- Department of Archaeology, Tel Aviv University, Tel Aviv, Israel
Introduction: The use of fire marks a critical milestone in human evolution, with its initial purposes debated among scholars. While cooking is often cited as the primary driver, this study proposes that meat and fat preservation, and predator protection were more likely the initial motivations for fire use by Homo erectus during the Lower Paleolithic (1.9–0.78 Ma).
Methods: Employing a bioenergetic approach, we compared the energetic returns of hunting versus plant gathering using ethnographic data, adjusted for Lower Paleolithic conditions. Caloric content of East African prey was calculated to assess consumption duration. Archeological evidence from early fire sites was analyzed for associations with large fauna.
Results: Hunting large prey (>100 kg) yielded significantly higher energetic returns (16,269 ca/h) than plant gathering (1,443 ca/h), with megaherbivores like hippopotamus providing sustenance for up to 22 days for a group of 25. Early fire sites consistently contained large fauna remains, suggesting prolonged prey consumption. Cooking offered modest energetic gains (e.g., ~1,200 ca/h for meat), insufficient to offset fire maintenance costs, unlike preservation and protection.
Discussion: The substantial energetic disparity supports hunting as a dominant subsistence strategy, with fire enhancing efficiency by preserving meat and deterring predators. The prevalence of megaherbivores in Lower Paleolithic sites and heightened predation risks underscore these priorities over cooking, which likely emerged as a secondary benefit. Ethnographic analogies underrepresent these dynamics due to megafaunal extinctions altering the environment and prey availability.
Conclusion: Meat preservation and predator protection, rather than cooking, were likely the primary drivers of early fire use, aligning with Homo erectus’ specialization in large prey acquisition. This reframes fire’s role in human evolution, suggesting it supported a hypercarnivorous lifestyle and potentially influenced cognitive development.
1 Introduction
The utilization of fire stands as a pivotal technological advancement in human history. Some scholars argue that rather than a sudden discovery, the mastery of fire’s control and application for human needs occurred gradually, with its origins dating back to the early Pleistocene (1–3). Substantial evidence supports the early use of fire by our ancestors during Lower Paleolithic times, starting about 1 million years before the present (4–9), although opposing perspectives exists (10). The significance of fire use in reconstructing early human adaptation strategies and capabilities holds paramount importance within Pleistocene archeology.
Wrangham, Jones (11, 12) hypothesized that the control of fire for cooking plants and meat was a driving force behind crucial adaptations in Homo erectus, including a reduction in digestive system size and an increase in brain size. This hypothesis, known as ‘the cooking hypothesis,’ has been a subject of debate and inquiry (2, 13–17).
The findings by Henry (18), and Henry, Büdel (19) that in some circumstances, the production of fire incurs higher bioenergetic costs than the benefits of cooking encourage reinvestigation of the reason for the use of fire by early to middle Lower Paleolithic humans. Assuming that fire was produced and maintained in the Lower Paleolithic, there is a need to consider alternative utilities or set of utilities that have rendered the acquisition and maintenance of fire economically profitable.
We employ a bioenergetic approach to explore the relative likelihood of cooking, meat and fat preservation, and protection from predators as drivers for the early use of fire. Based on accepted evidence of fire in archeological sites, we define the early use of fire as spanning from 1.9 to 0.78 million years ago (Ma), with relevance to Homo erectus (sensu lato).
2 Materials and methods
The need to preserve and protect acquired prey arises when it is large enough to provide food for the group for several days. The extent of human involvement in acquiring large prey remains a subject of ongoing debate within the scholarly community [(e.g., 20, 21)]. However, for this study, it suffices to acknowledge that early humans, specifically Homo erectus, did consume meat and fat of large prey. Lower Paleolithic sites are notably characterized by a substantial presence of megaherbivores, and other large animals’ remains, some of which exhibit cut marks as well as other evidence of human manipulation [(e.g., 22–24)]. Smaller animal taxa were also found at Lower Paleolithic sites. However, it was demonstrated that the caloric contribution of megaherbivores was unprecedented [(e.g., 25, 26)].
The relevance of ethnographic data to Lower Paleolithic circumstances is contested (27–29). In many cases, the basic preconditions for analogy of similarity in technological and environmental circumstances are unmet, specifically as they relate to large herbivores exploitation (30).
To arrive at relative energetic returns of gathering versus hunting, we used only the closest analogous available ethnographic data to the early to middle Lower Paleolithic circumstances. We based our calculations on data assembled by Kelly (31) (Table 4–5) and Morin, Bird (32) (Supplementary ESM4).
Kelly (31) dataset comprises 105 data points detailing energetic returns on plant gathering and 25 data points of hunting medium-sized prey as no data on large prey exists. Notably, the dataset does not specify the weight of the animals in question. In our analysis, we classified medium-sized animals as roughly equivalent to the size of sheep and larger.
Morin, Bird (32) dataset encompassed 129 data points. It encompasses returns derived from various hunting methods, including using guns, dogs, traps, and bow/spear, across multiple biomes, including the rainforest biome. It encompasses records of hunting a diverse range of fauna, from birds, reptiles, and rodents to larger prey. We computed average returns to ensure our results align as closely as possible with the early to middle Lower Paleolithic context. In Morin, Bird (32) dataset, hunting with firearms yielded returns above the average, while hunting in rainforests yielded returns much below the average, showing that technology and environment are substantial factors in generating energetic returns. Consequently, we calculated the average returns within Morin, Bird (32) dataset as follows:
1. The average return of the dataset (N = 129)
2. The average return on hunting with bows/spears (N = 38)
3. The average return on hunting prey, larger than 100 kgs in a non-rainforest biome with bows and spears (N = 8). We consider this sample to be the closest to Lower Paleolithic conditions.
To ascertain the period of consumption that prey provides to a typical group of 25 individuals (33), we calculated the caloric content of typical East African prey animals. The raw data comes from Ledger (34), who dissected 252 East African herbivores of 16 species.
In the computation of averages within category 3 (prey >100 kg, non-rainforest), sourced from the Morin, Bird (32) table, we deliberately excluded prey weighing less than 100 kilograms. This exclusion is justified because prey in the 100-kilogram range typically contains approximately 60,000 calories, which a group of hunter-gatherers can reasonably consume in a single day. In stark contrast, a single 1.5-ton Hippopotamus, yielding about a million calories, can sustain such a group for 22 days (Table 1). Consequently, the relative economic significance of protection from predators and preservation in the context of medium-small prey would have been considerably lower, compared to larger prey.
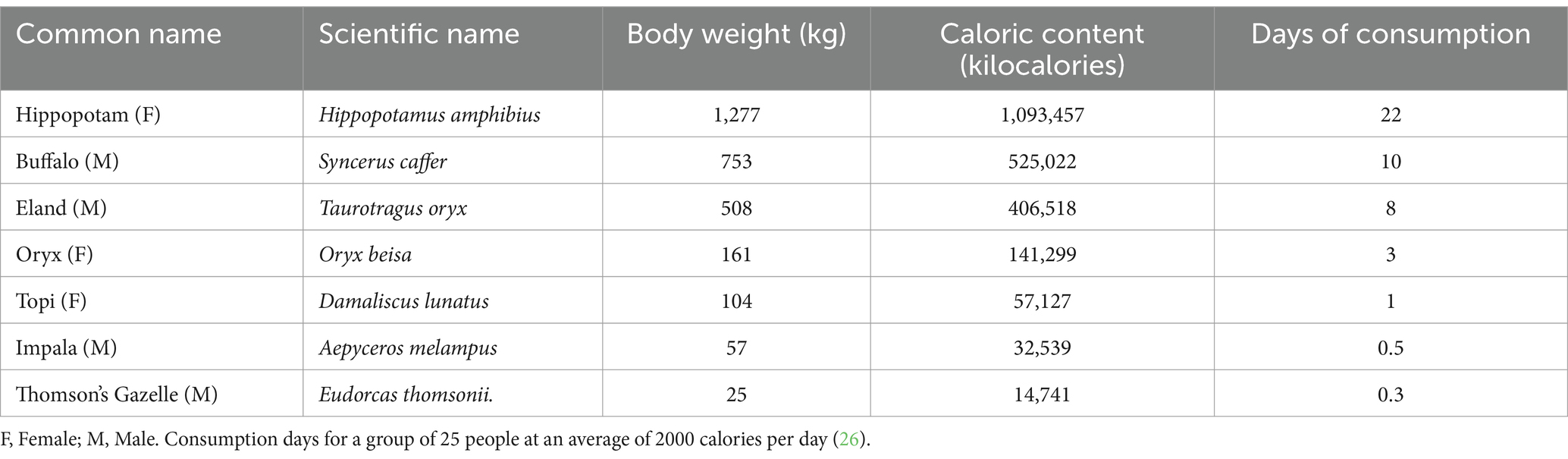
Table 1. Caloric content of selected East African prey [based on data in (34); see Supplementary material].
