- 1College of Biological and Food Engineering, Jilin Engineering Normal University, Changchun, China
- 2Department of Gastrointestinal Colorectal and Anal Surgery, The China-Japan Union Hospital of Jilin University, Changchun, China
- 3Department of Burns Surgery, The First Hospital of Jilin University, Changchun, China
Quinoa is a highly nutritious whole-grain crop with unique values as both a food and medicinal supplement. At present, the roles played by the intestinal microflora in human health are gaining considerable attention from the research community, and studies to date have shown that the occurrence of a range of diseases may be associated with an imbalance of the intestinal flora. The bioactive compounds of quinoa affect the production of SCFAs and the adjustment of intestinal pH. In this article, we review the mechanisms underlying the effects of different quinoa constituents on the intestinal flora, the effects of these constituents on the intestinal flora of different hosts, and progress in research on the therapeutic properties of quinoa constituents, to provide a better understanding of quinoa in terms its dual medicinal and nutritional properties. We hope this review will provide a useful reference for approaches that seek to enhance the composition and activities of the intestinal flora.
1 Quinoa
Chenopodium quinoa, a plant originating from the Andes Mountains of South America, can be divided into white, red, and black color varieties. It is a hardy plant noted for its broad environmental tolerance to low temperatures, drought, and saline–alkaline conditions, and has become a traditional food crop on account of its excellent cold tolerance and ability to grow in high-altitude regions. Today, C. quinoa is mainly cultivated in South America and Asia, and in China, it is grown primarily in Shanxi and Qinghai provinces, and the northwest of the country. It is noted as a particularly nutrient-rich grain crop and a valuable source of proteins, fat, minerals, vitamins, fiber, and other nutrients, the levels of which tend to be higher than those in more common cultivated grain crops (1, 2). Quinoa is considered a whole-grain product containing a rich variety of bioactive substances, including phenols, saponins, and glucans, and can play potentially essential roles in the treatment of different diseases (3–5), based on the anti-oxidative, anti-cancer, anti-radiation, antibacterial and cholesterol metabolism-regulatory effects of its constituent (6). Figure 1 shows the nutrient contents of quinoa and Figure 2 presents a profile of the associated bioactive substances, which have been widely described in previous studies (6–13).
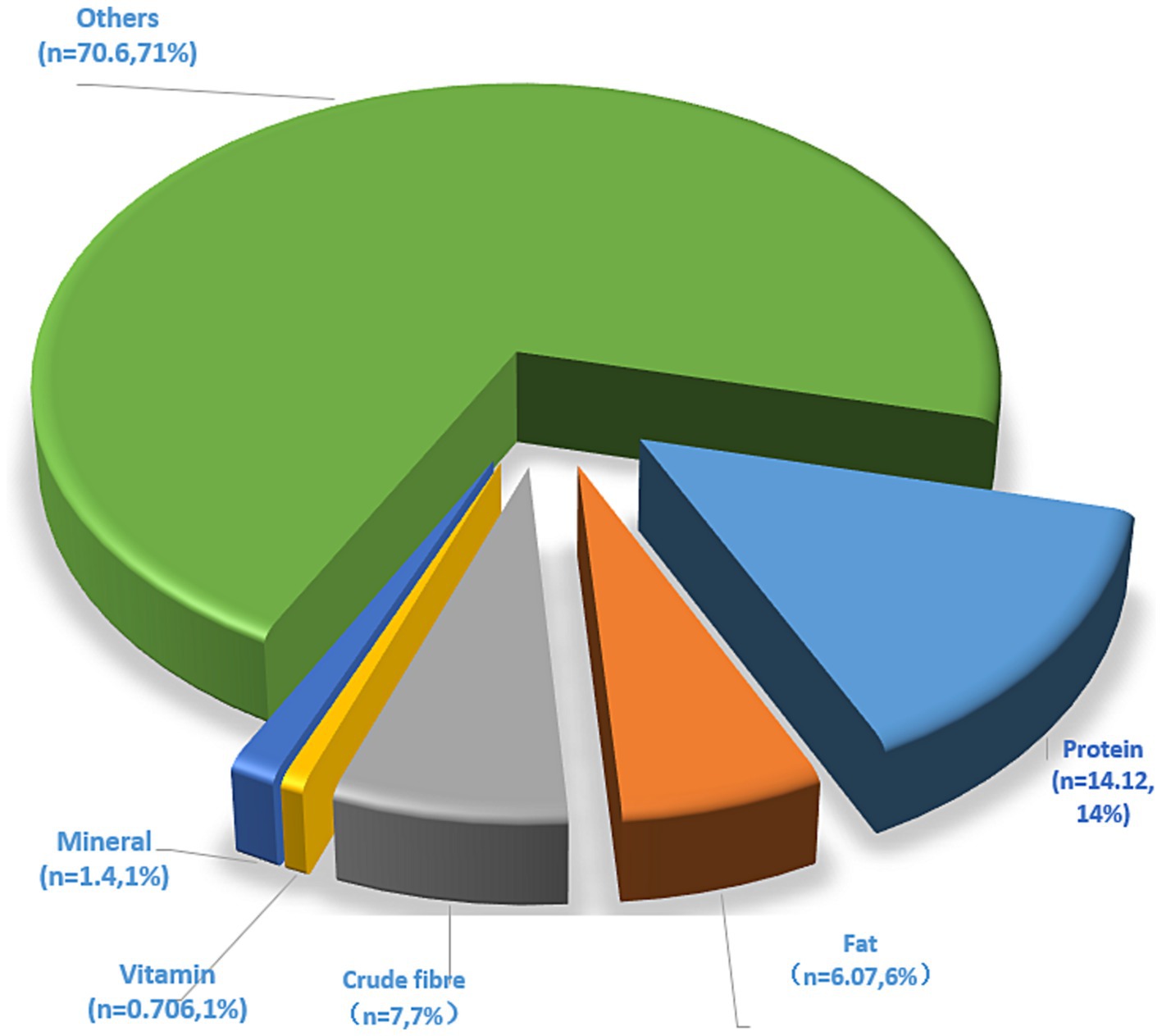
Figure 1. Nutrients of quinoa. Data were obtained from the US Department of Agriculture database. In the figure, n represents the proportion of this substance.
Quinoa is considered an example of a plant with dual nutritional and medicinal properties, in that it can either be consumed as a food or applied for therapeutic purposes, depending on the context (Figure 3). In addition to being consumed as a whole grain, quinoa can be processed to produce a diverse range of food products, including yogurt, bread, noodles, biscuits, ice cream, porridge, and meal replacement powders. However, despite its medicinal value, such as in the supplementation of amino acids, there has to date been comparatively little in-depth research to assess the potential medicinal uses of quinoa and its therapeutic effects (13–15).
In the past few years, there has been an increase in research assessing the application of quinoa in the treatment of liver cancer, obesity, diabetes, colon cancer, and other diseases (Figure 4). In this context, recent discoveries regarding the mechanisms underlying gut–brain–liver andintestinal flora –brain interactions have led to an increasing recognition of the importance of intestinal flora as a mediator in the body’s resistance and response to disease. It is accordingly speculated that the beneficial effects of consuming quinoa may be associated with an enhancement of the health and activities of gut microbiota (16–29).
1.1 Intestinal flora
Intestinal flora is a key component of the microenvironments of the small and large intestines. It is a dynamic and complex microbial system that plays essential roles in host metabolism, the occurrence of disease, and immune regulation (30). The principal bacterial taxa comprising intestinal flora include Bacteroidetes, Actinobacteria (mainly Bifidobacterium), Proteobacteria (mainly Enterobacter), and Firmicutes (mainly Lactobacillus, Enterococcus, and Clostridium). The outer mucosal layer of the colon is primarily populated by bacteria in the phyla Firmicutes, Bacteroidetes, Actinobacteria, Proteobacteria, Fusobacteria, and Verrucomicrobia. It is composed of six bacterial genera, accounting for more than 90% of the total intestinal flora, with genera of Verrucomicrobia accounting for 3–5% (31). Different dominant strains inhabit different intestinal sites, wherein interspecific competition and inhibition contribute to maintaining the equilibrium of the intestinal microenvironment.
Based on their different effects on the host, the bacterial taxa comprising the intestinal flora can be classified as pathogenic, beneficial, and neutral. The pathogenic bacteria, which can cause foodborne intestinal diseases, food poisoning, and other harmful diseases, include Clostridium perfringens and species of Proteus and Salmonella, which account for 10% of the total intestinal flora. Host-beneficial bacteria, accounting for approximately 20% of the total intestinal flora, include Clostridium butyricum, and species of Akkermansia, Lactobacillus, and Bifidobacterium, which play particularly important roles in the intestine that anti-inflammatory effect, mutual inhibition with harmful bacteria, etc. Neutral bacteria, also referred to as opportunistic pathogens, account for approximately 70% of the total number of intestinal bacteria, among which are Escherichia coli, Lactobacillus, Streptococcus, and Veillonella. Under normal conditions, these bacteria are generally harmless, although they can become pathogenic in response to the interference of adverse external factors (31–33). Increasingly, it is becoming evident that these gut bacteria play key roles in regulating multiple body processes, about which comparatively little is known at present, thereby highlighting the necessity of further in-depth research.
