- Department of Neurosurgery, Union Hospital, Tongji Medical College, Huazhong University of Science and Technology, Wuhan, Hubei, China
Vitamin D (VitD) insufficiency is a worldwide health problem and affects billions of people. Spinal cord injury (SCI) patients seem more susceptible to developing suboptimal levels of VitD. However, the literature regarding its impact on the prognosis of SCI is limited. Thus, in this review, we systematically investigated the published studies via a combination of keywords associated with SCI and VitD in four medical databases (Medline, Embase, Scopus, and Web of Science). All included studies were analyzed, and selected clinical data on the prevalence of VitD insufficiency (serum 25-hydroxyvitamin D < 30 ng/ml) and deficiency (serum 25-hydroxyvitamin D < 20 ng/ml) were collected for further meta-analysis via random effects. Through literature review, a total of 35 studies were eligible and included. The meta-analysis of VitD status (13 studies, 1,962 patients) indicated high prevalence of insufficiency (81.6% [75.7, 87.5]) and deficiency (52.5% [38.1, 66.9]) after SCI. Besides, low levels of VitD were reported to be associated with a higher risk of skeletal diseases, venous thromboembolism, psychoneurological syndromes, and chest illness after injury. Existing literature suggested that supplemental therapy might act as an adjuvant treatment to facilitate post-injury rehabilitation. Non-human experimental studies highlighted the neuroprotective effect of VitD, which was associated with enhancing axonal and neuronal survival, suppressing neuroinflammation, and modulating autophagy. Therefore, the current evidence suggests that the prevalence of VitD insufficiency is high in the SCI population, and low-level VitD may impair functional restoration after SCI. VitD supplemental treatment may have potential benefits to accelerate rehabilitation in mechanistically related processes after SCI. However, due to the limitation of the available evidence, more well-designed randomized controlled trials and mechanism experimental research are still needed to validate its therapeutic effect, elucidate its neuroprotective mechanism, and develop novel treatments.
1. Introduction
Vitamin D (VitD) insufficiency and deficiency are described as a pandemic associated with various chronic diseases in all age populations despite the commercialization of VitD supplements and ongoing prophylaxis projects in the general population (1, 2). The national health and nutrition examination survey in the United States indicated that 23–24% and 64% population had a serum total of 25(OH)D less than 20 and 30 ng/mL, respectively (3, 4). In the European Union, Cashman et al. (5) summarized multiple national surveys and indicated that 40.4% of European individuals had serum 25(OH)D concentrations less than 20 ng/ml on average. Spinal cord injury (SCI) is a potentially devastating event featured by severe sensorimotor deficits and autonomic dysfunction with a high burden on both family and society. SCI patients seem to be more susceptible to VitD insufficiency and deficiency due to an unbalanced diet, co-existing diseases, obesity, and lack of sunlight exposure secondary to physical inactivity (6, 7). Besides, several researchers indicated that post-injury autonomic dysfunction usually caused malfunctioning of endocrinological feedback systems, such as VitD-parathyroid hormone axis, and impaired VitD absorption through skin gastrointestinal tract, which directly led to low levels of VitD in SCI individuals (8–10). However, the mechanism underlying post-SCI VitD level alternation is still poorly understood, and literature summarizing its status and impact on the post-injury prognosis is limited.
Despite its classical role in calcium and phosphorus metabolism, VitD is a neurosteroid and exerts a neuroprotective effect in various neurological diseases. In an animal model of Parkinson’s disease, VitD treatment attenuated the injury of dopaminergic neurons via suppressing the release of proinflammatory cytokines and upregulating anti-inflammatory signaling (11). Similar protective findings were also observed in the facial nerve injury model, in which the administration of VitD3 could facilitate functional restoration by increasing myelination after 12 weeks of treatment (12).
Recent evidence highlighted the unique value of VitD in post-SCI rehabilitation. VitD deficiency in individuals with SCI has been implicated as a primary etiologic, and environmental factor responsible for multiple musculoskeletal issues (e.g., osteoporosis, fracture, chronic pain, etc.) (6, 7). At the same time, low-level VitD is associated with an increased risk of several neuropsychic diseases (e.g., chronic pain, depression, anxiety, PTSD, etc.) (13, 14). New findings also suggest that VitD insufficiency and deficiency were associated with malfunctioning of autonomic nerve system (e.g., a change of endocrinological feedback systems, impaired cardiac autonomic functions, etc.), which might delay the SCI rehabilitation (8, 15). In addition, the pressure injury, an often-occurring complication after SCI, was also reported to be associated with VitD status (16). Notably, Aminmansour et al. (17) applied a combination therapy of progesterone and VitD in a randomized, double-blinded, placebo-controlled study of acute traumatic SCI. They observed that this treatment plan was associated with better functional outcomes. Nevertheless, the published clinical and experimental studies regarding the effect of VitD in SCI are few, and its therapeutic effect remains to be determined.
In this review, we hypothesized that VitD might play an essential role in post-SCI rehabilitation. To validate this hypothesis, we performed a meta-analysis of published studies regarding the prevalence of VitD insufficiency and deficiency in SCI patients, and systematically summarized the clinical and experimental evidence of VitD’s effect in post-SCI rehabilitation.
2. Methods
2.1. Search strategy, study selection, and eligibility criteria
This study was performed according to the Preferred Reporting Items for Systematic Reviews and Meta-Analyses guidelines (PRISMA, PRISMA 2020 checklist in supplement materials), and approved by the Ethics Committee of the Tongji Medical College, Huazhong University of Science and Technology (18). Details of this systematic review and meta-analysis were registered on PROSPERO (registration number 2021: CRD42021262207), and can be accessed at1. It was a secondary analysis of the completed studies, and the written consent was waived, respectively. A combination of keywords on VitD and SCI and Boolean Operators were applied in four medical databases (Medline, Embase, Scopus, and Web of Science) to retrieve English literature in September 2021. Per the formulated literature search (search strategy in supplement materials), we sought studies (published between 1974 and August 31, 2021) on the VitD status and its impact on the prognosis after spinal cord injury. Both clinical and non-human experimental studies were included in this review to investigate the impact of VitD on the prognosis of SCI and its underlying neuroprotective mechanism.
The retrieved studies were verified and quantified before merging via Endnote X9 software (Clarivate Analytics), and the duplicates were removed accordingly. The titles, abstracts, and full texts of identified publications were further evaluated to search for eligible studies. The search was supplemented by the reference list of review articles and selected publications. Reviews, book chapters, case reports, small case series (case number less than 10), conference abstracts, editorial notes, and letters were excluded. Other exclusion criteria included repeated research on the same cohort of patients and unavailable full text. Two independent investigators comprehensively reviewed all selected publications to ensure their eligibility for inclusion in the review. Any disagreement regarding the eligibility of the literature was resolved by thoroughly discussing the publication with a third independent investigator.
2.2. Inclusion and exclusion criteria, data extraction, and quality evaluation of meta-analysis
Among the eligible articles, the studies that reported VitD status (VitD deficiency and insufficiency) in the SCI population were selected for meta-analysis. The spinal cord injury (SCI) is defined as damage to the spinal cord resulting from trauma (e.g., result of accident, fall, etc.) or from nontraumatic disease (e.g., degeneration of the spinal column, tumor, etc.). Both traumatic and non-traumatic SCI were included in this review.
The exclusion criteria include (1) studies in a specific population (i.e., athletes), (2) research without qualified data, (3) and the majority of the patients already received VitD treatment. We applied the assessment tool for case series studies from the National Institutes of Health (NIH) to rate the quality of eligible studies in the metanalysis (19). There are nine criteria in the score. For each item, we scored one point for “yes” and zero point for “no,” “not available,” “not reported,” and “cannot determine.” The scores are gauged from all nine criteria (0–9) and generated to represent the overall quality of the study. Data were extracted by two reviewers and included: first author; publication year; country; case number; mean or media age; gender ratio; study design; VitD measure parameter; mean or media injury duration; injury type (acute and chronic); injury extent (incomplete and complete motor function impairment); injury level (paraplegia and tetraplegia); serum VitD status (insufficiency and deficiency).
Based on the international standard for neurological classification spinal cord injury (ISNCSCI), the ASIA Impairment Scale (AIS) is applied to evaluate the injury extent. Unless otherwise stated, complete motor function impairment is used when no motor preserved below the neurological level (AIS A and B) with incomplete motor function impairment referring to AIS C and D (20). Unless otherwise stated, tetraplegia is defined as the impairment or loss of sensorimotor function in the cervical levels of the spinal cord, and paraplegia refers to the impairment or loss of sensorimotor function in the thoracic, lumbar or sacral (but not cervical) segments of the spinal cord (20).
Per clinical practice guidelines of Endocrine Society, we used the serum 25-hydroxyvitamin D [25(OH)D] level to evaluate VitD status in SCI patients (21). We defined VitD insufficiency as serum concentration of 25(OH)D less than 30 ng/ml and VitD deficiency as serum concentration of 25(OH)D less than 20 ng/ml in this study (21, 22).
2.3. Statistical analysis
A single-arm meta-analysis was performed via Stata (version 15.1). The combined prevalence and 95% confidence interval of VitD deficiency and insufficiency were calculated by the random-effects model. Egger’s and Begger’s tests were performed to assess publication bias, and p < 0.05 was considered statistically significant. Subgroup analyses (i.e., case number, age, gender, in/out-patient, acute/chronic injury, injury level, injury duration, injury extent, etc.) were carried out to discuss and clarify the source of heterogeneity.
3. Results
3.1. Literature selection
We initially identified 1,341 publications after the systematic search in the four databases, including 135 from Medline, 491 from Embase, 429 from Scopus, and 286 from Web of Science. Among these, 634 papers were removed as duplicates and 707 unique studies were assessed for eligibility according to article type, title, abstract, and full texts (Figure 1). Two papers were supplemented from the references list of existing publications. Ultimately, 35 studies were included for the further systematic review and meta-analysis and presented in the supplemental material. There were 21 studies showing post-SCI VitD status (Table 1, (22–34) and Table 2, (35–42)), seven studies regarding the adverse effects associated with a low level of VitD (Table 3, (22, 23, 27, 28, 33, 43, 44)), eight studies that evaluated the potential therapeutic effect of VitD supplement (Table 4 (17, 35, 45–50)), and five non-human experimental researches exploring the underlying mechanism of VitD after SCI (Table 5, (51–55)).
