The correlation between iodine and metabolism: a review
- 1Department of Endocrinology, Shengjing Hospital of China Medical University, Shenyang, China
- 2Department of General Surgery, The Fourth Affiliated Hospital of China Medical University, Shenyang, China
Iodine is involved in the synthesis of thyroid hormones and plays a crucial role in human life. Both iodine deficiency and excess are common issues in certain populations. Iodine also has extrathyroidal effects on organs that can uptake it independently of thyroid hormones. Recently, multiple clinical studies have shown a connection between iodine intake and metabolic disorders, such as metabolic syndrome, obesity, diabetes, hypertension, and dyslipidemia. However, the results of these studies have been inconsistent, and the mechanisms behind these associations are still not well understood. Therefore, in this review, we aim to examine the recent research progress regarding the relationship between iodine and metabolic disorders, along with the relevant mechanisms.
1 Introduction
Iodine is an elementary micronutrient for human life. It exist in various forms in human body, such as iodide atom (I−), molecular iodine (I2), triiodide (I3), iodine anion (HI2O−), and iodine-binding molecules (such as iodolipids) (1). I− is a kind of reducing agent that can be oxidized by peroxidase enzymes to generate thyroid hormones (THs). Tri-iodothyronine (T3) and thyroxine (T4) are essential regulators of energetic metabolism (1). Recent studies have indicated that iodine, aside from its role in the thyroid, also functions as an antioxidant, immunomodulator, and differentiator in various organs and tissues (2).
Metabolic syndrome (MetS) is defined as the combination of metabolic disorders including abdominal obesity, hypertension (HBP), dyslipidemia, and hyperglycemia (3). MetS is widely prevalent worldwide and poses serious issues such as cardiovascular disease (CVD), tumors, and total mortality (4). The development of MetS involves multiple factors, with research indicating that oxidative stress and chronic inflammatory conditions play a vital role (5). Changes in dietary habits are another contributing aspect to the prevalence of MetS. Consequently, factors associated with MetS, such as iodine nutritional status, may partially explain its occurrence of MetS.
In this review, we focus on iodine and its connection to metabolic disorders, as well as the associated mechanisms. The findings will aid us in acquiring a comprehensive understanding of iodine’s role and provide supporting evidence for an appropriate and secure iodine nutrition standard.
2 The molecular basis of extrathyroidal effects of iodine
Sodium iodide symporter (NIS) is the most effective and specific transporter of I− expressed on thyroid follicular epithelial cells. Many other organs can also actively accumulate iodine, including the salivary glands, stomach, lactating mammary gland, ovary, prostate, and pancreas. In addition to NIS and Pendrin, new iodine transporters, including cystic fibrosis transmembrane conductance regulator (CFTR), anoctamin 1 (ANO1), and sodium multivitamin transporter (SMVT), have recently been discovered to be expressed in these organs (2). Therefore, iodine itself has extrathyroidal effects on organs that can take it up.
3 Sources and safety concentration of iodine
In order to eliminate iodine deficiency diseases, most countries have implemented universal salt iodization (USI). However, excess iodine intakes can occur due to the consumption of iodized salt, drinking water, animal milk rich in iodine, specific types of seaweeds, iodine-containing dietary supplements, and from a combination of these sources. Some pharmaceuticals (like Amiodarone), disinfectants, and iodine-containing contrast media, can also be common sources of iodine (6). Over 90 percent of dietary iodine is absorbed under normal conditions (7). The recommended dietary intake of iodine is 150–299 μg/day (8, 9). The appropriate range of median urinary iodine concentration (mUIC) should be 100–299 μg/L. Japanese average iodine consumption is 1,200 μg/day, which is 7.2 times higher than that of the British and 5.7 times higher than that of Americans (10). Iodine excess can increase the risk of hyperthyroidism and subclinical hypothyroidism (11). However, euthyroid individuals are usually tolerant to iodine-induced thyroid diseases as their thyroid function rapidly normalizes upon discontinuation of excessive iodine consumption (8, 12). A moderately high iodine intake has been shown to be beneficial in reducing the incidence of breast and prostate cancer in some epidemiological studies (13–15).
4 Clinical research on the correlation between iodine status and metabolic disorders
Recent research has examined the impact of iodine itself on the prevalence of metabolic disorders. The majority of clinical studies were published within the past 5 years. Various indicators were used to evaluate iodine nutritional status, including urinary iodine concentration (UIC), water iodine concentration (WIC), and daily iodine consumption. However, due to the use of different indicators for assessing iodine nutrition, varying diagnostic criteria for metabolic disorders, and differences in the age and gender of the subjects, the studies did not yield consistent findings. The representative studies on the relationship between iodine and metabolic disorders have been summarized in Table 1.
4.1 Iodine and metabolic syndrome
In cross-sectional studies, the relationship between UIC and MetS prevalence was found to be inversely associated or exhibited a U-shaped curve, with the lowest point observed at a UIC of 300–499 μg/L (24, 26). A prospective study indicated that dietary iodine and seaweed consumption was inversely associated with MetS incidence in Korean postmenopausal women (19). Furthermore, high seaweed intake was negatively associated with the incidence of MetS in men with the TG and TT genotypes of lipoprotein lipase gene (LPL) rs17482735 (31), However, another cross-sectional study conducted on school-age children and their parents revealed that high UIC was associated with MetS (30).
