- 1Department of Public Health and Community Medicine, Faculty of Medicine, Universitas Sriwijaya, Palembang, Indonesia
- 2Department of Public Health Sciences, Faculty of Public Health, Universitas Sriwijaya, Palembang, Indonesia
- 3Faculty of Health Science, Universitas Pembangunan Nasional Veteran Jakarta, Jakarta, Indonesia
- 4Faculty of Medicine, Universitas Indonesia, Jakarta, Indonesia
- 5Indonesia Health Development Center, Jakarta, Indonesia
- 6Research Center for Molecular Biology Eijkman, National Research and Innovation Agency (BRIN), Cibinong, Indonesia
Background: Prediabetes is defined by blood glucose levels that are higher than normal but below the diagnostic threshold for diabetes. Environmental factors associated with diabetes may contribute to its development through alterations in the gut microbiota. Recent studies suggest that changes in the composition and function of the gut microbiota play a role in the pathogenesis of diabetes mellitus and other metabolic disorders.
Objective: This study aims to systematically examine taxonomic abundance and its implications in the gut microbiota of individuals with prediabetes, identify key dysbiotic patterns, and explore their potential role in inflammation, insulin resistance, and progression to type 2 diabetes.
Methods: We conducted a systematic literature review following PRISMA guidelines. The review included sources from PubMed, ClinicalKey, ScienceDirect, Springer, and Scopus. We retrieved original research articles published in English that focused on prediabetes and gut microbiota from 2015 to the date of our search. Out of 827 full-text articles screened, 6 were selected based on defined inclusion and exclusion criteria.
Results: Dysbiosis of the gut microbiota in prediabetes is characterized by a reduction in butyrate-producing bacteria such as Faecalibacterium prausnitzii and Roseburia, along with an increase in potentially harmful taxa such as Escherichia/Shigella and Prevotella species. This imbalance is associated with systemic inflammation and insulin resistance, evidenced by elevated levels of C-reactive protein (CRP), interleukin-6 (IL-6), tumor necrosis factor-alpha (TNF-α), and lipopolysaccharide-binding protein (LBP). Increased intestinal permeability facilitates the translocation of bacterial components such as lipopolysaccharides (LPS), further linking gut microbiota changes to the development of insulin resistance and type 2 diabetes.
Conclusion: This review highlights the need for further research to explore the potential therapeutic role of gut microbiota in prediabetes.
Systematic Review Registration: Prospero; Identifier CRD42025637369.
Introduction
The human gastrointestinal (GI) tract is one of the largest interfaces (250–400 m2) between the host, environmental factors, and antigens in the human body. Over an average lifetime, approximately 60 tonnes of food pass through the digestive tract, introducing an abundance of microorganisms from the environment that can impact gut integrity (1). Gastrointestinal health in childhood significantly influences immune function and metabolic control, laying the foundation for long-term health outcomes. Gut microbiota and gut function during infancy and early childhood have been associated with improved immune responses, a lower risk of chronic diseases, and better overall wellbeing in adulthood (2). The assemblages of bacteria, archaea, and eukaryotes that colonize the digestive tract are collectively referred to as “gut microbiota.” These microbes have co-evolved with their hosts over thousands of years, forming complex and mutually beneficial relationships (3, 4). The number of microorganisms inhabiting the GI tract has been estimated to exceed 1014, outnumbering human cells by a factor of ten and containing over 100 times the genomic content (microbiome) of the human genome (3, 5). However, recent estimates suggest that the human cell-to-bacterial ratio is closer to 1:1 (6). Due to the significant number of bacterial cells in the body, the host and its microbiota are often considered a “superorganism” (5, 7). Prediabetes is characterized by blood glucose levels that are higher than normal but below the diagnostic threshold for diabetes. Individuals with prediabetes frequently present with overweight and insulin resistance (8).
Insulin resistance is the primary underlying mechanism of metabolic syndrome, diabetes, and prediabetes. It is also a key factor in obesity-related cardiometabolic diseases (9). Previous studies have explored the characteristics of gut microbiota and its role in metabolizing key nutrients in insulin resistance. Carbohydrate metabolism by commensal organisms has been proposed to contribute up to 10% of total host energy, highlighting its potential role in the pathogenesis of prediabetes and obesity (10, 11).
Current methods for diagnosing, screening, and managing prediabetes remain limited due to an incomplete understanding of its pathophysiology. Genetic predisposition plays a role in diabetes risk, but environmental factors such as an unhealthy diet, a sedentary lifestyle, and smoking also contribute. Some diabetes-related environmental risk factors may exert their diabetogenic effects through alterations in the gut microbiota. The gut microbiota has the potential to influence adiposity and glucose metabolism, impacting human health and a variety of diseases. Altered composition and function of the gut microbiota have recently been implicated in the pathogenesis of type 2 diabetes (T2D) and other metabolic disorders (12).