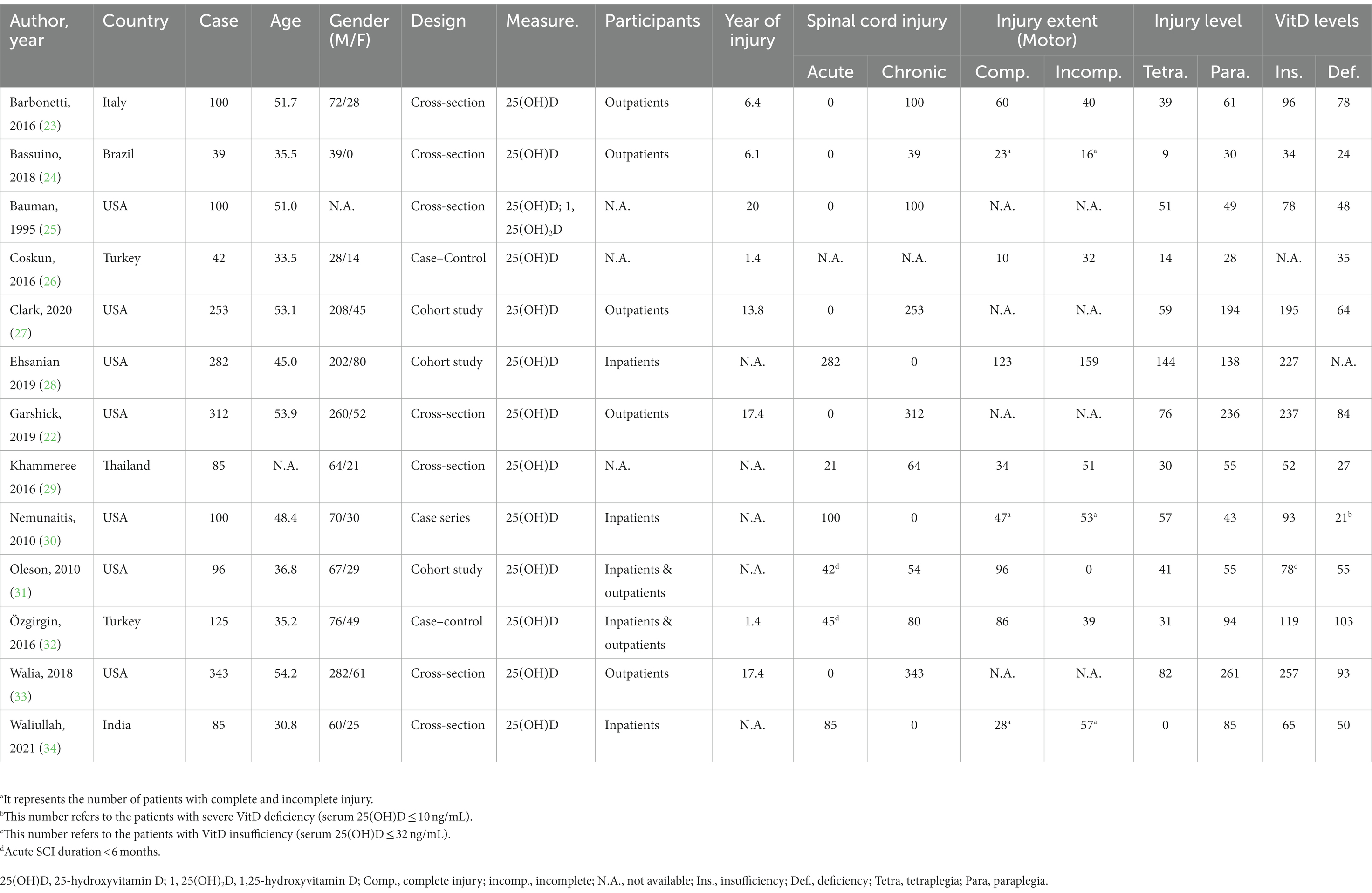
Table 1. The summarization of included studies in meta-analysis regarding the prevalence of vitamin D insufficiency and deficiency in SCI patients.
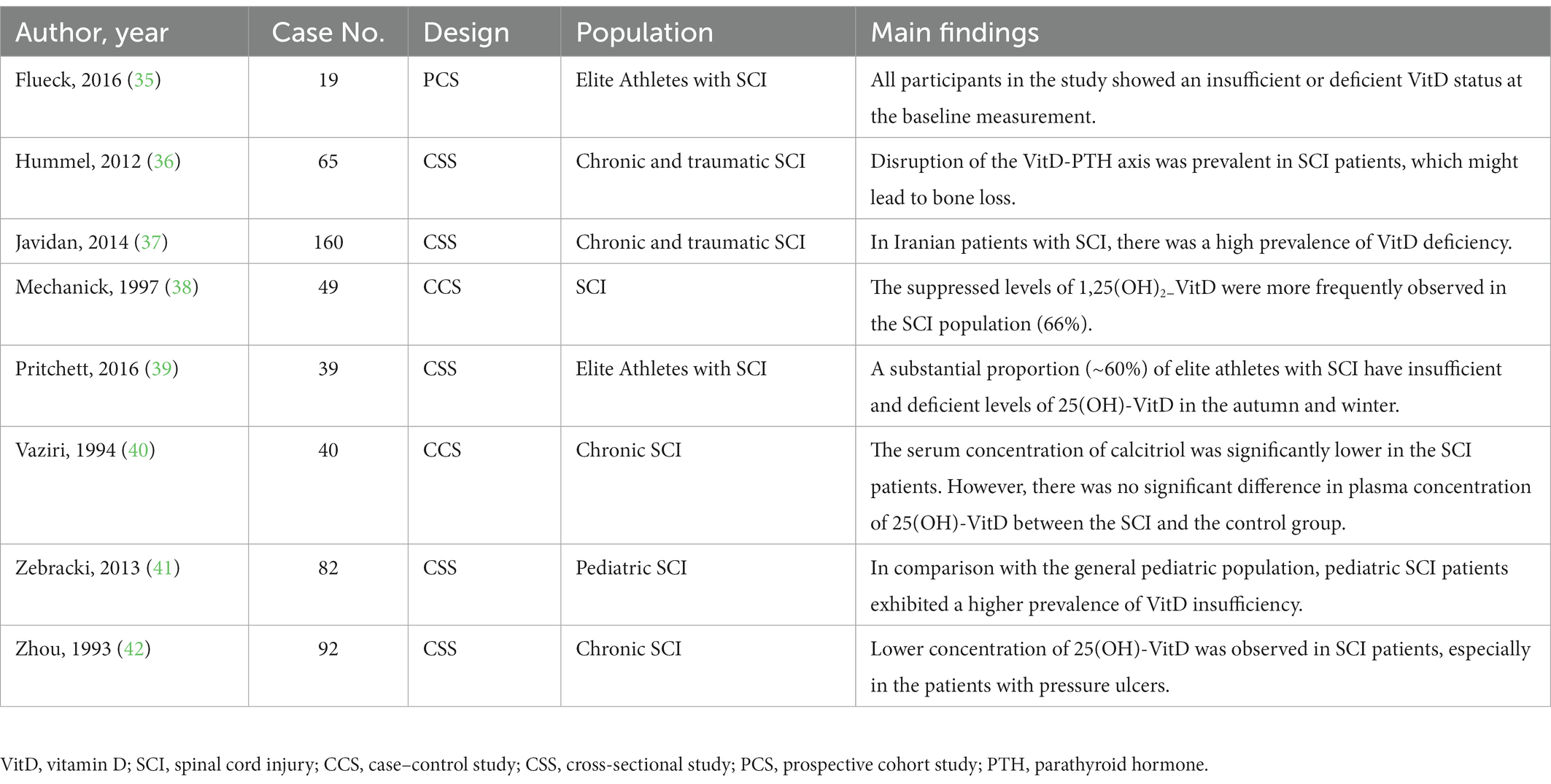
Table 2. The summary of the literature regarding VitD status after spinal cord injury (not involved in the meta-analysis).
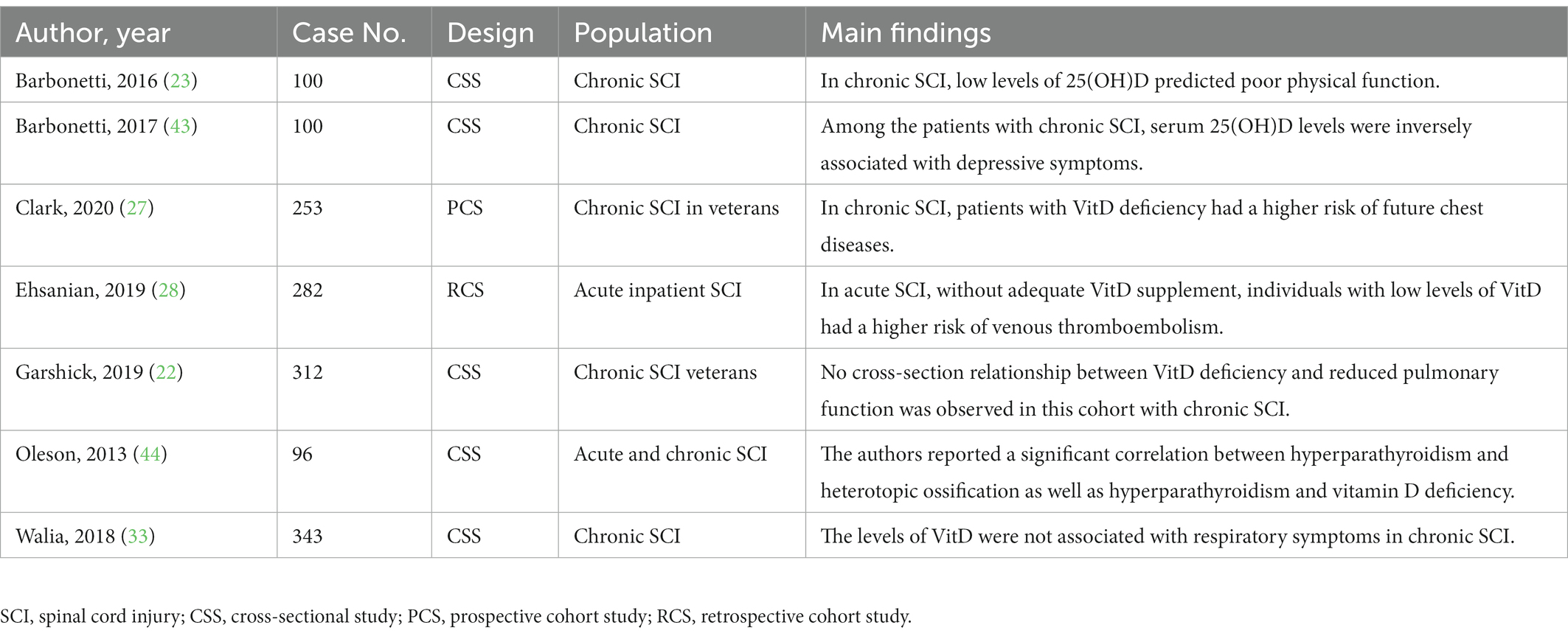
Table 3. Literature summarization of the adverse effects associated with low levels of vitamin D after spinal cord injury.