4.2 Iodine and obesity
In a large epidemiological study (TIDE) conducted in China, the prevalence of central obesity significantly decreased when the UIC was 300 μg/L or higher. The odds ratio (OR) for central obesity with an UIC of ≥800 μg/L was 0.797 (p < 0.05) (24). Among school-age children in China, overweight children exhibited a lower UIC compared to children with normal weight (32). Women with obesity also demonstrated a significantly lower UIC in comparison to both themselves after undergoing bariatric surgery and women with normal weight (33). In a randomized controlled trial (RCT), the body fat percentage of the participants who consumed tablets containing iodine-reduced kelp powder showed a significant decrease in comparison to those who took the placebo (34). In a 4-week placebo-controlled study, seaweed fucoxanthin supplementation (1 mg/day) decreased waist circumference (WC) and fat mass in obese Japanese individuals. In addition, fucoxanthin supplementation (3 mg/day) decreased visceral fat, body mass index (BMI), and weight (35). However, mUIC was positively associated with obesity among Colombian women of reproductive age (36).
4.3 Iodine and hyperglycemia
The association between UIC and the prevalence of diabetes exhibited a U-shaped curve in the TIDE study (24). In a cohort study involving 71,264 women, individuals with higher levels of iodine intake were found to be at a higher risk of developing type 2 diabetes mellitus (T2DM) in comparison to those with inadequate iodine intake (18). Adults in iodine-sufficient (IS, mUIC 200–299.99 μg/L) and iodine-excess (IE, mUIC ≥300 μg/L) areas exhibited higher blood glucose levels compared to those in iodine-adequate area (IA, mUIC 100–199.99 μg/L) (23). Low UIC was also reported to be associated with an increased risk of elevated fasting plasma glucose (FPG) in females (27). A study revealed that patients with T2DM had lower UIC levels compared to healthy individuals. Furthermore, UIC showed a negative correlation with insulin resistance in subjects with T2DM (37). However, in a 4-week clinical trial, seaweed supplementation (48 g/day) decreased blood glucose levels in Korean patients with T2DM (38).
Higher iodine status may potentially protect against hyperglycemia during pregnancy. A study indicated that pregnant and lactating women in the IE area (mWIC >300 μg/L) had lower blood glucose levels and a lower prevalence of hyperglycemia (29). Furthermore, a higher concentration of iodine in the placenta was associated with a reduced incidence of gestational diabetes mellitus (GDM) among 471 pregnant women (20). However, Bell et al. did not find any correlation between UIC and the prevalence of GDM (39).
4.4 Iodine and hypertension
In the TIDE study, researchers observed a U-shaped curve in the relationship between UIC and the prevalence of hypertension. The lowest point was found at a UIC range of 300–499 μg/L (24). Two studies conducted in China revealed that adults in IS and IE areas exhibited higher blood pressure levels compared to those in IA area (23, 29). Meanwhile, iodine deficiency was identified as a risk factor for preeclampsia and hypertensive disease of pregnancy (HPD) (40). However, a randomized case–control study revealed that wakame (Undaria pinnatifida) intake (5 g/day) in brown algae significantly decreased blood pressure in 36 older Japanese individuals with hypertension (41).
4.5 Iodine and dyslipidemia
The National Health and Nutrition Examination Survey (NHANES) 2007–2012 reported that subjects with the lowest decile of UIC were more likely to be at risk for elevated total cholesterol (TC) (adjusted odds ratio (aOR) = 1.51) and elevated low-density lipoprotein (LDL) cholesterol (aOR = 1.58), compared to those with the highest decile of UIC (21). US adolescents with low UIC had a significantly higher risk of hypercholesterolemia, elevated non-high-density lipoprotein (HDL), and elevated LDL compared to those with normal UIC (22). RCTs have reported that iodine supplementation reduces hypercholesterolemia incidence in overweight women (42) and also decreased serum LDL-C levels in overweight Japanese adults (34). Seaweed supplementation increased HDL-C levels and decreased TG levels in Korean patients with T2DM (38). A meta-analysis found that brown seaweed intake significantly decreased the levels of TC (mean difference (MD): −3.001; 95% CI: −5.770, −0.232) and LDL-C (MD: −6.519; 95% CI: −12.884, −0.154) (43). However, there is a observational study that propose the opposite conclusion (23). Two studies have found that the relationship between iodine status and dyslipidemia is either a U-shaped (24) or inverted U-shaped curve (28).
4.6 Iodine and hyperuricemia and gout
So far, the relationship has only been reported in one epidemiological study, which found an inverse association between UIC and the prevalence of hyperuricemia and gout. Subjects in the IS and IE groups had a lower probability of having hyperuricemia and gout in comparison to those in the IA group (25).
4.7 Iodine and mortality risk
Longitudinal data indicated an excess mortality in individuals with ID (UIC <100 μg/L) after adjusting for confounding factors. The HRs for all-cause mortality were 1.29 in individuals with UIC of 50–99 μg/L, and 1.71 in individuals with UIC of less than 50 μg/L. Iodine excess did not increase the risk of mortality (17). However, the NHANES III reported contradictory outcomes. In a median follow-up period lasting 19.2 years, having a UIC higher than 400 μg/L indicated an increased risk (HR = 1.19) for all-cause mortality. There was no observed correlation between low UIC and an increased risk of mortality (16).
