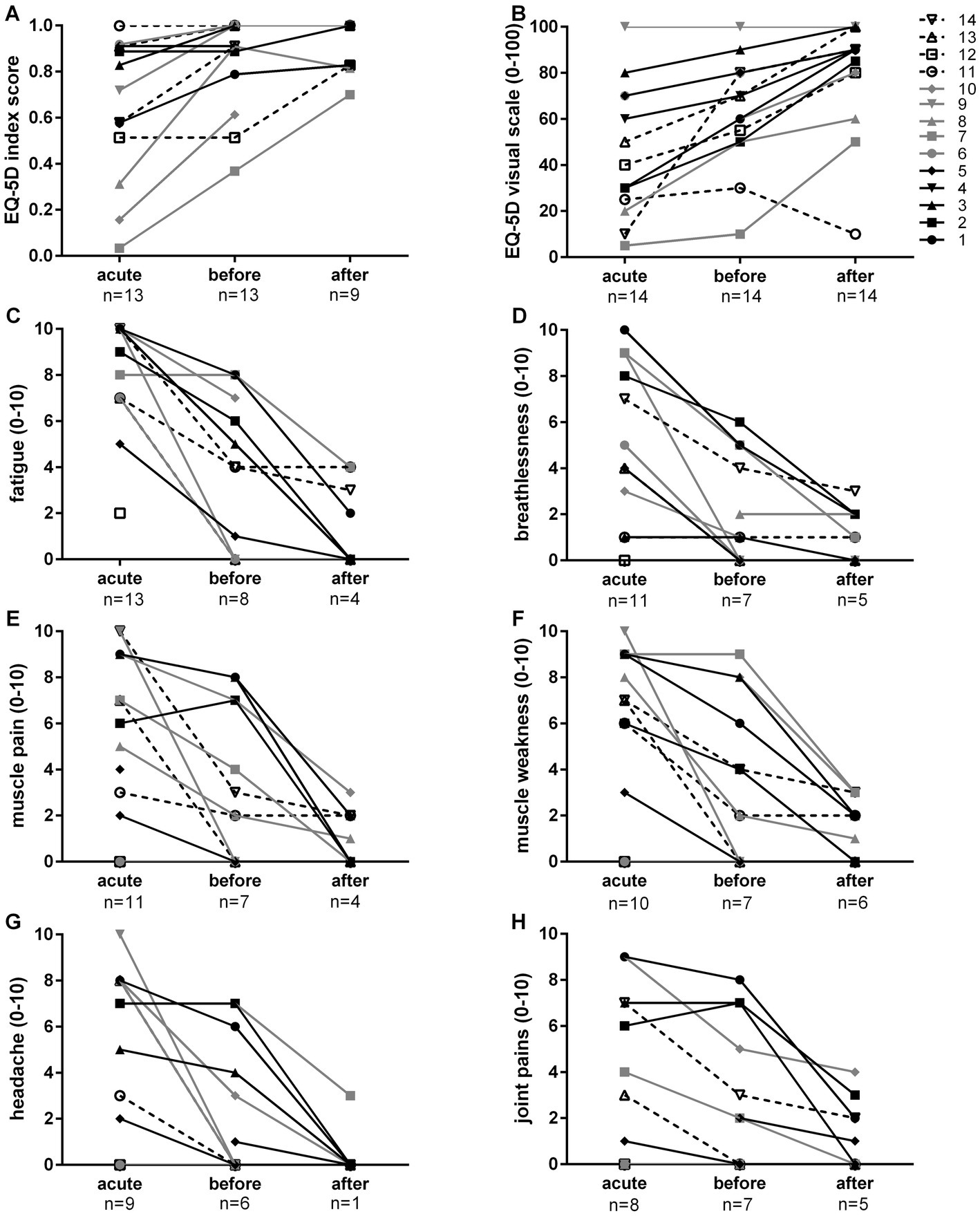
Figure 1. Changes in EQ-5D-5L index score (A) and visual scale (B) as well as most frequently mentioned self-reported symptoms (fatigue C; breathlessness D; muscle pain E; muscle weakness F; headache G; joint pains H) on a visual scale from 0 (none) to 10 (maximum). The EQ-5D-5L index score is based on the five dimensions mobility, self-care, usual activities, pain, and anxiety/depression. Each dimension has five response levels: no problems, slight problems, moderate problems, severe problems, unable to/extreme problems. The index is calculated by applying a scoring algorithm (21). A value of 1 reflects full health, a value of 0 reflects worst health. The EQ-5D visual scale provides a quantitative measure of the perceived overall health. The number of patients who answered (response > 0) is displayed below the time point.