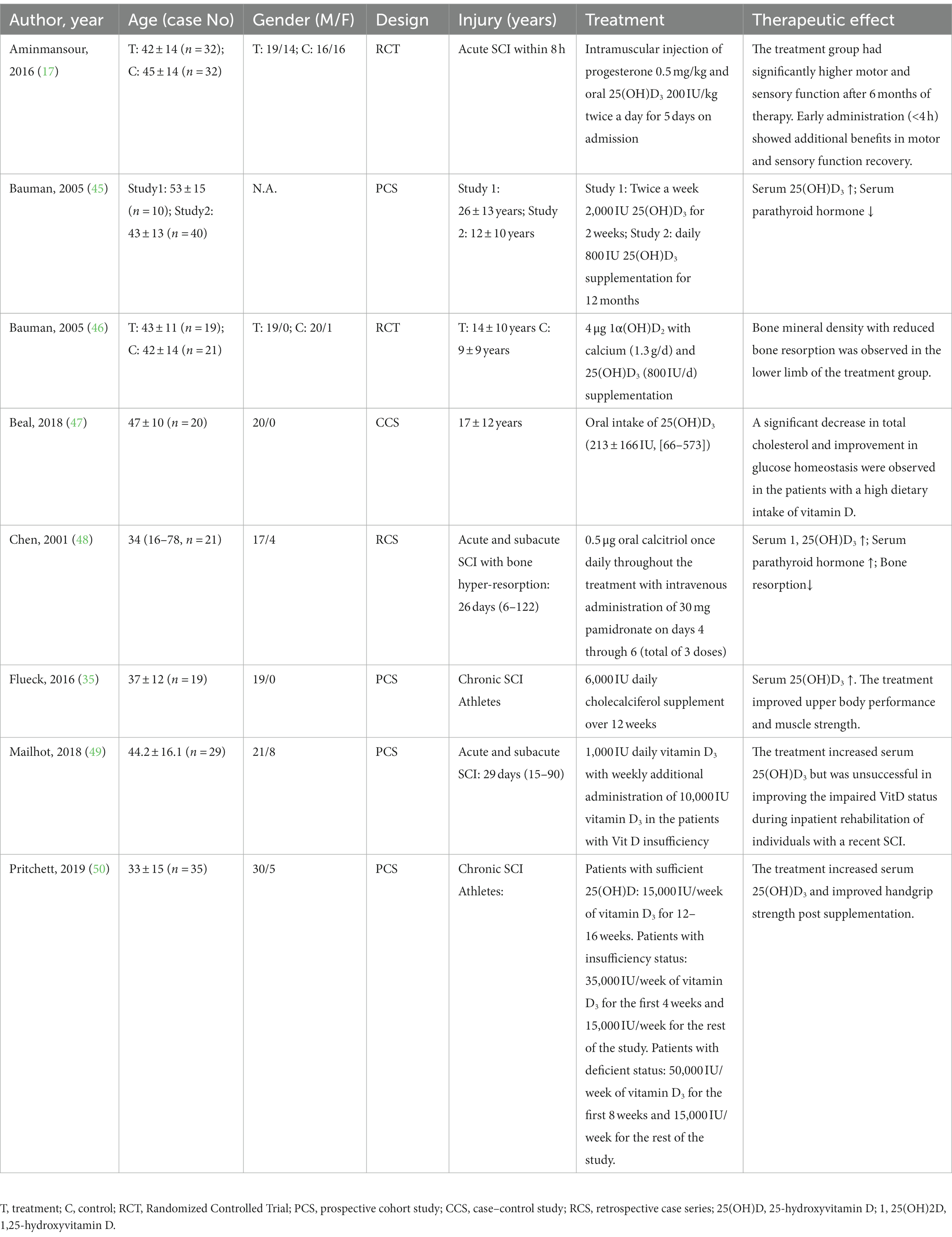
Table 4. The summary of clinical studies administrating different vitamin D supplemental regimens in SCI patients.
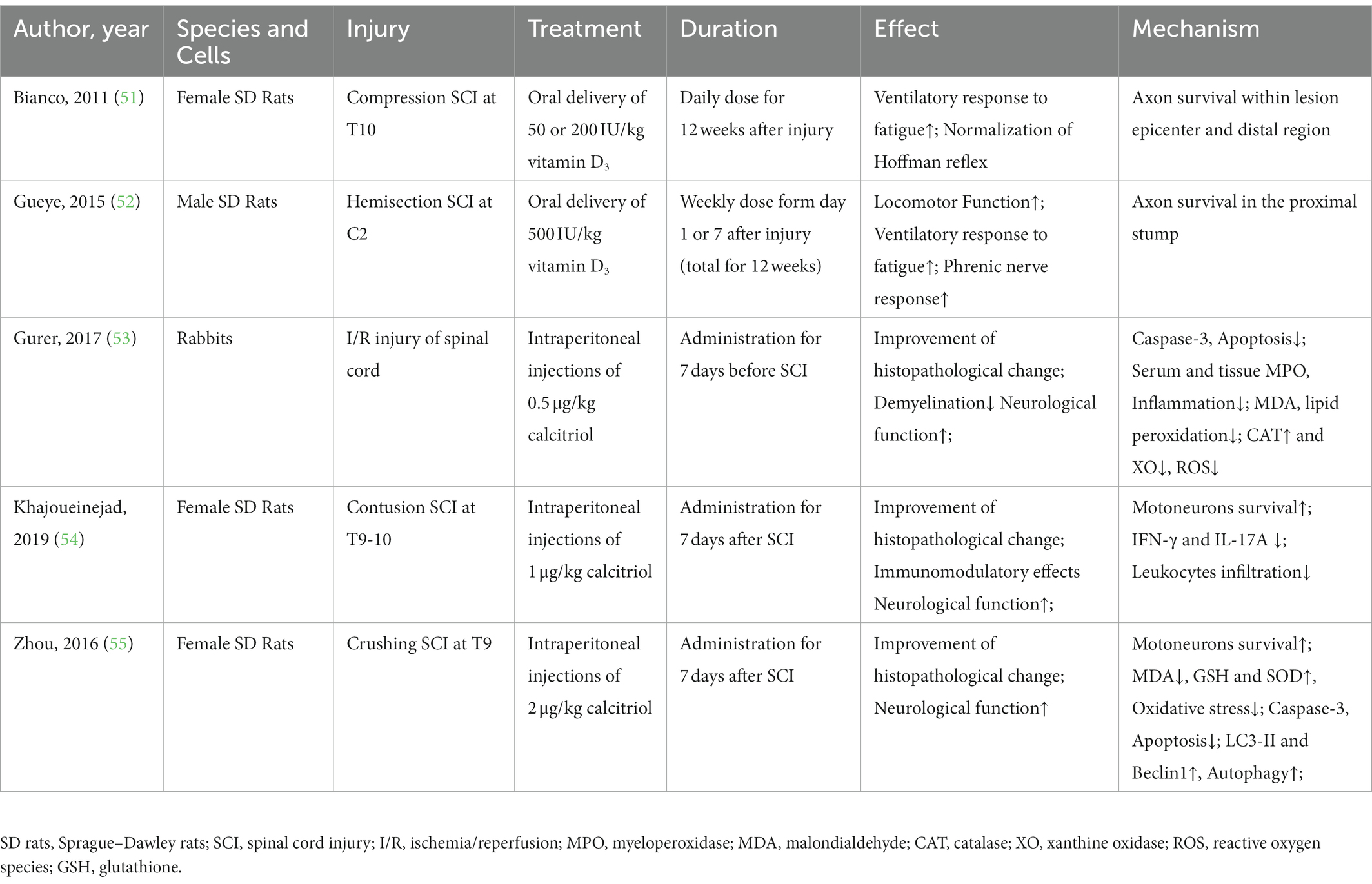
Table 5. The literature investigating the neuroprotective mechanism of Vitamin D in non-human experimental spinal cord injury.
3.2. Meta-analysis of vitamin D status in the patients with SCI
3.2.1. Included literature for the meta-analysis
In the 21 studies regarding VitD status after SCI, three studies investigated specific populations (e.g., children, athletes, etc.), one study involved patients receiving extra VitD supplements, and four studies were unqualified for meta-analysis, which were excluded accordingly. Thus, the remaining 13 investigations were included for further analysis, and their details were summarized in Table 1.
3.2.2. The prevalence of insufficient and deficient VitD status after SCI
Among the selected articles, 12 studies reported the incidence of VitD insufficiency, and 11 studies recorded the prevalence of VitD deficiency, which were analyzed, respectively. The quality score of the selected studies varied from 3 to 7 points (Supplementary Table S1).
The prevalence of insufficient VitD status after SCI was reported from 61.2% to 96.0% in the 12 eligible studies with 1,920 participants. The pooled prevalence determined by the random-effects model was 81.6% (95% CI: 75.7–87.5) with significant heterogeneity (I2 = 92.5%, p = 0.00, Figure 2A). The p values of Egger’s and Begg’s tests were 0.091 and 0.537 respectively, suggesting no significant publication bias. Subgroup analysis was performed as described (Supplementary Tables S2–S8) to further investigate potential heterogeneity sources. There was more substantial heterogeneity among studies with less than 200 patients, and years of injury less than 10 years. Additionally, insufficient VitD status were more prevalent in the studies with participants less than 200 (patients ≤ 200, p = 0.01), more female patients (male/female ≤ 3, p < 0.01) and media or mean years of injury less than 10 years (p < 0.01).
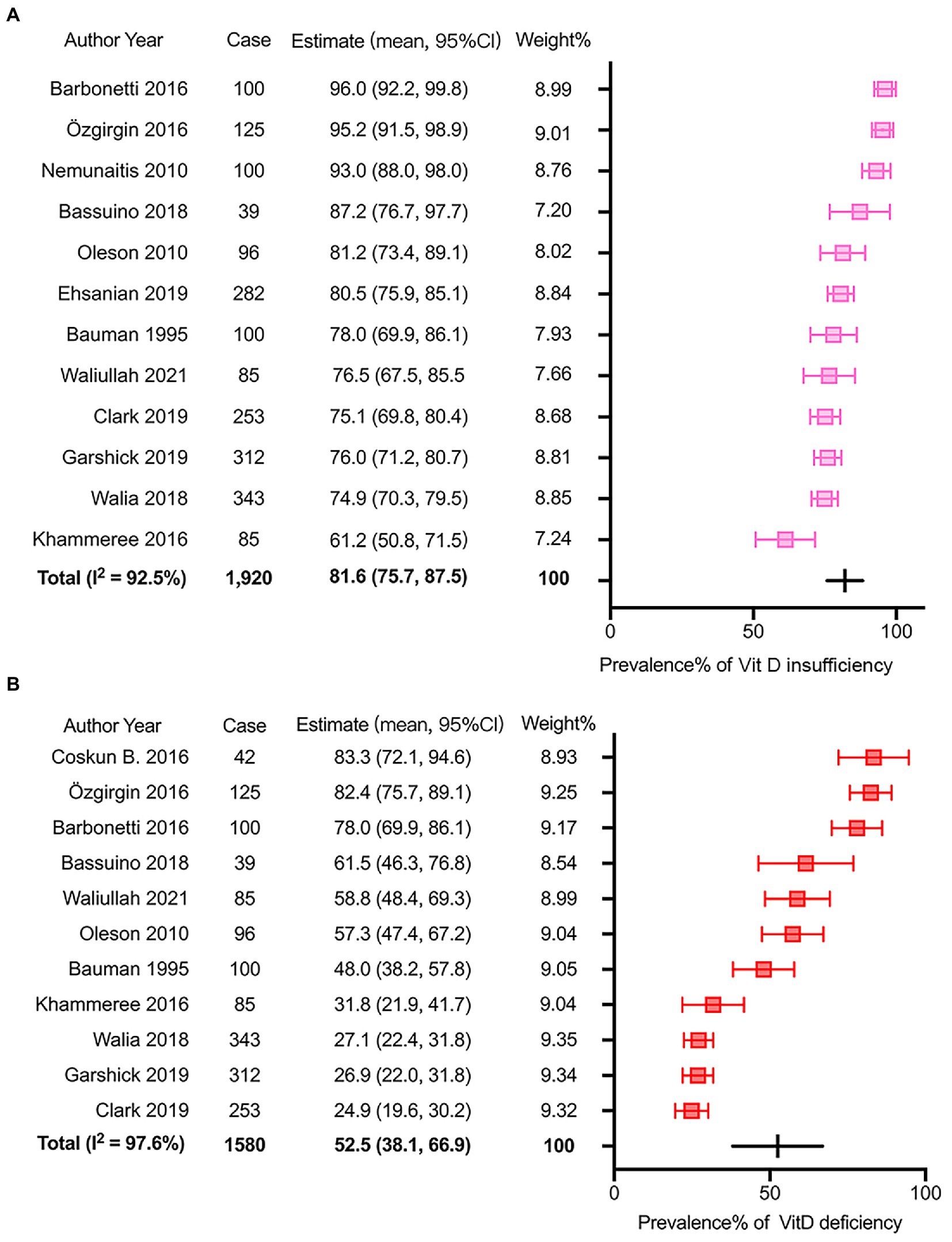
Figure 2. Meta-analysis of the prevalence of insufficient and deficient Vitamin D status after SCI. (A) the prevalence of Vitamin D insufficiency after SCI. (B) the prevalence of Vitamin D deficiency after SCI.