5 Basic research on the correlation between iodine and metabolism
Clinical studies have demonstrated that iodine nutrition has an impact on metabolism. However, the mechanism involved in these effects is still poorly understood and requires further investigation. Due to varying dosages of iodine administration, durations of intervention, experimental animals, and thyroid hormone values, different in vivo studies have failed to reach a consensus. Kroupova et al. discovered that iodine intake could result in a dose-dependent elevation in blood cholesterol levels among hens (44). A study demonstrated that iodine excess (2.4 and 4.8 mg/L) could induce hepatic steatosis in BaLB/c mice, in a dose-dependent manner (45). However, higher iodine intake was found to benefit lipid metabolism in mice without significant differences observed in thyroid hormone levels and body weights among different groups (46). Iodine deficiency increased fat contribution to energy expenditure through elevated thyrotropin (TSH) in male mice (47). The metabolomics study of the repeated intervention of potassium iodide (KI) on adult male rats indicated a metabolic shift in the thyroid. This shift was also observed in the plasma and urine, and the metabolites were involved in pathways of metabolic regulators, branched-chain amino acids, oxidant stress, and inflammation-associated response (48).
6 The extrathyroidal mechanisms of iodine
Iodine is a micronutrient that possesses antimicrobial properties. Iodine treatment in obese mice showed a weight-reducing effect and modified the gut microbiota, leading to an increase in pathogenic bacteria and a decrease in beneficial bacteria. Conversely, contrasting response patterns were observed in mice with normal weight (49). Another study found a significant relationship between the use of vulvar povidone iodine disinfection and the colonization of neonatal oral microbiota (50). Additionally, the intestinal microbiota also contributed to iodine absorption (51). Therefore, it is feasible that iodine has an impact on metabolism by altering the microbiota.
There is considerable evidence indicating that iodine has extrathyroidal effects as an antioxidant, especially in breast diseases and certain tumors (14). Iodide has been found to be highly efficient in scavenging reactive oxygen species (ROS), thus reducing damage caused by free oxygen radicals (52). In lactating women, the iodine content in breast milk exhibits a negative correlation with the activity of catalase, superoxide dismutase (SOD), and glutathione peroxidase (GSH-Px), as well as adiponectin levels (53). As an obesity-related hormone, adiponectin also plays a crucial role in regulating insulin sensitivity. Seaweed supplementation increased antioxidant enzyme activities in a clinical trial of Korean patients with T2DM (38). Administering an iodide supplement between 100 and 300 μg/d increased the total antioxidant status in human serum (54). However, a recently published study showed that excessive iodine levels lead to cell growth inhibition, oxidative stress, and cellular apoptosis in pancreatic beta cells (55). By regulating oxidative status, iodine is associated with changes in insulin sensitivity or metabolism.
Chronic inflammatory condition paves the way for the development of metabolic disorders. Iodine also has well-known anti-inflammatory and immunomodulatory effects. Both PENDRIN and NIS were expressed on the surface of human leukocytes. The application of sodium iodide (NaI) to leukocytes resulted in a significant rise in the production of both pro- and anti-inflammatory cytokines (56). Administering an iodide supplement had a slight effect on the plasma concentration of inflammation markers and acute-phase proteins (54). Orally administered potassium iodide (15 mg/kg/day for 3 days) significantly inhibited the neutrophil chemotaxis in peripheral blood (57). Fernando et al. provided a summary of the current understanding regarding the potential anti-inflammatory properties of marine algae derivatives (58). They have been shown to reduce inflammation by targeting various cellular mechanisms, such as inhibiting pro-inflammatory enzymes like cyclooxygenase-2 (COX-2) and inducible nitric oxide synthase (iNOS), modulating mitogen-activated protein kinase (MAPK) pathways, and blocking nuclear factor kappa B (NF-κB) activation (59). MAPK pathway controls cellular growth processes and mitoses. Additionally, it is crucial for insulin resistance (60). NF-κB signaling is particularly relevant in inflammation-related diseases, including metabolic disorders. Therefore, it is plausible that iodine could have an impact on metabolism by modulating chronic inflammation.
I2 exhibited antiproliferative and apoptotic effects in mammary cancer models (61) through generating iodine-containing lipids (6-IL) and increasing peroxisome proliferator activated receptor-γ (PPARγ) expression (62, 63). PPARγ, expressed primarily in adipose tissue, promotes the differentiation of adipocytes, uptake of fatty acids, storage of triglycerides in lipid droplets. It increases insulin sensitivity and glucose metabolism (64). PPARα, PPARβ/δ and PPARγ are the three identified isoforms of PPARs. Table 2 presents the summarized relevant studies on the connection between iodine and PPARs. Additionally, excess iodine administration considerably hindered the activity of type 2 deiodinase (D2) in various organs, such as the pituitary, liver, and kidney (71–73). D2 is responsible for converting T4 to bioactive T3, which in turn promotes adaptive thermogenesis and is involved in weight maintenance (74). Figure 1 provides an overview of the potential mechanisms of iodine on metabolism.