In recent years, studies have shown that the occurrence of diseases is closely related to the reduction of beneficial bacteria. Based on this research, people have made great achievements in the treatment of intestinal flora disorders by supplementing probiotics or supplementing synbiotics. Since grains are rich in components that promote the growth of beneficial bacteria (34, 35), we focused our attention on the beneficial effects of grains on intestinal flora.
1.2 Further research on intestinal flora can provide a reference for the occurrence of diseases related to intestinal flora
One of the primary contributions of intestinal flora is the production of beneficial metabolites, such as short-chain fatty acids (SCFAs), which mainly include acetic, propionic, and butyric acids. SCFAs can bind to the G-protein-coupled receptors (GPCR) GPR41 and GPR43, thereby inducing the secretion of glucagon-like peptide-1 (GLP-1), peptide YY (PYY), and leptin, which enhance the body’s energy metabolism (36). SCFAs can, to a certain extent, influence glucose and lipid metabolism by inhibiting the synthesis of fat, participating in immune regulatory processes, promoting intestinal digestion and absorption, reducing intestinal pH, and playing an important role in maintaining the balance of the intestinal flora (37). An unfavorable imbalance among intestinal flora can influence the nervous and immune systems via the brain–intestine–kidney and brain–intestine–bone marrow axes. Among the disorders that have been established to be associated with imbalanced intestinal flora is inflammatory bowel disease (IBD), in which patients are characterized by changes in the proportions, diversity, and composition of gut flora, which is reflected in an altered balance among pathogenic, conditional pathogenic, and beneficial bacteria, with increases in pathogenic types and reductions in beneficial bacteria (38). Generally, such imbalances between beneficial and pathogenic bacteria contribute to the development of diseases and disorders, and in this regard, some studies have found that changes in the intestinal microenvironment are reflected in increases or reductions in microbial metabolites, which in turn will influence the normal host metabolic activities and may be accompanied by metabolic disease (36). These findings accordingly highlight the close relationship between intestinal flora and human health, and thus, further research on intestinal flora could provide a reference for the occurrence of diseases associated with the activities of these microorganisms.
A number of cereal crop plants characterized by coarse grains have been established to influence the regulation of intestinal flora. For example, a study on polysaccharides derived from the coarse-grained Tartary buckwheat based in vitro-simulated fermentation found that these polysaccharides have a regulatory effect on intestinal flora and SCFA metabolites (39). Among these SCFAs, the contents of acetic, propionic, and butyric acids were observed to have increased during in vitro fermentation, which, compared with the control, was accompanied by corresponding increases in the abundance of probiotic flora, thus contributing to a regulation of the intestinal microenvironment. In a further study, Zhao et al. demonstrated that consumption of adzuki beans can alleviate obesity caused by a high-fat diet, which was found to be closely associated with the activities of intestinal flora (40). Compared with mice in a high-fat diet group, those consuming an adzuki bean-supplemented diet were found to have a significantly enriched bacterial flora in terms of abundance, although there were no obvious changes in bacterial diversity. The intestinal flora of mice fed the adzuki bean supplement was found to contain a greater abundance of probiotic strains, which partially compensated for the detrimental effects of a high-fat diet on the intestines of mice, and also contributed to a reduction in the biosynthesis of lipopolysaccharides. Accordingly, these findings indicate the positive effects of adzuki beans in regulating intestinal flora. Similarly, examination of the effects of different proportions of buckwheat in regulating the abundance of intestinal flora has indicated that high doses of dietary buckwheat can promote increases in the abundance of intestinal flora, reduce the intestinal absorption of cholesterol, and enhance the intestinal excretion of cholesterol (41). These findings accordingly provide important clues regarding the potential mechanisms whereby coarse-grain products can contribute to improving the intestinal microenvironment.
Based on the research conducted to date, it is speculated that the natural active constituents of quinoa can influence disease development by regulating intestinal flora. In this review, we accordingly focus on the regulatory effects of quinoa extracts and their active constituents on the composition and activity of intestinal flora.
2 The regulatory effects of quinoa bioactive components on intestinal flora
2.1 Saponins
The saponins in quinoa are characterized by their low bioavailability, poor absorption, and prolonged intestinal residence. These characteristics indicate the potential availability for metabolism by intestinal microorganisms and that they can serve as a source of secondary glycosides or aglycosides (42). Studies have shown that (43) Under the action of intestinal flora, quinoa saponin can be effectively hydrolyzed into sapogenin by the host, the release of sapogenin is related to the initial microbial composition of intestinal microflora, and the initial intestinal microflora interferes with the later conversion process of sapogenin of quinoa saponin through some mechanism. In addition, these saponins have been shown to play a beneficial role in regulating the intestinal flora in rats (44). A comparison of rats administered high, medium, and low doses of quinoa saponins revealed significant differences between the experimental and control groups with respect to the diversity of intestinal flora. The findings of metabolomic analyses revealed that these saponins influence multiple processes, including the metabolism of vitamin B6 and tryptophan and the ammonia cycle, thus regulating host metabolism and modifying the composition of the intestinal flora in rats. The findings of this study also provided evidence to indicate that in contrast to low doses, medium and high doses of quinoa saponins may have potentially toxic effects on the kidneys. These observations accordingly indicate that it would probably be safe to consume low amounts of quinoa saponins in the diet or quinoa-based food products.
Although an imbalance in the intestinal flora has been established to influence host metabolism (43), with metabolites having both beneficial and detrimental effects on the intestinal flora, the respective mechanisms of action remain unclear. Within the intestines, quinoa saponins can be effectively microbially hydrolyzed to yield sapogenin, the release of which has been shown to influence the initial microbial composition of intestinal microflora, which in turn interferes with the subsequent conversion of sapogenin. In this regard, Li et al. (42) have reported that total quinoa saponins have a bidirectional regulatory effect on intestinal microorganisms, with the ability to selectively promote the growth of beneficial bacteria. For example, treatment with quinoa saponins has been found to promote a significant increase in the relative abundance of Lactobacillus, has a beneficial effect on butyrate-producing bacteria, promotes health by enhancing the relative abundance of anti-inflammatory intestinal microbiota, inhibits the excessive growth of harmful bacteria, and reduces the production of lipopolysaccharides (LPS). Moreover, long-term supplementation with quinoa saponins is associated with anti-obesity effects by promoting a significant reduction in fat accumulation in obese rats, enhancing energy metabolism, and modifying the composition of intestinal flora. These findings also indicate a potential link between the composition of the intestinal flora and enhanced glucose tolerance and reductions in the levels of IL-6 and serum LPS levels. On the basis of these findings, it would thus appear that quinoa saponins have positive regulatory effects on intestinal flora.
Studies on rats, examining the effects of saponins derived from different varieties of quinoa have shown that quinoquinosides have membranolytic activity on small intestinal mucosal cells, with the effect being most pronounced with the quinoquinosides in bitter grains, and is associated with marked food aversion and impaired growth responses in rats. However, there is no clear evidence to indicate any deleterious effects on animal health (45). In a further study, Chen et al. extracted saponins from different colored varieties of quinoa and compared their compositions, digestion, and fermentation characteristics (46). They accordingly found that the quinoquinosides of black and white varieties had a higher saponin content than those from a red variety. The quinoquinosides of black quinoa were found to inhibit the growth of harmful bacteria (e.g., Shigella spp.) and promotes the growth of probiotic strains (Lactobacillus and Bifidobacterium spp.). Conversely, intestinal flora also releases sapogenin from quinoquinoside saponins. In a study assessing the safety of quinoa saponins and their effects on intestinal flora, in which rats were orally administered quinoa saponins for 90 days, the authors established that the maximum non-toxic dose of these saponins was in the range between 5 and 50 mg/kg (47). This result may be due to the consistent and inseparable relationship between the pathological characteristics of chronic kidney injury shown by the high-dose group of female rats after the experiment. More experiments are needed to verify the dose relationship between quinoa saponin and the body. Analysis of the intestinal flora of the treated rats revealed that the orally administered saponins promoted an increase in the number of intestinal flora species in female rats with relatively poor intestinal flora. Contrastingly, in male rats with relatively abundantintestinal flora, these saponins were found to alter the composition of the intestinal flora and also promoted an increase in the abundance of beneficial bacteria, whereas no corresponding increase was observed regarding the abundance of harmful bacteria. These findings thus provide further evidence to indicate that quinoa saponins have a positive effect with respect to improving intestinal flora, although the underlying mechanisms remain to be determined.