Figures 1C–H, 2A–H show symptom intensity on a visual scale from 0 (none) to 10 (maximum). The most common symptoms were fatigue, breathlessness, muscle pain, muscle weakness, headache, joint pains, sleep difficulties and chest pain/tightness. Improvement was reported by many patients after the acute phase. Ongoing symptoms could be further improved by LF in the majority of the cases (Figure 1). Less frequent post-acute sequelae such as depression, cognitive impairment, cough/sore throat, dizziness as well as smell and taste disorders improved in all after LF (Figure 2). While fever was predominantly a symptom during the acute phase, abdominal pain, skin rash, diarrhea, and nausea were rare. Nevertheless, improvement was observed during LF (Supplementary Figure S2).
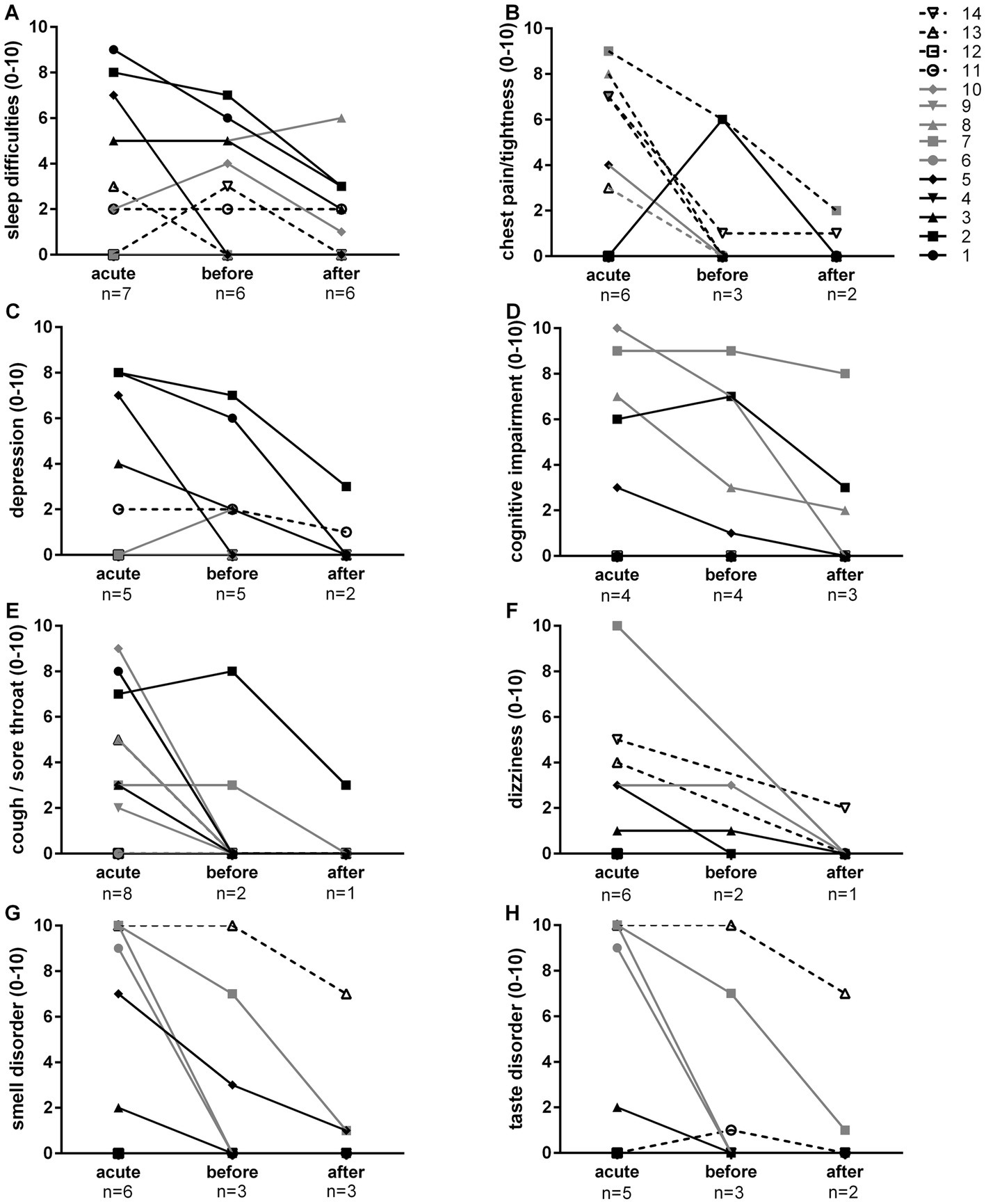
Figure 2. Self-reported symptoms (sleep difficulties, A; chest pain/tightness, B; depression, C; cognitive impairment, D; cough/sore throat, E; dizziness, F; smell disorder, G; taste disorder, H) on a visual scale from 0 (none) to 10 (maximum). The number of patients that reported to experience the symptom (response > 0) are indicated below for the acute phase, as well as before and after long-term fasting.