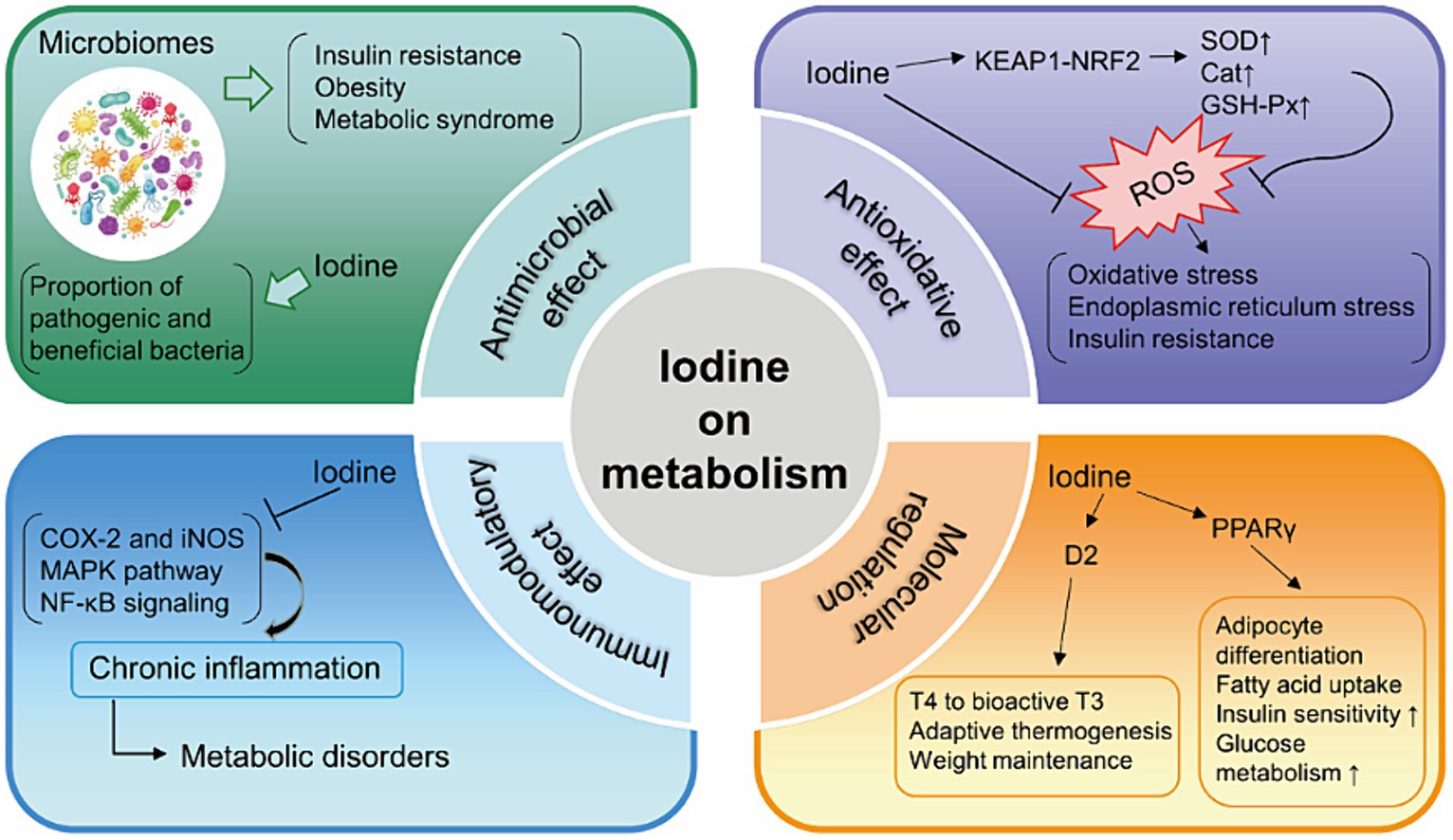
Figure 1. Summary of the mechanisms of iodine on metabolism. KEAP1, Kelch-like ECH-associated protein 1; NRF2, NF-E2-related factor 2; SOD, superoxide dismutase; Cat, catalase; GSH-Px, glutathione peroxidase; ROS, reactive oxygen species; COX-2, cyclooxygenase-2; iNOS, inducible nitric oxide synthase; MAPK, modulating mitogen-activated protein kinase; NF-κB, nuclear factor kappa B; PPARγ, peroxisome proliferator activated receptor-γ; D2, type 2 deiodinase; T4, thyroxine; T3, tri-iodothyronine.
7 Summary
This review validates that iodine has effects on glucose metabolism, lipid metabolism, and obesity. The influence of iodine may be attributed to its antioxidant and immunomodulatory properties. Although the connections between iodine and metabolism are inconsistent, both iodine deficiency and prolonged iodine excess may pose a risk to thyroid disorders. It is important to maintain population iodine status within an optimal range. Further prospective studies and research on mechanisms are needed to establish an evidence-based and safe standard for iodine nutrition.
Author contributions
LZ: Writing – original draft. FS: Writing – original draft. CL: Writing – review & editing. XZ: Writing – review & editing.
Funding
The author(s) declare that no financial support was received for the research, authorship, and/or publication of this article.
Conflict of interest
The authors declare that the research was conducted in the absence of any commercial or financial relationships that could be construed as a potential conflict of interest.
The reviewer FZ declared a shared parent affiliation with the authors to the handling editor at the time of review.
Publisher’s note
All claims expressed in this article are solely those of the authors and do not necessarily represent those of their affiliated organizations, or those of the publisher, the editors and the reviewers. Any product that may be evaluated in this article, or claim that may be made by its manufacturer, is not guaranteed or endorsed by the publisher.