Ruoyu (44) and Zhang et al. (47) reported that quinoa saponin has no adverse effect on the host at a small dose of less than 5 mg/kg, on the contrary, at higher doses, such as 5 mg/kg-500 mg/kg, there is an adverse effect on the host. Under the influence of a higher dose of quinoa saponin, the host will cause kidney damage and chronic inflammation in the gastrointestinal tract. At present, more details of the safe dosage of quinoa saponin need to be further explored. In contrast, further exploration of the safe dosage range of quinoa saponin is of greater significance for the application of quinoa saponin. In addition to increasing the utilization of quinoa saponin, the related mechanism of saponin improving intestinal flora can also be further revealed.
2.2 Polyphenolic compounds
Among the polyphenolic constituents of quinoa, esculin has been identified as a polyphenol with medicinal properties. In a study using red junglefowl, Agarwal et al. examined the effects of quercetin 3-glucoside, 5% inulin, 5% quinoa cellulose, and other treatments on the microbiota populating the duodenum and cecum of these birds (48). The findings of duodenal 16rDNA analysis revealed that compared with the control group, treatment with 1% quinoa quercetin promoted increases in the numbers of in the group of probiotic microorganisms, reduced the numbers of potentially pathogenic E. coli, and increased the relative abundance of the dominant bacterial phylum Firmicutes in the intestinal flora. In addition, the combination of 1% quinoa quercetin and 5% quinoa cellulose resulted in an increase in goblet cells, which is good for the intestinal flora. The more goblet cells, the more mucin they produce, which strengthens the first line of defense of the intestinal flora (49). Another hypothesis is that acidic goblet cells may lower luminal pH, thereby influencing resident bacteria and directing the intestinal flora in a positive direction. These findings thus indicated that 1% quinoa quercetin has a prebiotic effect on the intestinal flora of red junglefowl, which could contribute to enhancing the composition of intestinal flora. Given the presumed pivotal role of intestinal flora in metabolic regulation, several studies have examined its effects on the metabolism of glucose and lipids, and in this regard, it has been established that the biological activity of quinoa polyphenols can also be manifested via their hypoglycemic effects mediated through inhibition of gastrointestinal digestive enzymes. In this process, quinoa polyphenols have been demonstrated to hinder the degradation of polysaccharides and retard the absorption of carbohydrates in the intestine by inhibiting α-amylase and α-glucosidase, which are key enzymes in the digestion and absorption of gastrointestinal sugars. Moreover, these compounds have also been found to contribute to lowering blood glucose levels (50). Collectively, these findings provide evidence indicating that quinoa polyphenols can enhance intestinal flora, which may be associated with the production of prebiotics and glucose metabolism-related mechanisms. Zhang et al. (51) reported that quinoa polyphenols could inhibit α-glucosidase activity in a dose-dependent manner through free polyphenols (FPE) and bound polyphenols (BPE). FPE and BRE inhibit α-glucosidase activity by binding to the enzyme site in a non-competitive mode and an un-competitive mod, thereby inhibiting the elevation of blood glucose level according to the previous reports on quinoa polyphenols, we speculate that the mechanism of quinoa polyphenols involved in intestinal flora may be that quinoa polyphenols inhibit enzymes involved in the regulation of the digestive tract, thereby affecting the abundance of intestinal flora and improving the microenvironment of intestinal flora. This also gives us an important hint that quinoa polyphenols have a great potential impact on the improvement of the body and provides strong support for the development and utilization of quinoa. The more detailed mechanism remains to be further explored.
2.3 Polysaccharides
The findings of previous studies have indicated that the extraction yield of quinoa polysaccharides differs among different cultivars, among which, the yield obtained from cultivar ‘NSL92331’ has been reported to be as high as high as 6.88% (52). Quinoa polysaccharides are considered prebiotics, and in this regard, simulated in vitro fermentation studies have revealed increases from 3.33 to 10.88% in the proportion of Bifidobacteria in a quinoa polysaccharide-treated group. Similarly, whereas Actinobacteria accounted for 5.28% of the intestinal flora in the control group, the proportion had increased to 16.98% in the quinoa polysaccharide group. Quinoa polysaccharides have been established to promote the growth of beneficial bacteria after fermentation, for example, Bifidobacterium and Collinsella, thereby indicating that these polysaccharides can be used as a crude source of fermented carbohydrates and have a significant prebiotic promotion effect on Bifidobacterium and other probiotic bacteria (53).
Quinoa polysaccharides can also contribute to ameliorating hyperlipidemia induced by consumption of a high-fat diet (HFD), the underlying process of which is regulated by intestinal flora. In a study in which Cao et al. examined the effects of quinoa polysaccharide supplementation in rats fed an HFD, the rats were orally administered a quinoa polysaccharide preparation (54). It was accordingly found that low-dose quinoa polysaccharide supplementation had regulatory effects on the bacterial community at the phylum, class, order, family, and genus levels. At the phylum level, the populations of Firmicutes, Bacteroidetes, and Proteobacteria were found to account for more than 90% of the total gut bacteria. However, this supplementation was observed to reduce the Firmicutes and increase those of Bacteroidetes, thereby leading to a reversal in the Firmicutes to Bacteroidetes ratio in the HFD group rats. Furthermore, in response to the low-dose polysaccharide treatment, there was an increase in the proportion of Proteobacteria, to levels similar to those observed in the normal control group. These findings thus provide further evidence supporting the role of quinoa polysaccharides as prebiotics in regulating intestinal flora. These reports indicate that quinoa polysaccharide has a positive effect on intestinal flora, and the related regulatory mechanism needs to be further explored. From the above reports, we also know that the imbalance of intestinal flora is not a simple process, but more a multi-sided regulation, and quinoa polysaccharide shows changes in intestinal flora, which also gives us favorable support for the development of quinoa.
Quinoa dietary fiber has been established to have a positive effect with respect to the regulation of intestinal flora, with SCFAs being notable beneficial products of the microbial fermentation of dietary fiber. The effects of quinoa bran soluble dietary fiber (QBSDF) on intestinal inflammation and homeostasis have been studied in mice with ulcerative colitis induced by sodium dextran sulfate (DSS) (55). Functional analyses of the intestinal flora in these mice revealed that QBSDF-induced improvements in the grouping, and structure of the intestinal flora community may include functional changes among these microbes. QBSDF was found to enhance the production of SCFAs (as determined from an analysis of fecal samples), attenuated DSS-induced dysbiosis of intestinal flora, and had positive effects on the maintenance of intestinal integrity. This fiber was also found to contribute to a significant enhancement of gut barrier function by promoting increases in the expression of tight junction proteins at both the mRNA and protein levels. Furthermore, the prebiotic properties of quinoa soluble cellulose have been confirmed based on an examination of the effects of intra-amniotic administration of 5% quinoa fiber to viable embryos mediated via its influence on the cecal bacterial population and intestinal morphology (45). Previous reports mentioned that there is another possible mechanism of quinoa polysaccharide related to SCFAs, which involves that quinoa polysaccharide may be related to the production of SCFAs during the fermentation process, which also involves the change of pH, thereby improving the living environment of beneficial bacteria and guiding the intestinal flora in a positive direction (Table 1).
2.4 Bioactive peptides
Bioactive peptides are peptide compounds that have positive effects on biological activities, and whereas microorganisms can metabolize protein peptides, bioactive peptides have in turn, been established to have regulatory effects on the structure of intestinal flora (59).
Quinoa proteins are an important source of bioactive peptides. In an in vivo study of quinoa proteins in spontaneously hypertensive rats (SHR), analysis of the gastrointestinal contents revealed the presence of numerous promising bioactive peptide precursors released from quinoa proteins. Moreover, examination of fecal microbes in SHR treated with quinoa proteins revealed that these microbes have more common characteristics regarding genus composition than those in non-hypertensive rats (59). Quinoa proteins were found to promote a significant reduction in the blood pressure of SHR, an increase in bacterial alpha diversity (the number, evenness, and abundance of species), and a shift in the structure of intestinal flora to one closer to that of non-hypertensive rats. These findings accordingly indicate that quinoa proteins may serve as a potential therapeutic candidate for reducing blood pressure by ameliorating the intestinal flora dysbiosis associated with hypertension. In this regard, quinoa proteins have been identified as a promising natural source of angiotensin-converting enzyme (ACE) inhibitory peptides and can reduce blood pressure in SHR, in which the fecal microbiota undergo significant alterations. A further study on bioactive peptides derived from quinoa proteins has similarly confirmed that as precursors of bioactive peptides, quinoa proteins have regulatory effects on intestinal flora. The effects of quinoa proteins and the associated hydrolysate have also been investigated in an azoxymethane/dextran sulfate sodium (AOM/DSS)-induced mouse model of colorectal cancer (CRC) (60). Analysis of the intestinal flora and potential mechanisms associated with SCFAs showed that quinoa proteins or protein hydrolysates can alleviate the clinical symptoms of CRC and increase the contents of SCFAs in colonic tissues. In addition, these proteins, or the hydrolysate thereof, were found to partially alleviate an imbalance in the intestinal microbiota of CRC mice, which could be attributed to the fact that quinoa proteins can contribute to reducing the abundance of pathogenic bacteria and promote increases in the relative abundance of probiotic strains. Furthermore, PICRUSt analysis revealed that the functional characteristics of the intestinal flora in the quinoa protein-treated group were similar to those in the control group. Collectively, the findings of this study revealed that quinoa proteins and their hydrolysate can contribute to ameliorating AOM/DSD-induced CRC in mice, by altering intestinal flora and enhancing the production of beneficial SCFAs.