Dysbiosis of the gut microbiota plays a significant role in metabolic disorders through several mechanisms, including increased gut permeability, low-grade endotoxemia, altered short-chain fatty acid (SCFA) production, and disruptions in branched-chain amino acid (BCAA) and bile acid metabolism. Changes in gut microbiota composition and function have been observed in individuals with prediabetes (13, 14). Although numerous studies have examined the relationship between gut microbiota and metabolic disorders, research specifically focusing on prediabetes remains scarce. Prediabetes represents a transitional phase toward T2D, yet the role of gut microbiota—particularly in inflammatory mechanisms and taxonomic abundance—has not been well clarified. Therefore, this review aims to systematically evaluate the correlation between gut microbiota, inflammation, and taxonomic abundance in prediabetes. Understanding these interactions may provide valuable insights into potential therapeutic strategies for preventing T2D. Additionally, diet is a major factor influencing gut microbiota composition, and an increasing number of diet-derived microbial metabolites have been linked to insulin secretion, insulin sensitivity, and the onset of T2D (15, 16).
Materials and methods
Protocol and registration
This systematic review was conducted following the Preferred Reporting Items for Systematic Reviews and Meta-Analyses (PRISMA) guidelines to ensure a structured and transparent approach. The protocol was registered in the International Prospective Register of Systematic Reviews (PROSPERO) under registration number CRD42025637369. A flow diagram will be provided to illustrate the study selection process.
Search strategy and data extraction
We have defined the criteria for considering studies as follows: the PICO(S) framework. The population of interest comprised individuals with prediabetes, while the intervention focused on the composition, diversity, or alterations in gut microbiota. The comparison group included healthy individuals without prediabetes or those with a normal glycemic status. The primary outcomes assessed were inflammatory biomarkers, disease phenotypes related to prediabetes, and the abundance of specific gut microbiota taxa.
A comprehensive literature search was conducted using PubMed, ClinicalKey, ScienceDirect, Springer, and Scopus for studies published between 2015 and 2025. The search strategy incorporated a combination of Medical Subject Headings (MeSH) and free-text terms. The search terms included “prediabetes” OR “pre-diabetic” OR “impaired glucose tolerance” OR “impaired fasting glucose” OR “HbA1c” AND “gut microbiota” OR “gut microbiome” OR “intestinal microbiota” OR “intestinal microbiome” OR “microbiota composition” OR “microbiota diversity” OR “gut dysbiosis” OR “gut bacteria.” To improve specificity, the term “metabolic phenotype” was expanded into more precise concepts, including “glucose metabolism,” “lipid metabolism,” and “energy homeostasis.” Similarly, the terms “obesity,” “insulin resistance,” and “glucose” were further detailed as “body mass index (BMI),” “adiposity,” “hyperinsulinemia,” and “fasting plasma glucose.” Additionally, to capture relevant studies on inflammatory biomarkers, synonyms such as “cytokines,” “chemokines,” “pro-inflammatory markers,” “tumor necrosis factor (TNF),” and “interleukins” were incorporated into the search terms. To enhance search precision, search terms were adapted to meet the specific requirements of each database. A table summarizing the exact search terms, search dates, and databases will be included in the full report.
Data extraction was conducted independently by two reviewers and cross-checked by a third reviewer to ensure accuracy, using a standardized data extraction form to collect key study details. Extracted information included study characteristics such as author, year of publication, study design, and setting; participant characteristics including age, sex, BMI, and diagnostic criteria for prediabetes; microbiota assessment covering specific gut microbiota taxa is analyzed and methods of assessment; inflammatory biomarkers such as cytokines, chemokines, and other inflammation markers were measured; and outcome measures including effect sizes, confidence intervals, and intervention details if applicable. Any disagreements during the extraction process were resolved through discussion or consultation with a fourth reviewer.
Eligibility criteria
The study designs considered for inclusion were observational studies, including cohort, case–control, and cross-sectional studies, as well as intervention studies. Studies were included if they assessed the relationship between gut microbiota and prediabetes, reported specific gut microbiota taxa and their role in prediabetes, and measured inflammatory biomarkers related to gut microbiota and metabolic changes. Additionally, studies were required to define prediabetes using standardized criteria such as HbA1c levels, fasting glucose levels, or the oral glucose tolerance test (OGTT). Only studies published in English were considered. Exclusion criteria included reviews, editorials, case reports, and studies without primary data.
Risk of bias assessment
Accordingly, we extracted information from our chosen articles, which included author and year of publication, study design and settings, and findings. The results were summed up and introduced using a table. Quality assessment was conducted collaboratively by three reviewers until consensus was reached. The Newcastle–Ottawa Scale (NOS) and the Joanna Briggs Institute (JBI) tool were used to assess methodological quality.
Results
Study selection
Our search results identified 827 publications. After screening by title and abstract, a total of 6 studies matched the eligibility criteria. After reading the full text, 6 articles were decided to be included in the study. We used the PRISMA flow diagram for searching and extracting data and presented it on the PRISMA flow diagram (Figure 1).
