- Research & Development Institute, House Wellness Foods Corp., Itami, Hyogo, Japan
The spice turmeric, which has the Latin name Curcuma longa (C. longa), has various physiological effects. This study evaluated the effects of a hot water mixture with supercritical carbon dioxide C. longa extracts, CLE, and the potential active components of C. longa, turmeronols A and B and bisacurone on inflammation and glucose metabolism. First, we investigated the effect of CLE and the potential active components of C. longa on lipopolysaccharide-induced inflammation in RAW264.7 macrophages. We found a significant decrease in the production of interleukin (IL)-1β, IL-6, tumor necrosis factor (TNF)-α, and nitric oxide with CLE, turmeronol A, and bisacurone, Significant inhibition of each of these substances was also observed, except for TNF-α with turmeronol B. The second part of our work was a 12-week randomized, double-blind, placebo-controlled study in healthy but borderline adults aged 40 to 69 years with overweight and normal/prediabetes glycemia. We compared blood inflammatory and glycometabolic markers in the CLE (n = 55) and placebo groups (n = 55). We found significantly lower serum high-sensitivity C-reactive protein and hemoglobin A1c levels in the CLE group. This group also showed significant improvements in postprandial hyperglycemia and insulin sensitivity indices. Our findings indicate that CLE may reduce low-grade inflammation and thus improve insulin sensitivity and postprandial hyperglycemia.
Clinical trial registration: https://center6.umin.ac.jp/cgi-open-bin/ctr/ctr_view.cgi?recptno=R000051492, UMIN-CTR, UMIN000045106.
1 Introduction
Acute inflammation is a physiological process for eliminating pathogens and dead cells and induces the repair and regeneration of damaged tissue. In contrast, chronic inflammation is an inflammatory response that is low-grade, persistent, and mediated by long-lived immune cells such as macrophages (1). The latter type of inflammation is induced by obesity (2), aging (3), and unhealthy lifestyle factors, such as unbalanced diet, physical inactivity, insufficient sleep, and psychological stress (1, 4, 5). Macrophages play a key role in the development of chronic inflammation by producing inflammatory mediators that induce cell damage, tissue infiltration of inflammatory cells, and impairment of systemic metabolisms such as glycometabolism (1, 6). Clinical human studies generally use C-reactive protein (CRP) as a marker of low-grade, systemic inflammation (5, 7), and previous studies have shown that mildly elevated CRP is associated with an increased risk of metabolic syndrome (8), diabetes (9), impaired cognitive function (10), coronary heart disease (11), and cancer (12).
Glucose is one of the energy sources for mammalian cells and has an important role in maintaining normal physiological homeostasis in humans (13). High systemic glucose levels are reduced by insulin, which is released from the pancreas and promotes glucose uptake in insulin-sensitive peripheral tissues, such as skeletal muscles, and inhibits hepatic gluconeogenesis (14). Excessive intake of nutrients such as carbohydrate and fat causes obesity through accumulation of visceral fat, which contributes to abnormal glycometabolism (14). One method for evaluating glucose tolerance, insulin secretory capacity, and insulin sensitivity is the oral glucose tolerance test (OGTT), which measures blood levels of glucose and insulin after oral administration of 75 g of glucose. The OGTT is also widely used to diagnose impaired glucose tolerance (prediabetes) and type 2 diabetes. As a marker of early impaired glucose homeostasis, the post-load glucose level in the OGTT is a more sensitive marker of prediabetes than fasting blood glucose and hemoglobin A1c (HbA1c) (15). Individuals with overweight or obesity have been reported to show an increased number of activated macrophages, which produce inflammatory mediators that can inhibit the action of insulin (6, 16). These chronic inflammatory states contribute to the induction of impaired insulin sensitivity (also called insulin resistance) and the development of type 2 diabetes (1, 5). In previous clinical studies, participants with overweight and low-grade inflammation exhibited a significant increase in glucose plasma levels in the OGTT (17).
The spice turmeric, which has the Latin name Curcuma longa (C. longa), belongs to the Zingiberaceae family and exerts a range of physiological effects (18). Curcumin, a lipophilic polyphenol, is a major component of C. longa and has various physiological activities, including anti-oxidant, anti-inflammation, and anti-obesity actions (19, 20). Curcumin also prevents the development of endothelial dysfunction and atherosclerosis (20). Curcumin-free C. longa water extracts have also been reported to exhibit numerous activities (21). Other components of C. longa include the sesquiterpenoids turmeronols A and B and bisacurone (22, 23). Turmeronols are known to inhibit the production of inflammatory mediators in immune cell lines by inhibiting the signaling pathway of nuclear factor kappa-light-chain-enhancer of activated B cells (NF-kB) (24). Bisacurone inhibits adhesion of monocytes to endothelial cells through down-regulation of vascular cell adhesion molecule 1 (VCAM-1) protein expression (25). In addition, water extracts of C. longa as well as bisacurone were reported to inhibit ethanol-induced liver injury (26). In diabetic animal models, bisacurone was found to reduce systemic glucose levels and suppress cardiotoxicity and nephropathy (27). C. longa water extracts are known to exert anti-oxidant and anti-inflammatory effects (28–30), prevent atrophy of skeletal muscle (31), increase dermal water content (32), reduce fatigue (33), and improve depression (34). In animal disease models, C. longa water extracts decreased expression of inflammatory cytokine and chemokine mRNA and prevented chronic inflammatory diseases, e.g., carbon tetrachloride-induced hepatitis (35) and non-alcoholic steatohepatitis (36). Also, in animal models, supercritical carbon dioxide C. longa extracts suppressed inflammation induced by carrageenan (37). A recent study using streptozotocin-induced diabetic rats demonstrated that C. longa extracts improved postprandial glucose levels (38). In addition, daily intake of a mixture of hot water and supercritical carbon dioxide C. longa extracts (CLE) improved mental health-related quality of life questionnaire scores (22, 23). However, to date, the effects of CLE on chronic inflammatory processes and postprandial glucose metabolism in humans remain unclear.
Therefore, to investigate whether CLE and its potential active ingredients turmeronols A and B and bisacurone have anti-inflammatory activities, we first conducted a non-clinical study in which we assessed their effects on the production of inflammatory mediators in macrophage cells stimulated with lipopolysaccharide (LPS). Subsequently, to evaluate the effect of CLE on chronic inflammation and postprandial glycometabolism, we conducted a randomized, double-blind, placebo-controlled trial to measure the levels of inflammatory markers and glycometabolic markers in male and female participants with overweight and glycemia in the normal/prediabetes range.
2 Materials and methods
2.1 Non-clinical study
2.1.1 Reagents
We used the following reagents: Dulbecco’s modified Eagle’s medium (DMEM), penicillin–streptomycin, LPS (Escherichia coli O127:B8; L3129), and dimethyl sulfoxide (Sigma-Aldrich, Saint Louis, MO, USA); fetal bovine serum (FBS) (HyClone, Logan, UT, USA); turmeronols A and B and bisacurone isolated from C. longa (Nagara Science, Gifu, Japan); and sulfanilamide, N-1-naphthylenediamide dihydrochloride, phosphoric acid, and sodium nitrite (Fujifilm Wako, Osaka, Japan).
2.1.2 Preparation of CLE
CLE (Turmeric Extract Mixture, House Wellness Foods) was produced as described elsewhere (22, 28, 39). In brief, for the hot water extract, C. longa rhizomes were placed in water at 98°C for 1 h, then the concentrated supernatant was combined with dextrin and spray dried. The supercritical carbon dioxide extract was obtained by placing rhizomes in carbon dioxide at 65°C at a pressure of 150 bar for 6 h. Then, the supernatant was concentrated, combined with vegetable oil, and filtered (membrane pore size, 1 μm). These two C. longa extracts were mixed at a ratio of 8 to 1 (the hot water extracts to the supercritical carbon dioxide extracts) and the mixture was used as CLE (Figure 1). The safety of CLE was confirmed in a previous clinical intervention study in which participants consumed excess doses of CLE (10 capsules per day, i.e., a 5-fold higher dose than in this study). The previous study found no adverse effects of the intervention on physical measurements, hematology and biochemistry tests, or urinalysis (23).
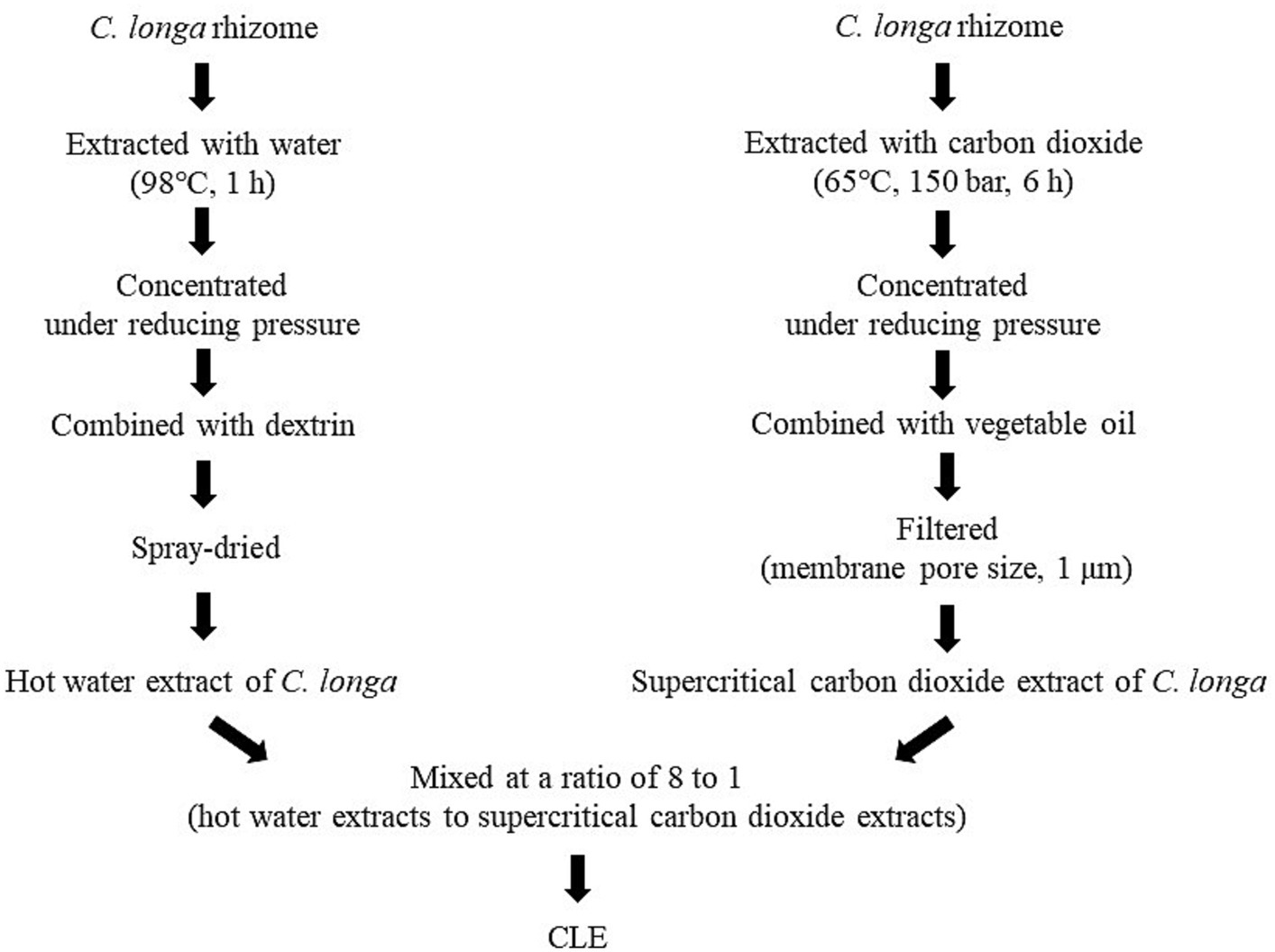
Figure 1. Preparation flow diagram of CLE. CLE, a mixture of a hot water extract and a supercritical carbon dioxide extract of Curcuma longa.
2.1.3 Measurement of turmeronols and bisacurone in CLE
CLE was dissolved in ethyl acetate, vigorously mixed with an equal volume of distilled water, and centrifuged at 400 × g for 5 min at room temperature. The ethyl acetate layer was collected and evaporated to dryness under nitrogen to remove the ethyl acetate. The residue was dissolved in methanol and then filtered and injected into an HPLC system under the following conditions. Turmeronol A: Separation was carried out on an octadecylsilyl (ODS) column (Chemical Evaluation Research Institute, Tokyo, Japan) with a mobile phase consisting of 0.1% formic acid-acetonitrile (52:48, v/v), and the derivative was detected at 238 nm. Turmeronol B: Separation was performed on a pyrenylethyl column (Nacalai Tesque Inc., Kyoto, Japan) with a mobile phase composed of distilled water–methanol (43:57, v/v), and the derivative was detected at 238 nm. Bisacurone: Separation was performed on an ODS column (FUJIFILM Wako Pure Chemical Corp., Osaka, Japan) with a mobile phase consisting of acetonitrile-0.005% trifluoroacetic acid (30,70, v/v), and the derivative was detected at 240 nm.
2.1.4 Cell culture
To create the cell line, RAW264.7 mouse macrophages (RIKEN BioResource Center, Tokyo, Japan) were seeded on 96-well plates (1.5 × 105 cells/well) and incubated for 24 h in 150 μL of DMEM with 10% FBS, 100 U/mL penicillin, and 100 μg/mL streptomycin at 37°C with 5% carbon dioxide. The cells were separated from the medium and preincubated for 1 h in FBS-free DMEM containing 600 μg/mL of CLE or turmeronol A, turmeronol B, or bisacurone; the concentrations of these potential active ingredients were determined beforehand and were equivalent to those naturally found in CLE, as follows: turmeronols A and B, both 1.1 μM, and bisacurone, 4.2 μM. In previous animal and clinical studies, the markers of inflammation and glycometabolism were improved by various plant extracts, including Houttuynia cordata Thunb. and Morus alba L. (40–43). These extracts significantly inhibited the production of inflammatory mediators at approximately 300–800 μg/mL in Raw264.7 cells (41, 44). The concentration of CLE was determined to be 600 μg/mL by conducting preliminary experiments based on these reports. Preliminary experiments had confirmed that none of the potential active components of CLE were cytotoxic (24, 45). In the last step, the cells were stimulated with LPS (final concentration, 200 ng/mL) for 15 h, and inflammatory factors were assessed in culture supernatants.