Regarding the prevalence of VitD deficiency after SCI, 11 articles with 1,580 participants were involved in the meta-analysis. The reported incidence ranged from 24.9 to 83.3%. The overall incidence calculated via the random-effects model was 52.5% (95% CI: 38.1–66.9). The I2 was 97.6%, which suggested significant heterogeneity among selected studies (p < 0.01, Figure 2B). The Egger’s and Begg’s tests exhibited that p values were 0.051 and 0.213 respectively, suggesting no significant publication bias. A similar subgroup analysis was performed to evaluate potential heterogeneity (Supplementary Tables S2–S8). More substantial heterogeneity was also observed in the research with less than 200 patients, and years of injury less than 10 years. Besides, VitD deficiency was more severe in the studies with smaller sample size (patients ≤ 200, p < 0.01), younger patients (mean or media Age ≤ 46 years, p < 0.01), more female patients (male/female ≤ 3, p < 0.01), and media or mean years of injury less than 10 years (p < 0.01).
3.3. Potential adverse impact of VitD insufficiency and deficiency on the post-SCI complications
Through this systematic literature search, we found seven eligible studies regarding on the post-SCI complications which might be associated with low levels of VitD (Table 3). The potential adverse impact included poor physical function (23), depressive symptoms (43), venous thromboembolism (28), heterotopic ossification and hyperparathyroidism (44), chest and respiratory symptoms (22, 27, 33).
3.4. Therapeutic effects of vitamin D supplements on the outcome of SCI
Earlier research suggested insufficient levels of VitD were prevalent among persons with SCI. The recommended dietary allowance for VitD is 600 IU/d for ages 1–70 years and 800 IU/d for those >70 years per the dietary reference intake report for calcium and VitD from the Institute of Medicine (56). Supplemental treatments with a dose similar to or higher than the VitD recommended dietary intakes were adopted in SCI patients to maintain an appropriate VitD level. Among the selected studies, there was eight clinical reports exhibiting the therapeutic effects of VitD supplements on the outcome of acute and chronic SCI. The existing literature suggested the VitD supplements had multiple beneficial effects on post-SCI rehabilitation, including correcting dysregulation of VitD-PTH axis (35, 45, 49, 50), reducing bone resorption (46, 48), facilitating motor and sensory functional restoration (17, 35, 50), improving carbohydrate metabolism (47), etc. (Table 4).
3.5. Neuroprotective effect of vitamin D in non-human experimental SCI
In the non-human experimental SCI, we retrieved five studies supporting the neuroprotective effect of VitD supplementation (51–55). The proposed mechanisms are multidimensional, involving promoting axonal survival (51, 52), reducing neuronal loss by attenuating oxidative stress (53, 55), suppressing neuroinflammation (54) and modulation of autophagy ((55), Table 5).
4. Discussion
In this systematic review, we included a total of 35 studies regarding its impact on the prognosis of SCI. The limited available studies suggest that the prevalence of VitD insufficiency and deficiency is relatively high in the SCI population, which may be associated with delayed neurofunctional restoration and several systematic complications. Besides, the current evidence from clinical and experimental studies shows that VitD supplement treatment may have potential benefits to accelerate rehabilitation in mechanistically related processes after SCI.
4.1. The prevalence of insufficient and deficient VitD status after SCI
Previous literature regarding the VitD status after SCI was limited, and existing data remains controversial. Several reports from Veterans Affairs hospitals in the US observed that the prevalence of VitD deficiency was around 25% in chronic SCI populations (22, 27). In contrast, Coskun Benlidayi et al. (26) and Özgirgin et al. (32) reported the rate could reach 80%–90% in SCI participants. We thus performed the meta-analysis in this review to estimate the prevalence of insufficient and deficient VitD status in the general SCI population worldwide. Overall, we retrieved 13 eligible papers with 1,962 participants. Among them, 81.6% of SCI patients had a serum total 25(OH)D less than 30 ng/ml and 52.5% of participants with VitD deficiency. Compared with the general population, pediatric SCI patients exhibited a higher prevalence of VitD insufficiency (41). Additionally, Pritchett et al. (39) noticed that a substantial proportion (~60%) of elite athletes with SCI also had insufficient and deficient levels of 25(OH)D in the autumn and winter. Taken together, there was a high prevalence of VitD insufficiency and deficiency in the SCI population.
4.2. Potential adverse impact of VitD insufficiency and deficiency on the post-SCI complications
VitD plays a fundamental role in calcium and phosphate metabolism. Its insufficiency or deficiency is associated with a higher risk of bone diseases (57). Histological investigations recently indicated that VDRs have a wide distribution in non-skeletal tissues, including vessels, skin, muscles, endocrine glands, kidneys, neural tissue, etc., which highlights its unique role in extra-skeletal disease (58). In the SCI population, the previous analysis showed a high prevalence of VitD insufficiency, and deficiency was associated with poor physical functions (23, 59). More importantly, this systematic review showed that VitD abnormality might be associated with several complications, which hampered functional restoration (Table 3).
The low serum VitD is associated with a higher risk of skeletal diseases after SCI (60). In the subjects with long-standing complete SCI, Frotzler et al. (61) observed considerable declines in bone density and a higher risk of historical fractures. Their findings demonstrated that bone loss after SCI could persist for an extended period. The mechanism underlying post-SCI skeletal abnormality is complicated, and recent evidence suggests that the disruption of the VitD-parathyroid hormone (PTH) axis contributes to this pathological process (36, 60). First of all, via interaction with VDR, lack of VitD may affect these osteoblasts and osteoclasts and lead to the disruption of bone microstructure and mass. Besides, low-level VitD results in dysregulation of calcium and phosphate homeostasis and abnormal fluctuations of PTH, which has an adverse effect on bones. In the chronic stage of SCI (more than 1 year), Bauman et al. (25) demonstrated a depressed level of VitD and reduction of the serum calcium concentration, which might lead to mild secondary hyperparathyroidism and accelerate the development of osteoporosis. Furthermore, in a study involving 96 SCI individuals, Oleson et al. (44) found that there was a correlation between hyperparathyroidism and heterotopic ossification as well as hyperparathyroidism and VitD deficiency, in which they inferred that low VitD and elevated PTH might increase the risk of heterotopic ossification.
VitD insufficiency and deficiency were also associated with a high risk of venous thromboembolism. Experimental data exhibited that VitD and VDR modulate the expression and activity of multiple coagulation-related proteins (e.g., plasminogen activator inhibitor-1, thrombospondin-1, etc.), which serve as adjunctive antithrombotic agents (62, 63). In a retrospective cohort study involving 282 acute SCI patients, a higher incidence of venous thromboembolism was noted in the subjects with VitD levels <30 ng/ml and an absence of VitD supplementation, which was consistent with the findings in other neurological injuries (28, 64).
Another emerging field of interest regarding VitD-related complications is secondary neurological disorders. VDR is known to express in both neuronal and glial cells in CNS, and VitD is involved in the regulation of neural differentiation and development, modulation of neuroinflammation, maintaining neuroplasticity, and expression of neurotrophins in various physiological and pathological contexts, which provide a rationale for the link between VitD and neurological comorbidities after SCI (65). Barbonetti et al. (43) recently looked into depression after SCI, and they observed that serum 25(OH)D levels were inversely associated with the psychiatric symptoms in the chronic stage. Whereas data is still limited, and future research is needed to clarify the impact of VitD on neurological comorbidity after SCI.
Previous studies in non-SCI populations reported a positive correlation between low VitD levels and increased risk of respiratory diseases (66, 67). Based on this, several research groups looked into the potential association between chest illness and VitD status after SCI. In 2018, Garshick et al. (22) and Walia et al. (33) accessed the cross-sectional associations between respiratory symptoms/pulmonary function and serum VitD levels in chronic SCI patients, and their analysis failed to establish the association. However, the researchers indeed observed that chronic obstructive pulmonary disease and low VitD levels coexisted in some SCI individuals and VitD supplementation might be beneficial in maintaining respiratory health (22, 33). Therefore, to further investigate the association between VitD levels and chest illness, Clark et al. (27) performed a prospective observational study, and they revealed that the reduction of VitD levels might be associated with an increased risk of future chest illness in chronic SCI, particularly in persons with deficient levels. Currently, high-quality evidence is still lacking in the field, and more clinical studies with rigorous design are needed to validate the association between VitD levels and chest illness.
Taken together, we think that SCI patients are at higher risk of developing VitD insufficiency and deficiency, and its underlying mechanism may be associated with the lifestyle change, post-SCI complications, and the corresponding vicious cycle (Figure 3). First of all, the primary and secondary injury in the spinal cord leads to severe motor and sensory dysfunction. Due to the impaired mobility and pain, adequate exposure to sunlight is difficult to achieve in those individuals as most have to stay indoors with decreased physical activity, which has a negative impact on the VitD3 synthesis in the skin (68). Secondly, there is converging evidence indicating that dramatic changes in social and family environments after SCI may trigger significant psychiatric stress, which may lead to comorbid psychiatric disorders (such as depression, anxiety, post-traumatic stress disorder, etc.) and makes them more vulnerable to VitD insufficiency (69, 70). It is noteworthy that the researchers also indicated that VitD was a negative acute phase reactant, and post-SCI systematic inflammation might decrease the levels of plasma VDBP, which exacerbated VitD insufficiency (71). Thirdly, SCI patients usually develop multiple complications and comorbidities, such as skeletal diseases, chest illness, neurological sequela, venous thromboembolism, intestinal dysfunction, etc., which may directly result in the lack of VitD or change the lifestyle and affect the level of VitD secondarily (72, 73). For example, persons with SCI are likely to develop pressure ulcers and dysregulation of intestinal microflora with significant diet change, which may affect VitD level synergically via impairing its food supply and absorption (42, 74). Notably, the low levels of VitD can also aggravate functional deficits, psychological stress and co-existing diseases, which start up a vicious cycle and hamper functional restoration after SCI.