The findings of a further study conducted by Fotschki et al. have revealed that flour containing quinoa protein can significantly enhance cecal microbial activity, the activities of α-glucosidase, β-glucosidase, and α-galactosidase, and the production of SCFAs in rats, while promoting a reduction in the pH of digesters, thereby indicating the favorable effects of these proteins on growth parameters and metabolism of intestinal flora (61). Furthermore, in a study evaluating the effects of quinoa seeds supplemented with phytase and protease alone or in combination on microbial activity and intestinal flora, it was found that the addition of phytase and protease to the basal diet had beneficial effects on the growth performance, intestinal flora ecology, and gut morphology of broilers (62). The addition of protease supplements and phytase to a quinoa seed-based diet promoted increases in the viable cell counts of Lactobacillus and reduced those of E. coli, which could be associated with the effects of these enzymes on protein digestibility, thereby altering amino acid bioavailability and influencing microbial composition, as well the antimicrobial properties of protease supplements.
The diseases mentioned in these reports include spontaneous hypertension and colorectal cancer, both of which show a close relationship with intestinal flora in these reports. However, it is extremely strange that in a report by Zhang et al. (51), it is mentioned that α-glucosidase activity was inhibited. However, Fotschki et al. (61) report increased the activities of α-glucosidase, which is obviously in conflict. Nevertheless, since the diseases targeted by these two reports and the varieties of quinoa tested in these two reports are different, this may be explained. This also gives us some hints that, perhaps because of its various bioactive functions, quinoa is the good choice for a versatile grain in the face of so many different diseases (Table 2).
Figure 5 shows the molecular structures of the chemical components of quinoa (5, 6, 63, 64, 66–70).
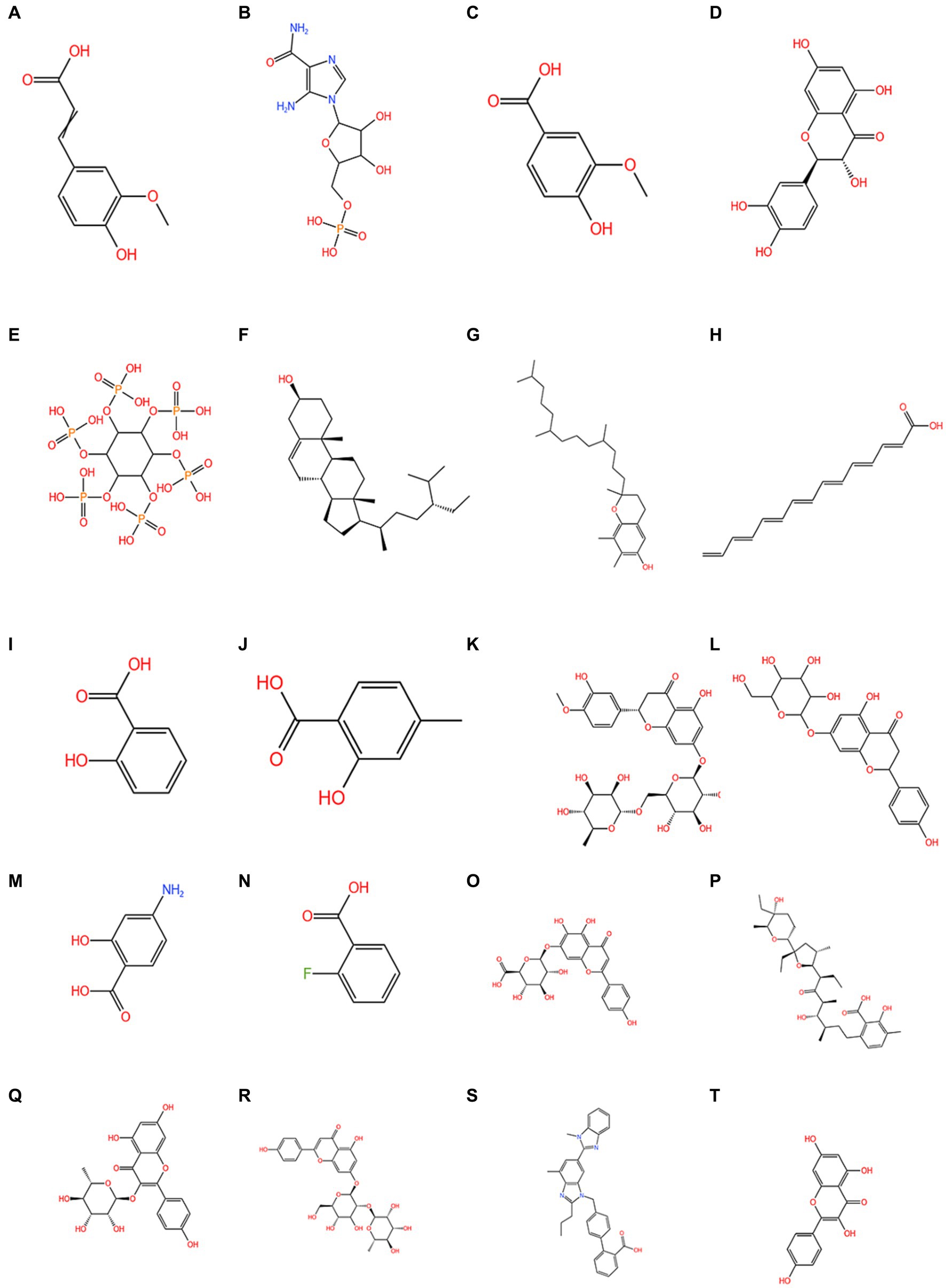
Figure 5. The chemical composition of quinoa. (A) 3-(4-hydroxy-3-methoxyphenyl)-2-propenoic acid; (B) 1h-Imidazole-4-carboxamide; (C) vanillic acid; (D) quercetin; (E) phytic acid; (F) phytosterol; (G) tocopherols; (H) C14-18 and C16-18-unsatd; (I) salicylic acid; (J) 4-methylsalicylic acid; (K) hesperetin 7-rhamnoglucoside; (L) naringenin-7-O-glucoside; (M) 4-aminosalicylic acid; (N) 2-fluorobenzoic acid; (O) scutellarin; (P) lasalocid; (Q) afzelin; (R) apigenin-7-O-neohesperidoside; (S) telmisartan; (T) kaempferol.
2.5 Improvements in the intestinal flora promoted by whole quinoa extract
Studies that have evaluated the prebiotics in quinoa and amaranth have shown that these can promote the production of SCFAs, lactate, and formate during fermentation, which can serve as suitable substrates for intestinal flora metabolism (71). The high acetate concentrations obtained from the fermentation of both these substrates may be associated with increases in Bifidobacterium, Proteus mirabilis, or Bacteroides, thereby indicating potential prebiotic effects on the intestinal flora. Bianchi et al. have also demonstrated that a symbiotic beverage based on a soybean and quinoa water extract (30% quinoa and 70% soybean) had positive effects on the colon, promoting increases in the populations of beneficial Lactobacillus and Bifidobacterium and reducing those of potentially harmful genera, such as Bacteroides and Clostridium (72). Administration of this beverage also promoted a significant reduction in ammonia ion production and enhanced the diversity and richness of Lactobacillus spp. without influencing their activity. These findings thus indicate that quinoa and soy are good candidate plants for delivering probiotics to the intestinal flora, thereby enhancing the composition of the intestinal flora and hence improving human health (72). The findings of these and similar studies have thus confirmed that whole quinoa extracts can improve intestinal flora by yielding fermentation products beneficial to intestinal microbial metabolism, as well as through their roles as prebiotics. All the above reports show that quinoa has a certain role in promoting the health of intestinal flora. What can be observed now is that after the animal quinoa experiment, the beneficial bacteria increases, and the harmful bacteria decreases. All the reports seem to prove this. In addition, there is evidence in the signal transduction pathway, which also gives us a great hint. Perhaps from the molecular mechanism, we can further discover the improvement of quinoa on intestinal flora, and then expand the utilization value of quinoa.