Patients were asked about their opinion, which therapies and activities helped the most to improve their symptoms. Out of the 14 patients, 10 mentioned fasting as the most helpful treatment. Six patients found physiotherapy helpful. Other treatments mentioned included ozone therapy, acupuncture, psychotherapy, and osteopathy. No adverse events were observed.
4. Discussion
We report the beneficial effects of LF in 14 patients suffering from sequelae of COVID-19. Perceived overall health was enhanced, with improvements in common symptoms like fatigue, breathlessness, muscle pain and weakness, headache, joint pains and sleep difficulties. Even less frequent, but classical COVID symptoms, like cognitive impairments, smell and taste disorders were ameliorated. Given the lack of therapeutic approaches to treat long COVID, our observations suggests that long-term fasting could be a non-pharmacological approach to treat long COVID. This is corroborated by a large number of studies showing that fasting targets multiple aspects of long COVID, which are elaborated upon in the following discussion.
Voluntarily renunciation of food intake during an acute infection is a known behavioral adaptation to enhance survival (24). Clinical experience and case reports have described the positive effects of fasting on infectious diseases such as typhoid and scarlet fever, or tonsillitis (25). Even controlled laboratory studies showed that when mice were infected with Listeria monocytogenes, 95% of normally fed mice died while only 5% of the previously starved mice died (26). This enhancement of survival can be linked to adaptive defense mechanisms that are triggered by fasting. When food intake is interrupted, the body switches to fat burning as primary energy source, leading to the production of ketone bodies. Recent research has demonstrated that nutritional ketosis can promote antiviral immunity in lung (27). The ketone body β-hydroxybutyrate directly increases CD8+ T effector cell cytokine production through effects on histone acetylation (28).
The known beneficial effects of fasting on brain metabolism could provide explanations for the enhancement in self-perceived health observed in this study. In the rodent brain, fasting reduced oxidative stress (29) and enhanced mitochondrial oxidative metabolism (30). This was linked to the activity of the ketone body beta-hydroxybutyrate (BHB) which improves brain function by triggering the releasing brain-derived neurotrophic factor, increasing cognitive performance, memory, synaptic plasticity, neurogenesis and resistance to injury and diseases (31). Fasting is even known to cause a pronounced stimulation of the hypothalamic–pituitary–adrenal axis conferring a pain-relieving effect (32). These fasting-induced hormonal changes could explain lower muscle and joint pain as well as headache found in this study. The marked improvements in muscle pain and weakness are supported by a study that showed an increased in leg muscle power and strength during a 10-days fast (15). In a larger group of 1,422 persons fasting for 4 to 21 days, fasting caused an elevation in emotional and physical well-being and increased energy levels (13, 18).
Furthermore, Fasting affects coagulation parameters in adults (13), potentially counteracting clotting tendency during PASC. Moreover, fasting promotes vasodilatation through adiponectin-mediated endothelial nitric oxide production in rats (33). This could mitigate endothelial dysfunction. Sleep quality increased after 7 fasting days which aligns with the observed reduction in sleep difficulties in other studies (34).
