- 1Department of Biophysics, All India Institute of Medical Sciences, New Delhi, India
- 2Centre for Rural Development and Technology, Indian Institute of Technology Delhi, New Delhi, India
- 3Department of Life Sciences, School of Natural Sciences, Shiv Nadar Institution of Eminence, Gautam Buddha Nagar, Uttar Pradesh, India
- 4Chemistry Department, Indian Institute of Technology Delhi, New Delhi, India
- 5Department of Analytical and Structural Chemistry, CSIR-Indian Institute of Chemical Technology, Hyderabad, India
- 6Laboratory for Multidisciplinary Research in Virology, Yenepoya (deemed to be University), Mangalore, India
Lung cancer, the second leading cause of cancer mortality, requires the development of novel therapeutic strategies due to emerging drug resistance and toxicity. With this objective, the present work explored the therapeutic potential of R. graveolens leaf extracts against EGFR_T790M-mediated drug resistance in NSCLC. To this end, we evaluated the functional and therapeutic potential of a panel of polar and non-polar solvent extracts using various in vitro assay systems. Among the extracts tested, EAE exhibited superior kinase inhibitory activity, which was more pronounced against the EGFR_T790M mutant phenotype. Accordingly, EAE exhibited a favorable cytotoxicity profile and potent growth inhibition of EGFR_T790M-positive NSCLC cells, as evident from its superior IC50 values in this cell type. Flow cytometry analysis further validated its inhibitory effects on the cell cycle and, well-supported by the data from the TUNEL assay, suggested induction of apoptosis in EAE-treated cells in a dose-dependent manner. Finally, mechanistic studies in EAE-treated cells showed that these outcomes were due to concentration-dependent inhibition of EGFR phosphorylation at Tyr1068 and Tyr1173. Importantly, this inhibition was consistently more pronounced in H1975 cells expressing the EGFR_T790M mutant phenotype. Further, pull-down assays, followed by mass spectrometry analysis, identified the most promising molecules within EAE. Together, the study highlighted the therapeutic potential of EAE from the leaves of Ruta graveolens for treating EGFR_T790M-mediated drug resistance in lung cancer.
1 Introduction
Cancer is the second leading cause of death globally after cardiovascular disease. It is responsible for high mortality, with an estimated 9.7 million deaths in 2022 (Bray et al., 2024). Recent research suggests that lung cancer is the most frequently diagnosed cancer worldwide. It accounts for nearly 2.5 million new cases annually and represents 12.4% of all cancers. With approximately 1.8 million deaths, it is the leading cause of cancer-related fatalities, accounting for 18.7% of all cancer deaths (Bray et al., 2024). Histopathologically, lung cancer is classified into SCLC and NSCLC (Spira, 2004). SCLC is defined by its inclination for early metastases and a rapid doubling time, with a 5-year survival rate of only 3% of patients (Simon and Wagner, 2003). Any epithelial lung cancer other than the SCLC is called NSCLC. It accounts for ∼85–90% of all lung cancers, with an overall 5-year survival rate in 15%–17% of patients (Siegel et al., 2023). Mutations in the intracellular tyrosine kinase (TK) domain of EGFR have been reported in the pathogenesis of several human malignancies, including NSCLC (Hirsch and Bunn, 2009; Inamura et al., 2010). Further, EGFR acts as a biomarker and a coherent target for treating NSCLC (Politi and Lynch, 2012).