References
1. De la Vieja, A, and Santisteban, P. Role of iodide metabolism in physiology and cancer. Endocr Relat Cancer. (2018) 25:R225–45. doi: 10.1530/erc-17-0515
2. Aceves, C, Mendieta, I, Anguiano, B, and Delgado-González, E. Molecular iodine has Extrathyroidal effects as an antioxidant, differentiator, and Immunomodulator. Int J Mol Sci. (2021) 22:1228. doi: 10.3390/ijms22031228
3. Alberti, KG, Eckel, RH, Grundy, SM, Zimmet, PZ, Cleeman, JI, Donato, KA, et al. Harmonizing the metabolic syndrome: a joint interim statement of the international diabetes federation task force on epidemiology and prevention; National Heart, Lung, and Blood Institute; American Heart Association; world heart federation; international atherosclerosis society; and International Association for the Study of obesity. Circulation. (2009) 120:1640–5. doi: 10.1161/circulationaha.109.192644
4. Saklayen, MG. The global epidemic of the metabolic syndrome. Curr Hypertens Rep. (2018) 20:12. doi: 10.1007/s11906-018-0812-z
5. Rani, V, Deep, G, Singh, RK, Palle, K, and Yadav, UC. Oxidative stress and metabolic disorders: pathogenesis and therapeutic strategies. Life Sci. (2016) 148:183–93. doi: 10.1016/j.lfs.2016.02.002
6. Opazo, MC, Coronado-Arrázola, I, Vallejos, OP, Moreno-Reyes, R, Fardella, C, Mosso, L, et al. The impact of the micronutrient iodine in health and diseases. Crit Rev Food Sci Nutr. (2022) 62:1466–79. doi: 10.1080/10408398.2020.1843398
7. Leung, AM, and Braverman, LE. Consequences of excess iodine. Nat Rev Endocrinol. (2014) 10:136–42. doi: 10.1038/nrendo.2013.251
8. Farebrother, J, Zimmermann, MB, and Andersson, M. Excess iodine intake: sources, assessment, and effects on thyroid function. Ann N Y Acad Sci. (2019) 1446:44–65. doi: 10.1111/nyas.14041
9. World Health Organization, United Nations Children’s Fund, International Council for Control of Iodine Deficiency Disorders (1999) Progress towards the elimination of iodine deficiency disorders (IDD). WHO Booklet. World Health Organization, Geneva, pp. 1–33.
10. Nagataki, S. The average of dietary iodine intake due to the ingestion of seaweeds is 1.2 mg/day in Japan. Thyroid. (2008) 18:667–8. doi: 10.1089/thy.2007.0379
11. Aceves, C, Anguiano, B, and Delgado, G. The extrathyronine actions of iodine as antioxidant, apoptotic, and differentiation factor in various tissues. Thyroid. (2013) 23:938–46. doi: 10.1089/thy.2012.0579
12. Bürgi, H. Iodine excess. Best Pract Res Clin Endocrinol Metab. (2010) 24:107–15. doi: 10.1016/j.beem.2009.08.010
13. Cann, SA, van Netten, JP, and van Netten, C. Hypothesis: iodine, selenium and the development of breast cancer. Cancer Causes Control. (2000) 11:121–7. doi: 10.1023/a:1008925301459
14. Smyth, PP. Role of iodine in antioxidant defence in thyroid and breast disease. Biofactors. (2003) 19:121–30. doi: 10.1002/biof.5520190304
15. Kamangar, F, Dores, GM, and Anderson, WF. Patterns of cancer incidence, mortality, and prevalence across five continents: defining priorities to reduce cancer disparities in different geographic regions of the world. J Clin Oncol. (2006) 24:2137–50. doi: 10.1200/jco.2005.05.2308
16. Inoue, K, Leung, AM, Sugiyama, T, Tsujimoto, T, Makita, N, Nangaku, M, et al. Urinary iodine concentration and mortality among U.S. adults. Thyroid. (2018) 28:913–20. doi: 10.1089/thy.2018.0034
17. Maldonado-Araque, C, Valdés, S, Badía-Guillén, R, Lago-Sampedro, A, Colomo, N, Garcia-Fuentes, E, et al. Iodine deficiency and mortality in Spanish adults: Di@bet.es study. Thyroid. (2021) 31:106–14. doi: 10.1089/thy.2020.0131
18. Mancini, FR, Rajaobelina, K, Dow, C, Habbal, T, Affret, A, Balkau, B, et al. High iodine dietary intake is associated with type 2 diabetes among women of the E3N-EPIC cohort study. Clin Nutr. (2019) 38:1651–6. doi: 10.1016/j.clnu.2018.08.015
19. Park, JK, Woo, HW, Kim, MK, Shin, J, Lee, YH, Shin, DH, et al. Dietary iodine, seaweed consumption, and incidence risk of metabolic syndrome among postmenopausal women: a prospective analysis of the Korean multi-rural communities cohort study (MRCohort). Eur J Nutr. (2021) 60:135–46. doi: 10.1007/s00394-020-02225-0
20. Neven, KY, Cox, B, Cosemans, C, Gyselaers, W, Penders, J, Plusquin, M, et al. Lower iodine storage in the placenta is associated with gestational diabetes mellitus. BMC Med. (2021) 19:47. doi: 10.1186/s12916-021-01919-4
21. Lee, KW, Shin, D, and Song, WO. Low urinary iodine concentrations associated with dyslipidemia in US adults. Nutrients. (2016) 8:171. doi: 10.3390/nu8030171
22. Wang, X, Xian, T, Zhang, L, Jia, X, Man, F, Liu, L, et al. Associations between urinary iodine concentration, lipid profile and other cardiometabolic risk factors in adolescents: a cross-sectional, population-based analysis. Br J Nutr. (2019) 121:1039–48. doi: 10.1017/s0007114518003860
23. Liu, J, Liu, L, Jia, Q, Zhang, X, Jin, X, and Shen, H. Effects of excessive iodine intake on blood glucose, blood pressure, and blood lipids in adults. Biol Trace Elem Res. (2019) 192:136–44. doi: 10.1007/s12011-019-01668-9
24. Jin, M, Zhang, Z, Li, Y, Teng, D, Shi, X, Ba, J, et al. U-shaped associations between urinary iodine concentration and the prevalence of metabolic disorders: a cross-sectional study. Thyroid. (2020) 30:1053–65. doi: 10.1089/thy.2019.0516