2.1.5 Measurement of inflammatory mediators
We used DuoSet enzyme-linked immunosorbent assay (ELISA) kits for mouse interleukin (IL)-1β, IL-6, and tumor necrosis factor (TNF)-α (R&D Systems, Rochester, MN, USA) to determine the concentrations of these inflammatory mediators. Procedures were performed according to the manufacturer’s instructions. In addition, we applied the Griess method to assess nitrite, a stable metabolite of nitric oxide (NO) (46, 47).
2.1.6 Statistical analysis
We used one-way analysis of variance (ANOVA) and Dunnett’s multiple comparison test to compare LPS-stimulated control and treated cells. Results are presented as means and standard deviation (SD). The statistical significance level was set at 0.05. Statcel 4 software (OMS Publishing, Tokorozawa, Japan) was used for statistical analysis.
2.2 Clinical intervention study
2.2.1 Study design
After the non-clinical study, we performed a 12-week, randomized, double-blind, placebo-controlled study from July to December 2021 at the Chiyoda Paramedical Care Clinic in Tokyo, Japan, by a contract research organization (CRO; CPCC Co., Ltd., Tokyo, Japan). The study was registered with the University hospital Medical Information Network (UMIN; registration number, UMIN000045106), approved by the institutional review board of Chiyoda Paramedical Care Clinic, and performed in accordance with the principles of the Declaration of Helsinki. All participants provided written informed consent prior to their enrollment in the study. The Consolidated Standards of Reporting Trials (CONSORT) 2010 flowchart and checklist (48) are provided as Figure 2 and Supplementary Table S1, respectively.
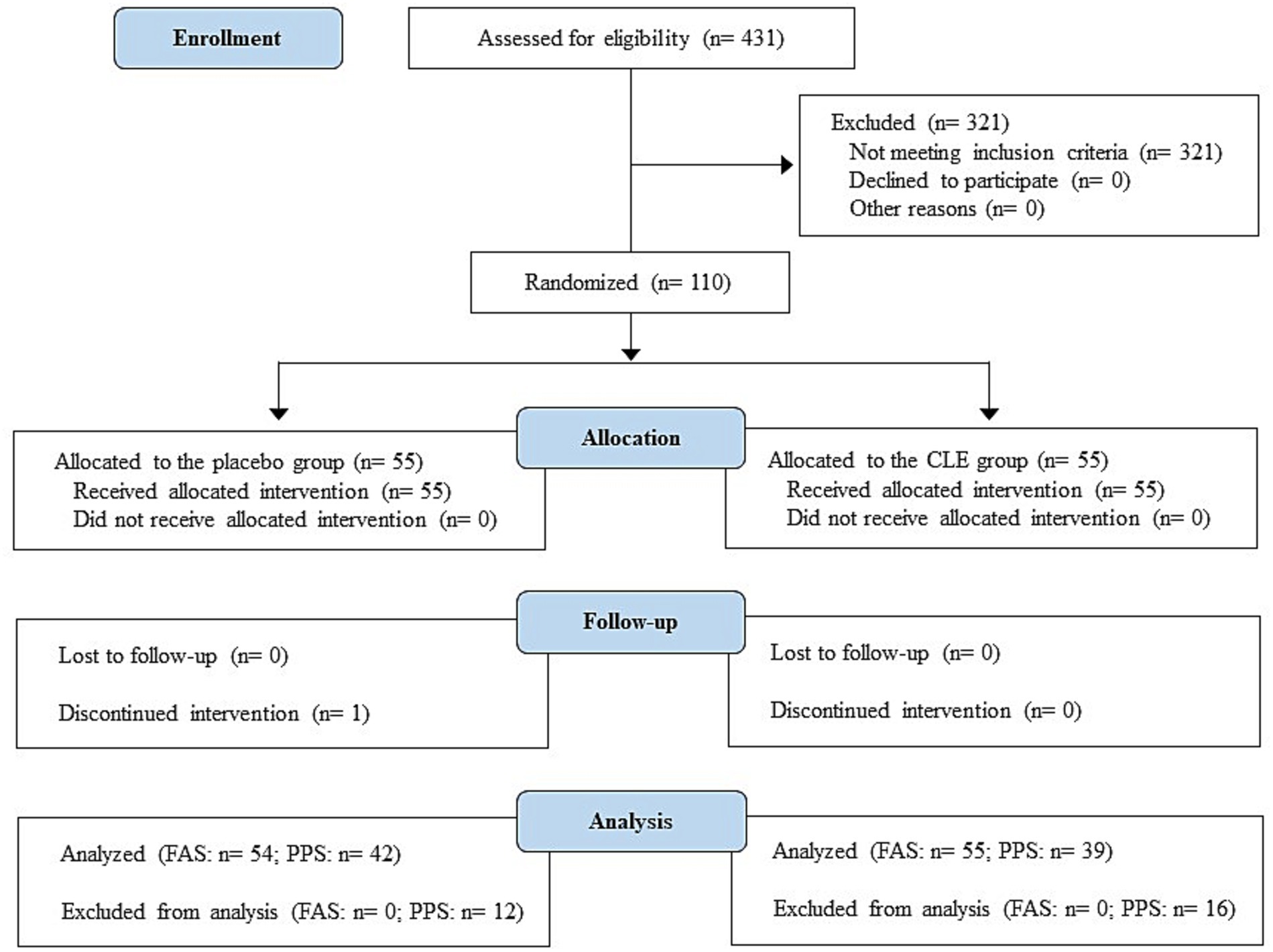
Figure 2. Study flow diagram (CONSORT 2010). CLE, a mixture of a hot water extract and a supercritical carbon dioxide extract of Curcuma longa; FAS, full analysis set; PPS, per-protocol set.
2.2.2 Enrollment of participants
A total of 431 consecutive male and female patients at Chiyoda Paramedical Care Clinic were recruited and assessed for eligibility from July to September 2021. The inclusion criteria were age 40 to 69 years, overweight (body mass index [BMI] ≥ 23 to <30 kg/m2) (49), and normal/prediabetes glycemia (fasting plasma glucose, < 126 mg/dL; 2-h post-load plasma glucose during OGTT, < 200 mg/dL) (50). The exclusion criteria were (1) current or planned dietary carbohydrate restriction with the aim to lose weight during the scheduled study period; (2) continued intake 3 or more times per week of medications or nutraceutical/functional foods that may influence the results of this study; (3) planned change to daily diet and/or exercise habits and living environment (e.g., moving house or relocating for work) during the planned study period; (4) extremely irregular meal timings; (5) excessive alcohol intake (mean daily consumption ≥60 g); (6) smoking (mean daily consumption ≥ two packs); (7) positive hepatitis C virus antibody or hepatitis B surface antigen test; (8) drug or food allergies (in particular soybeans and gelatin); (9) recent (past 4 weeks), current, or planned participation in another clinical study during the study period; (10) pregnancy or breastfeeding in women or planned pregnancy during the study period; (11) history of or current treatment for a severe disease; (12) blood donation of 200 mL in the past month; (13) if male, blood donation of 400 mL in the past 3 months; (14) if female, blood donation of 400 mL in the past 4 months; (15) if male, total blood donation of more than 1,200 mL, calculated as the sum of donations in the past year and the estimated sampling amount in the study; (16) if female, total blood donation of more than 800 mL, calculated as the sum of donations in the past year and the estimated sampling amount in the study; and (17) deemed unsuitable for the study by the study investigators.
Of the 431 screened participants, 110 were found to be suitable for inclusion and enrolled. Participants were randomly allocated to the study groups by a stratified randomization method that considered sex, age, BMI, fasting and postprandial glucose, and high-sensitivity CRP (hsCRP) level (CLE group, n = 55; placebo group, n = 55). The authors randomly assigned numbers to the CLE and placebo capsules, and the CRO did the same for the participants; the randomization lists were kept in a secure location until locking of the database. All participants and study investigators were blinded to the group allocation.
2.2.3 Experimental foods
Table 1 details the test capsule composition. CLE and placebo base capsules were composed of gelatin, glycerin, soybean-derived emulsifier, and beeswax. The CLE was the same as that tested in the RAW264.7 macrophages (see Section 2.1). The coloring agents carob and tartrazine were added to placebo capsules to give them the same appearance as CLE capsules.
2.2.4 Intervention
The intervention period was from September to December 2021. During the 12-week study, participants ingested two CLE or placebo capsules/day. Study visits were performed at 0, 4, 8, and 12 weeks and consisted of an interview with an experienced physician, physical assessments, and an OGTT. At the same visits, hematology and biochemistry tests and urinalysis were performed by a contracted laboratory company (BML, Inc., Tokyo, Japan). Before study visits, participants were allowed to consume only water from 9:00 pm on the evening before.
During the study, participants completed a daily diary with information on disease occurrence and symptoms; consumption of test capsules, nutraceutical/functional foods, and drugs (reasons for taking them, dose, and length of use); vaccinations (reasons for vaccination, date of vaccination, and adverse effects); exercise (exercise or no exercise, days of exercise, duration of exercise); any dietary carbohydrate restriction or dieting to lose weight (duration of dietary carbohydrate restriction/dieting); sleep duration (sleeping hours); and change of living environment. All participants were instructed to comply with the following study rules throughout the 12 weeks: (1) maintain usual lifestyle, including dietary, exercise, alcohol use, and smoking habits and use of medicine; (2) avoid consuming nutraceutical/functional foods (excluding study capsules); (3) do not donate blood; and (4) do not drink excessive amounts of alcohol or smoke a large number of cigarettes, do not perform extreme amounts of exercise, and do not fast or consume an unbalanced diet.
2.2.5 Measurement of blood parameters
The hsCRP serum level and HbA1c were measured by standard latex agglutination turbidimetry, plasma glucose was measured by the hexokinase method, and serum insulin was measured with chemiluminescent immunoassay.
2.2.6 The OGTT procedure
After a blood sample was obtained for measurement of fasting glucose and insulin levels, participants ingested a solution containing 75 g of glucose. Blood sampling was conducted after 30, 60, and 120 min for measurement of post-load blood glucose and insulin levels, and the areas under the curve (AUCs), times to peak concentration, insulinogenic indices, and insulin sensitivity indices were calculated.
2.2.7 Calculation of insulinogenic index
The insulinogenic index was used to evaluate insulin secretory capacity in the first phase (within 30 min) after glucose load. It was calculated according to the following formula: insulinogenic index = [insulin 30 min – insulin 0 min]/[glucose 30 min – glucose 0 min]. A low score for this index indicates impaired insulin secretion capacity and is also used as a predictor of type 2 diabetes (51).
2.2.8 Calculation of insulin sensitivity indices
Both the Cederholm index and the Stumvoll index, which are calculated from the OGTT values of glucose and insulin, were used as indices of estimated insulin sensitivity. These indices are highly correlated with the results of an insulin clamp test, which can accurately quantify insulin sensitivity but is not suitable for large-scale studies because it is laborious and expensive (52). These indices were calculated according to the following formula: Cederholm index = [75,000 + (glucose 0 min – glucose 120 min) × 1.15 × 180 × 0.19 × body weight]/[(120 × mean glucose 0–120 min) × log (mean insulin 0–120 min)]; Stumvoll index = 0.156–0.0000459 × insulin 120 min – 0.000321 × insulin 0 min – 0.00541 × glucose 120 min. Low scores for these indices indicate reduced insulin sensitivity and are reported to be associated with an increased risk of type 2 diabetes (53). Previous intervention studies showed that anti-diabetic drugs and functional foods improve not only the scores of the Cederholm and Stumvoll indices but also postprandial glucose levels (54, 55).
2.2.9 Sample size
To calculate the minimum sample size necessary to ensure adequate statistical power, we used the G Power 3.1.9 program (University of Dusseldorf, Germany) and results of a previous clinical study. In that study, an aqueous extract of Adansonia digitata L., which is known to have anti-inflammatory activities (56), reduced the change in postprandial glucose levels from 0 min to 60 and 120 min after glucose load by at least 25% compared with the placebo group (57). The required sample size was calculated to be at least 39 participants per group based on the following assumption: 25% reduction in post-load glucose by CLE, Cohen’s d value of 0.65, statistical power of 80%, and type 1 error of 5%. Some previous clinical studies that used OGTT estimated the required sample size to be 50 to 55 participants per group, assuming a maximum dropout rate of 20% (58, 59). Therefore, in the present study, the sample size was determined to be 55 participants per group.
2.2.10 Statistical analysis
Data from all randomized participants, i.e., the intention-to-treat (ITT) population, were included in the statistical analysis. Safety was evaluated in the full analysis set (FAS), and efficacy was analyzed in the per-protocol set (PPS); the PPS did not include participants who missed any study visits. The IBM SPSS statistical software package (version 26) for Windows (IBM Corp., Armonk, NY, USA) was used for all statistical analyses. Results are shown as mean (SD). Intergroup comparisons of baseline characteristics were performed with the two-tailed unpaired Student’s t test in case of homogeneous variance and the Aspin-Welch t test in case of heterogeneous variance, whereby sex and urinalysis were analyzed by a two-tailed Mann–Whitney U test. To analyze changes from baseline, we used repeated measures two-way ANOVA (two groups × three-time points) and an SPSS general linear model to determine the main effects of group, time, and group × time; then, we used simple main effect tests to compare groups at each visit (22, 60–62). The statistical significance level was set at 0.05.