2.6 The effects of quinoa on the intestinal flora in different hosts
In addition to studies on rodents and poultry, researchers have also assessed the intestinal flora-associated effects of quinoa in a range of other hosts. For example, Traughber et al. evaluated the effects of five ancient grains (including rice and quinoa) as carbohydrate sources on blood glucose and insulin levels in dogs (73). The intestinal flora of animals in the quinoa group were found to be characterized by an increase in the relative abundance of Bacteroidetes, and these authors established that diets comprising up to 40% of these grains, would have beneficial effects on overall host health, without adversely influencing nutrient digestibility, and had no significant effects on the levels of blood glucose or insulin in healthy dogs. These findings imply that quinoa-based diets might benefit dogs with obesity, insulin resistance, or diabetes. In a further study using alpacas, Carroll et al. examined the effects of four dietary supplements of quinoa, barley, amaranth, or soybean meal on the gastrointestinal tracts of these animals, and accordingly found that none of the four supplements significantly altered the composition of the compartment 1 C1 microbiota in alpacas. Moreover, they detected no significant difference between normal and poor alpacas with respect to the abundance of different (Operational Taxonomic Unit) orders (74). A study in humans consuming quinoa bread showed (75) that a small but not significant change in the type of grain consumed (whole grain quinoa instead of 20 g refined wheat flour) did not significantly modulate the intestinal flora, with a small but not significant increase in the relative abundance of Firmicutes in the control (quinoa free) and quinoa bread groups. Furthermore, in their study of the effects of quinoa extract on oviparous animals, Gee et al. examined the guts of chicks treated with quinoa extract (5% quinoa fiber) administered to the amniotic cavity of eggs containing viable embryos (45). Compared with the control group, this treatment was found to promote increases in the number of goblet cells in the villi and crypts. In addition, consumption of quercetin 3-glucoside and soluble quinoa fiber was found to have beneficial effects on the morphology of the duodenal brush border membrane and duodenal microbiota. In their study assessing the effects of quinoa supplementation in fish (76), Ahmed examined the histological structure of the intestinal wall in tilapia, and found that compared with the control group, fish fed a 20% quinoa-supplemented diet were characterized by significant increases in villus width and goblet cell number, although no significant differences were observed with respect to villus length. Thus, collectively, studies on the intestinal flora of a range of different hosts have provided evidence to indicate that consumption of quinoa can have positive regulatory effects on intestinal flora, which provides further potential clues for quinoa as a drug for intestinal flora regulation.
Table 3 summarizes recent research on quinoa in terms of disease treatment (58, 77–82).
3 Summary and discussion
In this review, we discuss the potential applications of quinoa in enhancing the composition and activity of intestinal flora, with a specific focus on the different physiologically active constituents of this grain, namely, saponins, polyphenolic compounds, polysaccharides, bioactive peptides, and dietary fiber. Quinoa saponins can be hydrolyzed by the intestinal flora to yield sapogenin, which has been demonstrated to have positive effects regarding the abundance of probiotic bacteria. However, at high doses, the saponins in quinoa may have toxic side effects on the kidneys and may adversely influence the bacterial metabolism of vitamin B6 and tryptophan and the ammonia cycle of intestinal flora. Moreover, saponins derived from different varieties of quinoa characterized by different grain color have been found to have differing effects on intestinal flora. In animal experiments, quinoa quercetin was found to increase the number of probiotic bacteria (such as Bifidobacterium and Phylum Firmicutes) and reduce the number of pathogenic bacteria in the duodenum and cecum. This quercetin has also been established to have regulatory effects on the host through its hypoglycemic effects, mediated via the glucose and lipid metabolism of intestinal flora. As a crude source of carbohydrates, quinoa polysaccharides have been demonstrated to play an active role in ameliorating hyperlipidemia induced by high-fat diets, promoting the activity of probiotic strains, inducing changes in pH, and yielding SCFAs. In addition, quinoa proteins can be used as a source of bioactive peptide precursors to alleviate the symptoms of colorectal cancer in mice and enhance the production of SCFAs in colonic tissues. These proteins have also been found to promote the activities of microbial α-glucosidase, β-glucosidase, and α-galactosidase in the cecum, and contribute to reductions in cecal pH. Studies in oviparous animals have also shown that protein digestibility may alter amino acid bioavailability, thereby influencing intestinal flora composition.
Collectively, studies conducted to date on the bioactive constituents of quinoa have revealed their common effects in promoting the abundance of probiotic bacteria and inhibiting pathogens, as well as their contribution in reducing intestinal pH and promoting increases in the production of SCFAs and other key factors. Moreover, these effects have been demonstrated in a diverse range of hosts, including mammals, birds, and fish, and evaluations of the therapeutic potential of using whole-quinoa extracts have facilitated further progress in disease-related research. For example, with respect to obesity, inflammatory bowel disease, liver cancer, and diabetes, quinoa has been found to have beneficial effects on the body, mediated via its regulatory effects on the intestinal flora, which further highlights the central role of these microbes in host regulatory processes, including those associated with the gut–brain–liver and intestinal microbiota–brain routes. In addition, there is evidence to indicate that the constituents of different varieties of quinoa have slightly differing effects, which could be associated with the different growth environments of these varieties.
The research of quinoa in intestinal flora, on the one hand, the observation of intestinal flora in the biological activity function of quinoa, it is found that quinoa can promote the growth of beneficial bacteria and inhibit the growth of harmful bacteria, regulate some enzymes of intestinal flora, and then create an environment conducive to the growth of beneficial bacteria, reverse the environment of intestinal flora. For example, bioactive peptides or polyphenolic compounds of quinoa affect the expression of certain enzymes through signaling pathways, which in turn affect the intestinal flora or other diseases. The mechanisms by which quinoa improves the structure of intestinal flora need to be further explored, which may be multi-faceted, and the achievements in molecular mechanisms may give us some hints.
Based on the research conducted to date, quinoa has now reached a certain stage of therapeutic development. However, despite the significant progress in this regard, there is still a lack of relevant experimental data for the therapeutic efficacy of quinoa as a human drug. As indicated by the animal study findings, intestinal flora can be considered an important switch that quinoa can contribute to operating by regulating the constituent microbial populations. It is confidently predicted that with more in-depth research on quinoa, the utilization of this grain for medicinal purposes can be enhanced, and effective approaches will be developed for beneficially manipulating the intestinal flora. However, there are still many problems about the actual application of quinoa, such as the taste of quinoa and the control of quinoa saponin. We should not only tap the positive factors of quinoa, but also should not ignore the difficulties encountered in the reality of quinoa. Although the current market development of quinoa is not very good, with the in-depth research on the improvement mechanism of quinoa on intestinal flora, it is believed that the quinoa market will also have a new round of development in the near future, and what we need to do is to deeply explore the role of quinoa in the “homology of medicine and food”.
Author contributions
HH: Investigation, Validation, Writing – original draft. CJ: Conceptualization, Investigation, Validation, Writing – original draft. XC: Formal analysis, Investigation, Writing – original draft. LZ: Investigation, Supervision, Writing – review & editing, Funding acquisition. YJ: Investigation, Supervision, Writing – review & editing. XM: Investigation, Supervision, Validation, Writing – review & editing. XL: Funding acquisition, Investigation, Project administration, Supervision, Validation, Writing – review & editing.
Funding
The author(s) declare financial support was received for the research, authorship, and/or publication of this article. This research was funded by the Department of Science and Technology of Jilin Province (no. 20220202076NC), the Education Department of Jilin Province (no. JJKH20220183KJ), and the PhD Research Project of Jilin Engineering Normal University (no. BSKJ201923), and the project of health research talents of Jilin Province (No.2023SCZ21). The APC was funded by XL.
Acknowledgments
We would like to thank Editage for English language editing.
Conflict of interest
The authors declare that the research was conducted in the absence of any commercial or financial relationships that could be construed as a potential conflict of interest.
Publisher’s note
All claims expressed in this article are solely those of the authors and do not necessarily represent those of their affiliated organizations, or those of the publisher, the editors and the reviewers. Any product that may be evaluated in this article, or claim that may be made by its manufacturer, is not guaranteed or endorsed by the publisher.