25. Lu, X, Shi, X, Li, Y, Chi, H, Liao, E, Liu, C, et al. A negative association between urinary iodine concentration and the prevalence of hyperuricemia and gout: a cross-sectional and population-based study in mainland China. Eur J Nutr. (2020) 59:3659–68. doi: 10.1007/s00394-020-02199-z
26. Zhao, J, Su, Y, Zhang, JA, Fang, M, Liu, X, Jia, X, et al. Inverse association between iodine status and prevalence of metabolic syndrome: a cross-sectional population-based study in a Chinese moderate iodine intake area. Diabetes Metab Syndr Obes. (2021) 14:3691–701. doi: 10.2147/dmso.s322296
27. Ezemaduka Okoli, CB, Woldu, HG, and Peterson, CA. Low urinary iodine concentration is associated with increased risk for elevated plasma glucose in females: an analysis of NHANES 2011–12. Nutrients. (2021) 13:4523. doi: 10.3390/nu13124523
28. Wang, D, Wan, S, Liu, P, Meng, F, Ren, B, Qu, M, et al. Associations between water iodine concentration and the prevalence of dyslipidemia in Chinese adults: a cross-sectional study. Ecotoxicol Environ Saf. (2021) 208:111682. doi: 10.1016/j.ecoenv.2020.111682
29. Wang, D, Wan, S, Liu, P, Meng, F, Zhang, X, Ren, B, et al. Relationship between excess iodine, thyroid function, blood pressure, and blood glucose level in adults, pregnant women, and lactating women: a cross-sectional study. Ecotoxicol Environ Saf. (2021) 208:111706. doi: 10.1016/j.ecoenv.2020.111706
30. Villatoro-Santos, CR, Ramirez-Zea, M, and Villamor, E. Urinary sodium, iodine, and volume in relation to metabolic syndrome in Mesoamerican children and their parents. Nutr Metab Cardiovasc Dis. (2022) 32:1774–83. doi: 10.1016/j.numecd.2022.04.022
31. Kwak, J, Hong, G, Lee, KJ, Kim, CG, and Shin, D. Effect of the interaction between seaweed intake and LPL polymorphisms on metabolic syndrome in middle-aged Korean adults. Nutrients. (2023) 15:2066. doi: 10.3390/nu15092066
32. Shan, X, Liu, C, Luo, X, Zou, Y, Huang, L, Zhou, W, et al. Iodine nutritional status and related factors among Chinese school-age children in three different areas: a cross-sectional study. Nutrients. (2021) 13:1404. doi: 10.3390/nu13051404
33. Lecube, A, Zafon, C, Gromaz, A, Fort, JM, Caubet, E, Baena, JA, et al. Iodine deficiency is higher in morbid obesity in comparison with late after bariatric surgery and non-obese women. Obes Surg. (2014) 25:85–9. doi: 10.1007/s11695-014-1313-z
34. Aoe, S, Yamanaka, C, Ohtoshi, H, Nakamura, F, and Fujiwara, S. Effects of daily kelp (Laminaria japonica) intake on body composition, serum lipid levels, and thyroid hormone levels in healthy Japanese adults: a randomized, double-blind study. Mar Drugs. (2021) 19:352. doi: 10.3390/md19070352
35. Hitoe, S, and Shimoda, H. Seaweed fucoxanthin supplementation improves obesity parameters in mild obese Japanese subjects. Funct Foods Health Dis. (2017) 7:246–62. doi: 10.31989/ffhd.v7i4.333
36. Beer, RJ, Herrán, OF, and Villamor, E. Median urinary iodine concentration in Colombian children and women is high and related to sociodemographic and geographic characteristics: results from a nationally representative survey. J Nutr. (2021) 151:940–8. doi: 10.1093/jn/nxaa392
37. Al-Attas, O, Al-Daghri, N, Alkharfy, K, Alokail, M, Al-Johani, N, Abd-Alrahman, S, et al. Urinary iodine is associated with insulin resistance in subjects with diabetes mellitus type 2. Exp Clin Endocrinol Diabetes. (2012) 120:618–22. doi: 10.1055/s-0032-1323816
38. Kim, MS, Kim, JY, Choi, WH, and Lee, SS. Effects of seaweed supplementation on blood glucose concentration, lipid profile, and antioxidant enzyme activities in patients with type 2 diabetes mellitus. Nutr Res Pract. (2008) 2:62–7. doi: 10.4162/nrp.2008.2.2.62
39. Bell, GA, Männistö, T, Liu, A, Kannan, K, Yeung, EH, Kim, UJ, et al. The joint role of thyroid function and iodine concentration on gestational diabetes risk in a population-based study. Acta Obstet Gynecol Scand. (2019) 98:500–6. doi: 10.1111/aogs.13523
40. Cuellar-Rufino, S, Navarro-Meza, M, García-Solís, P, Xochihua-Rosas, I, and Arroyo-Helguera, O. Iodine levels are associated with oxidative stress and antioxidant status in pregnant women with hypertensive disease. Nutr Hosp. (2017) 34:661–6. doi: 10.20960/nh.460
41. Hata, Y, Nakajima, K, Uchida, J-I, Hidaka, H, and Nakano, T. Clinical effects of brown seaweed, Undaria pinnatifida (wakame), on blood pressure in hypertensive subjects. J Clin Biochem Nutr. (2001) 30:43–53. doi: 10.3164/jcbn.30.43
42. Herter-Aeberli, I, Cherkaoui, M, El Ansari, N, Rohner, R, Stinca, S, Chabaa, L, et al. Iodine supplementation decreases hypercholesterolemia in iodine-deficient, overweight women: a randomized controlled trial. J Nutr. (2015) 145:2067–75. doi: 10.3945/jn.115.213439
43. Shin, D, Shim, SR, Wu, Y, Hong, G, Jeon, H, Kim, CG, et al. How do Brown seaweeds work on biomarkers of dyslipidemia? A systematic review with Meta-analysis and Meta-regression. Mar Drugs. (2023) 21:220. doi: 10.3390/md21040220
44. Kroupova, V, Kratochvil, P, Kaufmann, S, Kursa, J, and Travnicek, J. Metabolic effect of iodine addition in laying hens. Vet Med. (2000) 43:207–12.