3 Results
3.1 Non-clinical study
3.1.1 Effects of CLE and its potential active components on the production of inflammatory mediators in RAW264.7 macrophage cells stimulated with LPS
LPS stimulation markedly increased the production of IL-1β, IL-6, and NO in LPS-stimulated RAW264.7 cells compared with unstimulated control cells, but the production of these substances was significantly inhibited by pretreatment of cells with CLE and turmeronol A, turmeronol B, and bisacurone at the concentrations contained in the CLE (Supplementary Figure S1). LPS stimulation also increased TNF-α protein production, but the increase was significantly inhibited by pretreatment of cells with CLE and all test compounds except turmeronol B at the concentrations contained in the CLE (Supplementary Figure S1).
3.2 Clinical intervention study
3.2.1 Participants
The flowchart of participants in the study is shown in Figure 2. A total of 110 out of 431 potential participants were randomly allocated to the CLE or placebo group (n = 55 per group). One participant in the placebo group declined to continue for personal reasons. In addition, some participants (n = 12 in the placebo group and n = 16 in the CLE group) were excluded from the efficacy assessment (PPS analysis) because they did not comply with the protocol for the following reasons: use of health foods (n = 3 in the placebo group and n = 1 in the CLE group), medical treatment of chronic diseases (n = 1 in the placebo group [lumbar spondylosis] and n = 3 in the CLE group [hypertension, hyperuricemia, and adipoma]), change of lifestyle during the scheduled study period (n = 2 in the CLE group), irregular dietary habits (n = 1 in the placebo group), and unsuitable for the study for other reasons (unreliable study results because of hemolysis during blood collection [n = 1 in the CLE group], symptoms with acute inflammation [n = 1 in the placebo group due to a common cold and n = 1 in the CLE group due to injuries], excessive exercise before visiting the study center [n = 1 in the placebo group and n = 1 in the CLE group], and suspected adverse effects of the Covid-19 vaccine (63) [n = 5 in the placebo group and n = 7 in the CLE group]). The other 81 participants were included in the PPS analysis.
The baseline characteristics were not significantly different between the two groups, but the Cederholm index was significantly higher in the CLE group (Tables 2, 3). The mean intake of test capsules was also not significantly different between the two groups (data not shown). During the study period, some participants exercised (n = 22 in the placebo group and n = 17 in the CLE group) and some did not (n = 20 in the placebo group and n = 22 in the CLE group); the number of participants who exercised did not differ significantly between the two groups (p = 0.507). In addition, the mean (SD) number of days of exercise was not significantly different between the two groups (placebo group, 11.0 [17.6] days; CLE group, 9.3 [17.7] days; p = 0.666).
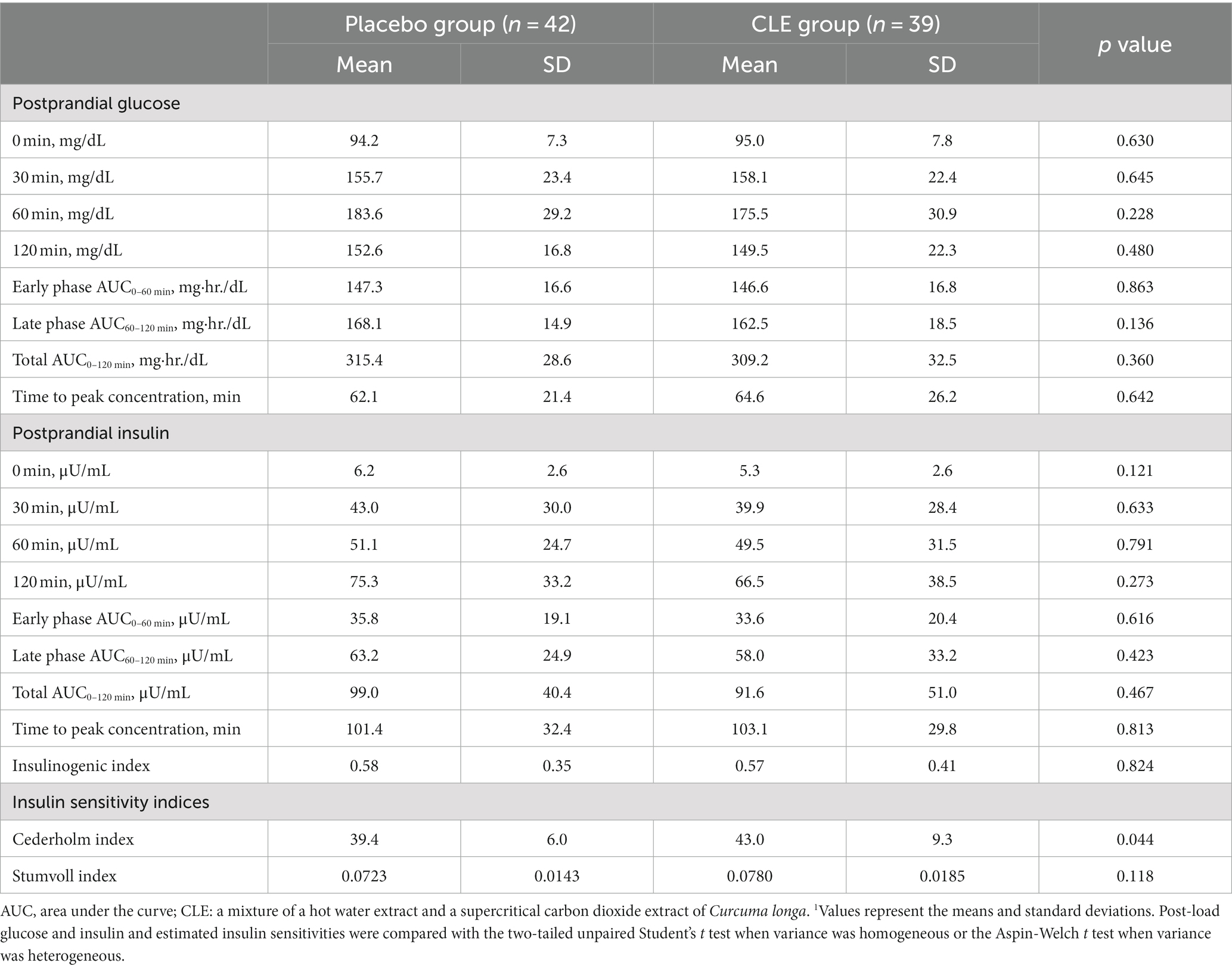
Table 3. Baseline values for post-load glucose and insulin, insulinogenic index, and insulin sensitivity indices in the oral glucose tolerance test (OGTT)1.
3.2.2 Effect of CLE on body weight and BMI
During the entire study period, the change in body weight from week 0 showed no significant differences between the two groups (repeated measures two-way ANOVA [r-ANOVA]), but in week 4, it was significantly higher in the CLE group than in the placebo group. The change in BMI from week 0 was also not significantly different between the two groups throughout the whole study period (r-ANOVA) but was significantly higher in the CLE group than in the placebo group in week 4. The change in body weight and BMI from week 0 showed no significant differences between the two groups at weeks 8 and 12 (data not shown).
3.2.3 Effect of CLE on serum hsCRP level
The change in hsCRP from week 0 showed no significant differences between the two groups throughout the whole study period (r-ANOVA), but it was significantly lower in the CLE group than in the placebo group at weeks 4 and 12 (Table 4).
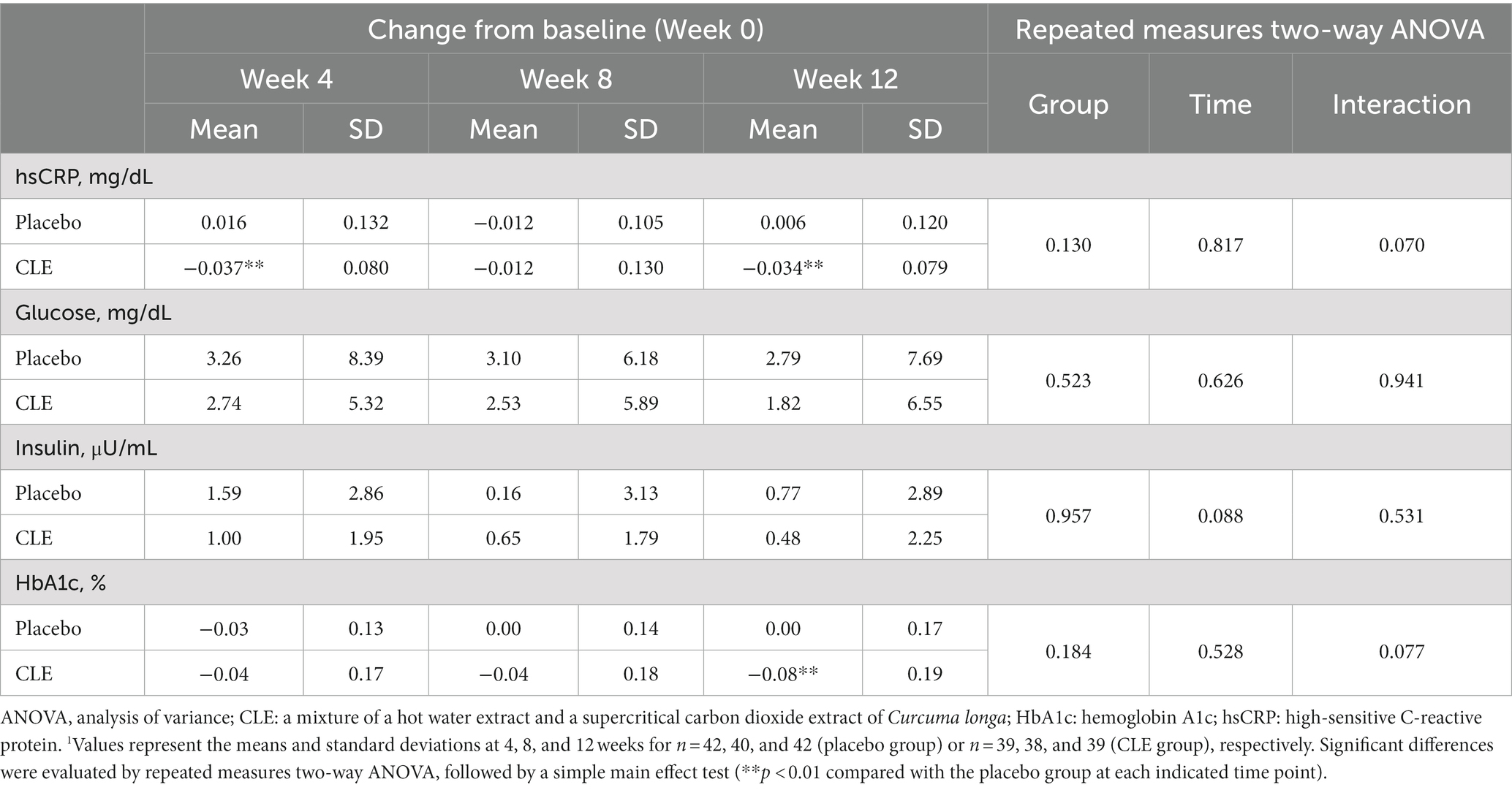
Table 4. Effect of Curcuma longa extract (CLE) on the change of fasting blood markers of systemic inflammation and glucose metabolism1.
3.2.4 Effect of CLE on fasting glucose, insulin, and HbA1c levels
The changes in fasting plasma glucose and serum insulin from week 0 were not significantly different between the two groups (Table 4). Although the change in HbA1c from week 0 showed no significant differences between the two groups throughout the whole study period (r-ANOVA), at week 12, it was significantly lower in the CLE group than in the placebo group (Table 4).
3.2.5 Effect of CLE on post-load glucose and insulin in OGTT
3.2.5.1 Week 0
The changes in glucose and insulin from 0 min were not significantly different between the two groups (Table 5). The change in insulin from 0 min showed no significant differences between the two groups throughout the OGTT (r-ANOVA), but at 120 min it tended to be lower in the CLE group than in the placebo group (p = 0.093) (Table 5).
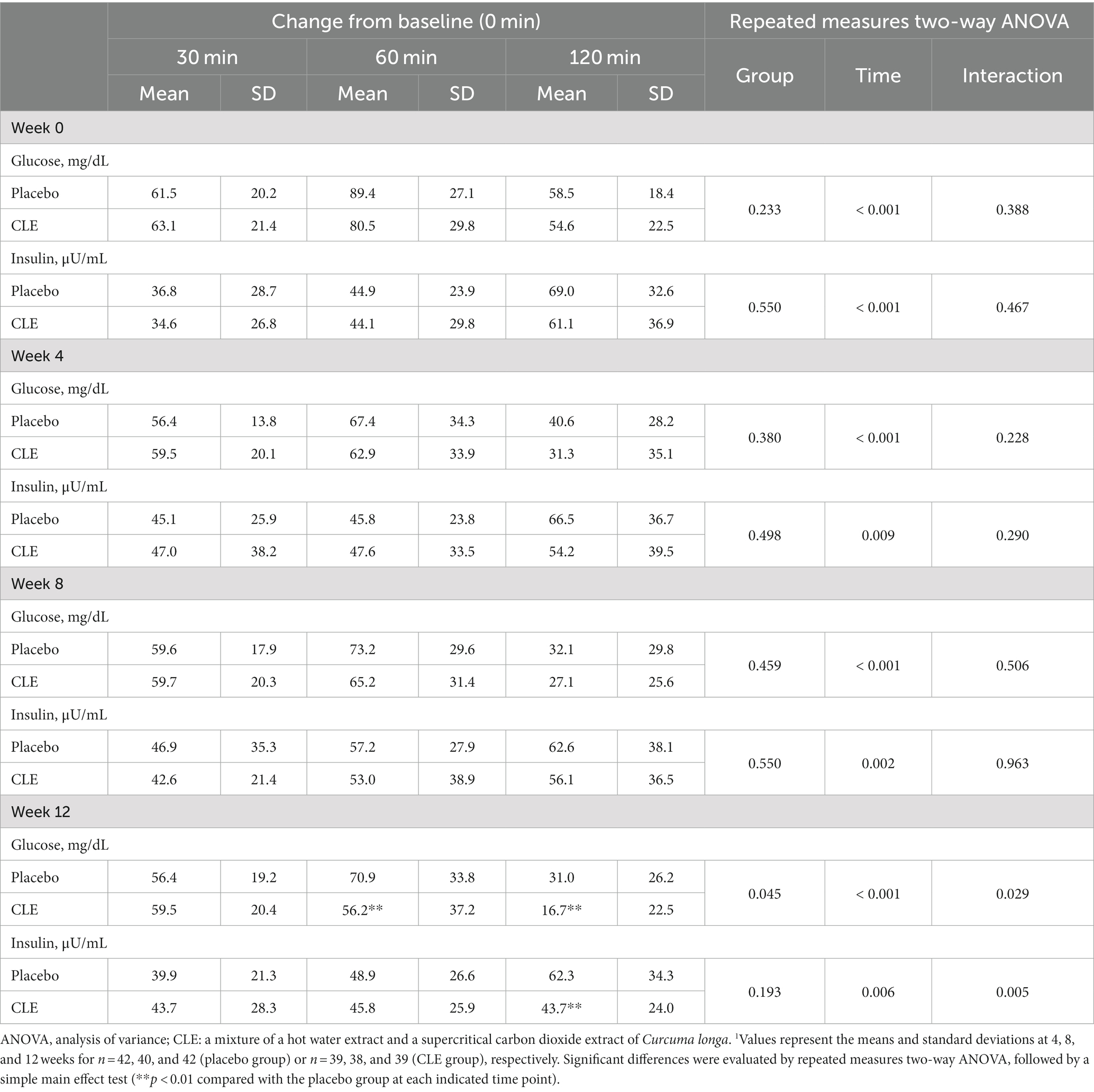
Table 5. Effect of Curcuma longa extract (CLE) on the change of post-load glucose and insulin levels during the oral glucose tolerance test (OGTT)1.