References
1. Li, X, Ran, R, Chen, G, and Zhao, P. Genomic variation underlying the breeding selection of quinoa varieties Longli-4 and CA3-1 in China. Int J Mol Sci. (2022) 23:14030. doi: 10.3390/ijms232214030
2. Bazile, D, Jacobsen, S-E, and Verniau, A. The global expansion of quinoa: trends and limits. Front Plant Sci. (2016) 7:622. doi: 10.3389/fpls.2016.00622
3. Hussain, MI, Farooq, M, Syed, QA, Ishaq, A, Al-Ghamdi, AA, and Hatamleh, AA. Botany, nutritional value, phytochemical composition and biological activities of quinoa. Plants. (2021) 10:2258. doi: 10.3390/plants10112258
4. Filho, AMM, Pirozi, MR, Borges, JTDS, Pinheiro Sant’Ana, HM, Chaves, JBP, and Coimbra, JSDR. Quinoa: nutritional, functional, and Antinutritional aspects. Crit Rev Food Sci Nutr. (2017) 57:1618–30. doi: 10.1080/10408398.2014.1001811
5. Lin, M, Han, P, Li, Y, Wang, W, Lai, D, and Zhou, L. Quinoa secondary metabolites and their biological activities or functions. Molecules. (2019) 24:2512. doi: 10.3390/molecules24132512
6. Dakhili, S, Abdolalizadeh, L, Hosseini, SM, Shojaee-Aliabadi, S, and Mirmoghtadaie, L. Quinoa protein: composition, structure and functional properties. Food Chem. (2019) 299:125161. doi: 10.1016/j.foodchem.2019.125161
7. Ren, G, Teng, C, Fan, X, Guo, S, Zhao, G, Zhang, L, et al. Nutrient composition, functional activity and industrial applications of quinoa (Chenopodium quinoa Willd.). Food Chem. (2023) 410:135290. doi: 10.1016/j.foodchem.2022.135290
8. Tabatabaei, I, Alseekh, S, Shahid, M, Leniak, E, Wagner, M, Mahmoudi, H, et al. The diversity of quinoa morphological traits and seed metabolic composition. Sci Data. (2022) 9:323. doi: 10.1038/s41597-022-01399-y
9. Sobota, A, Świeca, M, Gęsiński, K, Wirkijowska, A, and Bochnak, J. Yellow-coated quinoa (Chenopodium quinoa Willd) - physicochemical, nutritional, and antioxidant properties. J Sci Food Agric. (2020) 100:2035–42. doi: 10.1002/jsfa.10222
10. Balakrishnan, G, and Schneider, RG. The role of Amaranth, quinoa, and millets for the development of healthy, sustainable food products-a concise review. Foods. (2022) 11:2442. doi: 10.3390/foods11162442
11. Shen, Y, Zheng, L, Peng, Y, Zhu, X, Liu, F, Yang, X, et al. Physicochemical, antioxidant and anticancer characteristics of seed oil from three Chenopodium quinoa genotypes. Molecules. (2022) 27:2453. doi: 10.3390/molecules27082453
12. Qian, G, Li, X, Zhang, H, Zhang, H, Zhou, J, Ma, X, et al. Metabolomics analysis reveals the accumulation patterns of flavonoids and phenolic acids in quinoa (Chenopodium quinoa Willd.) grains of different colors. Food Chem X. (2023) 17:100594. doi: 10.1016/j.fochx.2023.100594
13. Ren, G, Zhu, Y, Shi, Z, and Li, J. Detection of Lunasin in quinoa (Chenopodium quinoa Willd.) and the in vitro evaluation of its antioxidant and anti-inflammatory activities. J Sci Food Agric. (2017) 97:4110–6. doi: 10.1002/jsfa.8278
14. Souid, A, Bellani, L, Tassi, EL, Ben Hamed, K, Longo, V, and Giorgetti, L. Early physiological, cytological and Antioxidative responses of the edible halophyte Chenopodium quinoa exposed to salt stress. Antioxidants. (2023) 12:1060. doi: 10.3390/antiox12051060
15. Ji, X, Wang, L, Zhao, J, and Jiang, J. Possible role of polypeptide-Chlorogenic acid interaction in the physicochemical and sensory characteristics of quinoa-modified coffee beverage. Food Chem. (2023) 425:136359. doi: 10.1016/j.foodchem.2023.136359
16. Valenzuela Zamudio, F, and Segura Campos, MR. Amaranth, quinoa and chia bioactive peptides: a comprehensive review on three ancient grains and their potential role in management and prevention of type 2 diabetes. Crit Rev Food Sci Nutr. (2022) 62:2707–21. doi: 10.1080/10408398.2020.1857683
17. Yao, Y, Yang, X, Shi, Z, and Ren, G. Anti-inflammatory activity of Saponins from quinoa (Chenopodium quinoa Willd.) seeds in lipopolysaccharide-stimulated RAW 264.7 macrophages cells. J Food Sci. (2014) 79:H1018–23. doi: 10.1111/1750-3841.12425
18. Sidorova, YS, Shipelin, VA, Petrov, NA, Zorin, SN, and Mazo, VK. Anxiolytic and antioxidant effect of Phytoecdysteroids and polyphenols from Chenopodium quinoa on an in vivo restraint stress model. Molecules. (2022) 27:9003. doi: 10.3390/molecules27249003
19. Hu, Y, Zhang, J, Zou, L, Fu, C, Li, P, and Zhao, G. Chemical characterization, antioxidant, immune-regulating and anticancer activities of a novel bioactive polysaccharide from Chenopodium quinoa seeds. Int J Biol Macromol. (2017) 99:622–9. doi: 10.1016/j.ijbiomac.2017.03.019
20. Fernández-López, J, Viuda-Martos, M, Sayas-Barberá, ME, Navarro-Rodríguez de Vera, C, Lucas-González, R, Roldán-Verdú, A, et al. Chia, Quinoa, and their coproducts as potential antioxidants for the meat industry. Plants. (2020) 9:1359. doi: 10.3390/plants9101359
21. He, X, Yang, W, Zhao, Q, and Qin, X. Controlled oxidation and digestion of Pickering emulsions stabilized by quinoa protein and (−)-Epigallocatechin-3-Gallate (EGCG) hybrid particles. Int J Biol Macromol. (2023) 253:126755. doi: 10.1016/j.ijbiomac.2023.126755
22. Guo, H, Hao, Y, Yang, X, Ren, G, and Richel, A. Exploration on bioactive properties of quinoa protein hydrolysate and peptides: a review. Crit Rev Food Sci Nutr. (2023) 63:2896–909. doi: 10.1080/10408398.2021.1982860
23. Liu, K, Chen, Y-Y, Li, X-Y, Li, Q-M, Pan, L-H, Luo, J-P, et al. Hydrolytic quinoa protein and cationic Lotus root starch-based micelles for co-delivery of quercetin and Epigallo-Catechin 3-Gallate in ulcerative colitis treatment. J Agric Food Chem. (2022) 70:15189–201. doi: 10.1021/acs.jafc.2c06376
24. Agarwal, A, Rizwana, N, Tripathi, AD, Kumar, T, Sharma, KP, and Patel, SKS. Nutritional and functional new perspectives and potential health benefits of quinoa and chia seeds. Antioxidants. (2023) 12:1413. doi: 10.3390/antiox12071413
25. Pathan, S, and Siddiqui, RA. Nutritional composition and bioactive components in quinoa (Chenopodium quinoa Willd.) greens: a review. Nutrients. (2022) 14:558. doi: 10.3390/nu14030558
26. López-Cervantes, J, Sánchez-Machado, D, De la Mora-López, DS, and Sanches-Silva, A. Quinoa (Chenopodium quinoa Willd.): exploring a superfood from Andean indigenous cultures with potential to reduce cardiovascular disease (CVD) risk markers. Curr Mol Pharmacol. (2021) 14:925–34. doi: 10.2174/1874467214999210111222233
27. López-Moreno, M, Jiménez-Moreno, E, Márquez Gallego, A, Vera Pasamontes, G, Uranga Ocio, JA, Garcés-Rimón, M, et al. Red quinoa hydrolysates with antioxidant properties improve cardiovascular health in spontaneously hypertensive rats. Antioxidants. (2023) 12:1291. doi: 10.3390/antiox12061291
28. An, T, Liu, J-X, Yang, X-Y, Lv, B-H, Wu, Y-X, and Jiang, G-J. Supplementation of quinoa regulates glycolipid metabolism and endoplasmic reticulum stress in the high-fat diet-induced female obese mice. Nutr Metab. (2021) 18:95. doi: 10.1186/s12986-021-00622-8
29. Pedrali, D, Giupponi, L, De la Peña-Armada, R, Villanueva-Suárez, MJ, and Mateos-Aparicio, I. The quinoa variety influences the nutritional and antioxidant profile rather than the geographic factors. Food Chem. (2023) 402:133531. doi: 10.1016/j.foodchem.2022.133531
30. Chen, Y, Zhou, J, and Wang, L. Role and mechanism of gut microbiota in human disease. Front Cell Infect Microbiol. (2021) 11:625913. doi: 10.3389/fcimb.2021.625913
31. Sebastián Domingo, JJ, and Sánchez Sánchez, C. From the intestinal Flora to the microbiome. Rev Esp Enferm Dig. (2018) 110:51–6. doi: 10.17235/reed.2017.4947/2017
32. Bäumler, AJ, and Sperandio, V. Interactions between the microbiota and pathogenic Bacteria in the gut. Nature. (2016) 535:85–93. doi: 10.1038/nature18849
33. Kim, S-K, Guevarra, RB, Kim, Y-T, Kwon, J, Kim, H, Cho, JH, et al. Role of probiotics in human gut microbiome-associated diseases. J Microbiol Biotechnol. (2019) 29:1335–40. doi: 10.4014/jmb.1906.06064
34. Ugural, A, and Akyol, A. Can Pseudocereals modulate microbiota by functioning as probiotics or prebiotics? Crit Rev Food Sci Nutr. (2022) 62:1725–39. doi: 10.1080/10408398.2020.1846493
35. Sergeev, IN, Aljutaily, T, Walton, G, and Huarte, E. Effects of Synbiotic supplement on human gut microbiota, body composition and weight loss in obesity. Nutrients. (2020) 12:222. doi: 10.3390/nu12010222
36. Koh, A, Molinaro, A, Ståhlman, M, Khan, MT, Schmidt, C, Mannerås-Holm, L, et al. Microbially produced imidazole propionate impairs insulin signaling through mTORC1. Cell. (2018) 175:947–961.e17. doi: 10.1016/j.cell.2018.09.055
37. Gurung, M, Li, Z, You, H, Rodrigues, R, Jump, DB, Morgun, A, et al. Role of gut microbiota in type 2 diabetes pathophysiology. EBioMedicine. (2020) 51:102590. doi: 10.1016/j.ebiom.2019.11.051
38. Qiu, P, Ishimoto, T, Fu, L, Zhang, J, Zhang, Z, and Liu, Y. The gut microbiota in inflammatory bowel disease. Front Cell Infect Microbiol. (2022) 12:733992. doi: 10.3389/fcimb.2022.733992
39. Yue, X. Effects of Tartary buckwheat polysaccharides on gut microbiota and its application in the development of yogurt. Master degree dissertation. Chengdu: Chengdu University (2022).