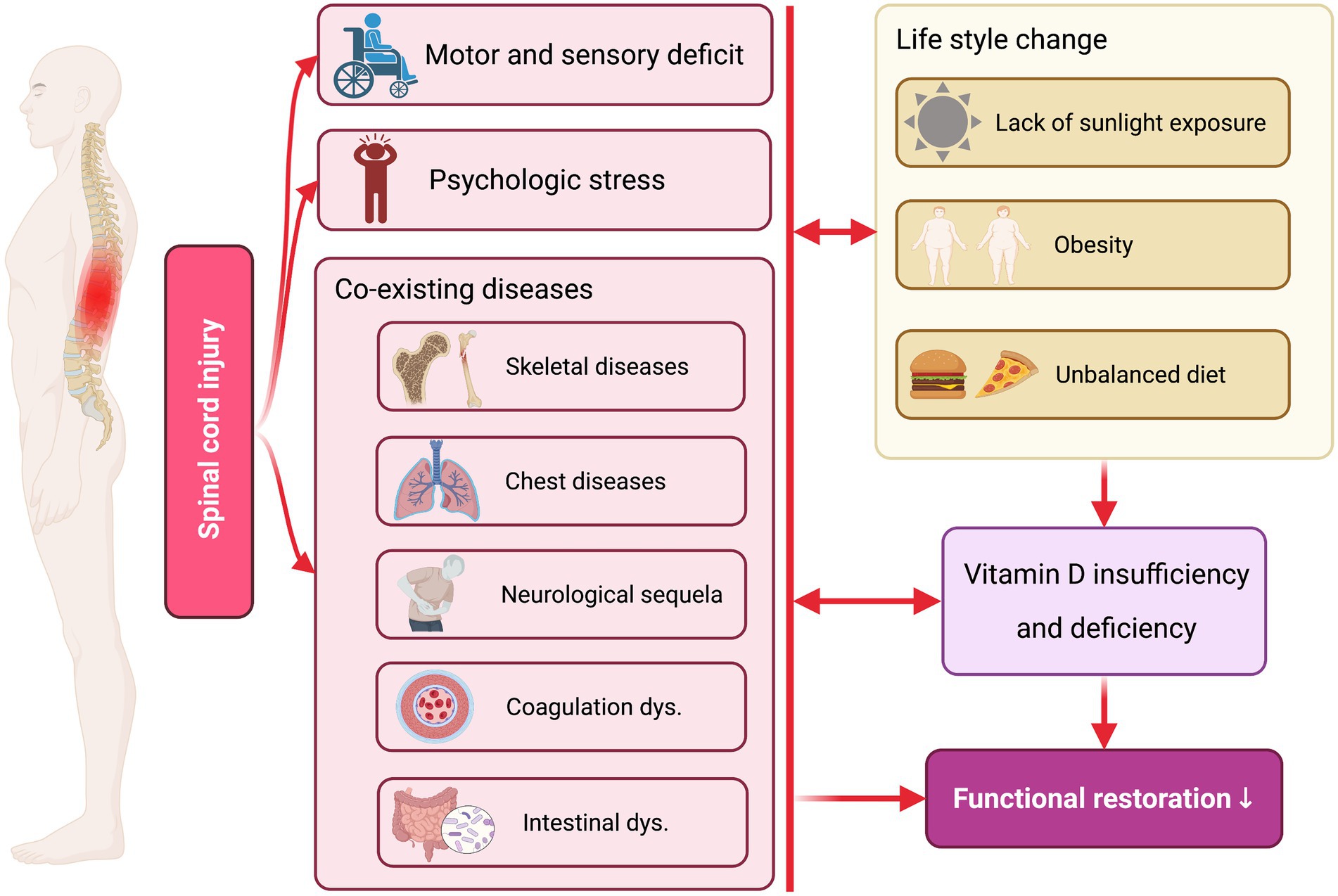
Figure 3. The potential mechanism underlying low Vitamin D levels after SCI. The primary and second injuries after SCI directly lead to severe motor and sensory deficits, psychological stress, and multiple co-existing diseases, which subsequently alter the patients’ lifestyle (e.g., lack of sunlight exposure, obesity, unbalanced diet, etc.). Subsequently, post-SCI functional deficits and unhealthy lifestyles contribute to reduced VitDlevelsl in the patients. Notably, the low levels of VitD can also aggravate functional deficits, psychological stress and co-existing diseases, which start up a vicious cycle and hamper functional restoration after SCI. Created with BioRender.com.
4.3. The therapeutic effects of vitamin D supplements on the outcome of SCI
In the acute and chronic stages of SCI, VitD supplemental treatment can substantially increase VitD concentration and modulate abnormal PTH fluctuation. In 2005, Bauman et al. (45) postulated two supplemental regimens for chronic SCI: the short-term regimen, 2,000 IU 25(OH)D3 twice a week for 2 weeks, and the long-term regimen, 800 IU 25(OH)D3 daily for 12 months, both of which led to a significant increase of plasma 25(OH)D levels and suppression of plasma PTH. However, they also noticed that the two plans failed to normalize serum VitD levels, which indicated higher doses and longer administration periods were required for the supplementation (45). Notably, later in 2013, they successfully developed an oral regimen for VitD replacement in the chronic SCI population. The patients were administered VitD3 at a dose of 2,000 IU daily for 3 months with 1.3 g oral calcium supplementation per day. Normal levels of VitD with a significant decrease in PTH in six of seven participants were restored at the end of the experiment (75). Meanwhile, in recent SCI with complete or incomplete sensorimotor impairments, Mailhot et al. (49) evaluated a VitD repletion protocol, in which participants were given 1,000 IU 25(OH)D3 daily for approximately 6 weeks with extra weekly administration of 10,000 IU 25(OH)D3 in the patients with VitD insufficiency. They found that the treatment increased serum 25(OH)D3 but was unsuccessful in improving the impaired VitD status.
Administration of VitD in SCI facilitates motor and sensory functional restoration. Recently, clinicians performed a randomized trial to assess the effects of progesterone and VitD on functional restoration after acute traumatic SCI. Their findings indicated that the synergic administration improved motor and sensory function after SCI (17). VitD treatment in wheelchair athletes with chronic SCI not only helps them maintain an adequate level of serum VitD but also improves their muscle strength. In a double-blinded study involving 20 indoor wheelchair athletes with VitD insufficiency, researchers administrated 6,000 IU cholecalciferol supplements daily over 12 weeks. The supplemental therapy restored VitD status to an optimal level and seemed to improve upper body performance and muscle strength (35). Similarly, Pritchett et al. (50) adopted a refined and hierarchical protocol pending on the baseline VitD status that exhibited VitD supplementation could increase the serum level of VitD, and improve handgrip strength in the elite athletes with chronic SCI.
There was also evidence supporting the beneficial effect of VitD supplementation on skeletal diseases. The addition of 1α(OH)D2 on the basis of routine calcium and VitD supplementation for 1 year was reported to increase the bone mineral density of lower limbs in chronic SCI at 6 months after treatment when compared with the placebo administration (46). In acute and subacute SCI, Chen et al. (48) combined calcitriol and pamidronate therapy, and the treatment significantly inhibited bone hyper-resorption via normalization of the VitD-PTH axis. Additionally, a case–control study investigating dietary VitD intakes in chronic SCI suggested that a higher dietary intake of VitD could influence cholesterol and glucose homeostasis, which improved carbohydrate metabolism (47).
4.4. Neuroprotective effect of vitamin D in non-human experimental SCI
In the non-human experimental SCI, we retrieved five studies supporting the neuroprotective effect of VitD supplementation (51–55). The proposed mechanisms are multidimensional, involving promoting axonal survival (51, 52), reducing neuronal loss by attenuating oxidative stress (53, 55), suppressing neuroinflammation (54) and modulation of autophagy ((55), Table 5). Oral delivery of VitD for 4 months in a compression SCI model at T10 level improved respiratory adjustment to fatigue and normalized Hoffman reflex via increasing the number of axons crossing the lesion site (51). Later, the same research group replicated the findings in a hemisection SCI model at a higher level (C2) (52). Notably, the short-term administration of calcitriol immediately after SCI was also reported to attenuate the histological damage and neuron loss by reducing oxidative stress, inhibiting apoptosis, and promoting autophagy. In addition, pretreatment of calcitriol before SCI also exhibited a protective effect on the ischemia/reperfusion injury of the spinal cord, which was mediated by inhibiting neuronal apoptosis and suppressing regional and general oxidative stress (53). The immunomodulatory property of VitD raises the potential that it may alter the functional status of microglia/macrophages and astrocytes, the key players in the post-SCI neuroinflammation, to improve repairment. Indeed, Khajoueinejad et al. (54) observed that VitD had an immunomodulatory effect on the proliferative response of lymphocytes in the spleen and lymph nodes, which was associated with reduced secretion of proinflammatory cytokines (IFN-γ and IL-17A) and less leukocyte infiltration into the lesion center. However, our knowledge regarding the neuroprotective mechanism of VitD is still limited, and well-designed mechanistic research is needed to elucidate its underlying mechanism.
From the evidence of VitD’s effect in both SCI and other neurological disease models, we speculated that the potential neuroprotective effect of Vitamin D might be mediated by its genomic and non-genomic effects (Figure 4). First of all, VitD diffuses through the cell membrane, binds to VDR, and dimerizes with RXR, which then translocates into the nucleus and binds to VitD response element (VDRE). VDRE locates on a large number of genes and its binding with VitD-VDR-RXR complex leads to the transcription of target genes, which could modulate inflammatory response (54, 76), attenuate oxidative stress via Nrf2 and Klotho pathway (53, 55, 77, 78), maintain intracellular calcium homeostasis (79), enhance the expression of multiple growth factors and neurotrophins (e.g., vascular endothelial growth factor [VEGF], brain-derived neurotrophic factor [BDNF], etc.) (80), promote axonal regeneration, angiogenesis and neurogenesis (51, 52) (81), modulation of autophagy (55), etc. (Figure 4). Besides, it was recognized that VitD could exert an immediate non-genomic effect through membrane VDR in CNS, which then modulated the functional status of calcium-and kinase-activated signaling pathways (82).
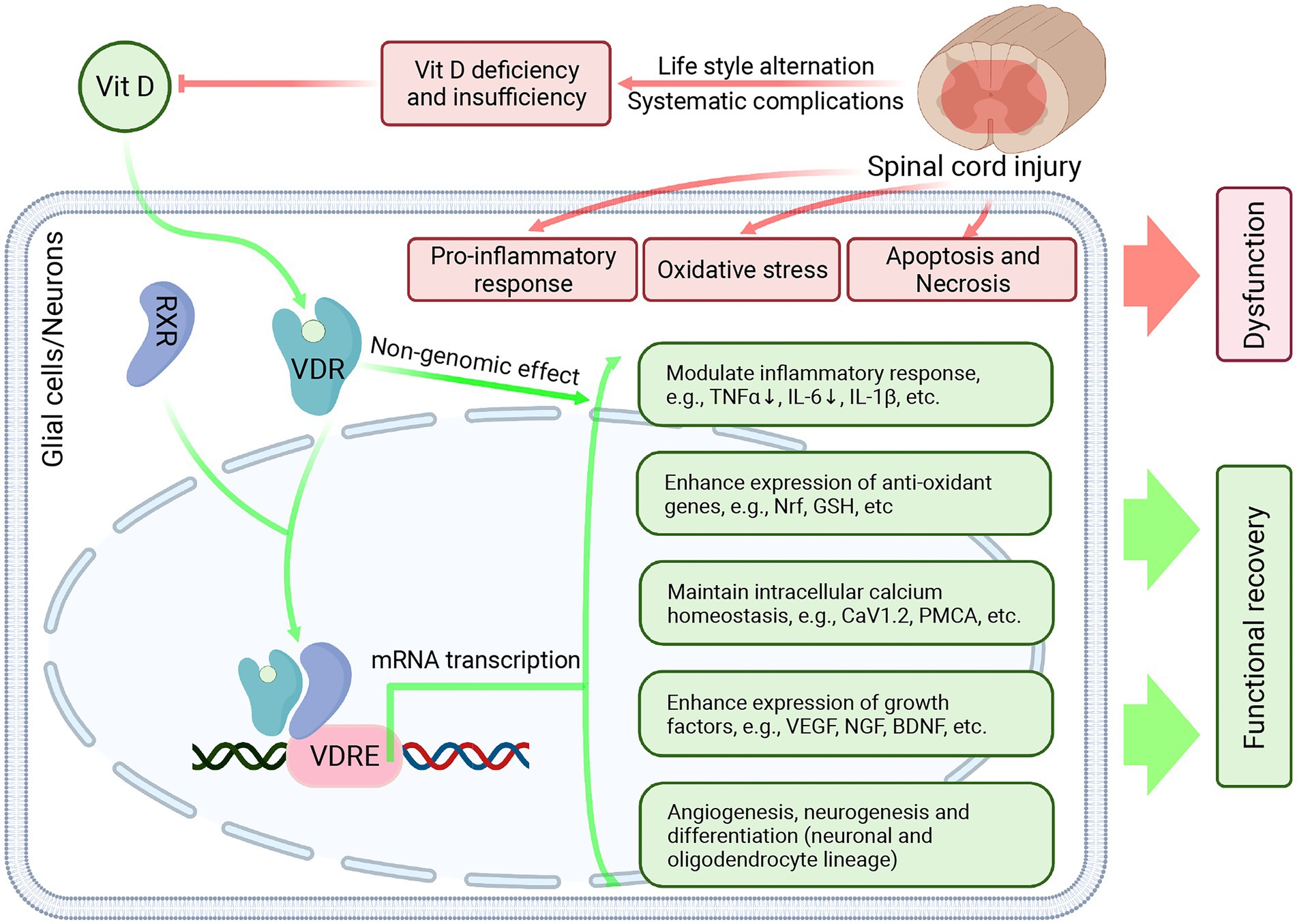
Figure 4. The potential neuroprotective effect of Vitamin D in SCI. After SCI, the primary impact and secondary injury lead to persistent inflammatory response, enhanced oxidative stress, neuronal apoptosis, and necrosis, which results in permanent functional deficits and delays neurofunctional restoration. Besides, due to lifestyle change and systematic complications, the VitD levels were significantly reduced in the SCI individuals. VitD treatment can combat secondary injury after SCI and exert a neuroprotective effect. In the glial cells and neurons, VitD treatment can facilitate its diffusion across the cell membrane. Then VitD binds to VDR and dimerizes with RXR. The VitD-VDR-RXR complex then translocates into the nucleus, binds to VDRE, and promotes the transcription of target genes, which can modulate inflammatory response, attenuate oxidative stress, maintain intracellular calcium homeostasis, enhance the expression of multiple growth factors and neurotrophins, promote angiogenesis and neurogenesis. Created with BioRender.com.