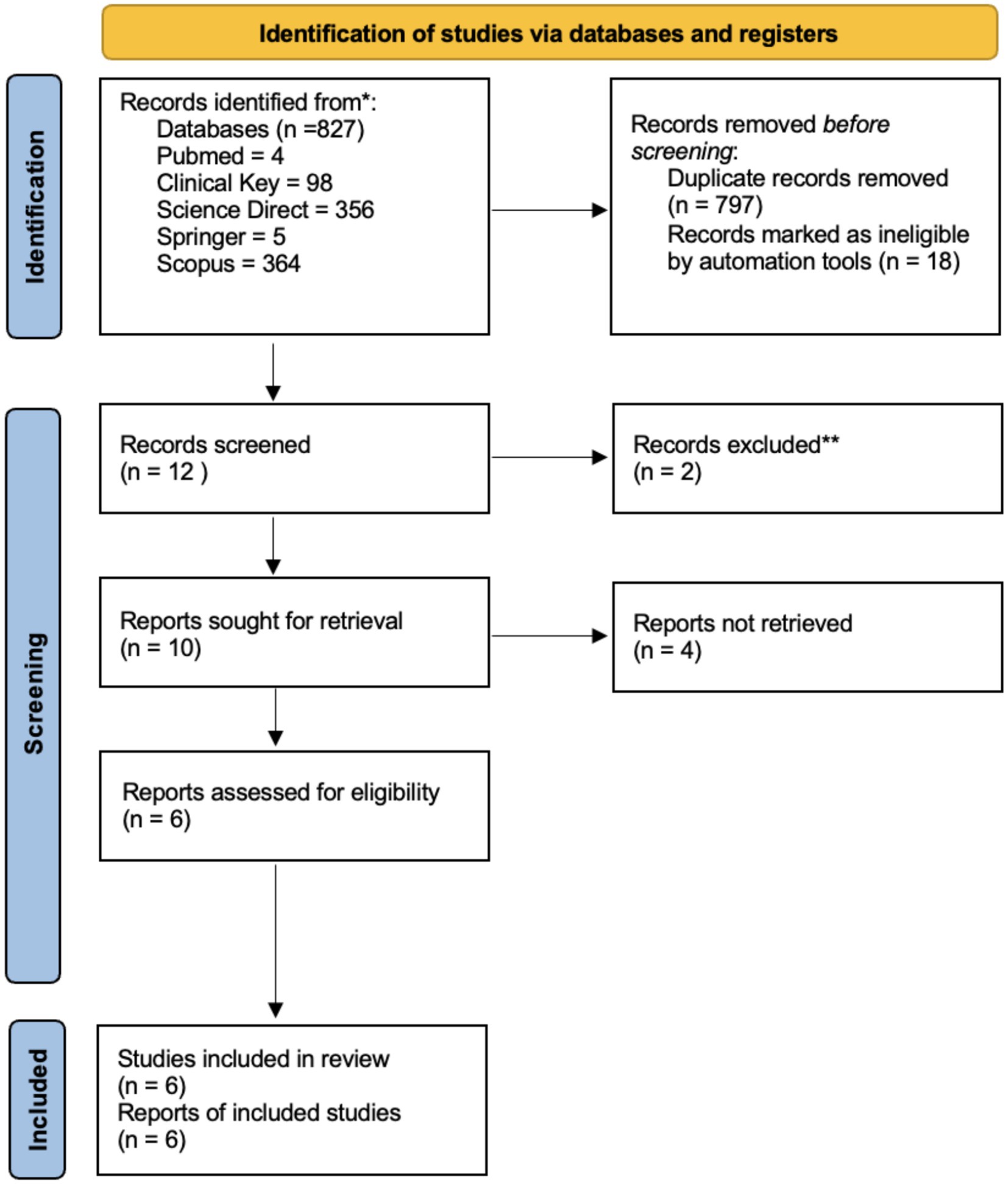
Figure 1. PRISMA flow diagram for the new systematic reviews, which included searches of databases and registers only.
Quality assessment and data synthesis
The selected articles were then assessed for quality and risk of bias using the Newcastle–Ottawa Scale (NOS) and the Joanna Briggs Institute (JBI) tool (Supplementary Table S1). Cross-sectional and case–control studies use the JBI Critical Appraisal Checklist. While, Cohort studies use Newcastle–Ottawa Scale (NOS) Critical Appraisal Checklist. Any discrepancies were addressed until a solution was found by three reviewers.
Study characteristics
The reviewed studies demonstrate significant associations between gut microbiota composition and metabolic conditions, including prediabetes and type 2 diabetes (T2D). The study by Wu et al. (14) indicated that diabetic individuals present differences in their gut microbiota profile from that of prediabetic individuals. T2D individuals with reduced butyrate-producing bacteria were said to correlate these changes with the insulin-resistance condition. Similarly, Pinna et al. (12), while working with examination, reported a particular operational taxonomic unit (OTU) occurring in normoglycemia—for example, described by Prevotella 9—and another OTU occurring in prediabetes—indicative of, say, Streptococcus—with peculiar inflammation-related patterns of microbiota in an Indian population. Increases in prediabetes were identified to be bacterial taxa such as Dorea and Ruminococcus by Allin et al. Meanwhile, Akkermansia muciniphila was notably reduced. Higher insulin-resistance association was found by Takeuchi et al. (11) for bacterial families such as Lachnospiraceae, whereas more fecal carbohydrate levels were again associated with inflammation. Exercise and weight loss, though, added to these similar microbiota effects over diet-based interventions, as pointed out by Beals et al. (8). Finally, altered microbiota, especially lower short-chain fatty acid producers, is demonstrated in T2D but not in prediabetes, suggesting that these changes occur at later disease stages. All these findings indicate the importance of gut microbiota in metabolic dysregulation, inflammation, and possible therapeutic strategies for the management of prediabetes and T2D (Table 1).