40. Zhao, Q, Hou, D, Fu, Y, Xue, Y, Guan, X, and Shen, Q. Adzuki bean alleviates obesity and insulin resistance induced by a high-fat diet and modulates gut microbiota in mice. Nutrients. (2021) 13:3240. doi: 10.3390/nu13093240
41. Tian, T, Xin-Li, P, and Hai-Ying, L. Research on the effects of various intake levels of buckwheat on intestinal function and intestinal Flora in Hypercholesterolemic mice. Sci Technol Cerealsoils Foods. (2021) 29:190–7. doi: 10.16210/j.cnki.1007-7561.2021.06.017
42. Li, W, Song, Y, Cao, Y-N, Zhang, L-L, Zhao, G, Wu, D-T, et al. Total Saponins from quinoa bran alleviate high-fat diet-induced obesity and systemic inflammation via regulation of gut microbiota in rats. Food Sci Nutr. (2022) 10:3876–89. doi: 10.1002/fsn3.2984
43. Del Hierro, JN, Cueva, C, Tamargo, A, Núñez-Gómez, E, Moreno-Arribas, MV, Reglero, G, et al. In vitro colonic fermentation of Saponin-rich extracts from quinoa, lentil, and fenugreek. Effect on Sapogenins yield and human gut microbiota. J Agric Food Chem. (2020) 68:106–16. doi: 10.1021/acs.jafc.9b05659
44. Ruoyu, Z, and Fei, Y. Changes of urine metabolism and gut microbiome in rats after quinoa Saponins Oral treatment. Mod Food Sci Technol. 38:999. doi: 10.13982/j.mfst.1673-9078.2022.8.0999
45. Gee, JM, Price, KR, Ridout, CL, Wortley, GM, Hurrell, RF, and Johnson, IT. Saponins of quinoa (Chenopodium quinoa): effects of processing on their abundance in quinoa products and their biological effects on intestinal mucosal tissue. J Sci Food Agric. (1993) 63:201–9. doi: 10.1002/jsfa.2740630206
46. Chen, J, Zhang, Y, Guan, X, Cao, H, Li, L, Yu, J, et al. Characterization of Saponins from differently colored quinoa cultivars and their in vitro gastrointestinal digestion and fermentation properties. J Agric Food Chem. (2022) 70:1810–8. doi: 10.1021/acs.jafc.1c06200
47. Zhang, R, Zhai, Q, Yu, Y, Li, X, Zhang, F, Hou, Z, et al. Safety assessment of crude Saponins from Chenopodium quinoa Willd. Husks: 90-day Oral toxicity and gut Microbiota & Metabonomics Study in rats. Food Chem. (2022) 375:131655. doi: 10.1016/j.foodchem.2021.131655
48. Agarwal, N, Kolba, N, Khen, N, Even, C, Turjeman, S, Koren, O, et al. Quinoa soluble Fiber and quercetin Alter the composition of the gut microbiome and improve brush border membrane morphology in vivo (Gallus gallus). Nutrients. (2022) 14:448. doi: 10.3390/nu14030448
49. Kim, YS, and Ho, SB. Intestinal goblet cells and mucins in health and disease: recent insights and Progress. Curr Gastroenterol Rep. (2010) 12:319–30. doi: 10.1007/s11894-010-0131-2
50. Hemalatha, P, Bomzan, DP, Sathyendra Rao, BV, and Sreerama, YN. Distribution of phenolic antioxidants in whole and milled fractions of quinoa and their inhibitory effects on α-amylase and α-glucosidase activities. Food Chem. (2016) 199:330–8. doi: 10.1016/j.foodchem.2015.12.025
51. Zhang, Y, Bai, B, Yan, Y, Liang, J, and Guan, X. Bound polyphenols from red quinoa prevailed over free polyphenols in reducing postprandial blood glucose rises by inhibiting α-glucosidase activity and starch digestion. Nutrients. (2022) 14:728. doi: 10.3390/nu14040728
52. Yurong, J, Junjie, Y, Xueting, S, Shuying, W, Guoquan, L, and Qian, M. Study on the best extraction technology and antioxidation of quinoa leaf polysaccharide. Chin J Food Sci. (2017) 17:110–8. doi: 10.16429/j.1009-7848.2017.02.015
53. Zeyneb, H, Pei, H, Cao, X, Wang, Y, Win, Y, and Gong, L. In vitro study of the effect of quinoa and quinoa polysaccharides on human gut microbiota. Food Sci Nutr. (2021) 9:5735–45. doi: 10.1002/fsn3.2540
54. Cao, Y, Zou, L, Li, W, Song, Y, Zhao, G, and Hu, Y. Dietary quinoa (Chenopodium quinoa Willd.) polysaccharides ameliorate high-fat diet-induced hyperlipidemia and modulate gut microbiota. Int J Biol Macromol. (2020) 163:55–65. doi: 10.1016/j.ijbiomac.2020.06.241
55. Liu, J, Wang, Z, Mai, P, Hao, Y, Wang, Z, and Wang, J. Quinoa bran soluble dietary Fiber ameliorates dextran sodium sulfate induced ulcerative colitis in BALB/c mice by maintaining intestinal barrier function and modulating gut microbiota. Int J Biol Macromol. (2022) 216:75–85. doi: 10.1016/j.ijbiomac.2022.06.194
56. Tang, Y, Li, X, Zhang, B, Chen, PX, Liu, R, and Tsao, R. Characterisation of Phenolics, Betanins and antioxidant activities in seeds of three Chenopodium quinoa Willd. Genotypes Food Chem. (2015) 166:380–8. doi: 10.1016/j.foodchem.2014.06.018
57. Tang, Y, Zhang, B, Li, X, Chen, PX, Zhang, H, Liu, R, et al. Bound Phenolics of quinoa seeds released by acid, alkaline, and enzymatic treatments and their antioxidant and α-glucosidase and pancreatic lipase inhibitory effects. J Agric Food Chem. (2016) 64:1712–9. doi: 10.1021/acs.jafc.5b05761
58. Liu, W, Zhang, Y, Qiu, B, Fan, S, Ding, H, and Liu, Z. Quinoa whole grain diet compromises the changes of gut microbiota and colonic colitis induced by dextran sulfate sodium in C57BL/6 mice. Sci Rep. (2018) 8:14916. doi: 10.1038/s41598-018-33092-9
59. Guo, H, Hao, Y, Fan, X, Richel, A, Everaert, N, Yang, X, et al. Administration with quinoa protein reduces the blood pressure in spontaneously hypertensive rats and modifies the fecal microbiota. Nutrients. (2021) 13:2446. doi: 10.3390/nu13072446
60. Fan, X, Guo, H, Teng, C, Yang, X, Qin, P, Richel, A, et al. Supplementation of quinoa peptides alleviates colorectal Cancer and restores gut microbiota in AOM/DSS-treated mice. Food Chem. (2023) 408:135196. doi: 10.1016/j.foodchem.2022.135196
61. Fotschki, B, Juśkiewicz, J, Jurgoński, A, Amarowicz, R, Opyd, P, Bez, J, et al. Protein-rich flours from quinoa and buckwheat Favourably affect the growth parameters, intestinal microbial activity and plasma lipid profile of rats. Nutrients. (2020) 12:2781. doi: 10.3390/nu12092781
62. Amiri, MYA, Jafari, MA, and Irani, M. Growth performance, internal organ traits, intestinal morphology, and microbial population of broiler chickens fed quinoa seed-based diets with Phytase or protease supplements and their combination. Trop Anim Health Prod. (2021) 53:535. doi: 10.1007/s11250-021-02980-0
63. Dostalíková, L, Hlásná Čepková, P, Janovská, D, Svoboda, P, Jágr, M, Dvořáček, V, et al. Nutritional evaluation of quinoa genetic resources growing in the climatic conditions of Central Europe. Foods. (2023) 12:1440. doi: 10.3390/foods12071440