3.2.5.2 Week 4
At week 4, the change in glucose from 0 min showed no significant differences between the two groups throughout the OGTT (r-ANOVA), but it tended to be lower in the CLE group than in the placebo group at 120 min (p = 0.078) (Table 5). The change in insulin from 0 min was not significantly different between the two groups throughout the OGTT (r-ANOVA), but it tended to be lower in the CLE group than in the placebo group at 120 min (p = 0.084) (Table 5).
3.2.5.3 Week 8
At week 8, the changes in glucose and insulin from 0 min showed no significant differences between the two groups (Table 5).
3.2.5.4 Week 12
At week 12, the change in glucose from 0 min was significantly lower in the CLE group than in the placebo group throughout the OGTT (r-ANOVA) and at 60 min and 120 min (Table 5; Figure 3). The change in insulin from 0 min showed no significant differences between the two groups throughout the OGTT (r-ANOVA), but it was significantly lower in the CLE group than in the placebo group at 120 min (Table 5; Figure 3).
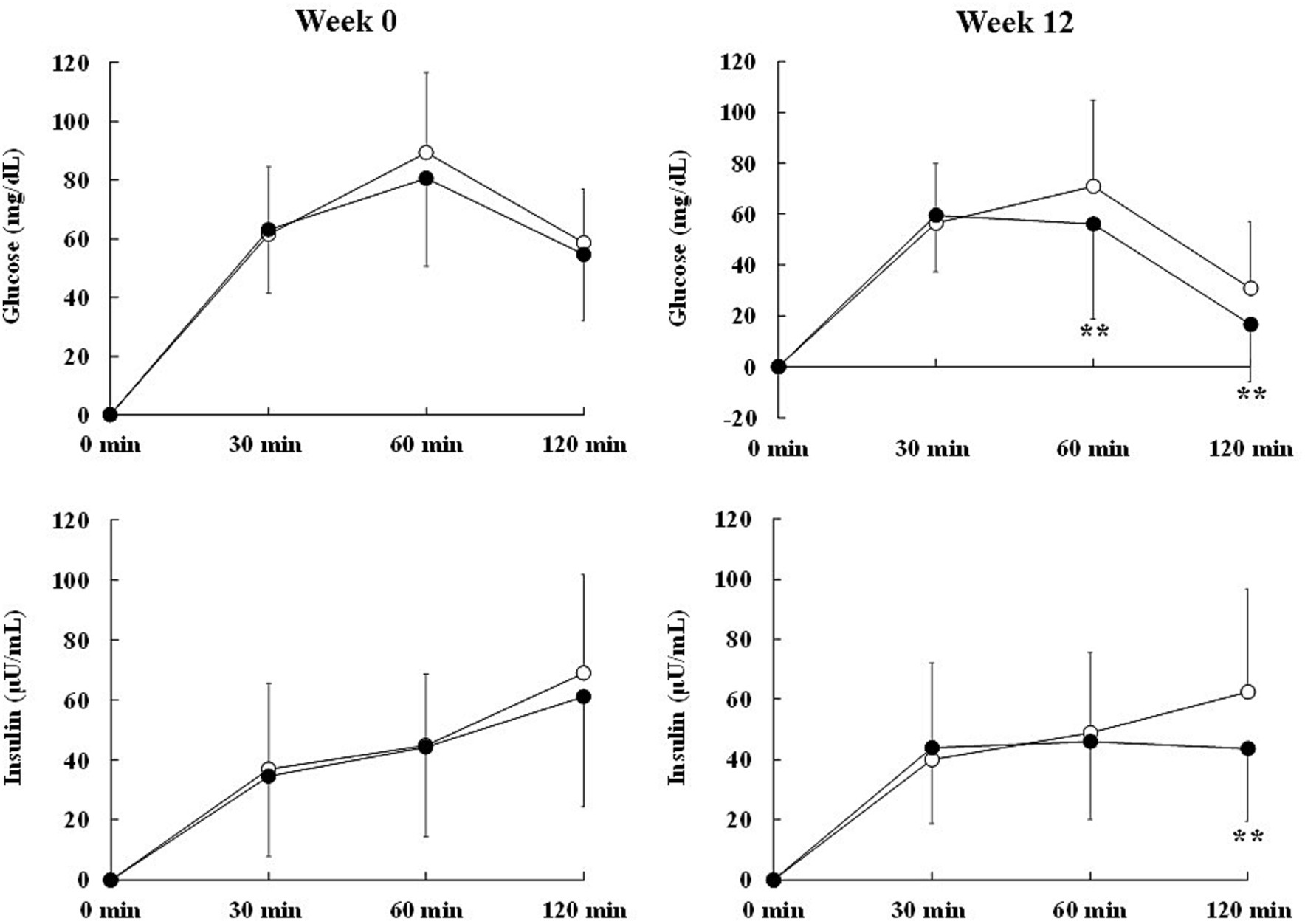
Figure 3. Effect of Curcuma longa extract (CLE) on the change in marker levels of post-load glycometabolism during the oral glucose tolerance test (OGTT) in middle-aged and elderly participants with overweight and glycemia in the normal/prediabetes range. Glucose and insulin levels after oral glucose load in the OGTT were measured at baseline and after 12 weeks of consuming test capsules containing CLE (CLE group; black circle, n = 39) or placebo (placebo group; white circle, n = 42). Data are means and standard deviations. Mean values in the CLE group were significantly different from those in the placebo group. **p < 0.01 (repeated measures two-way analysis of variance, followed by a simple main effect test). CLE, a mixture of a hot water extract and a supercritical carbon dioxide extract of Curcuma longa; OGTT, oral glucose tolerance test.
3.2.6 Effect of CLE on post-load values at 120 min and AUC of glucose and insulin during the OGTT
The change from week 0 in post-load glucose at 120 min showed no significant differences between the two groups throughout the OGTT (r-ANOVA), but it was significantly lower in the CLE group than in the placebo group at week 12 (Table 6). The change from week 0 in post-load insulin at 120 min showed no significant differences between the two groups throughout the OGTT (r-ANOVA), but it tended to be lower in the CLE group than in the placebo group at week 12 (p = 0.097) (Table 6). The changes from week 0 in the AUC of early-phase glucose and insulin in the first hour after administration of oral glucose (AUC0–60 min) showed no significant differences between the two groups (Table 6). The change from week 0 in the AUC of late-phase glucose (AUC60–120 min) was not significantly different between the two groups throughout the OGTT (r-ANOVA) but was significantly lower in the CLE group than in the placebo group at week 12 (Table 6), and the change from week 0 in the AUC of late-phase insulin (AUC60–120 min) showed no significant differences between the two groups throughout the OGTT (r-ANOVA) but tended to be lower in the CLE group than in the placebo group at week 12 (p = 0.066) (Table 6). The change from week 0 in the AUC of total glucose (AUC0–120 min) showed no significant differences between the two groups throughout the OGTT (r-ANOVA) but tended to be lower in the CLE group than in the placebo group at week 12 (p = 0.079) (Table 6), and the change from week 0 in the AUC of total insulin (AUC0–120 min) showed no significant differences between the two groups (Table 6).
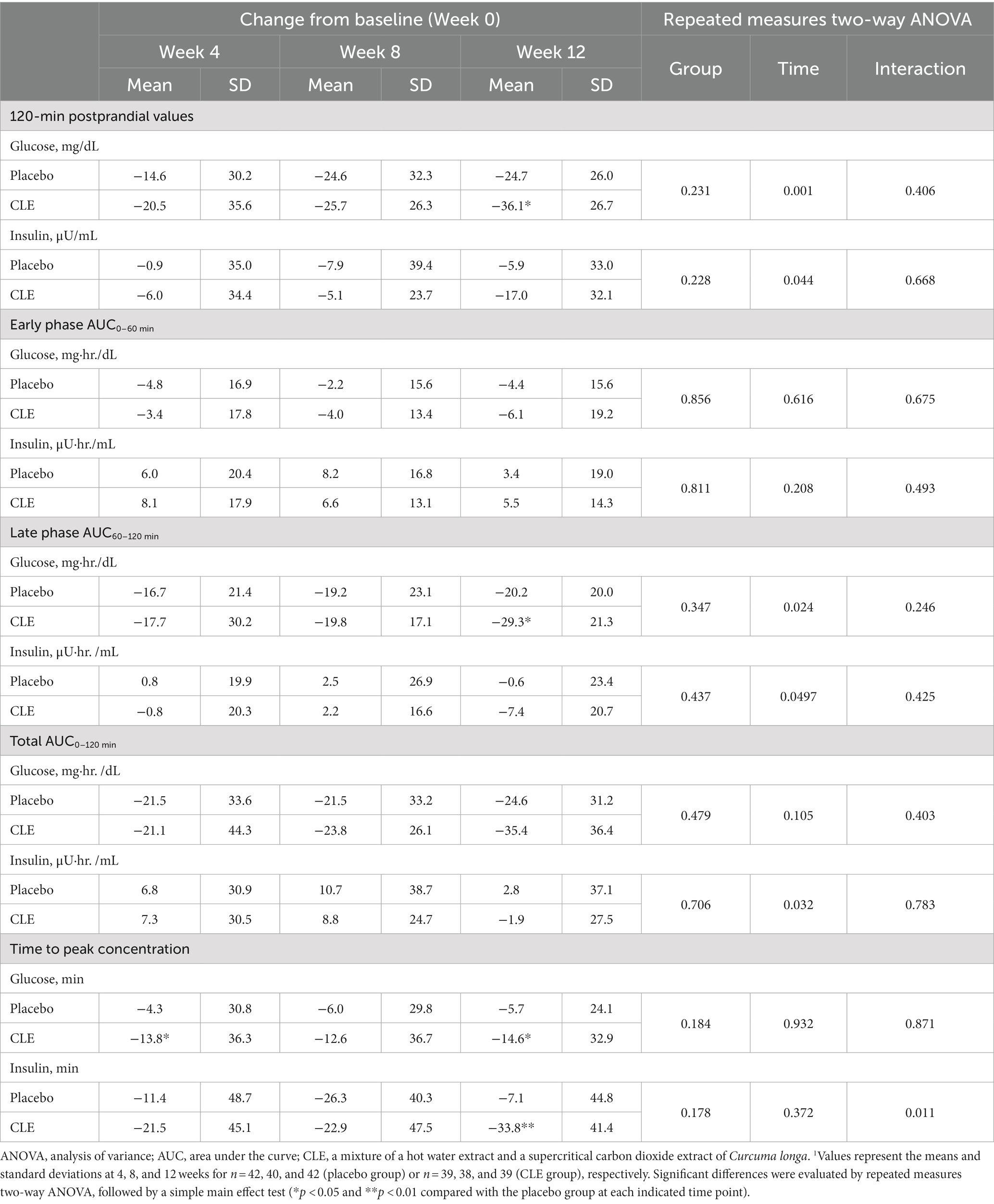
Table 6. Effect of Curcuma longa extract (CLE) on the change in 120-min post-load glucose and insulin values, area under the curve (AUC), and time to peak concentrations of glucose and insulin in the oral glucose tolerance test (OGTT)1.
3.2.7 Effect of CLE on time to peak concentration of glucose and insulin during the OGTT
The change from week 0 in the time to peak concentration of glucose showed no significant differences between the two groups throughout the whole study period (r-ANOVA) but was significantly lower in the CLE group than in the placebo group at weeks 4 and 12 (Table 6). The change from week 0 in the time to peak concentration of insulin showed no significant differences between the two groups throughout the whole study period (r-ANOVA) but was significantly lower in the CLE group than in the placebo group at week 12 (Table 6).
3.2.8 Effect of CLE on insulinogenic index
The changes from week 0 in the insulinogenic index were not significantly different between the two groups (Table 7).