While it is true that bows were not accessible to hunters in the early to middle Lower Paleolithic, energetic return data exclusively focusing on spear employment is notably absent. Furthermore, the other categories encompassed within the hunting technology parameter, namely guns, held even less relevance in hunting large prey during the early to middle Lower Paleolithic. Mass hunting, traps, and hand digging, the remaining categories, were predominantly employed for hunting small animals in the dataset, rendering them equally irrelevant in the analogy.
3 Results
3.1 Days of storage of prey by size
To demonstrate the relative duration of the predation risk and preservation need, we calculated in Table 1 the days of subsistence that prey in Ledger (34) dataset would provide a group with 25 members (26). Early Lower Paleolithic elephants (Elephas racki) could weigh 10 tons, so several times the heaviest animal in the data set (Hippopotamus amphibius at 1.4 tons), so they would have provided meat and fat for a much longer period. However, the hunting of large prey motivated several contemporary hunting groups to consolidate at the hunting site (35), so by analogy, the figures might have been lower than calculated but in any case, remain high.
3.2 The difference between hunting and plant gathering returns
Table 2 presents the average bioenergetic return derived from plant gathering and prey hunting, quantified in calories per hour (ca/h) and the corresponding 95% confidence intervals (95% CI). It is evident from Table 2 that hunting in a rainforest biome yields a substantially lower average return of 3,342 ca/h, albeit still double that of plant gathering. Plant gathering yields an average return of 1,443 ca/h with minimal disparity in the returns from various plant foods, such as seeds and tubers, as indicated by the narrow 95% confidence interval range of 1,025–1861 ca/h. It is less than one tenth of the return of 16,269 calories per hour on hunting >100 kgs prey with bow and arrows in non-rainforest biomes. It also less than one tenth of the average of the energetic return on the whole dataset of 14,877 calories per hour.
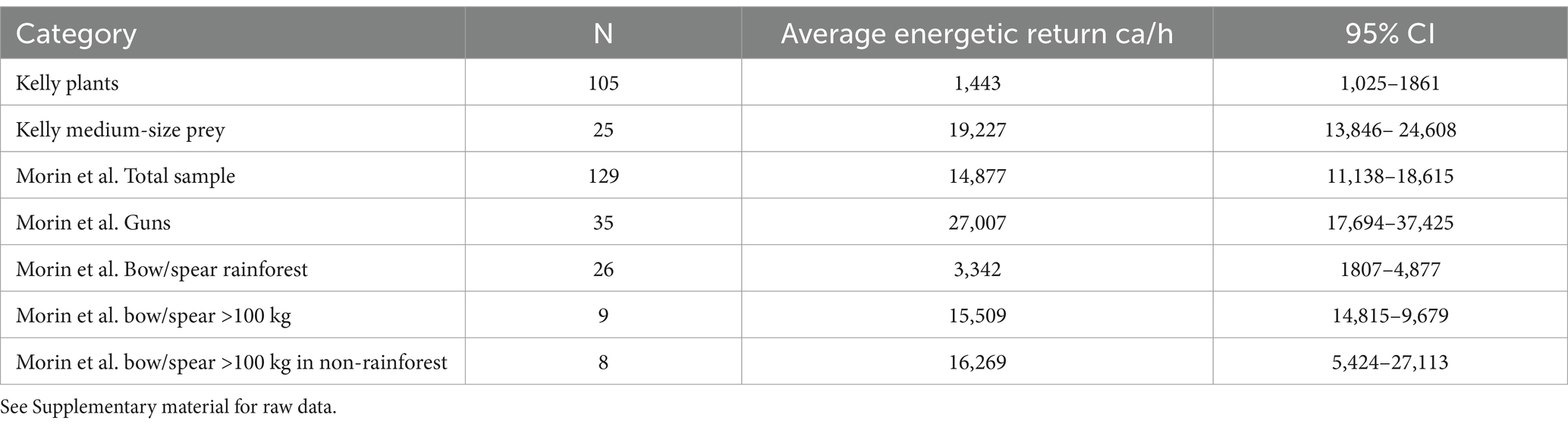
Table 2. Average energetic returns (calories per hour) from plant gathering and prey hunting (31, 32).
3.3 The association between fire remains and large prey
In an ideal scenario, our approach would involve compiling a list of early and middle Lower Paleolithic sites featuring large fauna and examining the presence or absence of fire in these archeological contexts. However, undertaking such an endeavor would prove futile due to the significantly poorer preservation of fire evidence than faunal remains. This challenge is particularly pronounced in open-air sites, which predominantly characterize the early to middle Lower Paleolithic period, as opposed to cave sites which usually reveal better fire preservation (14, 36). Following these preservation limitations, we have assembled in Table 3 a list of all reported earliest sites (dating back to before 0.78 million years ago) where fire has been claimed to be present and for which a faunal record is available. Remarkably, our examination of these sites reveals a consistent pattern—all contain remains of very large herbivores. It should be noted that early fire does not lend itself easily to be identified, so it might be the case that other Lower Paleolithic sites bearing large mammals did contain a fire that was not recognized. Under different circumstances, as a different number of individual prey animals hunted, the size of the group, and the time spent at the site, different decisions regarding the use of fire would have been taken. We suggest that in cases where the consumption of megaherbivores was planned for a prolonged duration, the use of fire was highly likely to preserve meat and fat and to keep predators away (37). Interestingly, Bellomo (38) (p. 194) analyzed the potential use of fire in Koobi Fora FXJ20 Main and after eliminating several uses states: “It is most likely that the early hominids at FxJj 20 Main primarily used fire as a source of protection, a source of light, and/or as a source of heat.”
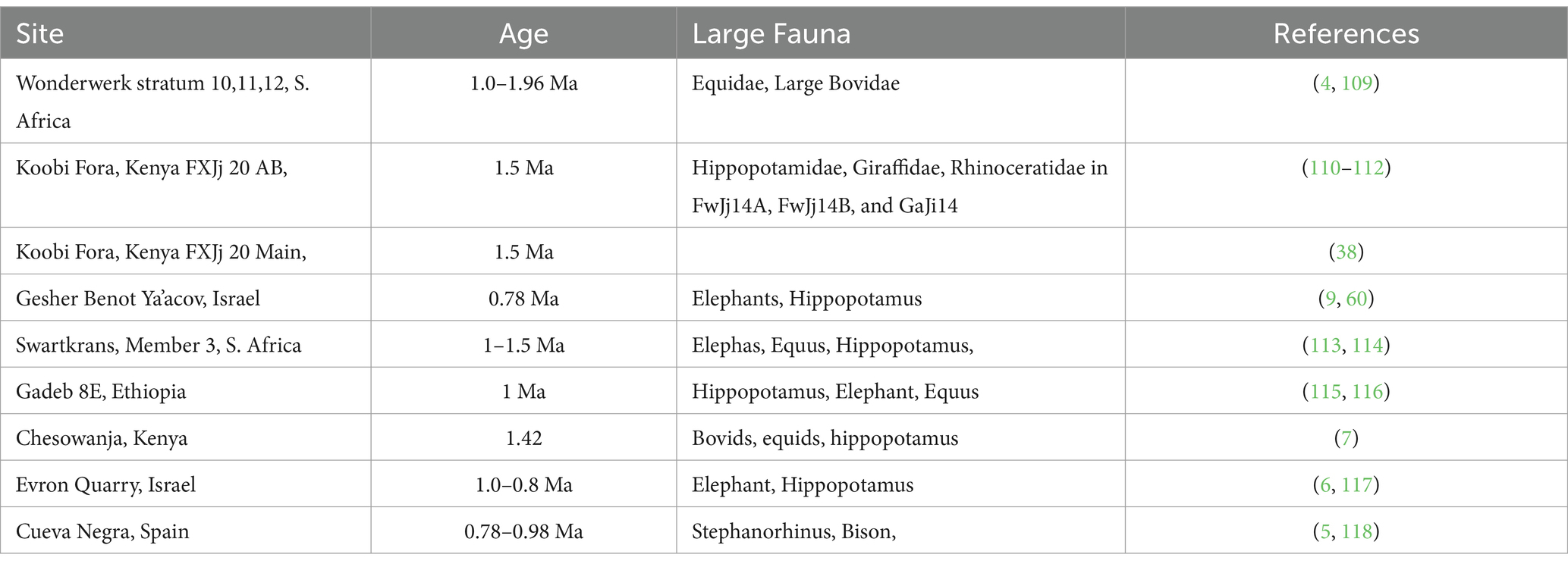
Table 3. Early and middle lower Paleolithic sites with evident traces of fire and the presence of large fauna.