Mutations in the EGFR kinase domain increase its catalytic activity and act as oncogenic drivers. Function-enhancing mutations in the EGFR gene are more prevalent in exons 18–21, which span the TK domain (Hodoglugil et al., 2013). The most effective approach in NSCLC therapy is targeting the EGFR signaling using TK inhibitors (TKI) (Bartholomew et al., 2017; Shah and Lester, 2020; Zhang et al., 2024; Zhou et al., 2009). Several TKIs were designed against mutant EGFR, among which erlotinib, gefitinib, afatinib, and osimertinib received FDA approval (Nan et al., 2017) (Figure 1). Patients who are initially responsive to EGFR TKIs eventually acquire resistance after 10–16 months of clinical benefit (Herst and Berridge, 2013). Moreover, 20%–30% of NSCLC patients show poor clinical response to EGFR TKIs (Wang et al., 2016). Median progression-free survival after the initiation of TKI treatment among NSCLC patients is about 10 months (Poh et al., 2024). This has been attributed to acquired drug resistance caused by mutations in the EGFR_TK, primarily the EGFR_T790M mutation (Ting et al., 2019). The EGFR_T790M mutation confers resistance to first-generation TKIs by increasing the affinity of TK for ATP, thereby competing out TKIs. Osimertinib, an FDA-approved third generation TKI, has potent inhibitory activity against EGFR_T790M-positive NSCLC but is associated with several adverse events, including an increased risk of cardiovascular diseases (Nishino and Hatabu, 2017). Further, high treatment costs and development of resistance against Osimertinib due to C797S mutation in EGFR (Guo et al., 2023; Thress et al., 2015; Song et al., 2016) limit its clinical use. Accordingly, there is an urgent need to develop novel therapeutics against NSCLC that comprehend the intratumoral EGFR heterogeneity, overcome TKI resistance, and cause milder or no adverse effects in the treated population. Natural products, such as plant secondary metabolites, possess tyrosine kinase inhibitory properties (Baier and Szyszka, 2020) and are promising candidates against drug-resistant EGFR kinases. Among the various natural sources explored in the literature, Ruta graveolens has demonstrated broad medical applications in treating multiple disorders, including lung cancer (Varamini, Soltani, and Ghaderi, 2009). A previous study by Fadlalla et al. reported that R. graveolens extract suppressed cancer cell proliferation by activating DNA damage pathways and inhibiting Akt activation (Fadlalla et al., 2011). Another study of R. graveolens extract showed the induction of cell death in glioblastoma cells and neural progenitors, but not neurons, through AKT and ERK 1/2 Activation (Gentile et al., 2015). Given that AKT and ERK are intermediate effectors of EGFR, we hypothesized that R. graveolens extracts may possess anti-EGFR kinase activities. We also sought to determine the specificity of these extracts for the drug-resistant EGFR_T790M mutant.
Ruta graveolens L. [Rutaceae] commonly known as rue or herb-of-grace, belongs to the Rutaceae family and is native to the Balkan Peninsula (Spence and Spence, 2023). Sterols of R. graveolens showed anticancer activity in the human lung cancer (A549) cell line with 81% inhibition at 100 μg/mL (Baker et al., 2017) and in the human epidermoid carcinoma (A431) cell line (Aherne and O’Brien, 2000; Réthy et al., 2006). Ruta graveolens is known for its analgesic and anti-inflammatory properties and has shown potential for selectively sensitizing cancer cells to apoptosis while sparing normal cells (Jinous, 2012). Among the metabolites reported so far in the literature, R. graveolens contains a diverse range of bioactive metabolites, including furanocoumarins, carotenoids, anthraquinone, furanoquinolones, and alkaloids (Kuzovkina, Al’terman, and Schneider, 2004; Hale et al., 2004; Eickhorst, DeLeo, and Csaposs, 2007). Rutin, which was first isolated from its leaves, and quercetin are the active flavonoids of R. graveolens (Pathak et al., 2003). Among these, psoralens, a subgroup of furanocoumarins, are well known for their ability to induce DNA interstrand cross-links upon exposure to ultraviolet (UV) light (DE RIE et al., 1995; Vogelsang et al., 1996).