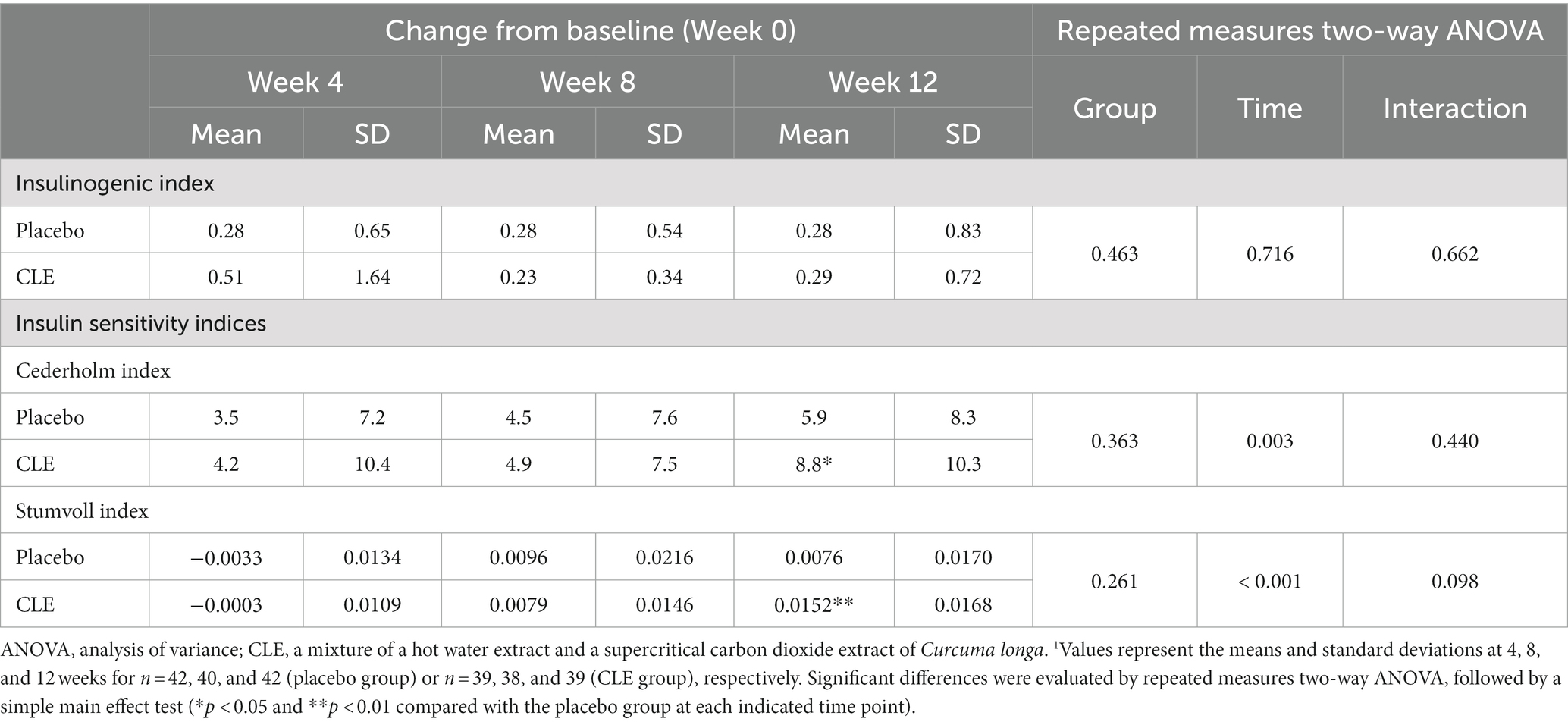
Table 7. Effect of Curcuma longa extract (CLE) on the change of insulinogenic indexes and insulin sensitivity indices1.
3.2.9 Effect of CLE on insulin sensitivity indices
The Cederholm index showed no significant differences in the change from week 0 between the two groups throughout the whole study period (r-ANOVA), but the change was significantly higher in the CLE group than in the placebo group at week 12 (Table 7). Similarly, the Stumvoll index showed no significant differences in the change from week 0 between the two groups throughout the whole study period (r-ANOVA), but the change was significantly higher in the CLE group than in the placebo group at week 12 (Table 7).
3.2.10 Safety of the intervention
Adverse events were assessed in the ITT population (placebo group, n = 55; CLE group, n = 55). In the placebo group, the following adverse events occurred: headache and shoulder pain (one case), toothache (one case), pharyngeal pain (two cases), arm pain (one case), abdomen pruritus (one case), stomach upset and diarrhea (one case), colon polyp (one case), common cold symptoms (seven cases), rhinitis (one case), diarrhea (one case), insect bite (one case), lumbar spondylosis (one case), increased uric acid (one case), positive urinary protein (four cases), positive urinary blood (one case), increased triglyceride (two cases), increased creatine phosphokinase (three cases), increased aspartate aminotransferase (one case), and increased body weight (five cases). In the CLE group, the following adverse events occurred: headache (three cases), eye pain (one case), bloodshot eyes (one case), infective conjunctivitis (one case), tooth decay (one case), bleeding and swelling of the gums (one case), pharyngeal pain (one case), shoulder adipoma (one case), fever and shoulder pain (two cases), fever and arm pain (one case), arm pain (one case), elbow bruise (one case), low back pain (one case), fatigue (one case), common cold symptoms (nine cases), diarrhea (one case), heat rash (one case), verruca vulgaris (one case), helicobacter pylori infection (one case), high blood pressure (one case), positive urinary glucose (one case), increased uric acid (one case), increased triglyceride (one case), increased creatine phosphokinase (three cases), increased CRP (two cases), increased alanine transaminase (one case), increased body weight (two cases), and decreased body weight (two cases). These adverse events were mild, and an experienced physician judged that they were unrelated to the dietary intervention.
Safety parameters (hematology and biochemistry tests, urinalysis, and physical measurements and tests) were assessed in the FAS population (placebo group, n = 54; CLE group, n = 55). The urinalysis and physical measurements were not significantly different between the two groups. The number of participants with reduced kidney function, defined as an estimated glomerular filtration rate (eGFR) below 60 mL/min/1.73 m2, was not significantly different between the CLE group (n = 5) and placebo group (n = 5) at week 0 but was significantly lower in the CLE group (n = 3) than in the placebo group (n = 10) at week 12 (chi-squared tests). The number of red blood cells was significantly lower in the CLE group than in the placebo group at weeks 4 and 8; the level of uric acid was significantly lower in the CLE group than in the placebo in week 8; and the level of total bilirubin at weeks 4 and 8 and chloride at week 4 was significantly higher in CLE group than in the placebo group. However, these changes were within the corresponding reference ranges.
4 Discussion
We investigated the effect of a mixture of a hot water extract and a supercritical carbon dioxide extract of C. longa (CLE) and its potential active ingredients (turmeronols A and B and bisacurone) on the production of inflammatory mediators in RAW264.7 macrophage cells stimulated with LPS. In addition, we performed a 12-week, randomized, double-blind, placebo-controlled study to investigate the effect of CLE on chronic inflammation and postprandial hyperglycemia in middle-aged and elderly participants with overweight and glycemia in the normal/prediabetes range. In the in vitro study, CLE, turmeronol A, and bisacurone significantly inhibited the production of IL-1β, IL-6, TNF-α, and NO in RAW264.7 cells stimulated with LPS, and turmeronol B significantly inhibited LPS-induced production of IL-1β, IL-6, and NO. In the clinical study, we found that intake of CLE significantly reduced serum hsCRP and HbA1c levels. Furthermore, in the OGTT, post-load plasma glucose level, time to peak concentration of glucose and insulin, and insulin sensitivity indices were significantly improved in the CLE group. These results suggest that CLE may improve insulin sensitivity and postprandial hyperglycemia by reducing chronic inflammation and that this reduction is due at least partly to the anti-inflammatory effects of turmeronols A and B and bisacurone.
Chronic inflammation is induced by several triggers, such as aging, accumulation of visceral fat, and unhealthy lifestyle. It is sustained by inflammatory mediators produced by inflammatory cells such as macrophages and is involved in the development of metabolic abnormalities and various inflammatory diseases (1). In the present study, CLE and its potential active compounds, i.e., turmeronols A and B and bisacurone, inhibited the production of inflammatory mediators in activated macrophages stimulated with LPS (Supplementary Figure S1). Considering that 600 μg/mL CLE contains 1.1 μM turmeronol A, 1.1 μM turmeronol B, and 4.2 μM bisacurone and that the sum of anti-inflammatory activity of the three potential active compounds appears to be comparable to that of CLE, all the anti-inflammatory effects of CLE may be attributable to the turmeronols and bisacurone. In previous animal studies, a hot water extract of C. longa containing turmeronols A and B and bisacurone (22) not only inhibited the mRNA expression of inflammatory mediators, including IL-1β, IL-6, TNF-α, and inducible NO synthase (iNOS), but also ameliorated various inflammatory pathologies in mouse models, such as ethanol-induced liver injury and non-alcoholic steatohepatitis (36). As mentioned above, CRP is generally used as a systemic inflammatory marker in human clinical studies. Recently, it was reported that the hsCRP assay, which can detect small increases in CRP levels, can be used as a marker of low-grade inflammation (5, 7). Epidemiological studies have reported that slightly higher hsCRP (approximately 0.1 mg/dL) is associated with an increased risk of various inflammatory diseases, including metabolic syndrome (8), diabetes (9), coronary heart disease (11), and cancer (12). The risk of these diseases may be decreased by anti-inflammatory drugs (64–66). In a previous intervention study, C. longa extracts containing turmeronols and bisacurone were shown to decrease the level of systemic inflammatory markers, such as hsCRP, TNF-α, IL-6, and soluble vascular cell adhesion molecule-1, and to improve health-related quality of life (22, 23). In the present study, we also confirmed that CLE significantly reduces the serum level of hsCRP (Table 4). These results suggest that CLE, which contains turmeronols A and B and bisacurone, may reduce chronic low-grade inflammation and thus may have the potential to reduce the risk of chronic inflammatory diseases.
Generally, systemic glucose tolerance is evaluated with an OGTT. The 2-h OGTT glucose value is needed for diagnosing the presence of prediabetes and diabetes, and an elevated 2-h post-load glucose is known to be associated with an increased risk of diabetes (50). Individuals with prediabetes were reported to exhibit increased plasma levels of glucose during the OGTT before showing increases in fasting blood glucose levels (67). A higher postprandial glucose level is an early sign of prediabetes and is associated with an increased risk of type 2 diabetes (15, 68). Impaired postprandial glucose metabolism is associated with chronic inflammation (69). For example, the administration of clodronate liposome, an agent that selectively depletes systemic macrophages, was shown to reduce systemic inflammation and improve postprandial glucose levels in dietary-induced obese mouse models (70, 71). Human studies reported that hsCRP levels were more strongly positively correlated with postprandial than with fasting blood glucose levels (69). Furthermore, a clinical intervention study showed that salsalate, an anti-inflammatory drug, improved hsCRP and postprandial glucose levels (72). In addition, large-scale observation studies found that people taking anti-inflammatory drugs such as aspirin have a lower risk of type 2 diabetes than people not taking such drugs (73). In the present study, CLE not only significantly inhibited the elevation of post-load glucose levels immediately after oral glucose load but also improved the level of post-load glucose 2 h later (Tables 5, 6). The CLE group also had a significantly shorter time to peak glucose concentration than the placebo group (Table 6). Previous clinical studies found that after glucose ingestion, insulin levels decreased once blood glucose had returned to baseline (51, 74, 75). In line with these studies, in the present study, we also observed that CLE significantly decreased the level of post-load insulin at 120 min after a reduction in glucose level at 60 min (Table 5). Taken together, these findings suggest that CLE may reduce the postprandial elevation of glucose level and promote postprandial glucose clearance and, consequently, may be able to decrease the risk of prediabetes and type 2 diabetes.
The regulation of postprandial glycometabolism is involved in various processes, such as intestinal glucose absorption, insulin secretion by the pancreas, and insulin resistance (14, 76). In the gastrointestinal tract, dietary carbohydrates are digested by enzymes such as α-glucosidase into monosaccharides, which are absorbed in the small intestine (76). Acarbose, an α-glucosidase inhibitor that suppresses the intestinal absorption of glucose, is known to inhibit the elevation of postprandial glucose in the early phase after oral carbohydrate load (within 30 min) (77, 78), and a clinical study found that the reduction in the insulin secretory response to oral glucose load increases postprandial glucose levels (74). An insulinogenic index can be used as a marker of early-phase insulin secretory capacity associated with β-cell function (51), and the present study found no significant differences between the placebo and CLE groups in post-load glucose levels in the early phase and in the insulinogenic index (Tables 5, 7). Therefore, CLE may not have the potential to inhibit the intestinal absorption of glucose or to enhance insulin secretion by the pancreas.
Insulin resistance, also known as impaired insulin sensitivity, refers to impaired effects of insulin, such as reduced glucose uptake in insulin-sensitive tissues, including skeletal muscles, adipose tissues, and liver (14). In the fasting state, glucose uptake occurs mainly in non–insulin-sensitive tissues such as the brain, whereas in the postprandial state, approximately 80% of total body glucose uptake occurs in skeletal muscles (14, 79). Thus, skeletal muscle is the main tissue responsible for postprandial glucose uptake and glucose disposal (14, 80). However, the expression levels of phosphorylated proteins associated with insulin-mediated glucose uptake are lower in skeletal muscle tissues from obese participants than in those from lean participants (81). In fact, studies found that the rate of glucose uptake is 50% lower in the skeletal muscle of obese participants with impaired glucose tolerance (14, 82). In addition, previous clinical studies showed that in the OGTT, participants with impaired glucose tolerance are characterized by a significant increase in post-load glucose levels in the late phase (60 to 120 min) but not by a reduction of insulin secretion in the early phase (75, 83). In another clinical study, a stratified analysis showed that the level of postprandial glucose was significantly higher in overweight participants with a high CRP level than in those with a low CRP level but that it had no effect on the insulin secretory response after oral glucose load (17). Intervention studies found that in the OGTT, the level of late-phase glucose (AUC60–120 min) was improved by food ingredients such as 5-aminolevulinic acid that inhibit production of inflammatory mediators in RAW264.7 macrophage cells stimulated with LPS (84, 85). In addition, anti-inflammatory agents such as salsalate and rosiglitazone significantly improved insulin sensitivity and postprandial glucose levels in patients with obesity or diabetes, whereas the insulin response after oral glucose loading was not significantly different between the agent group and the placebo group (72, 86). Treatment with a water extract of C. longa increased glucose uptake in mouse skeletal muscle tissues under hyperglycemic culture conditions (87), and in the present study, CLE significantly improved post-load glucose levels and glucose AUC in the late phase (60 to 120 min) in the OGTT. Although no significant differences between the two groups were observed in early insulin response after oral glucose load, the Cederholm and Stumvoll insulin sensitivity indices both significantly improved in the CLE group (Table 7). Recently, insulin sensitivity was reported to influence the shape of the post-load glucose and insulin curves in the OGTT; impaired insulin sensitivity slows the onset of insulin action, such as glucose uptake, and causes a delay in the time until peak glucose and insulin concentrations in the OGTT (74, 88). In the present study, during the OGTT, the time to peak concentrations of glucose and insulin were both significantly shorter in the CLE group than in the placebo group (Table 6). These results suggest that CLE could potentially increase postprandial glucose uptake in skeletal muscles by improving impaired insulin sensitivity associated with chronic inflammation.