3.4 The energetic return on cooking
A consensus exists in the literature that cooking enhances the bioavailability of energy from plant-based foods, such as tubers and seeds (39–42). While the denaturation of proteins and the loss of structural integrity in meat are expected to augment its energetic value when cooked, several factors, including the toughening of meat fibers, Maillard reactions, and particularly the loss of fat during field roasting, counteract these benefits. Experimental studies involving mice fed cooked and uncooked meat—designed to eliminate the variable of fat loss—have yielded inconclusive results regarding the net energetic contribution of cooked meat. Moreover, these studies have not quantified the magnitude of any such contribution (17, 40).
Bioenergetic returns for both plant-based and animal-based foods are commonly calculated based on their theoretical maximum caloric content rather than their actual digestible caloric contribution, which remains empirically undetermined. Consequently, the values presented in Table 2 represent maximal estimates. Given the low initial average bioenergetic return of 1,443 ca/h for plant-based foods and a narrow 95% confidence interval (1025–1861 ca/h), the caloric gains attributable to cooking plants are likely to be minimal compared to those for meat. Magargal (42) (Table 3) quantifies the increase in digestibility due to cooking as 14–23%, 12.7, and 3.7% for starch, protein, and lipid components of plant foods, respectively. This energetic increase equates to a modest increase in energetic return, on the order of a few hundred calories per hour. Even if these digestibility gains were doubled, they would still be insufficient to offset the caloric cost of maintaining a fire, estimated to be in the high hundreds of calories per hour (18, 19) (Table 3) or be comparable to the return benefits of preservation and predator protection of large prey. While using smaller, short-term fires for episodic cooking could potentially reduce collection costs, it is less probable that such fires would leave detectable traces hundreds of thousands of years later (43).
As for meat, the positive impact of cooking on protein digestibility (12.7%) is relevant to only half of the caloric content in large animals (44) (Table 1). The remaining caloric content is lipid-based, for which cooking enhances digestibility by a mere 3.7%. Consequently, the weighted average increase in digestibility due to cooking would contribute approximately 8%, or around 1,200 ca/h, to the overall energetic return. Consequently, energetic return for uncooked meat would approximate 15,000 ca/h (i.e., ~16,200 ca/h minus 1,200 ca/h).
The energic cost of maintaining fires is highly variable and contingent upon environmental factors, making it difficult to quantify precisely. Nevertheless, even under the assumption of low cost for cooking plants, the energetic return from uncooked meat remains over 10 times greater than that from cooked plants (~15,000 ca/h versus 1,443 ca/h). In summary, whether cooked or uncooked, the bioenergetic yield from hunting substantially surpasses that from plant gathering.
4 Discussion
4.1 Energetic return and behavior
According to the Optimal Foraging Model (OFM) (45), the pronounced disparity in energetic returns between plant gathering and prey hunting suggests a predominance of hunting in early human subsistence strategies.
The OFM model employs the relative availability of prey and plant resources to predict dietary choices based on a priori rankings of food items. It is thus crucial to note that the abundance of large prey was considerably higher in periods preceding the Late Quaternary, prior to the megafaunal extinction events (23, 46–48). The extinction of megaherbivores led to a significant reduction in the faunal carrying capacity of ecosystems while concurrently increasing the vegetation carrying capacity (49–52). Consequently, large prey was more readily available to early humans than in contemporary settings, and its relative abundance compared to plant resources was likely higher.
4.2 Large prey consumption in the lower Paleolithic
A conspicuous presence of large and very large prey in early to middle Lower Paleolithic archeological sites is very common. Recent analyses of the archeozoological and paleontological East African record portray H. erectus as a habitual hunter of large prey (24, 53, 54). Preference for large prey animals during the Pleistocene is a conventional interpretation of archeological assemblages [(e.g., 55, 56)]. Large animals, including elephants, are a common feature in African early Pleistocene sites (55, 57–59) and sites outside Africa, such as Latame, Ubeidiya, Revadim and Gesher Benot Yaaq’ov in the Levant, Dmanisi in Georgia (25, 60–64), Marathousa in Greece (65, 66), 21 sites with Mommuyhus meridionalis remains in association with Acheulean or Oldowan stone tools in Spain (67), Castel de Guido and eight other elephant butchering sites in Italy (68) and Schoningen in Germany (69, 70). Indeed, according to Werdelin and Lewis (71), beginning 1.5 Mya, humans became members of the hypercarnivore guild, specializing in acquiring large herbivores, as evidenced by the extinction of sabertooth predators and some hyenas. At the same time, there was no unusual decline in the under-21 kg hypo-carnivore group. A similar phenomenon of large carnivore extinction a few hundred thousand years after humans’ arrival was recently described in Spain (72). The decline of large carnivores in conjunction with human peopling and competition was also identified in Italy (73). Large animals, including elephants, continued to be a visible component of archeological sites worldwide throughout the Pleistocene (23, 67, 68, 74–78). Stiner (79) (p. S288) writes, “… hominins were big-game hunters, and they were rather specialized in their focus on ungulate prey.”
A multidisciplinary literature review including evidence from human physiology and genetics, archeology, paleontology, and zoology found that H. erectus was likely a hypercarnivore, consuming over 70% of his energy from meat and fat and specializing in obtaining large prey (80). We conclude that large prey contributed significantly to humans’ bioenergetic economy during the studied period. Consequently, large prey preservation by smoking and protection must have been a significant economic need for Paleolithic hunter-gatherers.
4.3 Meat preservation
A prominent impetus for fire utilization could be its role in food preservation through smoking and drying, extending the shelf-life of energetically valuable resources. This fire use would render fire a more energetically efficient tool for preserving the yield of hunting activities than for cooking plants or meat. The early use of fire for meat preservation may be less controversial than for predator protection, as evidence suggests various methods of meat preservation were employed, including drying and smoking, by late Paleolithic and recent hunter-gatherers (8, 43, 81, 82). Moreover, it was demonstrated that late Lower Paleolithic humans intentionally preserved marrow within fallow-deer limb bones for several weeks while stored in a cave, thus supporting the attention paid to food preservation in the Paleolithic (83). Additionally, there is proof that humans can consume putrid meat (84), and thus, prey consumption could be extended beyond our modern standards.
The paucity of fire evidence in early archeological sites could be due to the poor preservation of campfires (14), or it may indicate that fires were primarily employed in sites characterized by intensive large prey consumption, like Gesher Benot Ya’acov where the energetic costs of wood collection would have been justified in order to preserve and protect the huge quantities of meat and fat consumed at the site (9, 25, 60). While the use of torches for active scavenging to deter predators from hunted prey (85) or to impede the mobility of megaherbivores during hunts (86) is theoretically conceivable, empirical evidence for such practices remains conspicuously absent from the archeological record.
4.4 Anti-predation demands on Homo erectus
The threat posed to Homo erectus, and its possession of prey would have been substantial. Interspecific killings are common among carnivores with intermediate differences in body size and comparable predatory habits (87). Homo erectus, with an estimated body weight of 60–70 kgs (88) and targeting large prey, meets this definition. Predatory species of the Hyaenidae family, such as the Hyaena hyaena and the Crocuta genus were kleptoparasites, and theft of prey was also observed in lions (89).
Mitigating predation pressure constitutes a significant facet of animal behavior, as extensively documented in the literature (90). For instance, chimpanzees employ arboreal nesting as a defensive strategy against predators (91). The transition to terrestrial sleep is generally attributed to Homo erectus (92). As an ancillary hypothesis, the necessity to guard large prey over extended periods by Homo erectus could have catalyzed this behavioral shift.
Notably, the risk profile for Homo erectus diverged substantially from that of other carnivores. Unlike many carnivores, humans often transported their kills to a centralized location (93). A single megaherbivore could contain calories ranging from 1 million to several million (25, 26, 94), thereby sustaining a community for an extended duration (Table 1). Consumption would span days, weeks, or even months. Gaudzinski-Windheuser, Kindler (26) (p. 11) posit that, based on the caloric content of Straight-tusked elephants at the Neumark-Nord 1 site, a local hunter-gatherer group of approximately 25 individuals could be sustained for a minimum of 3 months, contingent upon the availability of food preservation methods.