Discussion
Diabetes is a chronic disease caused by an inherited or acquired deficiency in insulin production by the pancreas or the body’s inability to use the insulin it produces adequately (17). The natural history of T2D includes a stage of prediabetes, in which blood glucose levels are higher than normal but not high enough for a diabetes diagnosis. Prevention of the disease is possible at this stage. Several studies with limited sample sizes have reported a possible association between gut microbiome composition and prediabetes (12). Studies have shown diverse associations between the gut microbiome and diabetes in European, American, and Chinese cohorts. However, the specific bacteria associated with diabetes vary across populations (18). In the population-based cross-sectional study by Hao Wu et al. (14), significant changes in the gut microbiota were observed at both compositional and functional levels in diabetes treatment-naive T2D individuals in the discovery cohort. These findings were confirmed in the validation cohort. Importantly, changes in the gut microbiota were already present in individuals with prediabetes but were more pronounced in those with CGI and strongly associated with insulin resistance. This supports the hypothesis that the gut microbiota is a modifiable determinant of T2D closely linked to dietary factors. Future studies should examine whether restoring altered microbial function, such as butyrate production, in individuals with IGT and CGI might help prevent or delay the progression to T2D (14).
Observations from the study by Pinna et al. (12), combined with phenotypic data and inflammatory biomarker levels, suggest that the gut microbiome’s role in the pathophysiology of prediabetes in Indian subjects differs from that in European populations. While chronic systemic inflammation is characteristic of the general Indian population, the observed anti-inflammatory and protective effects induced by factors in the Indian gut microbiome appear crucial in determining gut health and modulating diabetes onset and progression. In the study by Allin et al. (8), individuals with prediabetes showed higher fasting plasma glucose, insulin, C-peptide, triacylglycerol, hsCRP, HbA1c, HOMA-IR, BMI, and waist circumference compared to normoglycemic individuals. Although genetic differences and disease phenotype expression exist, microbial composition changes in individuals with prediabetes appear common across diverse pathological phenotypes characterized by low-grade inflammation. The most significant microbial alteration is the depletion of Clostridium and Akkermansia muciniphila (1).
Most studies (Table 2) reveal reduced alpha diversity with the depletion of butyrate-producing bacteria, such as Faecalibacterium prausnitzii and Roseburia, compared to normoglycemic controls. Furthermore, prediabetes is associated with a significant enrichment of Escherichia/Shigella, Streptococcus, Prevotella-2, Vibrio, dan Alloprevotella, with some showing more than a 4-fold increase. These microbial changes correlate with systemic inflammation, elevated IL-6, TNF-α, and CRP, as well as insulin resistance (HOMA-IR). Geographic and dietary factors have influenced the microbiota and shaped unique microbiome profiles related to glucose intolerance. This has resulted in an altered gut microbiota characterized by dysbiosis, low-grade inflammation, and reduced SCFA production, playing a major role in prediabetes pathogenesis.
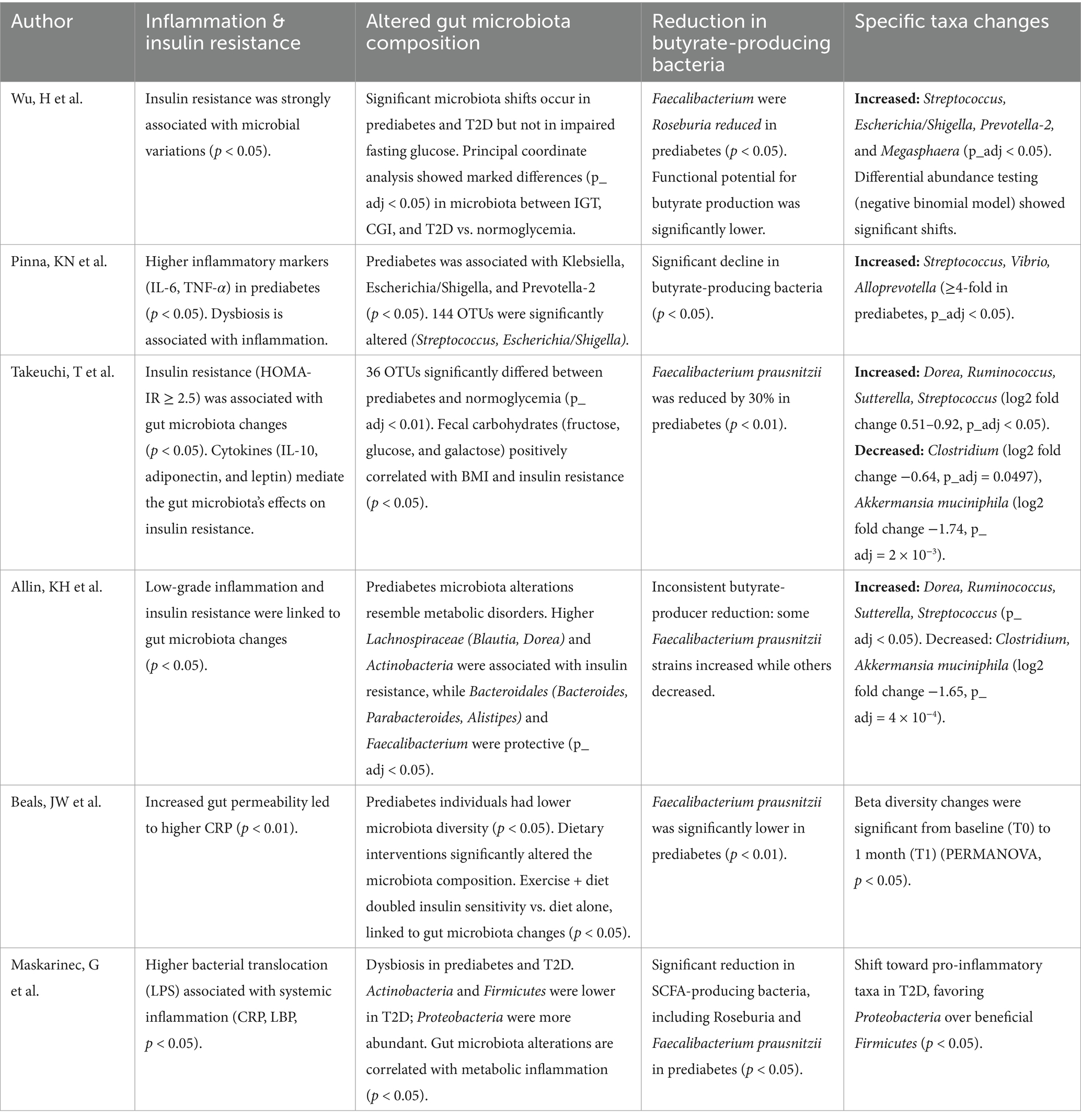