Based on the available literature, this study aimed to identify bioactive molecules from the leaves of R. graveolens with targeted efficacy against drug-resistant EGFR_T790M mutant lung cancer. Accordingly, the study (Figure 2) investigated the effectiveness of various solvent extracts of leaves from R. graveolens against the EGFR_T790M drug-resistant phenotype of NSCLC. Among all the solvent extracts, EAE exhibited higher potency for the EGFR_T790M mutant phenotype. Mechanistically, EAE inhibited EGFR phosphorylation at Tyr1068 and Tyr1173, suggesting its action within the EGFR kinase domain. Pulldown assays followed by mass spectrometry analysis identified specific inhibitors unique to EGFR_T790M.
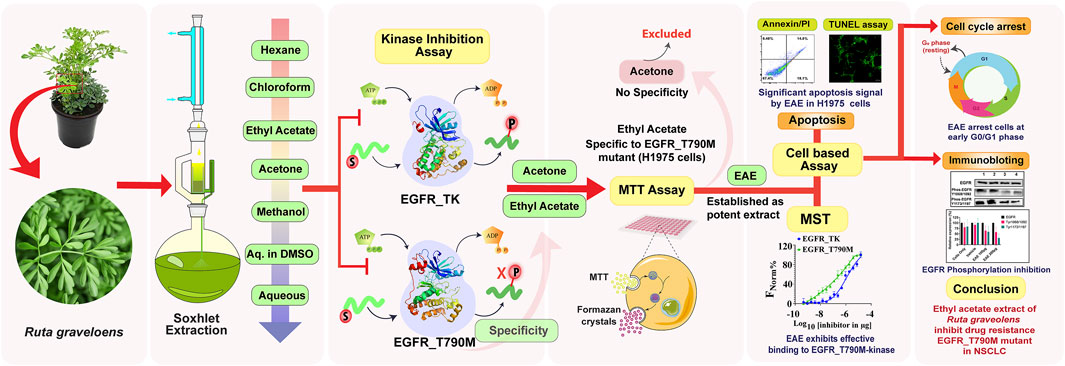
Figure 2. Graphical abstract illustrating the methodology and the bioactivity of EAE of Ruta graveolens against drug-resistant EGFR-mediated NSCLC.
2 Materials and methods
2.1 Cloning, expression, and purification of recombinant EGFR_WT and EGFR_T790M
Human EGFR_TK coding for the target region (residues 696-1022) was amplified from the human EGFR Addgene vector (Cat #11011) and cloned into pFastBac HT-B plasmid with N-terminal 6X-His-tag (pF-EGFR_WT696-1022) (Greulich et al., 2005). The EGFR_T790M mutant (pF-EGFR_T790M) was generated from this clone using the mutant primers by site-directed mutagenesis. Recombinant clones were transpositioned into DH10Bac cells harboring bacmid DNA to generate recombinant Bacmid DNA. Purified recombinant EGFR_TK and EGFR_T790M bacmids were transfected into Sf21 cells, and the recombinant viruses were rescued from the harvested culture supernatants. Plaque-purified recombinant virus clones were used to infect fresh monolayers of Sf21 cells to produce virus stocks for large-scale virus cultures. Infected cell pellets produced in high-density ExpiSf9™ cells were lysed in the reaction/storage buffer containing 25 mM Tris, 150 mM NaCl, 5 mM BME pH 8.0, and 10% Glycerol. The recombinant EGFR_TK and EGFR_T790M proteins were purified using a 2-stage purification method involving Ni-NTA affinity column chromatography followed by size-exclusion chromatography with 16/600 75 PG column (GE). The purity of the recombinant proteins was confirmed by PAGE and Western blot. Purified proteins were stored at −80°C in the storage buffer described above.