During this extended period, the partially consumed carcass would inevitably attract both predators and scavengers, thereby elevating the risk to both the prey and the human group. Disentangling the risk of prey theft from the risk of predation upon humans is both impractical and likely inconsequential. While the economic cost of theft can be quantified (Table 2), the risk to human life defies easy quantification. The presence of partially consumed megaherbivores would have intensified the predation risk, making it a more acute issue for Homo erectus compared to its predecessors, who generally targeted smaller prey (24).
In summary, the risk profile for Homo erectus extended beyond the immediate threat of loss of life to include the potential forfeiture of substantial energetic investments in procuring food. Moreover, the temporal proximity of predators shifted from intermittent episodes lasting minutes or hours to a near-constant presence, particularly if the habitual consumption of large prey constituted a significant portion of the diet. Thus, fire could provide a significant risk-reduction mean to humans in the discussed period. The partial transition to cave habitation during the Late Middle Pleistocene [(e.g., 36)] mitigated predation risks by constraining the avenues of approach available to predators. While caves were frequented by apex predators such as cave lions, cave bears, and hyenas, partially consumed prey and preserved anatomical components [(e.g., 83)] would have invariably attracted both predators and scavengers. Despite this, scant evidence suggests interspecific prey sharing within cave environments, possibly attributable to the use of fire [(e.g., 95)].
Other uses of fire by current hunter gatherers such as warmth, ritual, raw material processing, light and protection from insect (43) cannot be ruled out but do not lend themselves to a simple economic modeling, the preferred method of investigation in this paper.
4.5 The relationship between prey preservation and protection hypothesis and the cooking hypothesis
We do not contend that fire was not used for cooking during the early to middle Lower Paleolithic periods and beyond. Empirical evidence indicates that fish cooking, for example, occurred at Gesher Benot Ya’akov approximately 780,000 years ago (96). Such culinary activities would not undermine our hypothesis; if a fire were available for prey preservation and protection, its multifunctional utility for cooking, warmth, illumination, and social cohesion would naturally be exploited at no additional cost. However, the assertion that Homo erectus’ reduced masticatory requirements and capacity for high-quality food consumption were contingent upon cooking (12) is challenging to reconcile. Zink and Lieberman (97) demonstrated that the mechanical reduction of food by stone tools into smaller fragments could diminish masticatory demands. Significant consumption of soft fat tissue by Homo erectus due to limited protein metabolism capabilities (25, 80, 98) could also enable a smaller masticatory system.
Contrary to Wrangham’s original hypothesis, which posits cooking as the catalyst for key evolutionary adaptations in Homo erectus, our hypothesis redirects the focus toward specialization in large prey hunting. Consumption of plants by hominins continued as it had for millions of years, and when cooking had taken place, it could have been done on fires that were initiated to preserve and protect prey. We have delineated additional, non-faunal evidence supporting Homo erectus’ specialization in large prey acquisition and describing possible adaptations that can be attributed to this specialization (44, 80). By specialization in large prey, we mean that Homo erectus were adapted and likely energetically dependent on the acquisition of significant quantities of large prey. There is evidence that Homo erectus did acquire smaller prey and consumed plants (60, 99). The cognitive demands associated with fire production and maintenance for preservation and protection could have been instrumental in driving the observed protracted increase in average brain volume in Homo erectus (100). This hypothesis is not so different than Wrangham’s as it set the need to produce fire as one of the drivers of Homo erectus’ evolution. However, it emphasizes the specialization in acquiring large prey as its driver.
4.6 Discrepancy between ethnography-based protection needs and energetic return data and early Paleolithic conditions
Ethnographic analogies with Paleolithic circumstances have been used to argue that, in the Paleolithic, protection from predators was a negligible requirement (43), and that the energetic return on hunting was much lower than our prediction, around 980 calories per hour (101). The validity of analogies between recent and Paleolithic hunter-gatherer behavior has been the subject of prolonged debate, sometimes leading to strong statements such as “To hell with Ethnoarchaeology” (27) and “The tyranny of ethnography” (28). Over 60 years ago, the founders of modern archeology established that any analogy between recent and later occurrence must meet the fundamental requirement of comparable environments and technologies (102). This is even more critical when seeking quantitative values like energetic returns, which are directly affected by environmental conditions and technological capabilities. We addressed this lacuna in detail in a paper dedicated to this problem (30), so we will only briefly review the main arguments here.
Foraging optimization models often use energetic returns as a key factor in determining optimal strategies. Accordingly, prey is added to the diet in descending order of energetic return (103, 104). Thus, the pronounced disparity in energetic returns between plant gathering and prey hunting suggests a predominance of hunting in early human subsistence strategies.
Another factor in food items ranking is the relative availability of prey and plant resources. It is thus crucial to note that the abundance of large prey was considerably higher in periods preceding the Late Quaternary Megafaunal Extinction and later, Holocene extinctions (23, 46–48). The extinction of megaherbivores led to a significant reduction in the faunal carrying capacity of ecosystems while concurrently increasing the vegetation carrying capacity (49–52). Consequently, large prey was more abundant compared to plant resources before the Late Quaternary, increasing its relative acquisition in previous periods, compared to ethnography-based findings.
Large herbivores also create and maintain open landscapes, which affect the relative ease of searching for and acquiring prey and plants, and the relative faunal biomass, which tends to be higher in open landscapes (105).
Kraft, Venkataraman (101) dataset which claims average energetic return of 982 cal/h is largely composed of rainforest groups who practice part-time horticulture. As we saw in Table 2, energetic returns in rainforest are some five times lower than in open forests. Moreover, their extremely low 982 calories per hour return does not align with Morin, Bird (32) returns, even when we normalize them for the search costs included in Kraft et al.’s net calculations. For example, a common Paleolithic prey, a buffalo, contains 550,000 calories (Supplementary material). Morin et al.’s average of 14,877 calories means that handling and pursuit would have taken (550,000 / 14,877) 37 h to complete. Applying Kraft et al.’s 982 calories per hour net return, including search, results in (550,000 / 982) 560 h for handling, pursuit, and search, so (560–37) 523 h for search. Assuming 8-h search days, this equates to 65 days of search before hunters were supposed to hunt one buffalo, while a buffalo could last a 25-person group for about 9 days (550,000 / (25 × 2,500)). This example clearly shows that the return on hunting could not have been that low, or there would not have been evidence for the acquisition and exploitation of buffalo (or Bos/Bison) in the Paleolithic. In other words, Kraft, Venkataraman (101) results may be correct for hunting of small prey like squirrels and rodents in a South American Jungle, where search time per calorie could be substantial but not for large highly visible prey almost nine hundred kgs bigger, which lives in large herds in the African open savannahs. On a side note, it is doubtful that humans could survive in the rainforest solely on hunting and gathering with such low returns without the relatively high return on horticulture (101).
Regarding McCauley, Collard (43) claim of little fire use for protection in recent hunter-gatherers, the effects of the extinctions of large herbivores and the resulting non-analogous present environment (48) on the need for fire for preservation and protection are substantial. The megafauna extinction also caused the extinction of large carnivores (106). The decline in large prey led to the hunting of smaller prey that lasted for less time, hence reducing the need for protection and preservation. Coupled with the presence of dogs in many recent hunter-gatherer camps, the need for fire use for protection must have declined substantially, compared to earlier periods.
In summary, McCauley, Collard (43) results and Kraft, Venkataraman (101) cannot be used as an inference to the Lower Paleolithic period.
5 Conclusion and future research
The study connects early fire use to dietary strategies, emphasizing the nutritional importance of meat and fat from large prey in the Lower Paleolithic. It challenges assumptions about the primacy of cooking in shaping human nutritional evolution, potentially influencing how we understand dietary adaptations in Homo erectus.
By focusing on bioenergetic costs and benefits, the paper reframes fire use as a strategic adaptation to maximize returns from hunting, a key subsistence activity for Homo erectus.
In archeology, behavioral ecology is often employed to elucidate decisions related to prey hunting and plant gathering (107).
In this context, we delved into the bioenergetic implications that sustaining fire incurs energetic costs, necessitating a net positive energetic return to justify its production and maintenance. Our hypothesis posits that the primary impetus for early fire use lay in the imperative to preserve and safeguard large prey from predators during the extended period of its consumption. We draw upon the significantly higher energetic returns associated with hunting prey than gathering plants to substantiate our hypothesis.