The cessation of food intake triggers the metabolic switch from glucose to fat and ketone utilization, which is reflected at the molecular level by the inactivation of the nutrient sensitive mammalian target of rapamycin (mTOR) pathway. It could be speculated that the effects of fasting on COVID are mediated by mTOR. Furthermore, mTOR signalling can activate the inflammasome, which might be associated with the cytokine storm during COVID The hyper-production of cytokines during COVID occurs especially in obese (35), where the mTOR signaling is already elevated and linked to weight gain and insulin resistance (36). This could be why obesity with its chronic inflammation is a risk factor for severe COVID-19 outcomes characterized by a strong inflammatory response in the adipose tissue (37, 38).
The dysregulation of the immune function is a hallmark of COVID-19. Fasting could possibly boost the antiviral immune function and survival of activated T cells via the production of BHB which has been showed to serve as a more efficient alternative fuel for T cells (27). This is supported by demonstrations that ketogenic diets have been reported to ameliorate clinical symptoms of pulmonary health conditions (39). A way to reduce inflammation is to diminish the volume of adipose tissue by a fasting-induced weight loss, as observed in the presented cases and previous studies, as well as reducing visceral fat (13). Further anti-inflammatory mechanisms could be triggered by activation of the Nuclear factor erythroid 2-related factor (Nrf)-2/heme oxygenase (HO)-1 signaling pathway (40). The anti-inflammatory effect of fasting is documented in inflammatory diseases like rheumatoid arthritis (14) and shown by the decrease of various parameters like tumor necrosis factor-α, or interleukin-6 (15). Additionally, the inactivation of the mTOR cascade promotes autophagy (41). This may help to clear viral RNA through lysosomes (42) and repair post-acute illness damages (43).
Fasting can improve cellular stress adaptation by eliminating free radicals. A 10-days fast increased total antioxidant capacity and diminished damages from lipid peroxidation (16, 17). Asthmatic patients had less oxidative stress after 8-weeks alternate day fasting (44). Moreover, cells switch during fasting into a protective mode, which prevents DNA damages and induces DNA repair (45). In mice, fasting activated hematopoietic stem cells, enhancing self-renewal and lineage-balanced regeneration of immune cells (46). These findings suggest that fasting could reverse immunosuppressive states and strengthen the innate immune system.
Patients suffering from COVID-19 have an increased risk to develop cardiovascular diseases (47). Furthermore, alternations in lipid profile of COVID-19 patients have been demonstrated (48). Fasting studies have shown both preventive and therapeutic improvements in glucose and insulin levels (15), cholesterol and triglyceride levels (19), high blood pressure (18), and fatty liver (49). The presented cases also displayed improvements in blood results and blood pressure measurements.
Studies have linked microbiome dysbiosis to SARS-CoV-2-infections and increased mortality (50). Fasting has been shown to influence gut microbiome composition, increased short chain fatty acid production, and reduce gut permeability (20). The influence on health effects during PSAC require further investigation.
The safety of fasting during acute virus infections raises concerns due to contradicting findings in mouse experiments. While fasting improved survival in models of bacterial inflammation, glucose utilization was found to be crucial for survival in models of viral inflammation (51). More studies are needed to assess the safety of fasting during an acute infection. Furthermore, it will be of importance to determine the optimal period for initiating fasting therapy after an acute infection as well as to determine the optimal fasting length. This case series is limited by the reliance on retrospective data of the acute phase, subjective evaluations, missing data, the lack of viral load assessment and consideration of other confounders. Future studies should also incorporate a non-fasting control group to enable the extrapolation of the effectiveness of the fasting treatment.
Altogether, an increasing number of studies showed that LF triggers well-orchestrated processes like autophagy, oxidative stress defense, anti-inflammatory responses, contributing to a normalization of the metabolism and improvements in cardiovascular risk factors. In long COVID patients, these effects could potentially restore organ function, decrease systemic inflammation and oxidative stress, and ultimately recover the health status. While further research is warranted, the improved well-being and reduced physical complaints observed in long COVID patients fasting at the Buchinger Wilhelmi Clinic suggests that LF could be a potent a non-pharmacological approach in reclaiming health after the contraction of serious infectious diseases.
Data availability statement
The original contributions presented in the study are included in the article/Supplementary material, further inquiries can be directed to the corresponding author.