2.2 Cell cultures
Given the commercial unavailability of a cell line harboring only the EGFR_T790M mutation, the H1975 cell line (ATCC Cat #CRL-5908), that harbors T790M/L858R mutations, was used as a suitable alternative to determine the efficacy of plant extracts against EGFR_T790M(Chen et al., 2023; El Kadi et al., 2018; Liu et al., 2021; Liu et al., 2020; Walter et al., 2013; Zhao et al., 2015; Zou et al., 2013). A431 cell line overexpressing the EGFR_WT (Merlino et al., 1984), A549 (kRAS Lung Cancer) (Giard et al., 1973) and Jurkat (T4 Lymphoma, EGFR Negative) (Abraham and Arthur, 2004) cell lines were kind gifts from Prof. Deepak Sehgal’s laboratory (Shiv Nadar University, Noida, India), whereas H1299 (nRAS-driven lung cancer) (Ohashi et al., 2013) cell line was a kind gift from Dr. Prabhat Malik (AIIMS, New Delhi, India). All the cell lines were maintained as per the suppliers’ recommendations.
2.3 Preparation of Ruta graveolens leaf extracts
The R. graveolens plant used in this work was cultivated and preserved at the Applied Secondary Metabolite Laboratory nursery at the Centre for Rural Development and Technology, IIT Delhi, India. Initially, Online flora (https://indiaflora-ces.iisc.ac.in/FloraKarnataka/herbsheet.php?id=3979&cat=1) was used to identify the plant. Further, the identity was confirmed by Dr. Sampat Kumar, Lecturer in Biology (Taxonomist), Davangere Government Pre-university College, Karnataka, India, and Dr. Hariprasad P., Associate Professor, CRDT, IITD, Delhi, India. The specimen is maintained in the Applied Secondary Metabolite lab, IIT Delhi, with voucher no. CRDT/ASM/179-WP. The above-ground portion of the mature plants was removed in June and July 2022 before the blossoming using a sterilized stainless-steel knife. The leaves of the gathered plant specimens were excised from stems using a sterile surgical blade. The leaf samples were desiccated in a hot air oven at 40°C ± 2°C for 48 h and coarsely pulverized using a mechanical blender (Prestige Stylo mixer grinder, 750 w, India). Fifty grams of the powder were placed in a thimble and subjected to solvent extraction using a Soxhlet apparatus with a processing capacity of 500 mL. The metabolites in the pulverized powder were extracted successively using six solvents in order of increasing polarity: hexane, chloroform, ethyl acetate, acetone, methanol, and water. Briefly, Soxhlet was operated at 45 °C for hexane, chloroform, and ethyl acetate and run for 6 h for each solvent. In the case of acetone, methanol, and water, the heating was maintained at 50°C and ran for 10 h. At reduced pressure, the solvent extracts were concentrated with a rotary evaporator (Hahn vapor, HS-2005S, 200–240 V, Korea). The water bath temperature and vacuum pressure for hexane (45°C, 60 mmHg), chloroform (45°C, 60 mmHg), ethyl acetate (45°C, 60 mmHg), acetone (45°C, 80 mmHg), methanol (50°C, 80 mmHg) and water (50°C, 40 mmHg) was set. The condenser temperature was set to 4°C for all solvents used. Subsequently, the remaining solvents were eliminated by spreading the samples as a thin layer on a glass plate and placing them in a vacuum oven (Thermo Scientific, Model–Lab Line, 3618-1CE) set at −90 kPa, 40°C ± 2°C for 24 h. The desiccated extracts were reconstituted in dimethyl sulfoxide (DMSO) at 500 mg/mL concentration for further use.
2.4 Kinase assay
The kinase activity of purified EGFR_TK and EGFR_T790M mutant protein was assayed using the ADP-Glo kinase assay kit (#V9102 Promega, Madison, WI) (A. Wang et al., 2017) according to a previously optimized protocol (Aiebchun et al., 2021; Bajaj et al., 1987; Bernas and Dobrucki, 2002; Brignola et al., 2002) In a 32 μL reaction volume. Peptide C (RAHEEIYHFFFAKKK), a substrate for EGFR, (Engel et al., 2013) was purchased from Pepmic Co. Ltd., China. Concentrations of the EGFR variants, the peptide substrate, and the ATP were optimized (Supplementary Figure S1) for a reaction time of 60 min at 37°C. Optimized conditions were used to set up the kinase reactions, followed by adding ADP-Glo reagent for 40 min to deplete all the unused ATP, leaving only the ADP produced from the phosphorylation reaction. Next, 16 μL per reaction of the kinase detection reagent was added to convert ADP to ATP, and, subsequently, luminescence was measured at 560 nm (SpectraMax i3x Multi-Mode Microplate Reader). The data was plotted to calculate respective IC50 values for different extracts using the GraphPad Prism v9.3.1.