In recent hunter-gatherers, the average energetic yield from plant foraging is less than one-tenth of the return obtained from the most analogous hunting prey weighing over 100 kg in non-rainforest African environments (Table 2). Although we argue that ethnographic data may not serve as an ideal analogy for the Lower Paleolithic, the substantial disparity in energetic returns between plant gathering and hunting and the higher relative density of large herbivores in the earlier times suggests that a similar, sizable difference likely existed during the early to middle Lower Paleolithic. The present energetic return gap that is measured on a background of increased relative biomass of plants (106) strengthen the case for the existence of the plant animal return gap during the Paleolithic period when relative plants abundance was smaller.
The substantial evidence for the consumption of large prey by Homo erectus validates a need to preserve and protect prey remains for many days. The relative gain of energetic return from the prevention of prey deterioration and theft substantially outweighs the gains that cooking could provide and thus should have been a more likely driver of the production of fire. Other uses of fire like warmth and light do not lend themselves to simple economic modeling so could not be rejected or supported using our method.
Although cooking is stated as the most common use of fire among recent hunter-gatherers, we argued that the presence of dogs and of smaller prey due to the megafauna extinction throughout the Pleistocene and the Holocene have reduced the need for the preservation of prey and the protection from diminishing guilds of large predators.
Since there is little knowledge of the extent of which Early Pleistocene predators and scavengers would have been deterred by fire, the relative importance of preservation and protection in the early use of fire remains open.
The cognitive adaptations that presumably were required for fire production, combined with the need to adapt to acquire large prey, together may explain the marked increase in Homo erectus’ brain volume. In return, large fat and meat deposits, as well as fire protection and preservation, sustainably supported the extensive caloric demands of Homo erectus. This hypothesis is associated with our unifying hypothesis explaining human physiological and cultural evolution as an adaptation to varying prey sizes during the Paleolithic period (44, 108).
The hypothesis could be further scrutinized by examining temporal trends in the co-occurrence of predator gnawing marks and human consumption marks on faunal remains and by contrasting gnawing rates at sites with and without fire traces. However, such testing is constrained by uncertainties surrounding the anthropogenic origins of fire at identified sites and the absence of fire at others. The scarcity of early sites with pertinent evidence further complicates empirical validation. However, the nexus between evidence of fire and the presence of large prey mammals at several early-middle Lower Paleolithic sites strongly supports the hypothesis presented here.
We hope the prey preservation and protection hypothesis will generate more relevant research and eagerly await further developments in the field.
Data availability statement
The original contributions presented in the study are included in the article/Supplementary material, further inquiries can be directed to the corresponding author/s.
Author contributions
MB-D: Writing – original draft, Writing – review & editing. RB: Writing – original draft, Writing – review & editing.
Funding
The author(s) declare that no financial support was received for the research and/or publication of this article.
Acknowledgments
We thank the reviewers for their useful comments.
Conflict of interest
The authors declare that the research was conducted in the absence of any commercial or financial relationships that could be construed as a potential conflict of interest.
Generative AI statement
The author(s) declare that no Gen AI was used in the creation of this manuscript.
Publisher’s note
All claims expressed in this article are solely those of the authors and do not necessarily represent those of their affiliated organizations, or those of the publisher, the editors and the reviewers. Any product that may be evaluated in this article, or claim that may be made by its manufacturer, is not guaranteed or endorsed by the publisher.
Supplementary material
The Supplementary material for this article can be found online at: https://figshare.com/articles/dataset/Cooking_or_protection_xlsx/23903907?file=41908134
References
1. Sandgathe, DM. Identifying and describing pattern and process in the evolution of hominin use of fire. Curr Anthropol. (2017) 58:S360–70. doi: 10.1086/691459
2. Chazan, M. Toward a long prehistory of fire. Curr Anthropol. (2017) 58:S351–9. doi: 10.1086/691988
3. Parker, CH, Keefe, ER, Herzog, NM, O'connell, JF, and Hawkes, K. The pyrophilic primate hypothesis. Evolutionary Anthropol: Issues, News, Rev. (2016) 25:54–63. doi: 10.1002/evan.21475
4. Berna, F, Goldberg, P, Horwitz, LK, Brink, J, Holt, S, Bamford, M, et al. Microstratigraphic evidence of in situ fire in the Acheulean strata of Wonderwerk cave, northern cape province, South Africa. Proc Natl Acad Sci. (2012) 109:E1215–20. doi: 10.1073/pnas.1117620109
5. Rhodes, S, Walker, M, López-Jiménez, A, López-Martínez, M, Haber-Uriarte, M, Fernández-Jalvo, Y, et al. Fire in the early Palaeolithic: evidence from burnt small mammal bones at Cueva Negra del Estrecho del Río Quípar, Murcia. Spain J Archaeolog Sci: Reports. (2016) 9:427–36. doi: 10.1016/j.jasrep.2016.08.006
6. Stepka, Z, Azuri, I, Horwitz, LK, Chazan, M, and Natalio, F. Hidden signatures of early fire at Evron quarry (1.0 to 0.8 Mya). Proc Natl Acad Sci. (2022) 119:e2123439119. doi: 10.1073/pnas.2123439119
7. Gowlett, JA, Harris, JW, Walton, D, and Wood, BA. Early archaeological sites, hominid remains and traces of fire from Chesowanja. Kenya Nature. (1981) 294:125–9.
8. Stancampiano, LM, Rubio-Jara, S, Panera, J, Uribelarrea, D, Pérez-González, A, and Magill, CR. Organic geochemical evidence of human-controlled fires at Acheulean site of Valdocarros II (Spain, 245 kya). Sci Rep. (2023) 13:7119. doi: 10.1038/s41598-023-32673-7
9. Alperson-Afil, N. Continual fire-making by hominins at Gesher Benot Ya ‘aqov, Israel. Quat Sci Rev. (2008) 27:1733–9. doi: 10.1016/j.quascirev.2008.06.009
10. Roebroeks, J, MacDonald, K, Scherjon, F, Gaudzinski-Windheuser, S, and Jöris, O. Establishing patterns of early fire use in human evolution. The Beef behind All Possible Pasts The Tandem Festschrift in Honour of Elaine Turner and Martin Street. (2021) 7:7–16. doi: 10.1126/sciadv.abj5567
11. Wrangham, RW, Jones, JH, Laden, G, Pilbeam, D, Conklin-Brittain, NL, Brace, CL, et al. The raw and the stolen. Curr Anthropol. (1999) 40:567–94.