45. Xia, Y, Qu, W, Zhao, LN, Han, H, Yang, XF, Sun, XF, et al. Iodine excess induces hepatic steatosis through disturbance of thyroid hormone metabolism involving oxidative stress in BALB/c mice. Biol Trace Elem Res. (2013) 154:103–10. doi: 10.1007/s12011-013-9705-9
46. Zhao, SJ, Ye, Y, Sun, FJ, Tian, EJ, and Chen, ZP. The impact of dietary iodine intake on lipid metabolism in mice. Biol Trace Elem Res. (2011) 142:581–8. doi: 10.1007/s12011-010-8767-1
47. Bocco, B, Fernandes, GW, Fonseca, TL, and Bianco, AC. Iodine deficiency increases fat contribution to energy expenditure in male mice. Endocrinology. (2020) 161:bqaa192. doi: 10.1210/endocr/bqaa192
48. Rosique, C, Lebsir, D, Benatia, S, Guigon, P, Caire-Maurisier, F, Benderitter, M, et al. Metabolomics evaluation of repeated administration of potassium iodide on adult male rats. Arch Toxicol. (2020) 94:803–12. doi: 10.1007/s00204-020-02666-w
49. Shen, H, Han, J, Li, Y, Lu, C, Zhou, J, Li, Y, et al. Different host-specific responses in thyroid function and gut microbiota modulation between diet-induced obese and normal mice given the same dose of iodine. Appl Microbiol Biotechnol. (2019) 103:3537–47. doi: 10.1007/s00253-019-09687-1
50. Li, H, Chen, S, Wu, L, Wang, H, Xiao, K, Gao, Y, et al. The effects of perineal disinfection on infant's oral microflora after transvaginal examination during delivery. BMC Pregnancy Childbirth. (2019) 19:213. doi: 10.1186/s12884-019-2350-3
51. Vought, RL, Brown, FA, Sibinovic, KH, and McDaniel, EG. Effect of changing intestinal bacterial flora on thyroid function in the rat. Horm Metab Res. (1972) 4:43–7. doi: 10.1055/s-0028-1094095
52. Küpper, FC, Carpenter, LJ, McFiggans, GB, Palmer, CJ, Waite, TJ, Boneberg, EM, et al. Iodide accumulation provides kelp with an inorganic antioxidant impacting atmospheric chemistry. Proc Natl Acad Sci USA. (2008) 105:6954–8. doi: 10.1073/pnas.0709959105
53. Gutierrez-Repiso, C, Velasco, I, Garcia-Escobar, E, Garcia-Serrano, S, Rodriguez-Pacheco, F, Linares, F, et al. Does dietary iodine regulate oxidative stress and adiponectin levels in human breast milk? Antioxid Redox Signal. (2014) 20:847–53. doi: 10.1089/ars.2013.5554
54. Soriguer, F, Gutiérrez-Repiso, C, Rubio-Martin, E, Linares, F, Cardona, I, López-Ojeda, J, et al. Iodine intakes of 100-300 μg/d do not modify thyroid function and have modest anti-inflammatory effects. Br J Nutr. (2011) 105:1783–90. doi: 10.1017/S0007114510005568
55. Arely, RJ, Cristian, AE, Omar, AX, Antonio, PJ, Isela, SR, Yeimy Mar, LR, et al. Iodine promotes glucose uptake through Akt phosphorylation and Glut-4 in adipocytes, but higher doses induce cytotoxic effects in pancreatic Beta cells. Biology (Basel). (2024) 13:26. doi: 10.3390/biology13010026
56. Bilal, MY, Dambaeva, S, Kwak-Kim, J, Gilman-Sachs, A, and Beaman, KD. A role for iodide and thyroglobulin in modulating the function of human immune cells. Front Immunol. (2017) 8:1573. doi: 10.3389/fimmu.2017.01573
57. Honma, K, Saga, K, Onodera, H, and Takahashi, M. Potassium iodide inhibits neutrophil chemotaxis. Acta Derm Venereol. (1990) 70:247–9.1972841. doi: 10.2340/0001555570247249
58. Fernando, IPS, Nah, JW, and Jeon, YJ. Potential anti-inflammatory natural products from marine algae. Environ Toxicol Pharmacol. (2016) 48:22–30. doi: 10.1016/j.etap.2016.09.023
59. Barbalace, MC, Malaguti, M, Giusti, L, Lucacchini, A, Hrelia, S, and Angeloni, C. Anti-inflammatory activities of marine algae in neurodegenerative diseases. Int J Mol Sci. (2019) 20:3061. doi: 10.3390/ijms20123061
60. Schultze, SM, Hemmings, BA, Niessen, M, and Tschopp, O. PI3K/AKT, MAPK and AMPK signalling: protein kinases in glucose homeostasis. Expert Rev Mol Med. (2012) 14:e1. doi: 10.1017/s1462399411002109
61. Mendieta, I, Nuñez-Anita, RE, Nava-Villalba, M, Zambrano-Estrada, X, Delgado-González, E, Anguiano, B, et al. Molecular iodine exerts antineoplastic effects by diminishing proliferation and invasive potential and activating the immune response in mammary cancer xenografts. BMC Cancer. (2019) 19:261. doi: 10.1186/s12885-019-5437-3