Ethics statement
Written informed consent was obtained from the individual(s) for the publication of any potentially identifiable images or data included in this article. Written informed consent was obtained from the participant/patient(s) for the publication of this case report.
Author contributions
FG: conceptualization, data collection, writing original draft, and preparation. RM: writing – review and editing. AC: data collection. FW: conceptualization, and writing – review and editing. All authors contributed to the article and approved the submitted version.
Acknowledgments
We are very grateful to the patients for participating in this case series.
Conflict of interest
FG, RM, and FW are employees of the Buchinger Wilhelmi Development and Holding GmbH, Überlingen. AC is employee of the Buchinger Wilhelmi Clinic Marbella.
Publisher’s note
All claims expressed in this article are solely those of the authors and do not necessarily represent those of their affiliated organizations, or those of the publisher, the editors and the reviewers. Any product that may be evaluated in this article, or claim that may be made by its manufacturer, is not guaranteed or endorsed by the publisher.
Supplementary material
The Supplementary material for this article can be found online at: https://www.frontiersin.org/articles/10.3389/fnut.2023.1195270/full#supplementary-material
Supplementary Figure S1 | Visualisation of the data collection.
Supplementary Figure S2 | Self-reported symptoms, fever (A), abdominal pain (B), skin rash (C), diarrhea (D) and nausea (E), on a visual scale from 0 (none) to 10 (maximum). The number of patients that reported to experience the symptom (response > 0) are indicated below for the acute phase, as well as before and after long-term fasting.
References
1. Davis, HE , McCorkell, L , Vogel, JM , and Topol, EJ . Long COVID: major findings, mechanisms and recommendations. Nat Rev Microbiol. (2023) 21:133–46. doi: 10.1038/s41579-022-00846-2
2. Crook, H , Raza, S , Nowell, J , Young, M , and Edison, P . Long covid—mechanisms, risk factors, and management. BMJ. (2021) 374:n1648. doi: 10.1136/bmj.n1648
3. Shah, W , Hillman, T , Playford, ED , and Hishmeh, L . Managing the long term effects of covid-19: summary of NICE, SIGN, and RCGP rapid guideline. BMJ. (2021) 372:n136. doi: 10.1136/bmj.n136
4. Yelin, D , Moschopoulos, CD , Margalit, I , Gkrania-Klotsas, E , Landi, F , Stahl, JP, et al. ESCMID rapid guidelines for assessment and management of long COVID. Clin Microbiol Infect. (2022) 28:955–72. doi: 10.1016/j.cmi.2022.02.018
5. Soriano, JB , Murthy, S , Marshall, JC , Relan, P , and Diaz, JV . A clinical case definition of post-COVID-19 condition by a Delphi consensus. Lancet Infect Dis. (2022) 22:e102–7. doi: 10.1016/S1473-3099(21)00703-9
6. Reiken, S , Sittenfeld, L , Dridi, H , Liu, Y , Liu, X , and Marks, AR . Alzheimer's-like signaling in brains of COVID-19 patients. Alzheimers Dement. (2022) 18:955–65. doi: 10.1002/alz.12558
7. Fernández-Castañeda, A , Lu, P , Geraghty, AC , Song, E , Lee, M-H , Wood, J, et al. Mild respiratory COVID can cause multi-lineage neural cell and myelin dysregulation. Cells. (2022) 185:2452–2468.e16. e16. doi: 10.1016/j.cell.2022.06.008
8. Barletta, MA , Marino, G , Spagnolo, B , Bianchi, FP , Falappone, PCF , Spagnolo, L, et al. Coenzyme Q10 + alpha lipoic acid for chronic COVID syndrome. Clin Exp Med. (2023) 23:667–78. doi: 10.1007/s10238-022-00871-8
9. Pretorius, E , Vlok, M , Venter, C , Bezuidenhout, JA , Laubscher, GJ , Steenkamp, J, et al. Persistent clotting protein pathology in long COVID/post-acute sequelae of COVID-19 (PASC) is accompanied by increased levels of antiplasmin. Cardiovasc Diabetol. (2021) 20:172. doi: 10.1186/s12933-021-01359-7