Table 2. Summary of gut microbiota diversity in prediabetes and its association with inflammation and taxonomic abundance.
Metagenomic studies show that approximately 90% of gut bacterial species in adults belong to Bacteroidetes and Firmicutes. Actinobacteria, Proteobacteria, and Verrucomicrobia are present in smaller proportions (18). The composition of the microbiome is influenced by many factors, and while the current study set out to find associations of microbial taxa with glycemic status, several measured covariates, including the subject’s physical/biochemical parameters as well as markers of inflammation, could influence the observed microbiome state (12). In the experimental study by Takeuchi et al. (11), individuals with abundant gut microbiota showed a higher proportion of insulin resistance. Escherichia/Shigella, Streptococcus, and Prevotella-2 showed a more than 4-fold increase in abundance and are known to ferment dietary fiber and produce metabolites such as SCFAs (19). Insulin resistance is also linked to elevated SCFA levels and has an association with its function in gluconeogenesis (20, 21). This study also showed that the end products of carbohydrate degradation, such as monosaccharides, which are readily absorbed and used by humans, are particularly increased in the feces of individuals with insulin resistance and metabolic syndrome. The abundant accumulation of monosaccharides has the potential to promote ectopic lipid accumulation and also trigger immune cells, resulting in low-grade inflammation and insulin resistance. This study also showed that IL-10 has the most prominent associations with fecal carbohydrates and shows its paradoxical effect to facilitate IR. Fructose is recognized as a key contributor to inflammation and insulin resistance due to its role in lipid accumulation, while galactose has a role in activating immune cells in energy metabolism (21–23).
Beals et al. (8) demonstrated that significant alterations in gut microbiota composition are closely linked to conditions such as obesity, metabolic syndrome, and diabetes. Unlike earlier studies that primarily focused on the relationship between gut microbiota and prediabetes, this study explored a novel approach by assessing whether the effects of administering or transplanting gut microbiota from healthy, lean individuals into participants with obesity and metabolic syndrome could enhance insulin sensitivity. Additionally, this study included an analysis of fecal samples collected at baseline. It found that a plant-based diet led to a substantial change in gut microbiota beta diversity, indicating a notable change in microbial composition. However, this study was unable to determine whether these changes in gut microbiota directly contributed to the insulin sensitivity improvements observed in the participants or were simply related to changes in dietary composition, which may not have independently influenced insulin action.
Finally, a cross-sectional study by Maskarinec et al. (16) showed quite different results compared to the studies that had been presented previously. A decrease in SCFA-producing bacteria, such as those producing butyrate, and a rise in Gram-negative, endotoxin-producing bacteria are observed in T2D cases but not in prediabetes cases. Prediabetes values were closer to normoglycemic cases than T2D. This shift might reflect an unfavorable microbiome composition in T2D. These studies are limited by their cross-sectional design, making it difficult to establish causality. Additionally, selection bias may influence the microbiota variations observed in different populations.