4.5. Future direction
In this systematic review, we retrieved a multi-level of evidence supporting the beneficial effects of VitD on post-SCI rehabilitation. However, the neuroprotective mechanisms of VitD have not been elucidated by current experimental studies, and most clinical studies in the field are observational and small-scale, which limits its wide application.
In the experimental research, more in-depth in vitro and in vivo investigations are needed to explicate the precise molecular mechanism underlying the neuroprotective effect of VitD. In the adult brain, VitD has both genomic and non-genomic actions on various neurological functions (82). It remains to be seen whether VitD could exert neuroprotection via similar VDR-depended mechanisms in the context of SCI. In particular, VitD modulated inflammatory response in CNS and immune systems (83). Future researchers should elucidate the exact effect of VitD in the systematic inflammation and neuroinflammation after SCI (84). Furthermore, VitD can enhance neural stem cell proliferation and differentiation into neurons and oligodendrocytes (85, 86). It would be interesting to explore whether VitD could interact with neural stem cells to promote neurons and myelin regeneration after SCI via enhancing and modulating endogenous neurogenesis (87–89). Recent literature indicated that small molecules combined with collagen hydrogel directed neurogenesis and migration of neural stem cells after implantation in the lesioned spinal cord (90). As consecutive systematic administration of VitD is known to have poor delivery efficiency in the lesion site and lead to adverse effects in a large dose, topical application of VitD treatment via a combination of hydrogel, small molecules, cells, and other small-releasing systems is a promising therapeutic strategy towards SCI.
In future clinical studies, clinicians should design large-scale, double-blinded, and random-control trials to validate the therapeutic effect of VitD as an adjuvant treatment on the functional restoration following SCI. We should explore and standardize the administration protocol of VitD monitoring and supplementation (e.g., dose, therapeutic window, duration, etc.) in the acute and chronic stages of SCI. Another interesting direction is combining VitD treatment with other micronutrients and medicine (e.g., progesterone, Vitamin E, Vitamin C, etc.) and determining whether they have a synergic effect on the improvement of SCI rehabilitation (17, 91).
4.6. Limitations
Our systematic review with meta-analysis has several limitations. First, in the meta-analysis of the prevalence of VitD insufficiency and deficiency, there is high heterogeneity among included studies. To diminish its impact on the validity of the results, a single-arm meta-analysis with random effect was applied. We also performed the subgroups analysis to explore the source of heterogeneity and identified more substantial heterogeneity among studies with patients less than 200 and years of injury less than 10 years. However, the high heterogeneity inevitably limits the outcomes of this meta-analysis. Second, in the systematic review, most of the included clinical papers are observational, hospital-based, and performed in different countries, which can introduce potential bias. Third, in the clinical studies, the inclusion and exclusion criteria vary, and the participants have different baseline levels of VitD, which may bias the results.
5. Conclusion
Based on the discovered protective and bioactive effect of VitD on neurological disorders, we hypothesize that VitD might play an essential role in post-SCI rehabilitation. Through this meta-analysis and systematic review, we retrieved multi-level evidence that supported this hypothesis, including (1) there was a high prevalence of VitD insufficiency and deficiency in the SCI population, (2) low-level of VitD was associated with several complications, which hampered the functional restoration, (3) the supplement treatment might have potential benefits to accelerate rehabilitation in mechanistically related processes after SCI. However, due to the limitation of the evidence, our results should be interpreted carefully, and more well-designed randomized controlled trials and mechanism experimental research are needed to validate its therapeutic effect, elucidate its neuroprotective mechanism, and develop novel treatments.
Data availability statement
The original contributions presented in the study are included in the article/supplementary material, further inquiries can be directed to the corresponding authors.
Author contributions
LW and DL: conceptualization, data curation, funding acquisition, project administration, supervision, writing—original draft, and writing—review and editing. LW, JG, JW, and YZ: formal analysis, investigation, methodology, resources, software, validation, and visualization. All authors contributed to the article and approved the submitted version.
Funding
This work was supported by National Nature Science Foundation of China (grant number 81771334), Natural Science Foundation of Hubei Province of China (grant number 2017CFB706) and Free Innovation Fund of Wuhan Union Hospital (grant number 2021xhyn105). The funders had no role in study design, data collection and analysis, decision to publish, or preparation of the paper.
Conflict of interest
The authors declare that the research was conducted in the absence of any commercial or financial relationships that could be construed as a potential conflict of interest.
Publisher’s note
All claims expressed in this article are solely those of the authors and do not necessarily represent those of their affiliated organizations, or those of the publisher, the editors and the reviewers. Any product that may be evaluated in this article, or claim that may be made by its manufacturer, is not guaranteed or endorsed by the publisher.
Supplementary material
The Supplementary material for this article can be found online at: https://www.frontiersin.org/articles/10.3389/fnut.2023.920998/full#supplementary-material
Abbreviations
BDNF, Brain-derived neurotrophic factor; CNS, Central nerves system; IL, Interleukin; NIH, National Institutes of Health; NMDA, N-methyl-D-aspartic acid; PTH, Parathyroid hormone; RXR, Retinoid X receptor; SCI, Spinal cord injury; TNF-α, Tumor necrosis factor-alpha; VDBP, Vitamin D binding protein; VDR, Vitamin D receptor; VDRE, Vitamin D response element; VEGF, Vascular endothelial growth factor; VitD, Vitamin D; UVB, Ultraviolet B.
Footnotes
References
1.Xu, L, Liu, B, Li, P, Li, J, Wang, J, Han, J, et al. Correlations of serum hormones and bone mineral density with fracture and balance ability of postmenopausal patients and effects of calcitriol. Med Sci Monit. (2018) 24:7309–15. doi: 10.12659/MSM.910792
2.Palacios, C, and Gonzalez, L. Is vitamin D deficiency a major global public health problem? J Steroid Biochem Mol Biol. (2014) 144:138–45. doi: 10.1016/j.jsbmb.2013.11.003
3.Schleicher, RL, Sternberg, MR, Looker, AC, Yetley, EA, Lacher, DA, Sempos, CT, et al. National Estimates of serum Total 25-Hydroxyvitamin D and metabolite concentrations measured by liquid chromatography-tandem mass spectrometry in the US population during 2007–2010. J Nutr. (2016) 146:1051–61. doi: 10.3945/jn.115.227728
4.Herrick, KA, Storandt, RJ, Afful, J, Pfeiffer, CM, Schleicher, RL, Gahche, JJ, et al. Vitamin D status in the United States, 2011–2014. Am J Clin Nutr. (2019) 110:150–7. doi: 10.1093/ajcn/nqz037
5.Cashman, KD, Dowling, KG, Škrabáková, Z, Gonzalez-Gross, M, Valtueña, J, De Henauw, S, et al. Vitamin D deficiency in Europe: pandemic? Am J Clin Nutr. (2016) 103:1033–44. doi: 10.3945/ajcn.115.120873
6.Lazo, MG, Shirazi, P, Sam, M, Giobbie-Hurder, A, Blacconiere, MJ, and Muppidi, M. Osteoporosis and risk of fracture in men with spinal cord injury. Spinal Cord. (2001) 39:208–14. doi: 10.1038/sj.sc.3101139
7.Boonen, S, Bischoff-Ferrari, HA, Cooper, C, Lips, P, Ljunggren, O, Meunier, PJ, et al. Addressing the musculoskeletal components of fracture risk with calcium and vitamin D: a review of the evidence. Calcif Tissue Int. (2006) 78:257–70. doi: 10.1007/s00223-005-0009-8
8.Wecht, JM, Krassioukov, AV, Alexander, M, Handrakis, JP, McKenna, SL, Kennelly, M, et al. International standards to document autonomic function following SCI (ISAFSCI): second edition. Top Spinal Cord Inj Rehabil. (2021) 27:23–49. doi: 10.46292/sci2702-23
9.Boehl, G, Raguindin, PF, Valido, E, Bertolo, A, Itodo, OA, Minder, B, et al. Endocrinological and inflammatory markers in individuals with spinal cord injury: a systematic review and meta-analysis. Rev Endocr Metab Disord. (2022) 23:1035–50. doi: 10.1007/s11154-022-09742-9
10.Naftchi, NE. Alterations of neuroendocrine functions in spinal cord injury. Peptides. (1985) 6:85–94. doi: 10.1016/0196-9781(85)90015-4
11.Calvello, R, Cianciulli, A, Nicolardi, G, De Nuccio, F, Giannotti, L, Salvatore, R, et al. Vitamin D treatment attenuates Neuroinflammation and dopaminergic Neurodegeneration in an animal model of Parkinson's disease, shifting M1 to M2 microglia responses. J Neuroimmune Pharmacol. (2017) 12:327–39. doi: 10.1007/s11481-016-9720-7
12.Montava, M, Garcia, S, Mancini, J, Jammes, Y, Courageot, J, Lavieille, JP, et al. Vitamin D3 potentiates myelination and recovery after facial nerve injury. Eur Arch Otorhinolaryngol. (2015) 272:2815–23. doi: 10.1007/s00405-014-3305-y
13.Eyles, DW, Burne, THJ, and McGrath, JJ. Vitamin D, effects on brain development, adult brain function and the links between low levels of vitamin D and neuropsychiatric disease. Front Neuroendocrinol. (2013) 34:47–64. doi: 10.1016/j.yfrne.2012.07.001
14.Tallqvist, S, Kauppila, AM, Vainionpää, A, Koskinen, E, Bergman, P, Anttila, H, et al. Prevalence of comorbidities and secondary health conditions among the Finnish population with spinal cord injury. Spinal Cord. (2022) 60:618–27. doi: 10.1038/s41393-021-00704-7
15.Canpolat, U, Özcan, F, Özeke, Ö, Turak, O, Yayla, Ç, Açıkgöz, SK, et al. Impaired cardiac autonomic functions in apparently healthy subjects with vitamin D deficiency. Ann Noninvasive Electrocardiol. (2015) 20:378–85. doi: 10.1111/anec.12233
16.Otero, TMN, Canales, C, Yeh, DD, Elsayes, A, Belcher, DM, and Quraishi, SA. Vitamin D status is associated with development of hospital-acquired pressure injuries in critically ill surgical patients. Nutr Clin Pract. (2019) 34:142–7. doi: 10.1002/ncp.10184
17.Aminmansour, B, Asnaashari, A, Rezvani, M, Ghaffarpasand, F, Amin Noorian, SM, Saboori, M, et al. Effects of progesterone and vitamin D on outcome of patients with acute traumatic spinal cord injury; a randomized, double-blind, placebo controlled study. J Spinal Cord Med. (2016) 39:272–80. doi: 10.1080/10790268.2015.1114224
18.Page, MJ, McKenzie, JE, Bossuyt, PM, Boutron, I, Hoffmann, TC, Mulrow, CD, et al. The PRISMA 2020 statement: an updated guideline for reporting systematic reviews. BMJ. (2021) 372:n71. doi: 10.1136/bmj.n71
19.National Institutes of Health (2014). Study quality assessment tools: quality assessment tool for case series studies. Bethesda, MD, U.S.A. Available at: https://www.nhlbi.nih.gov/health-topics/study-quality-assessment-tools.