64. Kuljanabhagavad, T, Thongphasuk, P, Chamulitrat, W, and Wink, M. Triterpene Saponins from Chenopodium quinoa Willd. Phytochemistry. (2008) 69:1919–26. doi: 10.1016/j.phytochem.2008.03.001
65. Li, Y, Feng, Z, Wu, T, You, H, Wang, W, Liu, X, et al. Quinoa peptides alleviate obesity in mice induced by a high-fat diet via regulating of the PPAR-α/γ signaling pathway and gut microbiota. Mol Nutr Food Res. (2023) 67:e2300258. doi: 10.1002/mnfr.202300258
66. Ruales, J, and Nair, BM. Properties of starch and dietary fibre in raw and processed quinoa (Chenopodium quinoa, Willd) seeds. Plant Foods Hum Nutr. (1994) 45:223–46. doi: 10.1007/BF01094092
67. Markova, YM, and Sidorova, YS. Amaranth, quinoa and buckwheat grain products: role in human nutrition and maintenance of the intestinal microbiome. Vopr Pitan. (2022) 91:17–29. doi: 10.33029/0042-8833-2022-91-6-17-29
68. El Hazzam, K, Hafsa, J, Sobeh, M, Mhada, M, Taourirte, M, El Kacimi, K, et al. An insight into Saponins from quinoa (Chenopodium quinoa Willd): a review. Molecules. (2020) 25:1059. doi: 10.3390/molecules25051059
69. Feng, Y, Fan, X, Zhang, S, Wu, T, Bai, L, Wang, H, et al. Effects of variety and origin on the metabolic and texture characteristics of quinoa seeds based on ultrahigh-performance liquid chromatography coupled with high-field quadrupole-Orbitrap high-resolution mass spectrometry. Food Res Int. (2022) 162:111693. doi: 10.1016/j.foodres.2022.111693
70. Karonen, M, and Pihlava, J-M. Identification of Oxindoleacetic acid conjugates in quinoa (Chenopodium quinoa Willd.) seeds by high-resolution UHPLC-MS/MS. Molecules. (2022) 27:5629. doi: 10.3390/molecules27175629
71. Gullón, B, Gullón, P, Tavaria, FK, and Yáñez, R. Assessment of the prebiotic effect of quinoa and Amaranth in the human intestinal ecosystem. Food Funct. (2016) 7:3782–8. doi: 10.1039/c6fo00924g
72. Bianchi, F, Rossi, EA, Sakamoto, IK, Adorno, MAT, Van de Wiele, T, and Sivieri, K. Beneficial effects of fermented vegetal beverages on human gastrointestinal microbial ecosystem in a simulator. Food Res. Int. (2014) 64:43–52. doi: 10.1016/j.foodres.2014.05.072
73. Traughber, ZT, He, F, Hoke, JM, Davenport, GM, Rodriguez-Zas, SL, Southey, BR, et al. Ancient grains as novel dietary carbohydrate sources in canine diets. J Anim Sci. (2021) 99:skab 080. doi: 10.1093/jas/skab080
74. Carroll, C, Olsen, KD, Ricks, NJ, Dill-McFarland, KA, Suen, G, Robinson, TF, et al. Bacterial communities in the alpaca gastrointestinal tract vary with diet and body site. Front Microbiol. (2018) 9:3334. doi: 10.3389/fmicb.2018.03334
75. Li, L, Houghton, D, Lietz, G, Watson, A, Stewart, CJ, Bal, W, et al. Impact of daily consumption of whole-grain quinoa-enriched bread on gut microbiome in males. Nutrients. (2022) 14:4888. doi: 10.3390/nu14224888
76. Ahmed, SAA, Abd El-Rahman, GI, Behairy, A, Beheiry, RR, Hendam, BM, Alsubaie, FM, et al. Influence of feeding quinoa (Chenopodium quinoa) seeds and prickly pear fruit (Opuntia Ficus Indica) Peel on the immune response and resistance to Aeromonas sobria infection in Nile Tilapia (Oreochromis niloticus). Animals. (2020) 10:2266. doi: 10.3390/ani10122266
77. Garcia-Mazcorro, JF, Mills, D, and Noratto, G. Molecular exploration of fecal microbiome in quinoa-supplemented obese mice. FEMS Microbiol Ecol. (2016) 92:fiw089. doi: 10.1093/femsec/fiw089
78. Wang, T-Y, Tao, S-Y, Wu, Y-X, An, T, Lv, B-H, Liu, J-X, et al. Quinoa reduces high-fat diet-induced obesity in mice via potential microbiota-gut-brain-liver interaction mechanisms. Microbiol Spectr. (2022) 10:e0032922. doi: 10.1128/spectrum.00329-22
79. Llopis, JM, Brown, D, and Saiz, B. Chenopodium quinoa and Salvia hispanica provide immunonutritional agonists to ameliorate hepatocarcinoma severity under a high-fat diet. Nutrients. (2020) 12:946. doi: 10.3390/nu12071946
80. Gual-Grau, A, Guirro, M, Crescenti, A, Boqué, N, and Arola, L. In vitro Fermentability of a broad range of natural ingredients by fecal microbiota from lean and obese individuals: potential health benefits. Int J Food Sci Nutr. (2022) 73:195–209. doi: 10.1080/09637486.2021.1954144
81. Liucheng, L. Study on quinoa alleviating diabetic symptoms in db/db mice by regulating insulin pathway and changing intestinal intestinal microbiome. Master degree dissertation. Jinan: Shandong University (2021).
82. Ahmadi, S, Nagpal, R, Wang, S, Gagliano, J, Kitzman, DW, Soleimanian-Zad, S, et al. Prebiotics from acorn and sago prevent high-fat-diet-induced insulin resistance via microbiome-gut-brain Axis modulation. J Nutr Biochem. (2019) 67:1–13. doi: 10.1016/j.jnutbio.2019.01.011
83. Fan, X, Guo, H, Teng, C, Zhang, B, Blecker, C, and Ren, G. Anti-Colon Cancer activity of novel peptides isolated from in vitro digestion of quinoa protein in Caco-2 cells. Foods. (2022) 11:194. doi: 10.3390/foods11020194
Keywords: intestinal flora, grain product, quinoa, SCFA, polyphenol compounds, saponins, polysaccharides, bioactive peptides
Citation: Huang H, Jia C, Chen X, Zhang L, Jiang Y, Meng X and Liu X (2024) Progress in research on the effects of quinoa (Chenopodium quinoa) bioactive compounds and products on intestinal flora. Front. Nutr. 11:1308384. doi: 10.3389/fnut.2024.1308384
Edited by:
Virginia Teresa Glicerina, University of Bologna, ItalyReviewed by:
Malgorzata Ziarno, Warsaw University of Life Sciences, PolandMaría Chávarri, Tecnalia Research and Innovation, Spain
Copyright © 2024 Huang, Jia, Chen, Zhang, Jiang, Meng and Liu. This is an open-access article distributed under the terms of the Creative Commons Attribution License (CC BY). The use, distribution or reproduction in other forums is permitted, provided the original author(s) and the copyright owner(s) are credited and that the original publication in this journal is cited, in accordance with accepted academic practice. No use, distribution or reproduction is permitted which does not comply with these terms.
*Correspondence: Xianjun Liu, bGl1eGlhbmp1bkBqbGVudS5lZHUuY24=; Xianglong Meng, bWVuZ3hsamR5eUBqbHUuZWR1LmNu
†These authors have contributed equally to this work