2.5 Cell viability assay
Semi-quantitative colorimetric MTT assays were performed to determine cell viability and cytotoxicity of the plant extracts. The assay involved seeding ∼104 cells per well of a 96-well culture plate and incubating the same at 37°C in a CO2 incubator to produce ∼80% confluent cell monolayers. Serial dilutions of plant extracts and DMSO vehicle control were prepared using the same dilution factor. Untreated control cells were treated with media only. All cells, including controls, were then incubated for 48 h. After 48 h of incubation, 100 μL of MTT reagent at 0.45 mg/mL was added per well and incubated for 4 h. Next, 100 μL DMSO was added to dissolve the formazan crystals over 2 h of incubation. The plates were read at 570 nm, background absorbance was subtracted, and the data was analyzed to determine the percent cell viability in treated versus untreated cells. Cell viability data was plotted against extract concentrations, and the IC50 values were derived from the dose-response curves.
2.6 Microscale thermophoresis binding assay
A Microscale thermophoresis assay was set up to determine the binding affinity of the selected extracts for EGFR_TK and EGFR_T790M kinases. Briefly, 200 nM of purified EGFR and its variant were fluorescently labeled with red Tris-NTA his-tag labeling dye (Nano temper Technologies, United States) as recommended. The mixture was kept in the dark for 30 min, followed by centrifugation at 15,000 × g for 10 min at 4°C. Two-fold serial dilutions of the extract were mixed, each with 200 nM of fluorescently labeled protein at a 1:1 ratio. MST was run on the Monolith NT.115 instrument at an ambient temperature of 25°C, with instrument parameters set to 50% LED and medium MST power. The samples were loaded into capillaries and placed inside the capillary tray of Monolith NT.115 MST instrument (nano temper technologies). The data generated for each extract, a protein combination from triplicate samples, was analyzed using Monolith NT.115 analysis software (Nanotemper Technologies).
2.7 Flow cytometry assays
Cell viability and induction of apoptosis were analyzed by flow cytometry using Alexa fluor 488 Annexin-V/Dead cell apoptosis kit (Cat # 13241, Invitrogen). For this, ∼3 × 105 cells were plated in each well of a 6-well plate to form sub-confluent monolayers and then treated with the selected plant extracts at pre-determined concentrations. Cells only, as untreated controls, were treated with media, whereas the vehicle controls had media supplemented with DMSO in the same proportion as the extracts and incubated for 48 h. After removing the culture supernatant, cells were treated with trypsin-EDTA, and the cell pellets were obtained by centrifugation. Cells were resuspended in Annexin binding buffer containing Annexin-V-Alexafluor 488, as described in the kit. Similarly, cell cycle inhibition by the selected plant extracts was analyzed using the Propidium Iodide (PI) reagent (Himedia SKU: ML067) according to the manufacturer’s protocol. The data was analyzed and plotted using CytExpert 2.5 software (Accuri Cytometers) and FloJo v10.0.