20.Kirshblum, SC, Burns, SP, Biering-Sorensen, F, Donovan, W, Graves, DE, Jha, A, et al. International standards for neurological classification of spinal cord injury (revised 2011). J Spinal Cord Med. (2011) 34:535–46. doi: 10.1179/204577211x13207446293695
21.Holick, MF, Binkley, NC, Bischoff-Ferrari, HA, Gordon, CM, Hanley, DA, Heaney, RP, et al. Evaluation, treatment, and prevention of vitamin D deficiency: an Endocrine Society clinical practice guideline. J Clin Endocrinol Metabol. (2011) 96:1911–30. doi: 10.1210/jc.2011-0385
22.Garshick, E, Walia, P, Goldstein, RL, Teylan, MA, Lazzari, AA, Tun, CG, et al. Associations between vitamin D and pulmonary function in chronic spinal cord injury. J Spinal Cord Med. (2019) 42:171–7. doi: 10.1080/10790268.2018.1432305
23.Barbonetti, A, Sperandio, A, Micillo, A, D'Andrea, S, Pacca, F, Felzani, G, et al. Independent Association of Vitamin D with Physical Function in people with chronic spinal cord injury. Arch Phys Med Rehabil. (2016) 97:726–32. doi: 10.1016/j.apmr.2016.01.002
24.Bassuino, MS, Kaminski, EL, Garcia, LO, Linden, R, Antunes, MV, Schneider, RH, et al. Factors related to decreased vitamin D levels in men with spinal cord injury living in a subtropical region. Scientia. Medica. (2018) 28:28381. doi: 10.15448/1980-6108.2018.2.28381
25.Bauman, WA, Zhong, YG, and Schwartz, E. Vitamin D deficiency in veterans with chronic spinal cord injury. Metab Clin Exp. (1995) 44:1612–6. doi: 10.1016/0026-0495(95)90083-7
26.Coskun Benlidayi, I, Basaran, S, Seydaoglu, G, and Guzel, R. Vitamin D profile of patients with spinal cord injury and post-stroke hemiplegia: all in the same boat. J Back Musculoskelet Rehabil. (2016) 29:205–10. doi: 10.3233/BMR-150615
27.Clark, K, Goldstein, RL, Hart, JE, Teylan, M, Lazzari, AA, Gagnon, DR, et al. Plasma vitamin D, past chest illness, and risk of future chest illness in chronic spinal cord injury (SCI): a longitudinal observational study (vol 10, pg 125, 2020). Spinal Cord. (2020) 58:513–3. doi: 10.1038/s41393-020-0437-3
28.Ehsanian, R, Timmerman, MA, Wright, JM, McKenna, S, Dirlikov, B, and Crew, J. Venous thromboembolism is associated with lack of vitamin D supplementation in patients with spinal cord injury and low vitamin D levels. PM R. (2019) 11:125–34. doi: 10.1016/j.pmrj.2018.09.038
29.Khammeree, T, Vichiansiri, R, Sawanyawisuth, K, and Manimmanakorn, N. Vitamin D abnormalities in Thai patients with spinal cord injuries. Asian Biomed. (2016) 10:595–601. doi: 10.5372/1905-7415.1006.528
30.Nemunaitis, GA, Mejia, M, Nagy, JA, Johnson, T, Chae, J, and Roach, MJ. A descriptive study on vitamin D levels in individuals with spinal cord injury in an acute inpatient rehabilitation setting. PM R. (2010) 2:202-208; quiz 228. doi: 10.1016/j.pmrj.2010.01.010
31.Oleson, CV, Patel, PH, and Wuermser, L-A. Influence of season, ethnicity, and chronicity on vitamin D deficiency in traumatic spinal cord injury. J Spinal Cord Med. (2010) 33:202–13. doi: 10.1080/10790268.2010.11689697
32.Özgirgin, N, Koyuncu, E, Nakipoğlu Yüzer, GF, Taşoğlu, Ö, and Yenigün, D. Is spinal cord injury a risk factor for vitamin D deficiency? Turkiye Fiziksel Tip ve Rehabilitasyon Dergisi. (2016) 62:57–63. doi: 10.5606/tftrd.2016.39260
33.Walia, P, Goldstein, RL, Teylan, M, Lazzari, AA, Hart, JE, Tun, CG, et al. Associations between vitamin D, adiposity, and respiratory symptoms in chronic spinal cord injury. J Spinal Cord Med. (2018) 41:667–75. doi: 10.1080/10790268.2017.1374020
34.Waliullah, S, Kumar, D, Kumar, D, Tewari, PG, Kumar, V, and Srivastava, RN. Prevalence of vitamin D deficiency in a young adult with acute spinal cord injury. Cureus. (2021) 13:e13791. doi: 10.7759/cureus.13791
35.Flueck, JL, Schlaepfer, MW, and Perret, C. Effect of 12-week vitamin D supplementation on 25 OH D status and performance in athletes with a spinal cord injury. Nutrients. (2016) 8:586. doi: 10.3390/nu8100586
36.Hummel, K, Craven, BC, and Giangregorio, L. Serum 25(OH)D, PTH and correlates of suboptimal 25(OH)D levels in persons with chronic spinal cord injury. Spinal Cord. (2012) 50:812–6. doi: 10.1038/sc.2012.67
37.Javidan, AN, Sabour, H, Latifi, S, Vafa, M, Shidfar, F, Khazaeipour, Z, et al. Calcium and vitamin D plasma concentration and nutritional intake status in patients with chronic spinal cord injury: a referral center report. JRMS. (2014) 19:881–4.
38.Mechanick, JI, Pomerantz, F, Flanagan, S, Stein, A, Gordon, WA, and Ragnarsson, KT. Parathyroid hormone suppression in spinal cord injury patients is associated with the degree of neurologic impairment and not the level of injury. Arch Phys Med Rehabil. (1997) 78:692–6. doi: 10.1016/S0003-9993(97)90075-7
39.Pritchett, K, Pritchett, R, Ogan, D, Bishop, P, Broad, E, and LaCroix, M. 25(OH)D status of elite athletes with spinal cord injury relative to lifestyle factors. Nutrients. (2016) 8:374. doi: 10.3390/nu8060374
40.Vaziri, ND, Pandian, MR, Segal, JL, Winer, RL, Eltorai, I, and Brunnemann, S. Vitamin D, parathormone, and calcitonin profiles in persons with long-standing spinal cord injury. Arch Phys Med Rehabil. (1994) 75:766–9. doi: 10.1016/0003-9993(94)90133-3
41.Zebracki, K, Hwang, M, Patt, PL, and Vogel, LC. Autonomic cardiovascular dysfunction and vitamin D deficiency in pediatric spinal cord injury. J Pediatr Rehabil Med. (2013) 6:45–52. doi: 10.3233/PRM-130236
42.Zhou, XJ, Vaziri, ND, Segal, JL, Winer, RL, Eltorai, I, and Brunnemann, SR. Effects of chronic spinal cord injury and pressure ulcer on 25(OH)-vitamin D levels. J Am Paraplegia Soc. (1993) 16:9–13. doi: 10.1080/01952307.1993.11735877
43.Barbonetti, A, Cavallo, F, D'Andrea, S, Muselli, M, Felzani, G, Francavilla, S, et al. Lower vitamin D levels are associated with depression in people with chronic spinal cord injury. Arch Phys Med Rehabil. (2017) 98:940–6. doi: 10.1016/j.apmr.2016.11.006
44.Oleson, CV, Seidel, BJ, and Zhan, T. Association of vitamin D deficiency, secondary hyperparathyroidism, and heterotopic ossification in spinal cord injury. J Rehabil Res Dev. (2013) 50:1177–86. doi: 10.1682/JRRD.2012.11.0206
45.Bauman, WA, Morrison, NG, and Spungen, AM. Vitamin D replacement therapy in persons with spinal cord injury. J Spinal Cord Med. (2005) 28:203–7. doi: 10.1080/10790268.2005.11753813
46.Bauman, WA, Spungen, AM, Morrison, N, Zhang, R-L, and Schwartz, E. Effect of a vitamin D analog on leg bone mineral density in patients with chronic spinal cord injury. J Rehabil Res Dev. (2005) 42:625–34. doi: 10.1682/JRRD.2004.11.0145
47.Beal, C, Gorgey, A, Moore, P, Wong, N, Adler, RA, and Gater, D. Higher dietary intake of vitamin D may influence total cholesterol and carbohydrate profile independent of body composition in men with chronic spinal cord injury. J Spinal Cord Med. (2018) 41:459–70. doi: 10.1080/10790268.2017.1361561
48.Chen, B, Mechanick, JI, Nierman, DM, and Stein, A. Combined calcitriol-pamidronate therapy for bone hyperresorption in spinal cord injury. J Spinal Cord Med. (2001) 24:235–40. doi: 10.1080/10790268.2001.11753580
49.Mailhot, G, Lamarche, J, and Gagnon, DH. Effectiveness of two vitamin D3 repletion protocols on the vitamin D status of adults with a recent spinal cord injury undergoing inpatient rehabilitation: a prospective case series. Spinal Cord Ser Cases. (2018) 4:96. doi: 10.1038/s41394-018-0129-9
50.Pritchett, K, Pritchett, RC, Stark, L, Broad, E, and LaCroix, M. Effect of vitamin D supplementation on 25(OH)D status in elite athletes with spinal cord injury. Int J Sport Nutr Exerc Metab. (2019) 29:18–23. doi: 10.1123/ijsnem.2017-0233
51.Bianco, J, Gueye, Y, Marqueste, T, Alluin, O, Risso, JJ, Garcia, S, et al. Vitamin D3 improves respiratory adjustment to fatigue and H-reflex responses in paraplegic adult rats. Neuroscience. (2011) 188:182–92. doi: 10.1016/j.neuroscience.2011.04.066
52.Gueye, Y, Marqueste, T, Maurel, F, Khrestchatisky, M, Decherchi, P, and Feron, F. Cholecalciferol (vitamin D-3) improves functional recovery when delivered during the acute phase after a spinal cord trauma. J Steroid Biochem Mol Biol. (2015) 154:23–31. doi: 10.1016/j.jsbmb.2015.06.007
53.Gurer, B, Karakoc, A, Bektasoglu, PK, Kertmen, H, Kanat, MA, Arikok, AT, et al. Comparative effects of vitamin D and methylprednisolone against ischemia/reperfusion injury of rabbit spinal cords. Eur J Pharmacol. (2017) 813:50–60. doi: 10.1016/j.ejphar.2017.07.028
54.Khajoueinejad, L, Askarifirouzjaei, H, Namazi, F, Mohammadi, A, Pourfathollah, AA, Rajaian, H, et al. Immunomodulatory effects of Calcitriol in acute spinal cord injury in rats. Int Immunopharmacol. (2019) 74:105726. doi: 10.1016/j.intimp.2019.105726
55.Zhou, KL, Chen, DH, Jin, HM, Wu, K, Wang, XY, Xu, HZ, et al. Effects of calcitriol on experimental spinal cord injury in rats. Spinal Cord. (2016) 54:510–6. doi: 10.1038/sc.2015.217
56.Ross, AC, Manson, JE, Abrams, SA, Aloia, JF, Brannon, PM, Clinton, SK, et al. The 2011 report on dietary reference intakes for calcium and vitamin D from the Institute of Medicine: what clinicians need to know. J Clin Endocrinol Metabol. (2011) 96:53–8. doi: 10.1210/jc.2010-2704