Specific genera within Firmicutes and Proteobacteria were found to be significantly more abundant in T2D cases. This indicates that certain bacterial groups may flourish in the altered gut environment associated with T2D. SCFAs have anti-inflammatory and gut barrier-supporting roles. SCFAs, especially butyrate, have been shown to help maintain gut physiologic hypoxia through several mechanisms to maintain tight junctions and gut epithelial integrity (24). Disruption of these pathways—reduced SCFA production, increased gut hypoxia, impaired tight junction integrity, and changes in microbial diversity—can negatively impact host health, suggesting that their reduction may exacerbate gut and systemic inflammation. LPS triggers Toll-like receptor 4 (TLR4) activation, leading to NF-κB pathway stimulation and increased pro-inflammatory cytokine production, contributing to insulin resistance. These alterations may promote systemic inflammation, reduce energy homeostasis, impair insulin sensitivity, and increase the risk of developing or exacerbating T2D (25). Epidemiologic evidence links the gut microbiome to T2D, with reduced butyrate-producing bacteria from the Firmicutes phylum commonly observed in T2D patients and more likely to have a higher presence of opportunistic pathogens (26, 27).
Metabolic endotoxemia refers to persistent, low-grade systemic inflammation linked to obesity and metabolic diseases, including T2D (28). Increased abundance of endotoxin-producing bacteria, particularly in the Escherichia/Shigella group (e.g., Escherichia coli) and other Proteobacteria, has been reported in T2D patients (28, 29). These bacteria contribute to endotoxin production, which is implicated in metabolic dysfunction. Endotoxins can trigger systemic inflammation via bacterial translocation across the gut barrier, a process implicated in metabolic dysregulation. The microbiome changes support the hypothesis that unfavorable bacterial patterns may contribute to T2D by promoting chronic low-grade inflammation (30) (Table 3).
Notably, these patterns were not observed in prediabetic participants, indicating their role may be specific to advanced metabolic dysfunction. But these results emphasize the gut microbiome’s involvement in T2D, providing insights for potential microbiome-targeted interventions to manage or prevent the disease. Specific microbiome signatures, such as the depletion of butyrate-producing Faecalibacterium prausnitzii and Roseburia and increased levels of pro-inflammatory taxa like Escherichia/Shigella and Prevotella-2, may identify at-risk individuals and forecast disease progression. Additional gut-derived metabolites that provide insight into host-microbiome interactions affecting insulin sensitivity and systemic inflammation include SCFAs and endotoxins. Apart from diagnosis, microbiome-targeted interventions, especially dietary changes, probiotics, and fecal microbiota transplantation (FMT), have some promise in restoring microbial imbalance and restricting metabolic health. Future research that links microbiome profiling with genetics, metabolism, and inflammatory markers may use machine learning and multi-omics approaches to improve predictions and personalize treatment strategies for metabolic disorders.
Conclusion
The gut microbiota present aberrancy in prediabetes. In prediabetes, gut microbiota dysbiosis, which consists of reduced butyrate-producing bacteria, contributes to both systemic inflammation and insulin resistance. Restore the microbiota balance, paving the way for microbiome-based treatments to prevent and treat prediabetes.
Data availability statement
The original contributions presented in the study are included in the article/Supplementary material, further inquiries can be directed to the corresponding author.
Author contributions
IL: Conceptualization, Data curation, Formal analysis, Methodology, Writing – original draft, Writing – review & editing. LH: Data curation, Methodology, Writing – original draft. HR: Data curation, Formal analysis, Methodology, Writing – review & editing. AiP: Data curation, Formal analysis, Methodology, Writing – original draft. DP: Writing – review & editing. AnP: Methodology, Resources, Writing – original draft, Writing – review & editing. FI: Methodology, Writing – review & editing. ST: Conceptualization, Formal analysis, Methodology, Writing – original draft.
Funding
The author(s) declare that financial support was received for the research and/or publication of this article. We gratefully acknowledge Danone Specialized Nutrition for giving financial support to publish this manuscript.
Acknowledgments
We gratefully acknowledge Danone Specialized Nutrition for giving financial support to publish this manuscript and the Varians Statistik Kesehatan for providing the boot camp and mentoring to improve the manuscript writing.
Conflict of interest
The authors declare that the research was conducted in the absence of any commercial or financial relationships that could be construed as a potential conflict of interest.
Generative AI statement
The authors declare that no Gen AI was used in the creation of this manuscript.
Publisher’s note
All claims expressed in this article are solely those of the authors and do not necessarily represent those of their affiliated organizations, or those of the publisher, the editors and the reviewers. Any product that may be evaluated in this article, or claim that may be made by its manufacturer, is not guaranteed or endorsed by the publisher.