HbA1c represents the percentage of circulating glycated hemoglobin and is an indicator of average blood glucose levels over the past one to three months (67, 89). The HbA1c value is known to reflect not only elevated blood levels of fasting glucose but also hyperglycemia after daily meals such as breakfast, lunch, and dinner (90). Additionally, a clinical study found that in participants with an HbA1c level below 6.2%, approximately 70 to 90% of the total HbA1c value is attributable to postprandial hyperglycemia (89, 90). In an intervention study, anti-inflammatory drugs significantly reduced HbA1c and postprandial glucose levels (86, 91). Similar to these reports, in the present study, CLE also significantly improved post-load glucose and HbA1c levels (Tables 4, 5). These results suggest that CLE may help to decrease the blood level of HbA1c by suppressing the elevation of glucose levels after a meal.
Insulin, a polypeptide hormone that binds to the insulin receptor, causes phosphorylation of the insulin receptor substrate-1 (IRS-1) at tyrosine residues, activates phosphoinositide 3-kinase (PI3K)/protein kinase B (Akt) signaling pathways, and promotes the translocation of glucose transporter-4 from the cytoplasm into the cell membrane, where it induces glucose uptake into cells (92). The development of insulin resistance is caused by inflammation associated with macrophages, which are activated by recognition of factors such as free fatty acids and damage-associated molecular patterns (1, 5). Activated macrophages abundantly produce inflammatory mediators, which inhibit the insulin signaling pathway (6). As mentioned above, in obese mouse models, selectively eliminating macrophages by administering clodronate liposome reduced macrophage-induced systemic inflammation and consequently improved not only postprandial glucose levels but also insulin resistance (70, 71). Other studies showed that treatment with IL-1β or TNF-α inhibits insulin-induced tyrosine phosphorylation of IRS-1; increases serine/threonine phosphorylation of IRS-1, which can block insulin signaling; and impairs glucose uptake in response to insulin in various cells, such as skeletal muscle cells (93–96). A genetic deficiency of IL-1 or TNF receptors was reported to suppress the development of insulin resistance in diet-induced obesity mouse models (97, 98), and in healthy participants, intravenous infusion of TNF-α was reported to decrease whole-body glucose uptake in insulin clamp tests (99). In a double-blind study, an IL-1 antibody improved postprandial glucose levels (91). IL-6 also reduces the expression of IRS-1 and glucose transporter-4 (100), and administration of IL-6–neutralizing antibodies improved insulin resistance in IkB kinase-β transgenic mice with constitutively activated signaling of NF-k Band hyperglycemia (98). NO interacts with superoxide to form peroxynitrite, which inhibits insulin signaling by s-nitrosylation or nitration of IRS-1, PI3K, and/or Akt (101). Furthermore, inhibition of iNOS or NF-kB decreases insulin resistance in leptin-deficient obese mouse models (100, 102). In the present study, turmeronols A and B and bisacurone significantly inhibited LPS-induced production of these inflammatory mediators in activated macrophages (Supplementary Figure S1). In addition, laboratory and animal studies showed that turmeronols A and B and bisacurone inhibit phosphorylation and nuclear translocation of NF-kB and suppress the mRNA expression of its target genes, including genes encoding IL-1β, IL-6, TNF-α, and iNOS, in various LPS-stimulated cells, including macrophages (24, 45, 103). Bisacurone was also shown to increase the protein expression of peroxisome proliferator-activated receptor alpha, which has anti-inflammatory effects by inhibiting NF-kB activation (104). Therefore, it appears that turmeronols A and B and bisacurone may prevent the production of inflammatory mediators in macrophages by inhibiting the activation of NF-kB and thus improve the exacerbation of insulin signaling associated with chronic inflammation. However, further research is needed to clarify the effects of turmeronols A and B and bisacurone in humans.
5 Conclusion
We investigated the effects of CLE and its potential active ingredients turmeronol A, turmeronol B, and bisacurone on chronic inflammation and postprandial glycometabolism. First, we assessed the inhibitory effects on the production of inflammatory mediators in RAW264.7 macrophage cells stimulated with LPS. Then, we performed a 12-week, randomized, double-blind, placebo-controlled study in middle-aged to elderly participants with overweight and glycemia in the normal/prediabetes range. In the in vitro study, CLE, turmeronols A and B, and bisacurone significantly inhibited the production of inflammatory mediators in RAW264.7 macrophages stimulated with LPS. In the clinical study, serum levels of hsCRP and HbA1c were significantly lower in the CLE group than in the placebo group, and in the OGTT, the post-load glucose plasma level, time to peak concentrations of glucose and insulin, and insulin sensitivity indices were also significantly improved in the CLE group. These results suggest that daily intake of CLE may have the potential to improve insulin resistance and postprandial hyperglycemia by reducing chronic low-grade inflammation.
Data availability statement
The original contributions presented in the study are included in the article/Supplementary material, further inquiries can be directed to the corresponding author.
Ethics statement
The studies involving humans were approved by the institutional review board of Chiyoda Paramedical Care Clinic (Tokyo, Japan). The studies were conducted in accordance with the local legislation and institutional requirements. The participants provided their written informed consent to participate in this study.
Author contributions
RU: Conceptualization, Data curation, Formal analysis, Investigation, Methodology, Project administration, Visualization, Writing – original draft, Writing – review & editing. CO-H: Conceptualization, Data curation, Investigation, Methodology, Visualization, Writing – original draft, Writing – review & editing. HS: Investigation, Writing – review & editing. RS: Investigation, Writing – review & editing. KM: Conceptualization, Methodology, Project administration, Supervision, Writing – review & editing. SM: Conceptualization, Methodology, Writing – review & editing. YY: Conceptualization, Methodology, Writing – review & editing. YH: Conceptualization, Methodology, Project administration, Supervision, Writing – review & editing.
Funding
The author(s) declare that no financial support was received for the research, authorship, and/or publication of this article.
Acknowledgments
The authors thank Koichi Taoka, Naohiro Mukaida, Yusuke Temmei, Takahiro Kishi, Shuya Taguchi, Yuuko Amemiya, and Yohei higashi from House Wellness Foods Corp. for their support in planning the study schedule, preparing the test capsules, and measuring potential active ingredients in CLE. The authors also thank Shukuko Ebihara from Chiyoda Paramedical Care Clinic and Makoto Ichinohe, Aiko Ishiguro, Toshiharu Namba, and Makiko Uchida from CPCC Co., Ltd. for their support in performing the study and statistical analyses. They also thank Esumi Co., Ltd. (Tokyo, Japan), a statistical consulting company, for their help with the statistical analyses and Jacquie Klesing (who was not involved in this study), Board-certified Editor in the Life Sciences (ELS), from Yamada Translation Bureau, Inc. (Osaka, Japan) for editing assistance with the manuscript.
Conflict of interest
RU, CO-H, HS, RS, KM, SM, YY, and YH are employed by House Wellness Foods Corp., which markets health food products. This study was funded by House Wellness Foods Corp. (Itami, Japan) and was conducted by a contract research organization (CRO; CPCC Co., Ltd., Tokyo, Japan). The CRO performed the data collection and analysis. The funder designed this study, supplied the tests capsules, prepared the manuscript, and decided to submit it for publication. The article processing charges were also funded by House Wellness Foods Corp.
Publisher’s note
All claims expressed in this article are solely those of the authors and do not necessarily represent those of their affiliated organizations, or those of the publisher, the editors and the reviewers. Any product that may be evaluated in this article, or claim that may be made by its manufacturer, is not guaranteed or endorsed by the publisher.
Supplementary material
The Supplementary material for this article can be found online at: https://www.frontiersin.org/articles/10.3389/fnut.2024.1324196/full#supplementary-material
References
1. Furman, D, Campisi, J, Verdin, E, Carrera-Bastos, P, Targ, S, Franceschi, C, et al. Chronic inflammation in the etiology of disease across the life span. Nat Med. (2019) 25:1822–32. doi: 10.1038/s41591-019-0675-0
2. Monteiro, R, and Azevedo, I. Chronic inflammation in obesity and the metabolic syndrome. Mediat Inflamm. (2010) 2010:1–10. doi: 10.1155/2010/289645
3. Calder, PC, Bosco, N, Bourdet-Sicard, R, Capuron, L, Delzenne, N, Dore, J, et al. Health relevance of the modification of low grade inflammation in ageing (inflammageing) and the role of nutrition. Ageing Res Rev. (2017) 40:95–119. doi: 10.1016/j.arr.2017.09.001
4. Simpson, N, and Dinges, DF. Sleep and inflammation. Nutr Rev. (2007) 65:244–52. doi: 10.1301/nr.2007.dec.S244-S252
5. Kolb, H, and Mandrup-Poulsen, T. The global diabetes epidemic as a consequence of lifestyle-induced low-grade inflammation. Diabetologia. (2010) 53:10–20. doi: 10.1007/s00125-009-1573-7
6. Heilbronn, LK, and Campbell, LV. Adipose tissue macrophages, low grade inflammation and insulin resistance in human obesity. Curr Pharm Des. (2008) 14:1225–30. doi: 10.2174/138161208784246153
7. Banait, T, Wanjari, A, Danade, V, Banait, S, and Jain, J. Role of high-sensitivity C-reactive protein (Hs-CRP) in non-communicable diseases: a review. Cureus. (2022) 14:e30225. doi: 10.7759/cureus.30225
8. Tamakoshi, K, Yatsuya, H, Kondo, T, Hori, Y, Ishikawa, M, Zhang, H, et al. The metabolic syndrome is associated with elevated circulating C-reactive protein in healthy reference range, a systemic low-grade inflammatory state. Int J Obes Relat Metab Disord. (2003) 27:443–9. doi: 10.1038/sj.ijo.0802260
9. Doi, Y, Kiyohara, Y, Kubo, M, Ninomiya, T, Wakugawa, Y, Yonemoto, K, et al. Elevated C-reactive protein is a predictor of the development of diabetes in a general Japanese population: the Hisayama study. Diabetes Care. (2005) 28:2497-500. doi: 10.2337/diacare.28.10.2497
10. Watanabe, Y, Kitamura, K, Nakamura, K, Sanpei, K, Wakasugi, M, Yokoseki, A, et al. Elevated C-reactive protein is associated with cognitive decline in outpatients of a general hospital: the project in Sado for Total health (PROST). Dement Geriatr Cogn Dis Extra. (2016) 6:10–9. doi: 10.1159/000442585
11. Arima, H, Kubo, M, Yonemoto, K, Doi, Y, Ninomiya, T, Tanizaki, Y, et al. High-sensitivity C-reactive protein and coronary heart disease in a general population of Japanese: the Hisayama study. Arterioscler Thromb Vasc Biol. (2008) 28:1385-91. doi: 10.1161/ATVBAHA.107.157164
12. Otani, T, Iwasaki, M, Sasazuki, S, Inoue, M, and Tsugane, S. Plasma C-reactive protein and risk of colorectal cancer in a nested case-control study: Japan public health center-based prospective study. Cancer Epidemiol Biomark Prev. (2006) 15:690–5. doi: 10.1158/1055-9965.EPI-05-0708
13. Szablewski, L . Glucose homeostasis–mechanism and defects In: E Rigobelo , editor. Diabetes – Damages and Treatments. London, UK: Intech Open (2010)
14. Solis-Herrera, C, Triplitt, C, Cersosimo, E, and DeFronzo, RA. Pathogenesis of type 2 diabetes mellitus In: KR Feingold, B Anawalt, MR Blackman, A Boyce, G Chrousos, and E Corpas, editors. Endotext. South Dartmouth, MA: MDText.com, Inc. (2021)
15. Meijnikman, AS, De Block, CEM, Dirinck, E, Verrijken, A, Mertens, I, Corthouts, B, et al. Not performing an OGTT results in significant underdiagnosis of (pre) diabetes in a high risk adult Caucasian population. Int J Obes. (2017) 41:1615–20. doi: 10.1038/ijo.2017.165