57.Ferrari, S, Manen, D, Bonjour, JP, Slosman, D, and Rizzoli, R. Bone mineral mass and calcium and phosphate metabolism in young men: relationships with vitamin D receptor allelic Polymorphisms1. J Clin Endocrinol Metabol. (1999) 84:2043–8. doi: 10.1210/jcem.84.6.5790
58.Wang, Y, Zhu, J, and DeLuca, HF. Where is the vitamin D receptor? Arch Biochem Biophys. (2012) 523:123–33. doi: 10.1016/j.abb.2012.04.001
59.Barbonetti, A, D'Andrea, S, Martorella, A, Felzani, G, Francavilla, S, and Francavilla, F. Low vitamin D levels are independent predictors of 1-year worsening in physical function in people with chronic spinal cord injury: a longitudinal study. Spinal Cord. (2018) 56:494–501. doi: 10.1038/s41393-017-0058-7
60.Lamarche, J, and Mailhot, G. Vitamin D and spinal cord injury: should we care? Spinal Cord. (2016) 54:1060–75. doi: 10.1038/sc.2016.131
61.Frotzler, A, Krebs, J, Göhring, A, Hartmann, K, Tesini, S, and Lippuner, K. Osteoporosis in the lower extremities in chronic spinal cord injury. Spinal Cord. (2020) 58:441–8. doi: 10.1038/s41393-019-0383-0
62.Koyama, T, Shibakura, M, Ohsawa, M, Kamiyama, R, and Hirosawa, S. Anticoagulant effects of 1alpha,25-dihydroxyvitamin D3 on human myelogenous leukemia cells and monocytes. Blood. (1998) 92:160–7. doi: 10.1182/blood.V92.1.160.413k16_160_167
63.Wu-Wong, JR, Nakane, M, and Ma, J. Vitamin D analogs modulate the expression of plasminogen activator inhibitor-1, thrombospondin-1 and thrombomodulin in human aortic smooth muscle cells. J Vasc Res. (2007) 44:11–8. doi: 10.1159/000097812
64.Moore, M, Goldin, Y, Patel, H, and Greenwald, BD. Low vitamin D level is associated with acute deep venous thrombosis in patients with traumatic brain injury. Brain Sci. (2021) 11:849. doi: 10.3390/brainsci11070849
65.Mpandzou, G, Aït Ben Haddou, E, Regragui, W, Benomar, A, and Yahyaoui, M. Vitamin D deficiency and its role in neurological conditions: a review. Rev Neurol. (2016) 172:109–22. doi: 10.1016/j.neurol.2015.11.005
66.Martineau, AR, Jolliffe, DA, Hooper, RL, Greenberg, L, Aloia, JF, Bergman, P, et al. Vitamin D supplementation to prevent acute respiratory tract infections: systematic review and meta-analysis of individual participant data. BMJ. (2017) 356:i6583. doi: 10.1136/bmj.i6583
67.Monlezun, DJ, Bittner, EA, Christopher, KB, Camargo, CA, and Quraishi, SA. Vitamin D status and acute respiratory infection: cross sectional results from the United States National Health and nutrition examination survey, 2001–2006. Nutrients. (2015) 7:1933–44. doi: 10.3390/nu7031933
68.Farrell, SW, Meyer, KJ, Leonard, D, Shuval, K, Barlow, CE, Pavlovic, A, et al. Physical activity, adiposity, and serum vitamin D levels in healthy women: the Cooper Center longitudinal study. J Womens Health (Larchmt). (2022) 31:957–64. doi: 10.1089/jwh.2021.0402
69.Schönenberg, M, Reimitz, M, Jusyte, A, Maier, D, Badke, A, and Hautzinger, M. Depression, posttraumatic stress, and risk factors following spinal cord injury. Int J Behav Med. (2014) 21:169–76. doi: 10.1007/s12529-012-9284-8
70.Milaneschi, Y, Hoogendijk, W, Lips, P, Heijboer, AC, Schoevers, R, van Hemert, AM, et al. The association between low vitamin D and depressive disorders. Mol Psychiatry. (2014) 19:444–51. doi: 10.1038/mp.2013.36
71.Waldron, JL, Ashby, HL, Cornes, MP, Bechervaise, J, Razavi, C, Thomas, OL, et al. Vitamin D: a negative acute phase reactant. J Clin Pathol. (2013) 66:620–2. doi: 10.1136/jclinpath-2012-201301
72.Shin, JI, Lee, NJ, and Cho, SK. Pediatric cervical spine and spinal cord injury: a National Database Study. Spine. (2016) 41:283–92. doi: 10.1097/BRS.0000000000001176
73.Kreinest, M, Ludes, L, Biglari, B, Küffer, M, Türk, A, Grützner, PA, et al. Influence of previous comorbidities and common complications on motor function after early surgical treatment of patients with traumatic spinal cord injury. J Neurotrauma. (2016) 33:2175–80. doi: 10.1089/neu.2016.4416
74.Fakhoury, HMA, Kvietys, PR, AlKattan, W, Anouti, FA, Elahi, MA, Karras, SN, et al. Vitamin D and intestinal homeostasis: barrier, microbiota, and immune modulation. J Steroid Biochem Mol Biol. (2020) 200:105663. doi: 10.1016/j.jsbmb.2020.105663
75.Bauman, WA, Emmons, RR, Cirnigliaro, CM, Kirshblum, SC, and Spungen, AM. An effective oral vitamin D replacement therapy in persons with spinal cord injury. J Spinal Cord Med. (2011) 34:455–60. doi: 10.1179/2045772311Y.0000000032
76.Evans, MA, Kim, HA, Ling, YH, Uong, S, Vinh, A, De Silva, TM, et al. Vitamin D(3) supplementation reduces subsequent brain injury and inflammation associated with ischemic stroke. NeuroMolecular Med. (2018) 20:147–59. doi: 10.1007/s12017-018-8484-z
77.Cui, C, Wang, C, Jin, F, Yang, M, Kong, L, Han, W, et al. Calcitriol confers neuroprotective effects in traumatic brain injury by activating Nrf2 signaling through an autophagy-mediated mechanism. Mol Med. (2021) 27:118. doi: 10.1186/s10020-021-00377-1
78.Berridge, MJ. Vitamin D cell signalling in health and disease. Biochem Biophys Res Commun. (2015) 460:53–71. doi: 10.1016/j.bbrc.2015.01.008
79.Brewer, LD, Thibault, V, Chen, KC, Langub, MC, Landfield, PW, and Porter, NM. Vitamin D hormone confers neuroprotection in parallel with downregulation of L-type calcium channel expression in hippocampal neurons. J Neurosci. (2001) 21:98–108. doi: 10.1523/JNEUROSCI.21-01-00098.2001
80.Koshkina, A, Dudnichenko, T, Baranenko, D, Fedotova, J, and Drago, F. Effects of vitamin D(3) in long-term Ovariectomized rats subjected to chronic unpredictable mild stress: BDNF, NT-3, and NT-4 implications. Nutrients. (2019) 11:1726. doi: 10.3390/nu11081726
81.Morello, M, Landel, V, Lacassagne, E, Baranger, K, Annweiler, C, Féron, F, et al. Vitamin D improves neurogenesis and cognition in a mouse model of Alzheimer's disease. Mol Neurobiol. (2018) 55:6463–79. doi: 10.1007/s12035-017-0839-1
82.Cui, X, Gooch, H, Petty, A, McGrath, JJ, and Eyles, D. Vitamin D and the brain: genomic and non-genomic actions. Mol Cell Endocrinol. (2017) 453:131–43. doi: 10.1016/j.mce.2017.05.035
83.Yeh, WZ, Gresle, M, Jokubaitis, V, Stankovich, J, van der Walt, A, and Butzkueven, H. Immunoregulatory effects and therapeutic potential of vitamin D in multiple sclerosis. Br J Pharmacol. (2020) 177:4113–33. doi: 10.1111/bph.15201
84.Wang, L, Gunduz, MA, Semeano, AT, Yılmaz, EC, Alanazi, FAH, Imir, OB, et al. Coexistence of chronic hyperalgesia and multilevel neuroinflammatory responses after experimental SCI: a systematic approach to profiling neuropathic pain. J Neuroinflammation. (2022) 19:264. doi: 10.1186/s12974-022-02628-2
85.Gomez-Pinedo, U, Cuevas, JA, Benito-Martín, MS, Moreno-Jiménez, L, Esteban-Garcia, N, Torre-Fuentes, L, et al. Vitamin D increases remyelination by promoting oligodendrocyte lineage differentiation. Brain Behav. (2020) 10:e01498. doi: 10.1002/brb3.1498
86.Shirazi, HA, Rasouli, J, Ciric, B, Rostami, A, and Zhang, GX. 1,25-Dihydroxyvitamin D3 enhances neural stem cell proliferation and oligodendrocyte differentiation. Exp Mol Pathol. (2015) 98:240–5. doi: 10.1016/j.yexmp.2015.02.004
87.Teng, YD, Wang, L, Zeng, X, Wu, L, Toktas, Z, Kabatas, S, et al. Updates on human neural stem cells: from generation, maintenance, and differentiation to applications in spinal cord injury research. Results Probl Cell Differ. (2018) 66:233–48. doi: 10.1007/978-3-319-93485-3_10
88.Thakor, DK, Wang, L, Benedict, D, Kabatas, S, Zafonte, RD, and Teng, YD. Establishing an Organotypic system for investigating multimodal neural repair effects of human Mesenchymal stromal stem cells. Curr Protoc Stem Cell Biol. (2018) 47:e58. doi: 10.1002/cpsc.58
89.Wang, L, Gu, S, Gan, J, Tian, Y, Zhang, F, Zhao, H, et al. Neural stem cells overexpressing nerve growth factor improve functional recovery in rats following spinal cord injury via modulating microenvironment and enhancing endogenous neurogenesis. Front Cell Neurosci. (2021) 15:773375. doi: 10.3389/fncel.2021.773375
90.Yang, Y, Fan, Y, Zhang, H, Zhang, Q, Zhao, Y, Xiao, Z, et al. Small molecules combined with collagen hydrogel direct neurogenesis and migration of neural stem cells after spinal cord injury. Biomaterials. (2021) 269:120479. doi: 10.1016/j.biomaterials.2020.120479
Keywords: spinal cord injury, vitamin D, deficiency, neuroprotection, progonosis, insufficiency
Citation: Wang L, Gan J, Wu J, Zhou Y and Lei D (2023) Impact of vitamin D on the prognosis after spinal cord injury: A systematic review. Front. Nutr. 10:920998. doi: 10.3389/fnut.2023.920998
Edited by:
Sakineh Shab-Bidar, Tehran University of Medical Sciences, IranReviewed by:
Anneke Hertig-Godeschalk, Swiss Paraplegic Center, SwitzerlandAnke Scheel-Sailer, Swiss Paraplegic Center, Switzerland
Copyright © 2023 Wang, Gan, Wu, Zhou and Lei. This is an open-access article distributed under the terms of the Creative Commons Attribution License (CC BY). The use, distribution or reproduction in other forums is permitted, provided the original author(s) and the copyright owner(s) are credited and that the original publication in this journal is cited, in accordance with accepted academic practice. No use, distribution or reproduction is permitted which does not comply with these terms.
*Correspondence: Deqiang Lei, ✉ bGRxdHpAMTYzLmNvbQ==; ✉ MjAwOVhIMDgzOEBodXN0LmVkdS5jbg==; Lei Wang, ✉ bGVpd2FuZ19uc0BodXN0LmVkdS5jbg==; ✉ bGVpd2FuZ19uc0Bob3RtYWlsLmNvbQ==