2.8 TUNEL [terminal deoxynucleotidyl transferase (TdT) dUTP Nick-end labeling] assay
The assay was performed using “Dead Cell Apoptosis Kit with Annexin V Alexa Fluor™ 488 and Propidium Iodide (PI) (Cat# V13241)”. Measurement of DNA fragmentation in apoptotic cells relies on TdT-mediated attachment of fluorescently labeled deoxynucleotides to the 3′-OH terminus of DNA breaks. For this, cells were seeded in a 6-well plate at ∼3 × 105 cells per well, grown to sub-confluency, and then treated with a predetermined concentration of the selected plant extract(s). Plates were incubated in a CO2 incubator for 48 h, monolayers rinsed with 1× Dulbecco’s PBS (DPBS), fixed in 4% paraformaldehyde for 25 min at 4°C, again rinsed twice with PBS and permeabilized with PBS-T containing 0.2% Triton X-100. Subsequently, cells were equilibrated at 37°C in a humid chamber for 60 min with TdT, and the reactions were terminated by adding 2× SSC, followed by three PBS rinses to remove excess fluorescein-12-dUTP. Necrotic cells were PI-labelled (#ML067 Himedia), while the live cells were labeled with DAPI for 15 min, followed by a rinse with nuclease-free water. Slides were mounted using one drop of anti-fade solution (Molecular Probes, Cat. #S7461) and analyzed under a fluorescence microscope for AlexFluor™ 488 at 520 nm, for PI at >620 nm and DAPI at 460 nm. All the images were captured on a Nikon Ti2E A1R MP Laser Scanning Confocal Microscope at a magnification of ×20 (Plan Apochromat 20X/0.75 NA).
2.9 Immunoblotting
Cells treated with/without EAE and lysed with RIPA buffer (#R0278, Sigma), were subjected to sonication for 2 min, and then centrifuged at 15,000 × g for 15 min at 4°C. The supernatant was collected and quantified using Bradford reagent (# 5000006 Bio-rad). Equal concentrations of proteins were resolved on 12% SDS-PAGE, transferred onto PVDF membranes (#IPVH00010 Merck), and treated with 5% non-fat milk powder in PBS-T. Primary antibodies were used at 1:1,000, while the peroxidase-conjugated anti-rabbit and anti-mouse IgG antibodies were used at 1:3,000 dilution (Bio-Rad). The blots were developed using the Chemiluminescent HRP Substrate (ECL kit, Bio-Rad). Antibodies used in the immunoblot assay were precision mouse anti-EGFR (#VMA00982 Bio-Rad), phosphor-EGFR (Tyr1068) [1092] (#AF3045, Affinity Biosciences), phosphor-EGFR (Tyr1173) [1197] (#AF3048, Affinity Biosciences), Goat-α-rabbit (#64524573, Bio-Rad), α-mouse (#32430, Bio-Rad), and Rabbit-α-GAPDH (#VPA00453, Bio-Rad) antibodies. Finally, using ImageJ, blots were subjected to densitometry analysis to quantify EGFR phosphorylation.
2.10 Pull-down assay and mass spectrometry analysis
2.10.1 Pull-down assay
To identify metabolites in EAE that specifically bound to EGFR_T790M and EGFR_TK, a pulldown experiment was performed using a 0.2 ml column containing 50 µl Ni-NTA resin. The column was washed with deionized water and three column volumes of binding buffer (50 mM Tris-HCl, pH 8.0; 150 mM NaCl; and 2 mM DTT). Then, 200 µg of purified EGFR-TK and, in a separate experiment, 200 µg of purified EGFR_T790M were loaded onto the column and incubated with rotation for 2 hours at 4°C. Unbound protein was removed with a wash buffer. Subsequently, 200 µg of plant extract (EAE) was added to the column and incubated overnight with constant rotation at 4°C. The following day, the column was washed five times with wash buffer, and the molecules bound to the protein were eluted with 100 µL of 50 mM citrate buffer, pH 3.0. The eluted plant extract fractions were lyophilized and used for mass spectrometry analysis.