10. Peluso, MJ , and Deeks, SG . Early clues regarding the pathogenesis of long-COVID. Trends Immunol. (2022) 43:268–70. doi: 10.1016/j.it.2022.02.008
11. Mesnage, R , Grundler, F , and Wilhelmi de Toledo, F . Leveraging the self-healing abilities of our body to promote healthy longevity. Public Health Toxicol. (2023) 3:1–3. doi: 10.18332/pht/161464
12. Wilhelmi de Toledo, F , Grundler, F , Sirtori, CR , and Ruscica, M . Unravelling the health effects of fasting: a long road from obesity treatment to healthy life span increase and improved cognition. Ann Med. (2020) 52:147–61. doi: 10.1080/07853890.2020.1770849
13. Wilhelmi de Toledo, F , Grundler, F , Bergouignan, A , Drinda, S , and Michalsen, A . Safety, health improvement and well-being during a 4 to 21-day fasting period in an observational study including 1422 subjects. PLoS One. (2019) 14:e0209353. doi: 10.1371/journal.pone.0209353
14. Müller, H , de Toledo, FW , and Resch, K-L . Fasting followed by vegetarian diet in patients with rheumatoid arthritis: a systematic review. Scand J Rheumatol. (2001) 30:1–10. doi: 10.1080/030097401750065256
15. Laurens, C , Grundler, F , Damiot, A , Chery, I , Le Maho, A-L , Le Maho, Y, et al. Is muscle and protein loss relevant in long-term fasting? A prospective trial about physiologic and metabolic adaptations. J Cachexia Sarcopenia Muscle. (2021) 12:1690–703. doi: 10.1002/jcsm.12766
16. Wilhelmi de Toledo, F , Grundler, F , Goutzourelas, N , Tekos, F , Vassi, E , Mesnage, R, et al. Influence of long-term fasting on blood redox status in humans. Antioxidants. (2020) 9:496. doi: 10.3390/antiox9060496
17. Grundler, F , Mesnage, R , Goutzourelas, N , Tekos, F , Makri, S , Brack, M, et al. Interplay between oxidative damage, the redox status, and metabolic biomarkers during long-term fasting. Food Chem Toxicol. (2020) 145:111701. doi: 10.1016/j.fct.2020.111701
18. Grundler, F , Mesnage, R , Michalsen, A , and Wilhelmi de Toledo, F . Blood pressure changes in 1610 subjects with and without antihypertensive medication during long-term fasting. J Am Heart Assoc. (2020) 9:e018649. doi: 10.1161/JAHA.120.018649
19. Grundler, F , Plonné, D , Mesnage, R , Müller, D , Sirtori, CR , Ruscica, M, et al. Long-term fasting improves lipoprotein-associated atherogenic risk in humans. Eur J Nutr. (2021) 60:4031–44. doi: 10.1007/s00394-021-02578-0
20. Mesnage, R , Grundler, F , Schwiertz, A , Le Maho, Y , and Wilhelmi de Toledo, F . Changes in human gut microbiota composition are linked to the energy metabolic switch during 10 d of Buchinger fasting. J Nutr Sci. (2019) 8:e36. doi: 10.1017/jns.2019.33
21. van Hout, B , Janssen, MF , Feng, YS , Kohlmann, T , Busschbach, J , Golicki, D, et al. Interim scoring for the EQ-5D-5L: mapping the EQ-5D-5L to EQ-5D-3L value sets. Value Health. (2012) 15:708–15. doi: 10.1016/j.jval.2012.02.008
22. McClure, NS , Sayah, FA , Xie, F , Luo, N , and Johnson, JA . Instrument-defined estimates of the minimally important difference for EQ-5D-5L index scores. Value Health. (2017) 20:644–50. doi: 10.1016/j.jval.2016.11.015
23. Wilhelmi de Toledo, F , Buchinger, A , Burggrabe, H , Hölz, G , Kuhn, C , Lischka, E, et al. Fasting therapy-an expert panel update of the 2002 consensus guidelines. Complement Med Res. (2013) 20:434–43. doi: 10.1159/000357602
24. Exton, MS . Infection-induced anorexia: active host defence strategy. Appetite. (1997) 29:369–83. doi: 10.1006/appe.1997.0116
25. Wilhelmi de Toledo, F . Therapeutisches fasten nach Buchinger und Immunsystem: Erfahrung und Hypothesen Ärztezeitschrift f. Naturheilverf. (1995) 36:331–41.