Supplementary material
The Supplementary material for this article can be found online at: https://www.frontiersin.org/articles/10.3389/fnut.2025.1577528/full#supplementary-material
References
1. Bengmark, S. Ecological control of the gastrointestinal tract. The role of probiotic flora. Gut. (1998) 42:2–7. doi: 10.1136/gut.42.1.2
2. Kadim, M, and Masita, BM. The importance of gut health in early life for long term health. World Nutr J. (2022) 5:1–8. doi: 10.25220/wnj.v05.s2.0001
3. Bäckhed, F, Ley, RE, Sonnenburg, JL, Peterson, DA, and Gordon, JI. Host-bacterial mutualism in the human intestine. Science. (2005) 307:1915–20. doi: 10.1126/science.1104816
4. Neish, AS. Microbes in gastrointestinal health and disease. Gastroenterology. (2009) 136:65–80. doi: 10.1053/j.gastro.2008.10.080
5. Gill, SR, Pop, M, DeBoy, RT, Eckburg, PB, Turnbaugh, PJ, Samuel, BS, et al. Metagenomic analysis of the human distal gut microbiome. Science. (2006) 312:1355–9. doi: 10.1126/science.1124234
6. Sender, R, Fuchs, S, and Milo, R. Revised estimates for the number of human and bacteria cells in the body. PLoS Biol. (2016) 14:e1002533. doi: 10.1371/journal.pbio.1002533
7. Luckey, TD. Introduction to intestinal microecology. Am J Clin Nutr. (1972) 25:1292–4. doi: 10.1093/ajcn/25.12.1292
8. Allin, KH, Tremaroli, V, Caesar, R, Jensen, BAH, Damgaard, MTF, Bahl, MI, et al. Aberrant intestinal microbiota in individuals with prediabetes. Diabetologia. (2018) 61:810–20. doi: 10.1007/s00125-018-4550-1
9. Beals, JW, Kayser, BD, Smith, GI, Schweitzer, GG, Kirbach, K, Kearney, ML, et al. Dietary weight loss-induced improvements in metabolic function are enhanced by exercise in people with obesity and prediabetes. Nat Metab. (2023) 5:1221–35. doi: 10.1038/s42255-023-00829-4
10. Gou, W, Ling, C, He, Y, Jiang, Z, Fu, Y, Xu, F, et al. Interpretable machine learning framework reveals robust gut microbiome features associated with type 2 diabetes. Diabetes Care. (2021) 44:358–66. doi: 10.2337/dc20-1536
11. Takeuchi, T, Kubota, T, Nakanishi, Y, Tsugawa, H, Suda, W, Kwon, AT-J, et al. Gut microbial carbohydrate metabolism contributes to insulin resistance. Nature. (2023) 621:389–95. doi: 10.1038/s41586-023-06466-x
12. Pinna, NK, Anjana, RM, Saxena, S, Dutta, A, Gnanaprakash, V, Rameshkumar, G, et al. Trans-ethnic gut microbial signatures of prediabetes subjects from India and Denmark. Genome Med. (2021) 13:1–20. doi: 10.1186/s13073-021-00851-9
13. Bouter, KE, van Raalte, DH, Groen, AK, and Nieuwdorp, M. Role of the gut microbiome in the pathogenesis of obesity and obesity-related metabolic dysfunction. Gastroenterology. (2017) 152:1671–8. doi: 10.1053/j.gastro.2016.12.048
14. Wu, H, Tremaroli, V, Schmidt, C, Lundqvist, A, Olsson, LM, Krämer, M, et al. The gut microbiota in prediabetes and diabetes: a population-based cross-sectional study. Cell Metab. (2020) 32:379–390.e3. doi: 10.1016/j.cmet.2020.06.011
15. Yamada, C, Mitsuhashi, T, Hiratsuka, N, Inabe, F, Araida, N, and Takahashi, E. Optimal reference interval for homeostasis model assessment of insulin resistance in a Japanese population. J Diabetes Investig. (2011) 2:373–6. doi: 10.1111/j.2040-1124.2011.00113.x
16. Maskarinec, G, Raquinio, P, Kristal, BS, Setiawan, VW, Wilkens, LR, Franke, AA, et al. The gut microbiome and type 2 diabetes status in the multiethnic cohort. PLoS One. (2021) 16:e0250855. doi: 10.1371/journal.pone.0250855