12. Wrangham, R. Control of fire in the Paleolithic evaluating the cooking hypothesis. Curr Anthropol. (2017) 58:S303–13. doi: 10.1086/692113
13. Roebroeks, W, and Villa, P. On the earliest evidence for habitual use of fire in Europe. Proc Natl Acad Sci. (2011) 108:5209–14. doi: 10.1073/pnas.1018116108
14. Gowlett, J. The discovery of fire by humans: a long and convoluted process. Philosop Trans Royal Society of London B: Biolog Sci. (2016) 371:20150164. doi: 10.1098/rstb.2015.0164
15. Barkai, R, Rosell, J, Blasco, R, and Gopher, A. Fire for a reason: barbecue at middle Pleistocene Qesem cave. Israel Current Anthropol. (2017) 58:S314–28. doi: 10.1086/691211
16. Rolland, N. Was the emergence of home bases and domestic fire a punctuated event? A review of the middle Pleistocene record in Eurasia. Asian Perspect. (2004) 43:248–80. doi: 10.1353/asi.2004.0027
17. Cornélio, AM, de Bittencourt-Navarrete, RE, de Bittencourt, BR, Queiroz, CM, and Costa, MR. Human brain expansion during evolution is independent of fire control and cooking. Front Neurosci. (2016) 10:167. doi: 10.3389/fnins.2016.00167
18. Henry, AG. Neanderthal cooking and the costs of fire. Curr Anthropol. (2017) 58:S329–36. doi: 10.1086/692095
19. Henry, AG, Büdel, T, and Bazin, P-L. Towards an understanding of the costs of fire. Quat Int. (2018) 493:96–105. doi: 10.1016/j.quaint.2018.06.037
20. Domínguez-Rodrigo, M, Baquedano, E, Organista, E, Cobo-Sánchez, L, Mabulla, A, Maskara, V, et al. Early Pleistocene faunivorous hominins were not kleptoparasitic, and this impacted the evolution of human anatomy and socio-ecology. Sci Rep. (2021) 11:16113–35. doi: 10.1038/s41598-021-94783-4
21. Pobiner, BL. The zooarchaeology and paleoecology of early hominin scavenging. Evolutionary Anthropol: Issues, News, Rev. (2020) 29:68–82. doi: 10.1002/evan.21824
22. Domínguez-Rodrigo, M. Hunting and scavenging by early humans: the state of the debate. J World Prehist. (2002) 16:1–54. doi: 10.1023/A:1014507129795
23. Dembitzer, J, Barkai, R, Ben-Dor, M, and Meiri, S. Levantine overkill: 1.5 million years of hunting down the body size distribution in the Paleolithic southern Levant. Quat Sci Rev. (2022) 276:107316. doi: 10.1016/j.quascirev.2021.107316
24. Linares Matás, GJ, and Yravedra, J. ‘We hunt to share’: social dynamics and very large mammal butchery during the Oldowan–Acheulean transition. World Archaeol. (2022) 53:224–54. doi: 10.1080/00438243.2022.2030793
25. Ben-Dor, M, Gopher, A, Hershkovitz, I, and Barkai, R. Man the fat hunter: the demise of Homo erectus and the emergence of a new hominin lineage in the middle Pleistocene (ca. 400 kyr). Levant PLoS One. (2011) 6:e28689. doi: 10.1371/journal.pone.0028689
26. Gaudzinski-Windheuser, S, Kindler, L, MacDonald, K, and Roebroeks, W. Hunting and processing of straight-tusked elephants 125.000 years ago: implications for Neanderthal behavior. Science. Advances. (2023) 9:eadd8186. doi: 10.1126/sciadv.add8186
27. Gosselain, OP. To hell with ethnoarchaeology! Archaeolog Dialog. (2016) 23:215–28. doi: 10.1017/S1380203816000234
28. Lieberman, DE, Bramble, DM, Raichlen, DA, and Shea, JJ. The evolution of endurance running and the tyranny of ethnography: a reply to Pickering and Bunn (2007). J Hum Evol. (2007) 53:439–42. doi: 10.1016/j.jhevol.2007.07.002
29. Wobst, HM. The archaeo-ethnology of hunter-gatherers or the tyranny of the ethnographic record in archaeology. Am Antiq. (1978) 43:303–9.
30. Ben-Dor, M, and Barkai, R. The importance of large prey animals during the Pleistocene and the implications of their extinction on the use of dietary ethnographic analogies. J Anthrop. Arch Androl. (2020) 59:101192. doi: 10.1016/j.jaa.2020.101192
31. Kelly, RL. The lifeways of hunter-gatherers: The foraging spectrum. Cambridge: Cambridge University Press (2013).
32. Morin, E, Bird, D, Winterhalder, B, and Bliege, BR. Deconstructing hunting returns: can we reconstruct and predict payoffs from pursuing prey? J Archaeol Method Theory. (2021) 29:561–623. doi: 10.1007/s10816-021-09526-6
33. Dominguez-Rodrigo, M, Cobo-Sanchez, L, Aramendi, J, and Gidna, A. The meta-group social network of early humans: a temporal–spatial assessment of group size at FLK Zinj (Olduvai Gorge, Tanzania). J Hum Evol. (2019) 127:54–66. doi: 10.1016/j.jhevol.2018.11.001
34. Ledger, HP. Body composition as a basis for a comparative study of some east African mammals. Symposium Zoolog Society of London. (1968) 21:289–310.
35. Marlowe, F. The Hadza: Hunter-gatherers of Tanzania University of California Press University of California Press, Berkley California (2010). 325 p.
36. Smith, GM, Ruebens, K, Gaudzinski-Windheuser, S, and Steele, TE. Subsistence strategies throughout the African middle Pleistocene: faunal evidence for behavioral change and continuity across the earlier to middle stone age transition. J Hum Evol. (2019) 127:1–20. doi: 10.1016/j.jhevol.2018.11.011
37. Herzlinger, G, and Goren-Inbar, N. Do a few tools necessarily mean a few people? A techno-morphological approach to the question of group size at Gesher Benot Ya'aqov. Israel J Hum Evol. (2019) 128:45–58. doi: 10.1016/j.jhevol.2018.11.008
38. Bellomo, RV. Methods of determining early hominid behavioral activities associated with the controlled use of fire at FxJj 20 Main, Koobi fora. Kenva J Hum Evol. (1994) 27:95.
39. Carmody, RN, and Wrangham, RW. The energetic significance of cooking. J Hum Evol. (2009) 57:379–91. doi: 10.1016/j.jhevol.2009.02.011
40. Carmody, RN, Weintraub, GS, and Wrangham, RW. Energetic consequences of thermal and nonthermal food processing. Proc Natl Acad Sci. (2011) 108:19199–203. doi: 10.1073/pnas.1112128108
41. Groopman, EE, Carmody, RN, and Wrangham, RW. Cooking increases net energy gain from a lipid-rich food. Am J Phys Anthropol. (2015) 156:11–8. doi: 10.1002/ajpa.22622
42. Magargal, K. The cost of cooking for foragers. J Hum Evol. (2022) 162:103091. doi: 10.1016/j.jhevol.2021.103091
43. McCauley, B, Collard, M, and Sandgathe, D. A cross-cultural survey of on-site fire use by recent hunter-gatherers: implications for research on Palaeolithic pyrotechnology. J Paleolithic Archaeol. (2020) 3:566–84. doi: 10.1007/s41982-020-00052-7
44. Ben-Dor, M, and Barkai, R. Prey size decline as a unifying ecological selecting agent in Pleistocene human evolution. Aust Q. (2021) 4:7. doi: 10.3390/quat4010007
45. Lupo, KD. Evolutionary foraging models in zooarchaeological analysis: recent applications and future challenges. J Archaeol Res. (2007) 15:143–89. doi: 10.1007/s10814-007-9011-1
46. Barnosky, AD, Koch, PL, Feranec, RS, Wing, SL, and Shabel, AB. Assessing the causes of late Pleistocene extinctions on the continents. Science. (2004) 306:70–5. doi: 10.1126/science.1101476
47. Smith, FA, Smith, REE, Lyons, SK, and Payne, JL. Body size downgrading of mammals over the late quaternary. Science. (2018) 360:310–3. doi: 10.1126/science.aao5987
48. Faith, JT, Rowan, J, and Du, A. Early hominins evolved within non-analog ecosystems. Proc Natl Acad Sci. (2019) 116:21478–83. doi: 10.1073/pnas.1909284116
49. Johnson, CN. Ecological consequences of late quaternary extinctions of megafauna. Proc R Soc Lond B Biol Sci. (2009) 276:2509–2519. doi: 10.1098/rspb.2008.1921
50. Rule, S, Brook, BW, Haberle, SG, Turney, CS, Kershaw, AP, and Johnson, CN. The aftermath of megafaunal extinction: ecosystem transformation in Pleistocene Australia. Science. (2012) 335:1483–6. doi: 10.1126/science.1214261
51. Bakker, ES, Gill, JL, Johnson, CN, Vera, FW, Sandom, CJ, Asner, GP, et al. Combining paleo-data and modern exclosure experiments to assess the impact of megafauna extinctions on woody vegetation. Proc Natl Acad Sci. (2016) 113:847–55. doi: 10.1073/pnas.1502545112
52. Hoag, C, and Svenning, J-C. African environmental change from the Pleistocene to the Anthropocene. Annu Rev Environ Resour. (2017) 42:27–54. doi: 10.1146/annurev-environ-102016-060653
53. Domínguez-Rodrigo, M, and Pickering, TR. The meat of the matter: an evolutionary perspective on human carnivory. Azania: Archaeolog Res Africa. (2017) 52:4–32. doi: 10.1080/0067270X.2016.1252066
54. Roach, NT, Du, A, Hatala, KG, Ostrofsky, KR, Reeves, JS, Braun, DR, et al. Pleistocene animal communities of a 1.5 million-year-old lake margin grassland and their relationship to Homo erectus paleoecology. J Hum Evol. (2018) 122:70–83. doi: 10.1016/j.jhevol.2018.04.014
55. Bunn, HT, and Ezzo, JA. Hunting and scavenging by Plio-Pleistocene hominids: nutritional constraints, archaeological patterns, and behavioural implications. J Archaeol Sci. (1993) 20:365–98.
56. Surovell, TA, and Waguespack, NM. Human prey choice in the late Pleistocene and its relation to megafaunal extinctions In: G Haynes, editor. American megafaunal extinctions at the end of the Pleistocene. Dordrecht: Springer (2009). 77–105.