16. Donath, MY, and Shoelson, SE. Type 2 diabetes as an inflammatory disease. Nat Rev Immunol. (2011) 11:98–107. doi: 10.1038/nri2925
17. Schönknecht, YB, Crommen, S, Stoffel-Wagner, B, Coenen, M, Fimmers, R, Stehle, P, et al. Influence of a proinflammatory state on postprandial outcomes in elderly subjects with a risk phenotype for cardiometabolic diseases. Eur J Nutr. (2022) 61:3077–83. doi: 10.1007/s00394-022-02870-7
18. Amalraj, A, Pius, A, Gopi, S, and Gopi, S. Biological activities of curcuminoids, other biomolecules from turmeric and their derivatives – a review. J Tradit Complement Med. (2017) 7:205–33. doi: 10.1016/j.jtcme.2016.05.005
19. Flori, L, Piragine, E, Spezzini, J, Citi, V, Calderone, V, and Martelli, A. Influence of polyphenols on adipose tissue: Sirtuins as pivotal players in the Browning process. Int J Mol Sci. (2023) 24:9276. doi: 10.3390/ijms24119276
20. Jabczyk, M, Nowak, J, Hudzik, B, and Zubelewicz-Szkodzińska, B. Curcumin in metabolic health and disease. Nutrients. (2021) 13:4440. doi: 10.3390/nu13124440
21. Aggarwal, BB, Yuan, W, Li, S, and Gupta, SC. Curcumin-free turmeric exhibits anti-inflammatory and anticancer activities: identification of novel components of turmeric. Mol Nutr Food Res. (2013) 57:1529–42. doi: 10.1002/mnfr.201200838
22. Uchio, R, Muroyama, K, Okuda-Hanafusa, C, Kawasaki, K, Yamamoto, Y, and Murosaki, S. Hot water extract of Curcuma longa L. improves serum inflammatory markers and general health in subjects with overweight or prehypertension/mild hypertension: a randomized, double-blind, placebo-controlled trial. Nutrients. (2019) 11:1822. doi: 10.3390/nu11081822
23. Uchio, R, Kawasaki, K, Okuda-Hanafusa, C, Saji, R, Muroyama, K, Murosaki, S, et al. Curcuma longa extract improves serum inflammatory markers and mental health in healthy participants who are overweight: a randomized, double-blind, placebo-controlled trial. Nutr J. (2021) 20:91. doi: 10.1186/s12937-021-00748-8
24. Okuda-Hanafusa, C, Uchio, R, Fuwa, A, Kawasaki, K, Muroyama, K, Yamamoto, Y, et al. Turmeronol A and turmeronol B from Curcuma longa prevent inflammatory mediator production by lipopolysaccharide-stimulated RAW264.7 macrophages, partially via reduced NF-kappa B signaling. Food Funct. (2019) 10:5779–88. doi: 10.1039/C9FO00336C
25. Sun, DI, Nizamutdinova, IT, Kim, YM, Cai, XF, Lee, JJ, Kang, SS, et al. Bisacurone inhibits adhesion of inflammatory monocytes or cancer cells to endothelial cells through down-regulation of VCAM-1 expression. Int Immunopharmacol. (2008) 8:1272–81. doi: 10.1016/j.intimp.2008.05.006
26. Uchio, R, Higashi, Y, Kohama, Y, Kawasaki, K, Hirao, T, Muroyama, K, et al. A hot water extract of turmeric (Curcuma longa) suppresses acute ethanol-induced liver injury in mice by inhibiting hepatic oxidative stress and inflammatory cytokine production. J Nutr Sci. (2017) 6:e3. doi: 10.1017/jns.2016.43
27. Cui, Y, Chi, J, and Wang, L. Protective efficacy of Bisacurone in diabetic cardiotoxicity in experimental animals via downregulation of inflammatory and apoptotic pathways. Lat Am J Pharm. (2020) 39:2507–19. Available at: http://www.latamjpharm.org/resumenes/39/12/LAJOP_39_12_1_27.pdf
28. Kawasaki, K, Muroyama, K, Yamamoto, N, and Murosaki, S. A hot water extract of Curcuma longa inhibits adhesion molecule protein expression and monocyte adhesion to TNF-alpha-stimulated human endothelial cells. Biosci Biotechnol Biochem. (2015) 79:1654–9. doi: 10.1080/09168451.2015.1039480
29. Kawasaki, K, Okuda-Hanafusa, C, Aoyagi, M, Taoka, K, Yamamoto, N, Muroyama, K, et al. Inhibitory effect of the compounds from the water extract of Curcuma longa on the production of PGE2 and NO in a macrophage cell line stimulated by LPS. Biosci Biotechnol Biochem. (2018) 82:2109–17. doi: 10.1080/09168451.2018.1511366
30. Mun, J, Kim, S, Yoon, HG, You, Y, Kim, OK, Choi, KC, et al. Water extract of Curcuma longa L. ameliorates non-alcoholic fatty liver disease. Nutrients. (2019) 11:2536. doi: 10.3390/nu11102536
31. Kim, S, Kim, K, Park, J, and Jun, W. Curcuma longa L. water extract improves dexamethasone-induced sarcopenia by modulating the muscle-related gene and Oxidative stress in mice. Antioxidants (Basel). (2021) 10:1000. doi: 10.3390/antiox10071000
32. Asada, K, Ohara, T, Muroyama, K, Yamamoto, Y, and Murosaki, S. Effects of hot water extract of Curcuma longa on human epidermal keratinocytes in vitro and skin conditions in healthy participants: a randomized, double-blind, placebo-controlled trial. J Cosmet Dermatol. (2019) 18:1866–74. doi: 10.1111/jocd.12890
33. Kawasaki, K, Muroyama, K, and Murosaki, S. Effect of a water extract of Curcuma longa on emotional states in healthy participants. Biosci Microbiota Food Health. (2018) 37:25–9. doi: 10.12938/bmfh.17-020
34. Yu, ZF, Kong, LD, and Chen, Y. Antidepressant activity of aqueous extracts of Curcuma longa in mice. J Ethnopharmacol. (2002) 83:161–5. doi: 10.1016/S0378-8741(02)00211-8
35. Sengupta, M, Sharma, GD, and Chakraborty, B. Hepatoprotective and immunomodulatory properties of aqueous extract of Curcuma longa in carbon tetra chloride intoxicated Swiss albino mice. Asian Pac J Trop Biomed. (2011) 1:193–9. doi: 10.1016/S2221-1691(11)60026-9
36. Uchio, R, Murosaki, S, and Ichikawa, H. Hot water extract of turmeric (Curcuma longa) prevents non-alcoholic steatohepatitis in mice by inhibiting hepatic oxidative stress and inflammation. J Nutr Sci. (2018) 7:e36. doi: 10.1017/jns.2018.27
37. Nagavekar, N, and Singhal, RS. Supercritical fluid extraction of Curcuma longa and Curcuma amada oleoresin: optimization of extraction conditions, extract profiling, and comparison of bioactivities. Ind Crop Prod. (2019) 134:134–45. doi: 10.1016/j.indcrop.2019.03.061
38. Sayeli, VK, and Shenoy, AK. Antidiabetic effect of bio-enhanced preparation of turmeric in streptozotocin-nicotinamide induced type 2 diabetic Wistar rats. J Ayurveda Integr Med. (2021) 12:474–9. doi: 10.1016/j.jaim.2021.04.010
39. Su, JY, Tan, LR, Lai, P, Liang, HC, Qin, Z, Ye, MR, et al. Experimental study on anti-inflammatory activity of a TCM recipe consisting of the supercritical fluid CO2 extract of Chrysanthemum indicum, patchouli oil and zedoary turmeric oil in vivo. J Ethnopharmacol. (2012) 141:608–14. doi: 10.1016/j.jep.2011.08.055
40. Chang, KW, Lin, TY, Fu, SL, Ping, YH, Chen, FP, and Kung, YY. A Houttuynia cordata-based Chinese herbal formula improved symptoms of allergic rhinitis during the COVID-19 pandemic. J Chin Med Assoc. (2022) 85:717–22. doi: 10.1097/JCMA.0000000000000732
41. Woranam, K, Senawong, G, Utaiwat, S, Yunchalard, S, Sattayasai, J, and Senawong, T. Anti-inflammatory activity of the dietary supplement Houttuynia cordata fermentation product in RAW264.7 cells and Wistar rats. PLoS One. (2020) 15:e0230645. doi: 10.1371/journal.pone.0230645
42. Takahashi, M, Mineshita, Y, Yamagami, J, Wang, C, Fujihira, K, Tahara, Y, et al. Effects of the timing of acute mulberry leaf extract intake on postprandial glucose metabolism in healthy adults: a randomised, placebo-controlled, double-blind study. Eur J Clin Nutr. (2023) 77:468–73. doi: 10.1038/s41430-023-01259-x
43. Zheng, XX, Li, DX, Li, YT, Chen, YL, Zhao, YL, Ji, S, et al. Mulberry leaf water extract alleviates type 2 diabetes in mice via modulating gut microbiota-host co-metabolism of branched-chain amino acid. Phytother Res. (2023) 37:3195–210. doi: 10.1002/ptr.7822
44. Suriyaprom, S, Srisai, P, Intachaisri, V, Kaewkod, T, Pekkoh, J, Desvaux, M, et al. Antioxidant and anti-inflammatory activity on LPS-stimulated RAW 264.7 macrophage cells of white mulberry (Morus alba L.) leaf extracts. Molecules. (2023) 28:4395. doi: 10.3390/molecules28114395
45. He, C, Miyazawa, T, Abe, C, Ueno, T, Suzuki, M, Mizukami, M, et al. Hypolipidemic and anti-inflammatory effects of Curcuma longa-derived Bisacurone in high-fat diet-fed mice. Int J Mol Sci. (2023) 24:9366. doi: 10.3390/ijms24119366
46. Kang, HJ, Hong, SH, Kang, KH, Park, C, and Choi, YH. Anti-inflammatory effects of Hwang-Heuk-san, a traditional Korean herbal formulation, on lipopolysaccharide-stimulated murine macrophages. BMC Complement Altern Med. (2015) 15:447. doi: 10.1186/s12906-015-0971-2
47. Park, JW, Kwon, OK, Kim, JH, Oh, SR, Kim, JH, Paik, JH, et al. Rhododendron album Blume inhibits iNOS and COX-2 expression in LPS-stimulated RAW264.7 cells through the downregulation of NF-kappa B signaling. Int J Mol Med. (2015) 35:987–94. doi: 10.3892/ijmm.2015.2107
48. Schulz, KF, Altman, DG, and Moher, D. CONSORT 2010 statement: updated guidelines for reporting parallel group randomised trials. BMC Med. (2010) 8:18. doi: 10.1186/1741-7015-8-18
49. Shiwaku, K, Anuurad, E, Enkhmaa, B, Nogi, A, Kitajima, K, Shimono, K, et al. Overweight Japanese with body mass indexes of 23.0-24. 9 have higher risks for obesity-associated disorders: a comparison of Japanese and Mongolians. Int J Obes Relat Metab Disord. (2004) 28:152–8. doi: 10.1038/sj.ijo.0802486
50. Bartoli, E, Fra, GP, and Carnevale Schianca, GP. The oral glucose tolerance test (OGTT) revisited. Eur J Intern Med. (2011) 22:8–12. doi: 10.1016/j.ejim.2010.07.008
51. Abdul-Ghani, MA, Tripathy, D, and DeFronzo, RA. Contributions of beta-cell dysfunction and insulin resistance to the pathogenesis of impaired glucose tolerance and impaired fasting glucose. Diabetes Care. (2006) 29:1130–9. doi: 10.2337/dc05-2179
52. Radikova, Z . Assessment of insulin sensitivity/resistance in epidemiological studies. Endocr Regul. (2003) 37:189–94. Available at: https://www.sav.sk/journals/endo/full/2003/2003_03g.pdf
53. Onishi, Y, Hayashi, T, Sato, KK, Leonetti, DL, Kahn, SE, Fujimoto, WY, et al. Comparison of twenty indices of insulin sensitivity in predicting type 2 diabetes in Japanese Americans: the Japanese American community diabetes study. J Diabetes Complicat. (2020) 34:107731. doi: 10.1016/j.jdiacomp.2020.107731
54. Theurey, P, Thang, C, Pirags, V, Mari, A, Pacini, G, Bolze, S, et al. Phase 2 trial with imeglimin in patients with type 2 diabetes indicates effects on insulin secretion and sensitivity. Endocrinol Diabetes Metab. (2022) 5:e371. doi: 10.1002/edm2.371
55. Honsek, C, Kabisch, S, Kemper, M, Gerbracht, C, Arafat, AM, Birkenfeld, AL, et al. Fibre supplementation for the prevention of type 2 diabetes and improvement of glucose metabolism: the randomised controlled optimal fibre trial (Opti FiT). Diabetologia. (2018) 61:1295–305. doi: 10.1007/s00125-018-4582-6
56. Ayele, Y, Kim, JA, Park, E, Kim, YJ, Retta, N, Dessie, G, et al. A methanol extract of Adansonia digitata L. Leaves inhibits pro-inflammatory iNOS possibly via the inhibition of NF-κB activation. Biomol Ther (Seoul). (2013) 21:146–52. doi: 10.4062/biomolther.2012.098
57. Rita, K, Bernardo, MA, Silva, ML, Brito, J, Mesquita, MF, Pintão, AM, et al. Adansonia digitata L. (Baobab Fruit) Effect on postprandial Glycemia in healthy adults: a randomized controlled trial. Nutrients. (2022) 14:398. doi: 10.3390/nu14020398
58. Majeed, M, Nagabhushanam, K, Devarajan, TV, Saklecha, S, Reddy, SVK, and Mundkur, L. A minor metabolite from Curcuma longa effective against metabolic syndrome: results from a randomized, double-blind, placebo-controlled clinical study. Food Funct. (2023) 14:4722–33. doi: 10.1039/D2FO03627D
59. Higashikawa, F, Noda, M, Awaya, T, Tanaka, T, and Sugiyama, M. 5-aminolevulinic acid, a precursor of heme, reduces both fasting and postprandial glucose levels in mildly hyperglycemic subjects. Nutrition. (2013) 29:1030–6. doi: 10.1016/j.nut.2013.02.008
60. Matsumoto, M, Kitada, Y, and Naito, Y. Endothelial function is improved by inducing microbial polyamine production in the gut: a randomized placebo-controlled trial. Nutrients. (2019) 11:1188. doi: 10.3390/nu11051188
61. Paschalis, V, Theodorou, AA, Kyparos, A, Dipla, K, Zafeiridis, A, Panayiotou, G, et al. Low vitamin C values are linked with decreased physical performance and increased oxidative stress: reversal by vitamin C supplementation. Eur J Nutr. (2016) 55:45–53. doi: 10.1007/s00394-014-0821-x