26. Wing, EJ , and Young, JB . Acute starvation protects mice against Listeria monocytogenes. Infect Immun. (1980) 28:771–6. doi: 10.1128/iai.28.3.771-776.1980
27. Karagiannis, F , Peukert, K , Surace, L , Michla, M , Nikolka, F , Fox, M, et al. Impaired ketogenesis ties metabolism to T cell dysfunction in COVID-19. Nature. (2022) 609:801–7. doi: 10.1038/s41586-022-05128-8
28. Luda, KM , Longo, J , Kitchen-Goosen, SM , Duimstra, LR , Ma, EH , Watson, MJ, et al. Ketolysis drives CD8+T cell effector function through effects on histone acetylation. Immunity. (2023) 56:2021–2035.e8. doi: 10.1016/j.immuni.2023.07.002
29. Li, L , Wang, Z , and Zuo, Z . Chronic intermittent fasting improves cognitive functions and brain structures in mice. PLoS One. (2013) 8:e66069. doi: 10.1371/journal.pone.0066069
30. Marosi, K , Kim, SW , Moehl, K , Scheibye-Knudsen, M , Cheng, A , Cutler, R, et al. 3-hydroxybutyrate regulates energy metabolism and induces BDNF expression in cerebral cortical neurons. J Neurochem. (2016) 139:769–81. doi: 10.1111/jnc.13868
31. Mattson, MP , and Arumugam, TV . Hallmarks of brain aging: adaptive and pathological modification by metabolic states. Cell Metab. (2018) 27:1176–99. doi: 10.1016/j.cmet.2018.05.011
32. Michalsen, A . Prolonged fasting as a method of mood enhancement in chronic pain syndromes: a review of clinical evidence and mechanisms. Curr Pain Headache Rep. (2010) 14:80–7. doi: 10.1007/s11916-010-0104-z
33. Wan, R , Ahmet, I , Brown, M , Cheng, A , Kamimura, N , Talan, M, et al. Cardioprotective effect of intermittent fasting is associated with an elevation of adiponectin levels in rats. J Nutr Biochem. (2010) 21:413–7. doi: 10.1016/j.jnutbio.2009.01.020
34. Michalsen, A , Schlegel, F , Rodenbeck, A , Lüdtke, R , Huether, G , Teschler, H, et al. Effects of short-term modified fasting on sleep patterns and daytime vigilance in non-obese subjects: results of a pilot study. Ann Nutr Metab. (2003) 47:194–200. doi: 10.1159/000070485
35. Hosoya, T , Oba, S , Komiya, Y , Kawata, D , Kamiya, M , Iwai, H, et al. Apple-shaped obesity: a risky soil for cytokine-accelerated severity in COVID-19. Proc Natl Acad Sci U S A. (2023) 120:e2300155120. doi: 10.1073/pnas.2300155120
36. Um, SH , Frigerio, F , Watanabe, M , Picard, F , Joaquin, M , Sticker, M, et al. Absence of S6K1 protects against age- and diet-induced obesity while enhancing insulin sensitivity. Nature. (2004) 431:200–5. doi: 10.1038/nature02866
37. Pepera, G , Tribali, M-S , Batalik, L , Petrov, I , and Papathanasiou, J . Epidemiology, risk factors and prognosis of cardiovascular disease in the coronavirus disease 2019 (COVID-19) pandemic era: a systematic review. RCM. (2022) 23:1. doi: 10.31083/j.rcm2301028
38. Stefan, N . SARS-CoV-2 fires up inflammation in adipose tissue. Nat Rev Endocrinol. (2023) 19:8–9. doi: 10.1038/s41574-022-00778-0
39. Mey, JT , Kirwan, JP , and Axelrod, CL . The role of nutrition in mitigating the effects of COVID-19 from infection through PASC. Nutrients. (2023) 15:866. doi: 10.3390/nu15040866
40. Dai, S , Wei, J , Zhang, H , Luo, P , Yang, Y , Jiang, X, et al. Intermittent fasting reduces neuroinflammation in intracerebral hemorrhage through the Sirt3/Nrf2/HO-1 pathway. J Neuroinflammation. (2022) 19:122. doi: 10.1186/s12974-022-02474-2