57. Dominguez-Rodrigo, M, Bunn, HT, Mabulla, AZP, Baquedano, E, Uribelarrea, D, Perez-Gonzalez, A, et al. On meat eating and human evolution: a taphonomic analysis of BK4b (upper bed II, Olduvai Gorge, Tanzania), and its bearing on hominin megafaunal consumption. Quat Int. (2014) 322-323:129–52. doi: 10.1016/j.quaint.2013.08.015
58. Domínguez-Rodrigo, M, Bunn, HT, and Yravedra, J. A critical re-evaluation of bone surface modification models for inferring fossil hominin and carnivore interactions through a multivariate approach: application to the FLK Zinj archaeofaunal assemblage (Olduvai Gorge, Tanzania). Quat Int. (2014) 322-323:32–43. doi: 10.1016/j.quaint.2013.09.042
59. Klein, RG. The archaeological significance of animal bones from Acheulean sites in southern Africa. Afr Archaeol Rev. (1988) 6:3–25.
60. Rabinovich, R, and Biton, R. The early–middle Pleistocene faunal assemblages of Gesher Benot Ya‘aqov: inter-site variability☆. J Hum Evol. (2011) 60:357–74. doi: 10.1016/j.jhevol.2010.12.002
61. Bar-Yosef, O, and Belmaker, M. Early and middle Pleistocene faunal and hominins dispersals through southwestern Asia. Quat Sci Rev. (2011) 30:1318–37. doi: 10.1016/j.quascirev.2010.02.016
62. Carotenuto, F, Tsikaridze, N, Rook, L, Lordkipanidze, D, Longo, L, Condemi, S, et al. Venturing out safely: the biogeography of Homo erectus dispersal out of Africa. J Hum Evol. (2016) 95:1–12. doi: 10.1016/j.jhevol.2016.02.005
63. Gabunia, L, Vekua, A, and Lordkipanidze, D. The environmental contexts of early human occupation of Georgia (Transcaucasia). J Hum Evol. (2000) 38:785–802. doi: 10.1006/jhev.1999.0383
64. Rabinovich, R, Ackermann, O, Aladjem, E, Barkai, R, Biton, R, Milevski, I, et al. Elephants at the middle Pleistocene Acheulian open-air site of Revadim quarry. Israel Quat Int. (2012) 276-277:183–97. doi: 10.1016/j.quaint.2012.05.009
65. Panagopoulou, E, Tourloukis, V, Thompson, N, Konidaris, G, Athanassiou, A, Giusti, D, et al. The lower Palaeolithic site of Marathousa 1, megalopolis, Greece: overview of the evidence. Quat Int. (2018) 497:33–46. doi: 10.1016/j.quaint.2018.06.031
66. Konidaris, G, Tourloukis, V, Boni, G, Athanassiou, A, Giusti, D, Thompson, N, et al. Marathousa 2: a new middle Pleistocene locality in the Megalopolis Basin (Greece) with evidence of hominin exploitation of megafauna (Hippopotamus). PaleoAnthropology. (2023) 2023:34–55. doi: 10.48738/2023.iss1.810
67. Rosell, J, and Blasco, R. Elephants and humans in Iberia: a zooarchaeological approach In: G Konidaris, R Barkai, T Vangelis, and K Harvati, editors. Human-elephant interactions: From past to present. Tuebingen: Tuebingen University Press (2021). 177–200.
68. Rocca, R, Boschin, F, and Aureli, D. Around an elephant carcass: Cimitero di Atella and Ficoncella in the behavioural variability during the early middle Pleistocene in Italy In: G Konidaris, R Barkai, T Vangelis, and K Harvati, editors. Human-elephant interactions: From past to present. Tuebingen: Tuebingen University Press (2021). 287–302.
69. Altamura, F, Lehmann, J, Rodríguez-Álvarez, B, Urban, B, van Kolfschoten, T, Verheijen, I, et al. Fossil footprints at the late lower Paleolithic site of Schöningen (Germany): a new line of research to reconstruct animal and hominin paleoecology. Quat Sci Rev. (2023) 310:108094. doi: 10.1016/j.quascirev.2023.108094
70. Serangeli, J, Verheijen, I, Rodríguez-Álvarez, B, Altamura, F, Bigga, G, Schoch, WH, et al. Schöningen: a reference site for the middle Pleistocene. J Mediterranean Earth Sci. (2023) 15:369–87. doi: 10.13133/2280-6148/18154
71. Werdelin, L, and Lewis, ME. Temporal change in functional richness and evenness in the eastern African Plio-Pleistocene carnivoran guild. PLoS One. (2013) 8:e57944. doi: 10.1371/journal.pone.0057944
72. Mateos, A, Hölzchen, E, and Rodríguez, J. Sabretooths, giant hyenas, and hominins: Shifts in the niche of scavengers in Iberia at the Epivillafranchian-Galerian transition. Palaeogeogr Palaeoclimatol Palaeoecol. (2023) 634:111926. doi: 10.1016/j.palaeo.2023.111926
73. Palombo, MR. Deconstructing mammal dispersals and faunal dynamics in SW Europe during the quaternary. Quat Sci Rev. (2014) 96:50–71. doi: 10.1016/j.quascirev.2014.05.013
74. Moore, CR, Kimball, LR, Goodyear, AC, Brooks, MJ, Daniel, IR Jr, West, A, et al. Paleoamerican exploitation of extinct megafauna revealed through immunological blood residue and microwear analysis, north and South Carolina, USA. Sci Rep. (2023) 13:9464. doi: 10.1038/s41598-023-36617-z
75. Yravedra, J, Rubio-Jara, S, Panera, J, and Martos, JA. Hominins and proboscideans in the lower and middle Palaeolithic in the Central Iberian Peninsula. Quat Int. (2017) 520:140–156. doi: 10.1016/j.quaint.2017.12.002
76. Brugère, A. Not one but two mammoth hunting strategies in the Gravettian of the Pavlov Hills area (southern Moravia). Quat Int. (2014) 337:80–9. doi: 10.1016/j.quaint.2014.05.016
77. Zhang, Y, Stiner, MC, Dennell, R, Wang, C, Zhang, S, and Gao, X. Zooarchaeological perspectives on the Chinese early and late Paleolithic from the Ma’anshan site (Guizhou, South China). J Archaeol Sci. (2010) 37:2066–77. doi: 10.1016/j.jas.2010.03.012
78. McBrearty, S. The middle Pleistocene of East Africa In: L Brarham and K Robson-Brown, editors. Human roots: Africa and Asia in the middle Pleistocene : Western Academic and Specialist Press (2001). 81–97.
79. Stiner, MC. An unshakable middle Paleolithic? Trends versus conservatism in the predatory niche and their social ramifications. Curr Anthropol. (2013) 54:S288–304. doi: 10.1086/673285
80. Ben-Dor, M, Sirtoli, R, and Barkai, R. The evolution of the human trophic level during the Pleistocene. Yb Phys Anthrop. (2021) 175:27–56. doi: 10.1002/ajpa.24247
81. Spyrou, A, Maher, LA, Martin, LA, Macdonald, DA, and Garrard, A. Meat outside the freezer: drying, smoking, salting and sealing meat in fat at an Epipalaeolithic megasite in eastern Jordan. J Anthropol Archaeol. (2019) 54:84–101. doi: 10.1016/j.jaa.2019.02.004
82. Sandgathe, DM, Dibble, HL, Goldberg, P, Mcpherron, SP, and Hodgkins, J. On the role of fire in Neandertal adaptations in Western Europe: evidence from Pech de l’Azé IV and roc de Marsal, France. PaleoAnthropology. (2011):216–42. doi: 10.4207/PA.2011.ART54
83. Blasco, R, Rosell, J, Arilla, M, Margalida, A, Villalba, D, Gopher, A, et al. Bone marrow storage and delayed consumption at middle Pleistocene Qesem cave, Israel (420 to 200 ka). Sci Adv. (2019) 5:eaav9822. doi: 10.1126/sciadv.aav9822
84. Speth, JD. Putrid meat and fish in the Eurasian middle and upper Paleolithic: are we missing a key part of Neanderthal and modern human diet? PaleoAnthropology. (2017) 2017:44–72. doi: 10.4207/PA.2017.ART105
85. Clark, JD, and Harris, JW. Fire and its roles in early hominid lifeways. Afr Archaeol Rev. (1985) 3:3–27.
86. Churchill, SE. Weapon technology, prey size selection, and hunting methods in modern hunter‐gatherers: Implications for hunting in the Palaeolithic and Mesolithic. Archeological Papers of the American Anthropological Association. (1993) 4:11–24.