62. Howell, DC . Statistical methods for psychology. 7th Edn Belmont, CA: Wadsworth Publishing Co., Inc. (2009) 461–483.
63. Blumenthal, K, Freeman, E, Saff, R, Robinson, L, Wolfson, A, Foreman, R, et al. Delayed large local reactions to mRNA-1273 vaccine against SARS-CoV-2. N Engl J Med. (2021) 384:1273–7. doi: 10.1056/NEJMc2102131
64. Donath, MY . Targeting inflammation in the treatment of type 2 diabetes: time to start. Nat Rev Drug Discov. (2014) 13:465–76. doi: 10.1038/nrd4275
65. Rothwell, PM, Price, JF, Fowkes, FG, Zanchetti, A, Roncaglioni, MC, Tognoni, G, et al. Short-term effects of daily aspirin on cancer incidence, mortality, and non-vascular death: analysis of the time course of risks and benefits in 51 randomised controlled trials. Lancet. (2012) 379:1602–12. doi: 10.1016/S0140-6736(11)61720-0
66. Ferrucci, L, and Fabbri, E. Inflammageing: chronic inflammation in ageing, cardiovascular disease, and frailty. Nat Rev Cardiol. (2018) 15:505–22. doi: 10.1038/s41569-018-0064-2
67. Ito, C, Maeda, R, Ishida, S, Sasaki, H, and Harada, H. Correlation among fasting plasma glucose, two-hour plasma glucose levels in OGTT and HbA1c. Diabetes Res Clin Pract. (2000) 50:225–30. doi: 10.1016/S0168-8227(00)00187-X
68. Ortiz-Martínez, M, González-González, M, Martagón, AJ, Hlavinka, V, Willson, RC, and Rito-Palomares, M. Recent developments in biomarkers for diagnosis and screening of type 2 diabetes mellitus. Curr Diab Rep. (2022) 22:95–115. doi: 10.1007/s11892-022-01453-4
69. Doi, Y, Kiyohara, Y, Kubo, M, Tanizaki, Y, Okubo, K, Ninomiya, T, et al. Relationship between C-reactive protein and glucose levels in community-dwelling subjects without diabetes: the Hisayama study. Diabetes Care. (2005) 28:1211-3. doi: 10.2337/diacare.28.5.1211
70. Bu, L, Gao, M, Qu, S, and Liu, D. Intraperitoneal injection of clodronate liposomes eliminates visceral adipose macrophages and blocks high-fat diet-induced weight gain and development of insulin resistance. AAPS J. (2013) 15:1001–11. doi: 10.1208/s12248-013-9501-7
71. Feng, B, Jiao, P, Nie, Y, Kim, T, Jun, D, van Rooijen, N, et al. Clodronate liposomes improve metabolic profile and reduce visceral adipose macrophage content in diet-induced obese mice. PLoS One. (2011) 6:e24358. doi: 10.1371/journal.pone.0024358
72. Fleischman, A, Shoelson, SE, Bernier, R, and Goldfine, AB. Salsalate improves glycemia and inflammatory parameters in obese young adults. Diabetes Care. (2008) 31:289–94. doi: 10.2337/dc07-1338
73. Hayashino, Y, Hennekens, CH, and Kurth, T. Aspirin use and risk of type 2 diabetes in apparently healthy men. Am J Med. (2009) 122:374–9. doi: 10.1016/j.amjmed.2008.09.044
74. Wang, X, Zhao, X, Zhou, R, Gu, Y, Zhu, X, Tang, Z, et al. Delay in glucose peak time during the oral glucose tolerance test as an indicator of insulin resistance and insulin secretion in type 2 diabetes patients. J Diabetes Investig. (2018) 9:1288–95. doi: 10.1111/jdi.12834
75. Wang, Q, Jokelainen, J, Auvinen, J, Puukka, K, Keinänen-Kiukaanniemi, S, Järvelin, MR, et al. Insulin resistance and systemic metabolic changes in oral glucose tolerance test in 5340 individuals: an interventional study. BMC Med. (2019) 17:217. doi: 10.1186/s12916-019-1440-4
76. Dirir, AM, Daou, M, Yousef, AF, and Yousef, LF. A review of alpha-glucosidase inhibitors from plants as potential candidates for the treatment of type-2 diabetes. Phytochem Rev. (2022) 21:1049–79. doi: 10.1007/s11101-021-09773-1
77. Kaneko, Y, Kuboki, K, Hiroi, N, Watanabe, T, Nishimura, C, and Yoshino, G. Effect of Miglitol, an α-glucosidase inhibitor, on postprandial glucose and lipid metabolism in patients with type 2 diabetes. Showa Univ J Med Sci. (2011) 23:217–25. doi: 10.15369/sujms.23.217
78. Gentilcore, D, Vanis, L, Wishart, JM, Rayner, CK, Horowitz, M, and Jones, KL. The alpha (α)-glucosidase inhibitor, acarbose, attenuates the blood pressure and splanchnic blood flow responses to intraduodenal sucrose in older adults. J Gerontol A Biol Sci Med Sci. (2011) 66:917–24. doi: 10.1093/gerona/glr086
79. Jumpertz, R, Thearle, MS, Bunt, JC, and Krakoff, J. Assessment of non-insulin-mediated glucose uptake: association with body fat and glycemic status. Metabolism. (2010) 59:1396–401. doi: 10.1016/j.metabol.2010.01.006
80. Abdul-Ghani, MA, and DeFronzo, RA. Pathogenesis of insulin resistance in skeletal muscle. J Biomed Biotechnol. (2010) 2010:476279. doi: 10.1155/2010/476279
81. Sylow, L, Tokarz, VL, Richter, EA, and Klip, A. The many actions of insulin in skeletal muscle, the paramount tissue determining glycemia. Cell Metab. (2021) 33:758–80. doi: 10.1016/j.cmet.2021.03.020
82. DeFronzo, RA . Pathogenesis of type 2 diabetes mellitus. Med Clin North Am. (2004) 88:787–835. doi: 10.1016/j.mcna.2004.04.013
83. Tanaka, Y, Atsumi, Y, Asahina, T, Hosokawa, K, Matsuoka, K, Kinoshita, J, et al. Usefulness of revised fasting plasma glucose criterion and characteristics of the insulin response to an oral glucose load in newly diagnosed Japanese diabetic subjects. Diabetes Care. (1998) 21:1133–7. doi: 10.2337/diacare.21.7.1133
84. Otaka, Y, Kanai, K, Okada, D, Nagai, N, Yamashita, Y, Ichikawa, Y, et al. Effects of Oral 5-Aminolevulinic acid on lipopolysaccharide-induced ocular inflammation in rats. Vet Sci. (2023) 10:207. doi: 10.3390/vetsci10030207
85. Nakamura, Y, Haraguchi, A, Horie, I, Kawakami, A, and Abiru, N. Pilot trial on the effect of 5-Aminolevulinic acid on glucose tolerance in patients with maternally inherited diabetes and deafness. Diabetes Ther. (2023) 14:447–59. doi: 10.1007/s13300-022-01335-8
86. Miyazaki, Y, Glass, L, Triplitt, C, Matsuda, M, Cusi, K, Mahankali, A, et al. Effect of rosiglitazone on glucose and non-esterified fatty acid metabolism in type II diabetic patients. Diabetologia. (2001) 44:2210–9. doi: 10.1007/s001250100031
87. Mohankumar, S, and McFarlane, JR. An aqueous extract of Curcuma longa (turmeric) rhizomes stimulates insulin release and mimics insulin action on tissues involved in glucose homeostasis in vitro. Phytother Res. (2011) 25:396–401. doi: 10.1002/ptr.3275
88. Chung, ST, Ha, J, Onuzuruike, AU, Kasturi, K, Galvan-De La Cruz, M, Bingham, BA, et al. Time to glucose peak during an oral glucose tolerance test identifies prediabetes risk. Clin Endocrinol. (2017) 87:484–91. doi: 10.1111/cen.13416
89. Woerle, HJ, Neumann, C, Zschau, S, Tenner, S, Irsigler, A, Schirra, J, et al. Impact of fasting and postprandial glycemia on overall glycemic control in type 2 diabetes importance of postprandial glycemia to achieve target HbA1c levels. Diabetes Res Clin Pract. (2007) 77:280–5. doi: 10.1016/j.diabres.2006.11.011
90. Monnier, L, Lapinski, H, and Colette, C. Contributions of fasting and postprandial plasma glucose increments to the overall diurnal hyperglycemia of type 2 diabetic patients: variations with increasing levels of HbA1c. Diabetes Care. (2003) 26:881–5. doi: 10.2337/diacare.26.3.881
91. Sloan-Lancaster, J, Abu-Raddad, E, Polzer, J, Miller, JW, Scherer, JC, De Gaetano, A, et al. Double-blind, randomized study evaluating the glycemic and anti-inflammatory effects of subcutaneous LY2189102, a neutralizing IL-1β antibody, in patients with type 2 diabetes. Diabetes Care. (2013) 36:2239–46. doi: 10.2337/dc12-1835
92. Li, M, Chi, X, Wang, Y, Setrerrahmane, S, Xie, W, and Xu, H. Trends in insulin resistance: insights into mechanisms and therapeutic strategy. Signal Transduct Target Ther. (2022) 7:216. doi: 10.1038/s41392-022-01073-0
93. Cho, KA, and Kang, PB. PLIN2 inhibits insulin-induced glucose uptake in myoblasts through the activation of the NLRP3 inflammasome. Int J Mol Med. (2015) 36:839–44. doi: 10.3892/ijmm.2015.2276
94. Nov, O, Kohl, A, Lewis, EC, Bashan, N, Dvir, I, Ben-Shlomo, S, et al. Interleukin-1beta may mediate insulin resistance in liver-derived cells in response to adipocyte inflammation. Endocrinology. (2010) 151:4247–56. doi: 10.1210/en.2010-0340
95. Jager, J, Gremeaux, T, Cormont, M, Le Marchand-Brustel, Y, and Tanti, JF. Interleukin-1beta-induced insulin resistance in adipocytes through down-regulation of insulin receptor substrate-1 expression. Endocrinology. (2007) 148:241–51. doi: 10.1210/en.2006-0692
96. de Alvaro, C, Teruel, T, Hernandez, R, and Lorenzo, M. Tumor necrosis factor alpha produces insulin resistance in skeletal muscle by activation of inhibitor kappa B kinase in a p 38 MAPK-dependent manner. J Biol Chem. (2004) 279:17070–8. doi: 10.1074/jbc.M312021200
97. de Roos, B, Rungapamestry, V, Ross, K, Rucklidge, G, Reid, M, Duncan, G, et al. Attenuation of inflammation and cellular stress-related pathways maintains insulin sensitivity in obese type I interleukin-1 receptor knockout mice on a high-fat diet. Proteomics. (2009) 9:3244–56. doi: 10.1002/pmic.200800761
98. de Luca, C, and Olefsky, JM. Inflammation and insulin resistance. FEBS Lett. (2008) 582:97–105. doi: 10.1016/j.febslet.2007.11.057
99. Krogh-Madsen, R, Plomgaard, P, Møller, K, Mittendorfer, B, and Pedersen, BK. Influence of TNF-alpha and IL-6 infusions on insulin sensitivity and expression of IL-18 in humans. Am J Physiol Endocrinol Metab. (2006) 291:E108–14. doi: 10.1152/ajpendo.00471.2005
100. Chen, L, Chen, R, Wang, H, and Liang, F. Mechanisms linking inflammation to insulin resistance. Int J Endocrinol. (2015) 2015:508409:1–9. doi: 10.1155/2015/508409
101. Marette, A . Molecular mechanisms of insulin resistance in obesity. CMR J. (2008) 1:5–9. Available at: https://www.myhealthywaist.org/cmrejournal/pdf/vol1/v1i1a3.pdf
102. Soskić, SS, Dobutović, BD, Sudar, EM, Obradović, MM, Nikolić, DM, Djordjevic, JD, et al. Regulation of inducible nitric oxide synthase (iNOS) and its potential role in insulin resistance, diabetes and heart failure. Open Cardiovasc Med J. (2011) 5:153–63. doi: 10.2174/1874192401105010153
103. Saji, R, Uchio, R, Fuwa, A, Okuda-Hanafusa, C, Kawasaki, K, Muroyama, K, et al. Turmeronols (A and B) from Curcuma longa have anti-inflammatory effects in lipopolysaccharide-stimulated BV-2 microglial cells by reducing NF-κB signaling. Biosci Microbiota Food Health. (2023) 42:172–9. doi: 10.12938/bmfh.2022-071
104. Ashida, H, Tian, X, Kitakaze, T, and Yamashita, Y. Bisacurone suppresses hepatic lipid accumulation through inhibiting lipogenesis and promoting lipolysis. J Clin Biochem Nutr. (2020) 67:43–52. doi: 10.3164/jcbn.20-16
Glossary
Keywords: Curcuma longa (turmeric) extract, bisacurone, turmeronol, chronic inflammation, C-reactive protein, hemoglobin A1c, insulin sensitivity, oral glucose tolerance test
Citation: Uchio R, Okuda-Hanafusa C, Sakaguchi H, Saji R, Muroyama K, Murosaki S, Yamamoto Y and Hirose Y (2024) Curcuma longa extract reduces serum inflammatory markers and postprandial hyperglycemia in healthy but borderline participants with overweight and glycemia in the normal/prediabetes range: a randomized, double-blind, and placebo-controlled trial. Front. Nutr. 11:1324196. doi: 10.3389/fnut.2024.1324196
Edited by:
Santiago Navas-Carretero, University of Navarra, SpainReviewed by:
Denisa Margina, Carol Davila University of Medicine and Pharmacy, RomaniaCharalampia Amerikanou, Harokopio University, Greece
Copyright © 2024 Uchio, Okuda-Hanafusa, Sakaguchi, Saji, Muroyama, Murosaki, Yamamoto and Hirose. This is an open-access article distributed under the terms of the Creative Commons Attribution License (CC BY). The use, distribution or reproduction in other forums is permitted, provided the original author(s) and the copyright owner(s) are credited and that the original publication in this journal is cited, in accordance with accepted academic practice. No use, distribution or reproduction is permitted which does not comply with these terms.
*Correspondence: Ryusei Uchio, dWNoaW9fcnl1c2VpQGhvdXNlLXdmLmNvLmpw