41. Hofer, SJ , Carmona-Gutierrez, D , Mueller, MI , and Madeo, F . The ups and downs of caloric restriction and fasting: from molecular effects to clinical application. EMBO Mol Med. (2022) 14:e14418. doi: 10.15252/emmm.202114418
42. Orvedahl, A , MacPherson, S , Sumpter, R Jr, Tallóczy, Z , Zou, Z , and Levine, B . Autophagy protects against Sindbis virus infection of the central nervous system. Cell Host Microbe. (2010) 7:115–27. doi: 10.1016/j.chom.2010.01.007
43. Gnoni, M , Beas, R , and Vásquez-Garagatti, R . Is there any role of intermittent fasting in the prevention and improving clinical outcomes of COVID-19?: intersection between inflammation, mTOR pathway, autophagy and calorie restriction. Virus. (2021) 32:625–34. doi: 10.1007/s13337-021-00703-5
44. Johnson, JB , Summer, W , Cutler, RG , Martin, B , Hyun, D-H , Dixit, VD, et al. Alternate day calorie restriction improves clinical findings and reduces markers of oxidative stress and inflammation in overweight adults with moderate asthma. Free Radic Biol Med. (2007) 42:665–74. doi: 10.1016/j.freeradbiomed.2006.12.005
45. Nencioni, A , Caffa, I , Cortellino, S , and Longo, VD . Fasting and cancer: molecular mechanisms and clinical application. Nat Rev Cancer. (2018) 18:707–19. doi: 10.1038/s41568-018-0061-0
46. Cheng, C-W , Adams, GB , Perin, L , Wei, M , Zhou, X , Lam, BS, et al. Prolonged fasting reduces IGF-1/PKA to promote hematopoietic-stem-cell-based regeneration and reverse immunosuppression. Cell Stem Cell. (2014) 14:810–23. doi: 10.1016/j.stem.2014.04.014
47. Giacca, M , and Shah, AM . The pathological maelstrom of COVID-19 and cardiovascular disease. Nat Cardiovasc Res. (2022) 1:200–10. doi: 10.1038/s44161-022-00029-5
48. Papotti, B , Macchi, C , Favero, C , Iodice, S , Adorni, MP , Zimetti, F, et al. HDL in COVID-19 patients: evidence from an Italian cross-sectional study. J Clin Med. (2021) 10:5955. doi: 10.3390/jcm10245955
49. Drinda, S , Grundler, F , Neumann, T , Lehmann, T , Steckhan, N , Michalsen, A, et al. Effects of periodic fasting on fatty liver index—a prospective observational study. Nutrients. (2019) 11:2601. doi: 10.3390/nu11112601
50. Din, AU , Mazhar, M , Waseem, M , Ahmad, W , Bibi, A , Hassan, A, et al. SARS-CoV-2 microbiome dysbiosis linked disorders and possible probiotics role. Biomed Pharmacother. (2021) 133:110947. doi: 10.1016/j.biopha.2020.110947
Keywords: long-term fasting, post-SARS-CoV-2 syndrome, long COVID, case report, well-being
Citation: Grundler F, Mesnage R, Cerrada A and Wilhelmi de Toledo F (2023) Improvements during long-term fasting in patients with long COVID – a case series and literature review. Front. Nutr. 10:1195270. doi: 10.3389/fnut.2023.1195270
Edited by:
Klara Komici, University of Molise, ItalyReviewed by:
Maria Chiara Grimaldi, Catholic University of the Sacred Heart, ItalyBenjamin D. Horne, Intermountain Healthcare, United States
Copyright © 2023 Grundler, Mesnage, Cerrada and Wilhelmi de Toledo. This is an open-access article distributed under the terms of the Creative Commons Attribution License (CC BY). The use, distribution or reproduction in other forums is permitted, provided the original author(s) and the copyright owner(s) are credited and that the original publication in this journal is cited, in accordance with accepted academic practice. No use, distribution or reproduction is permitted which does not comply with these terms.
*Correspondence: Franziska Grundler, ZnJhbnppc2thLmdydW5kbGVyQGJ1Y2hpbmdlci13aWxoZWxtaS5jb20=