17. World Health Organization. Global reports on diabetes. Geneva: World Health Organization (2016).
18. Diener, C, Reyes-Escogido, M, De, L, Jimenez-Ceja, LM, Matus, M, Gomez-Navarro, CM, et al. Progressive shifts in the gut microbiome reflect prediabetes and diabetes development in a treatment-naive Mexican cohort. Front Endocrinol (Lausanne). (2021) 11:1–13. doi: 10.3389/fendo.2020.602326
19. Gold, A, and Zhu, J. Not just a gut feeling: a deep exploration of functional bacterial metabolites that can modulate host health. Gut Microbes. (2022) 14:2125734. doi: 10.1080/19490976.2022.2125734
20. den Besten, G, Lange, K, Havinga, R, van Dijk, TH, Gerding, A, van Eunen, K, et al. Gut-derived short-chain fatty acids are vividly assimilated into host carbohydrates and lipids. Am J Physiol Gastrointest Liver Physiol. (2013) 305:G900–10. doi: 10.1152/ajpgi.00265.2013
21. Zhang, J. Microbial stars: shedding light on gut microbes’ role in insulin resistance and innovative diabetes therapies. Gut Microbes. (2024) 16:2307581. doi: 10.1080/19490976.2024.2307581
22. Chang, C-H, Curtis, JD, Maggi, LB, Faubert, B, Villarino, AV, O’Sullivan, D, et al. Posttranscriptional control of T cell effector function by aerobic glycolysis. Cell. (2013) 153:1239–51. doi: 10.1016/j.cell.2013.05.016
23. Dekker, MJ, Su, Q, Baker, C, Rutledge, AC, and Adeli, K. Fructose: a highly lipogenic nutrient implicated in insulin resistance, hepatic steatosis, and the metabolic syndrome. Am J Physiol Metab. (2010) 299:E685–94. doi: 10.1152/ajpendo.00283.2010
24. Chapkin, RS, Navarro, SL, Hullar, MAJ, and Lampe, JW. Diet and gut microbes act coordinately to enhance programmed cell death and reduce colorectal Cancer risk. Dig Dis Sci. (2020) 65:840–51. doi: 10.1007/s10620-020-06106-8
25. Zhang, F, Wang, M, Yang, J, Xu, Q, Liang, C, Chen, B, et al. Response of gut microbiota in type 2 diabetes to hypoglycemic agents. Endocrine. (2019) 66:485–93. doi: 10.1007/s12020-019-02041-5
26. Qin, J, Li, Y, Cai, Z, Li, S, Zhu, J, Zhang, F, et al. A metagenome-wide association study of gut microbiota in type 2 diabetes. Nature. (2012) 490:55–60. doi: 10.1038/nature11450
27. Zhang, X, Shen, D, Fang, Z, Jie, Z, Qiu, X, Zhang, C, et al. Human gut microbiota changes reveal the progression of glucose intolerance. PLoS One. (2013) 8:e71108. doi: 10.1371/journal.pone.0071108
28. Boulangé, CL, Neves, AL, Chilloux, J, Nicholson, JK, and Dumas, M-E. Impact of the gut microbiota on inflammation, obesity, and metabolic disease. Genome Med. (2016) 8:42. doi: 10.1186/s13073-016-0303-2
29. Thingholm, LB, Rühlemann, MC, Koch, M, Fuqua, B, Laucke, G, Boehm, R, et al. Obese individuals with and without type 2 diabetes show different gut microbial functional capacity and composition. Cell Host Microbe. (2019) 26:252–264.e10. doi: 10.1016/j.chom.2019.07.004
30. Doumatey, AP, Adeyemo, A, Zhou, J, Lei, L, Adebamowo, SN, Adebamowo, C, et al. Gut microbiome profiles are associated with type 2 diabetes in urban Africans. Front Cell Infect Microbiol. (2020):10. doi: 10.3389/fcimb.2020.00063/full
31. Citronberg, JS, Curtis, KR, White, E, Newcomb, PA, Newton, K, Atkinson, C, et al. Association of gut microbial communities with plasma lipopolysaccharide-binding protein (LBP) in premenopausal women. ISME J. (2018) 12:1631–41. doi: 10.1038/s41396-018-0064-6
32. Salvadori, M, and Rosso, G. Update on the gut microbiome in health and diseases. World J Methodol. (2024) 14:89196. doi: 10.5662/wjm.v14.i1.89196
33. Hakansson, AP, Orihuela, CJ, and Bogaert, D. Bacterial-host interactions: physiology and pathophysiology of respiratory infection. Physiol Rev. (2018) 98:781–811. doi: 10.1152/physrev.00040.2016
34. Hou, K, Wu, ZX, Chen, XY, Wu, ZX, Chen, XY, Wang, JQ, et al. Microbiota in health and diseases. Signal Transduct Target Ther. (2022) 7:135. doi: 10.1038/s41392-022-00974-4
Keywords: prediabetes, gut microbiota, gastrointestinal microbiota, dysbiosis, insulin resistance
Citation: Liberty IA, Hanifah L, Rachmat HF, Putri AA, Pratiwi D, Pramono AP, Idris F and Tjekyan S (2025) Implication of taxonomic abundance of gut microbiota in prediabetes: a systematic review. Front. Nutr. 12:1577528. doi: 10.3389/fnut.2025.1577528
Edited by:
George Grant, Independent Researcher, Aberdeen, United KingdomReviewed by:
Monika Yadav, Sanjay Ghodawat University, IndiaRicha Dwivedi, Meharry Medical College, United States
Copyright © 2025 Liberty, Hanifah, Rachmat, Putri, Pratiwi, Pramono, Idris and Tjekyan. This is an open-access article distributed under the terms of the Creative Commons Attribution License (CC BY). The use, distribution or reproduction in other forums is permitted, provided the original author(s) and the copyright owner(s) are credited and that the original publication in this journal is cited, in accordance with accepted academic practice. No use, distribution or reproduction is permitted which does not comply with these terms.
*Correspondence: Iche Andriyani Liberty, aWNoZWFuZHJpeWFuaWxpYmVydHlAZmsudW5zcmkuYWMuaWQ=
†ORCID: Iche Andriyani Liberty, http://orcid.org/0000-0001-7697-6593