87. Donadio, E, and Buskirk, SW. Diet, morphology, and interspecific killing in Carnivora. Am Nat. (2006) 167:524–36. doi: 10.1086/501033
88. McHenry, H, and Coffing, K. Australopithecus to Homo: transformations in body and mind. Annu Rev Anthropol. (2000) 29:125–46. doi: 10.1146/annurev.anthro.29.1.125
89. Hayward, M. Prey preferences of the spotted hyaena (Crocuta crocuta) and degree of dietary overlap with the lion (Panthera leo). J Zool. (2006) 270:606–14. doi: 10.1111/j.1469-7998.2006.00183.x
90. Kotrschal, A, Deacon, AE, Magurran, AE, and Kolm, N. Predation pressure shapes brain anatomy in the wild. Evol Ecol. (2017) 31:619–33. doi: 10.1007/s10682-017-9901-8
91. Pruetz, JD, Fulton, S, Marchant, LF, McGrew, WC, Schiel, M, and Waller, M. Arboreal nesting as anti-predator adaptation by savanna chimpanzees (Pan troglodytes verus) in southeastern Senegal. American J Primatol: Official J American Society of Primatologists. (2008) 70:393–401. doi: 10.1002/ajp.20508
92. Fruth, B, Tagg, N, and Stewart, F. Sleep and nesting behavior in primates: a review. Am J Phys Anthropol. (2018) 166:499–509. doi: 10.1002/ajpa.23373
93. Isaac, GL. The Harvey lecture series, 1977-1978. Food sharing and human evolution: archaeological evidence from the Plio-Pleistocene of East Africa. J Anthropol Res. (1978) 34:311–25.
94. Guil-Guerrero, JL, Tikhonov, A, Ramos-Bueno, RP, Grigoriev, S, Protopopov, A, Savvinov, G, et al. Mammoth resources for hominins: from omega-3 fatty acids to cultural objects. J Quat Sci. (2018) 33:455–63. doi: 10.1002/jqs.3026
95. Rosell, J, and Blasco, R. Home sharing: carnivores in anthropogenic assemblages of the middle Pleistocene. J Taphon. (2009) 7:305–24.
96. Zohar, I, Alperson-Afil, N, Goren-Inbar, N, Prévost, M, Tütken, T, Sisma-Ventura, G, et al. Evidence for the cooking of fish 780,000 years ago at Gesher Benot Ya’aqov. Israel Nature Ecol Evolution. (2022) 6:2016–28. doi: 10.1038/s41559-022-01910-z
97. Zink, KD, and Lieberman, DE. Impact of meat and lower Palaeolithic food processing techniques on chewing in humans. Nature. (2016) 531:500–3. doi: 10.1038/nature16990
98. Ben-Dor, M, Gopher, A, and Barkai, R. Neandertals' large lower thorax may represent adaptation to high protein diet. Am J Phys Anthropol. (2016) 160:367–78. doi: 10.1002/ajpa.22981
99. Melamed, Y, Kislev, ME, Geffen, E, Lev-Yadun, S, and Goren-Inbar, N. The plant component of an Acheulian diet at Gesher Benot Ya ‘aqov, Israel. Proc Natl Acad Sci. (2016) 113:14674–9. doi: 10.1073/pnas.1607872113
100. Rightmire, GP. Brain size and encephalization in early to mid-Pleistocene Homo. American J Physical Anthropol: Official Pub American Association of Physical Anthropolog. (2004) 124:109–23. doi: 10.1002/ajpa.10346
101. Kraft, TS, Venkataraman, VV, Wallace, IJ, Crittenden, AN, Holowka, NB, Stieglitz, J, et al. The energetics of uniquely human subsistence strategies. Science. (2021) 374:eabf0130. doi: 10.1126/science.abf0130
103. Pyke, GH, Pulliam, HR, and Charnov, EL. Optimal foraging: a selective review of theory and tests. Q Rev Biol. (1977) 52:137–54.
104. Stephens, DW, and Charnov, EL. Optimal foraging: some simple stochastic models. Behav Ecol Sociobiol. (1982) 10:251–63.
105. Pearce, EA, Mazier, F, Davison, CW, Baines, O, Czyżewski, S, Fyfe, R, et al. Beyond the closed-forest paradigm: cross-scale vegetation structure in temperate Europe before the late-quaternary megafauna extinctions. Earth History and Biodiversity. (2025) 3:100022. doi: 10.1016/j.hisbio.2025.100022
106. Galetti, M, Moleón, M, Jordano, P, Pires, MM, Guimaraes, PR Jr, Pape, T, et al. Ecological and evolutionary legacy of megafauna extinctions. Biol Rev. (2018) 93:845–62. doi: 10.1111/brv.12374
107. Bird, DW, and O’Connell, JF. Behavioral ecology and archaeology. J Archaeol Res. (2006) 14:143–88. doi: 10.1007/s10814-006-9003-6
108. Ben-Dor, M, and Barkai, R. The evolution of Paleolithic hunting weapons: a response to declining prey size. Aust Q. (2023) 6:46. doi: 10.3390/quat6030046
109. Brink, J, Holt, S, and Horwitz, LK. The oldowan and early acheulean mammalian fauna of Wonderwerk cave (northern Cape Province, South Africa). Afr Archaeol Rev. (2016) 33:223–50. doi: 10.1007/s10437-016-9223-1
110. Hlubik, S, Cutts, R, Braun, DR, Berna, F, Feibel, CS, and Harris, JW. Hominin fire use in the Okote member at Koobi fora, Kenya: new evidence for the old debate. J Hum Evol. (2019) 133:214–29. doi: 10.1016/j.jhevol.2019.01.010
111. Pobiner, BL, Rogers, MJ, Monahan, CM, and Harris, JW. New evidence for hominin carcass processing strategies at 1.5 ma, Koobi fora, Kenya. J Hum Evol. (2008) 55:103–30. doi: 10.1016/j.jhevol.2008.02.001
112. Hlubik, S, Berna, F, Feibel, C, Braun, D, and Harris, JW. Researching the nature of fire at 1.5 Mya on the site of FxJj20 AB, Koobi fora, Kenya, using high-resolution spatial analysis and FTIR spectrometry. Curr Anthropol. (2017) 58:S243–57. doi: 10.1086/692530
113. De Ruiter, D. Revised faunal lists for members 1-3 of Swartkrans. South Africa Ann Transvaal Mus. (2003) 40:29–41.
114. Brain, CK, and Sillent, A. Evidence from the Swartkrans cave for the earliest use of fire. Nature. (1988) 336:464–6.
115. Barbetti, M. Traces of fire in the archaeological record, before one million years ago? J Hum Evol. (1986) 15:771–81.
116. Clark, JD, and Kurashina, H. An analysis of earlier stone age bifaces from Gadeb (locality 8E), northern bale highlands. Ethiopia South African Archaeolog Bulletin. (1979) 34:93–109.
117. Chazan, M. Butchering with small tools: the implications of the Evron quarry assemblage for the behaviour of Homo erectus. Antiquity. (2013) 87:350–67. doi: 10.1017/S0003598X00048997
118. Walker, M, López-Martínez, M, Carrión-García, J, Rodríguez-Estrella, T, Del-Toro, MS-N, Schwenninger, J-L, et al. Cueva Negra del Estrecho del Río Quípar (Murcia, Spain): a late early Pleistocene hominin site with an “Acheulo-Levalloiso-Mousteroid” Palaeolithic assemblage. Quat Int. (2013) 294:135–59. doi: 10.1016/j.quaint.2012.04.038
Keywords: fire, cooking, predators, human evolution, bioenergetics
Citation: Ben-Dor M and Barkai R (2025) A bioenergetic approach favors the preservation and protection of prey, not cooking, as the drivers of early fire. Front. Nutr. 12:1585182. doi: 10.3389/fnut.2025.1585182
Edited by:
Antonia Matalas, Harokopio University, GreeceReviewed by:
Ivo Jacobs, Lund University, SwedenMaria Ntinou, Aristotle University of Thessaloniki, Greece
Copyright © 2025 Ben-Dor and Barkai. This is an open-access article distributed under the terms of the Creative Commons Attribution License (CC BY). The use, distribution or reproduction in other forums is permitted, provided the original author(s) and the copyright owner(s) are credited and that the original publication in this journal is cited, in accordance with accepted academic practice. No use, distribution or reproduction is permitted which does not comply with these terms.
*Correspondence: Miki Ben-Dor, YmVuZG9yLm1pY2hhZWxAZ21haWwuY29t