62. Nuñez-Anita, RE, Arroyo-Helguera, O, Cajero-Juárez, M, López-Bojorquez, L, and Aceves, C. A complex between 6-iodolactone and the peroxisome proliferator-activated receptor type gamma may mediate the antineoplastic effect of iodine in mammary cancer. Prostaglandins Other Lipid Mediat. (2009) 89:34–42. doi: 10.1016/j.prostaglandins.2009.04.001
63. Nava-Villalba, M, Nuñez-Anita, RE, Bontempo, A, and Aceves, C. Activation of peroxisome proliferator-activated receptor gamma is crucial for antitumoral effects of 6-iodolactone. Mol Cancer. (2015) 14:168. doi: 10.1186/s12943-015-0436-8
64. Montaigne, D, Butruille, L, and Staels, B. PPAR control of metabolism and cardiovascular functions. Nat Rev Cardiol. (2021) 18:809–23. doi: 10.1038/s41569-021-00569-6
65. Tepmongkol, S, Keelawat, S, Honsawek, S, and Ruangvejvorachai, P. Rosiglitazone effect on radioiodine uptake in thyroid carcinoma patients with high thyroglobulin but negative total body scan: a correlation with the expression of peroxisome proliferator-activated receptor-gamma. Thyroid. (2008) 18:697–704. doi: 10.1089/thy.2008.0056
66. Aceves, C, García-Solís, P, Arroyo-Helguera, O, Vega-Riveroll, L, Delgado, G, and Anguiano, B. Antineoplastic effect of iodine in mammary cancer: participation of 6-iodolactone (6-IL) and peroxisome proliferator-activated receptors (PPAR). Mol Cancer. (2009) 8:33. doi: 10.1186/1476-4598-8-33
67. Soriano, O, Delgado, G, Anguiano, B, Petrosyan, P, Molina-Servín, ED, Gonsebatt, ME, et al. Antineoplastic effect of iodine and iodide in dimethylbenz[a]anthracene-induced mammary tumors: association between lactoperoxidase and estrogen-adduct production. Endocr Relat Cancer. (2011) 18:529–39. doi: 10.1530/erc-11-0065
68. Olivo-Vidal, ZE, Rodríguez, RC, and Arroyo-Helguera, O. Iodine affects differentiation and migration process in trophoblastic cells. Biol Trace Elem Res. (2016) 169:180–8. doi: 10.1007/s12011-015-0433-1
69. Bigoni-Ordóñez, GD, Ortiz-Sánchez, E, Rosendo-Chalma, P, Valencia-González, HA, Aceves, C, and García-Carrancá, A. Molecular iodine inhibits the expression of stemness markers on cancer stem-like cells of established cell lines derived from cervical cancer. BMC Cancer. (2018) 18:928. doi: 10.1186/s12885-018-4824-5
70. Moreno-Vega, A, Vega-Riveroll, L, Ayala, T, Peralta, G, Torres-Martel, JM, Rojas, J, et al. Adjuvant effect of molecular iodine in conventional chemotherapy for breast Cancer. Randomized pilot study. Nutrients. (2019) 11:1623. doi: 10.3390/nu11071623
71. Li, N, Jiang, Y, Shan, Z, and Teng, W. Prolonged high iodine intake is associated with inhibition of type 2 deiodinase activity in pituitary and elevation of serum thyrotropin levels. Br J Nutr. (2011) 107:674–82. doi: 10.1017/s0007114511003552
72. Calil-Silveira, J, Serrano-Nascimento, C, Laconca, RC, Schmiedecke, L, Salgueiro, RB, Kondo, AK, et al. Underlying mechanisms of pituitary-thyroid Axis function disruption by chronic iodine excess in rats. Thyroid. (2016) 26:1488–98. doi: 10.1089/thy.2015.0338
73. Sun, X, Zhang, X, Jiang, Y, Bao, S, Shan, Z, and Teng, W. Expression of Iodotyrosine deiodinase in thyroid and other organs in iodine-deficient and iodine-excess rats. Biol Trace Elem Res. (2015) 167:272–9. doi: 10.1007/s12011-015-0328-1
Keywords: iodine, metabolism, obesity, dyslipidemia, antioxidant
Citation: Zhang L, Shang F, Liu C and Zhai X (2024) The correlation between iodine and metabolism: a review. Front. Nutr. 11:1346452. doi: 10.3389/fnut.2024.1346452
Edited by:
Akira Sugawara, Tohoku University, JapanReviewed by:
Fan Zhang, The First Affiliated Hospital of China Medical University, ChinaSonya Vasto, University of Palermo, Italy
Copyright © 2024 Zhang, Shang, Liu and Zhai. This is an open-access article distributed under the terms of the Creative Commons Attribution License (CC BY). The use, distribution or reproduction in other forums is permitted, provided the original author(s) and the copyright owner(s) are credited and that the original publication in this journal is cited, in accordance with accepted academic practice. No use, distribution or reproduction is permitted which does not comply with these terms.
*Correspondence: Xiaodan Zhai, zhaixiaodan0712@126.com
†These authors have contributed equally to this work and share first authorship