2.10.2 LC-MS/MS analysis
The lyophilized plant extracts from the pull-down assay were reconstituted in 400 µL of methanol and filtered through a 0.22 µm syringe filter before being injected into the LC-MS. The LC-ESI-HRMS and MS/MS analysis of plant extracts were carried out on Orbitrap Exploris 120 mass spectrometer (Thermo Fisher Scientific, United States) coupled to Vanquish UHPLC (Thermo Fisher Scientific, United States). An autosampler introduced an injection volume of 10 µL of the sample into the mass spectrometer. The chromatographic separation was achieved using the Aquity UHPLC BEH C18 column (50 × 2.1 mm × 1.7-µm, M/s. Waters India Pvt. Ltd.), column temperature: 40°C and auto-sampler temperature: 7°C. A two-component mobile phase was used at a flow rate of 0.4 mL/min, where 0.05% formic acid in ultrapure water was used as mobile phase A and 0.05% formic acid in acetonitrile was used as mobile phase B, respectively. The optimized gradient program was set as follows: (time in min/% of Mobile phase B) 0–2/2, 2–10/98, 10–17/98, 17–20/2, and 20–22/2.
Mass spectral data was acquired in both the positive and negative ionization modes using the Heated-electrospray ionization (H-ESI) as an ionization source. The typical MS conditions were: the capillary voltage was set at 3500 V and 2500 V for positive and negative ionization modes, respectively; nitrogen (N2) gas was used as the sheath gas and auxiliary gas, and their flow was maintained at 50 (Arbitrary value) and 10 (Arbitrary value), respectively. The ion transfer tube and vaporizer temperatures were set at 300°C and 350°C, respectively; the CID gas pressure was set at 1.5 mTorr. The mass spectrometer was operated in full scan mode with data-dependent MS2 (ddMS2) acquisition. The full scan parameters are: scan range (m/z) 75–1,000, orbitrap resolution 60,000, RF lens (%) 70, and profile data acquisition. The ddMS2 parameters were as follows: isolation window (m/z) 2, collision energy type- normalized, HCD collision energies (%) were kept at 20, 30, and 40 eV, orbitrap resolution was set to 15,000 (FWHM), centroid-acquisition data type, peak filters: intensity threshold 2. e5, singly charged charge state, dynamic exclusion duration = 5 s. The acquired raw data was further processed using Compound Discoverer (v3.3, Thermo Fisher Scientific) for untargeted metabolite identification. Identification was based on accurate mass, retention time, and MS/MS spectral matching against online databases (ChemSpider, mzCloud, mzVault, MassList, HMDB). The resulting table (m/z, retention time, ion adduct, peak intensity) was refined by removing redundant ions, duplicates, and ions with CV > 15%. Finally, chromatograms (Supplementary Figures S2A,B, S3A,B, S4A,B) and MS/MS spectra quality were manually inspected to ensure accurate metabolite identification.
2.11 Statistical analyses
For statistical analyses, experimental data for each point was obtained from at least three replicates and represented as data mean ± standard deviation. The IC50 concentrations of the extracts were derived by modeling the data sets using a non-linear regression model. Statistical significance between different experimental groups was calculated by performing two-way ANOVA, paired t-tests, and one sample t-test. A p-value of <0.05 is considered significant in all the experiments. All the statistical analyses were performed using the built-in statistical methods available in GraphPad Prism version 9.3.1. (GraphPad, United States).
3 Results
Lung Cancer remains one of the significant challenges to combat, especially with the development of drug resistance, including EGFR_T790M mutation. This study is focused on finding plant-based therapeutic solutions to overcome the EGFR_T790M mediated drug resistance in NSCLC. In this direction, extracts from R. graveolens have been shown to target EGFR signaling pathways through ERK/AKT inhibition. Accordingly, in the present study, a variety of solvent extracts from R. graveolens were prepared by standard Soxhlet extraction methods. Subsequently, recombinant EGFR variants were produced using the Baculovirus expression system, followed by the development of kinase-inhibition assays. From these assays, potential extracts having specificity for the EGFR_T790M mutant were identified and characterized in cell-based assays for various anticancer properties to establish the mode of action as well as to identify potential bioactive molecules mediating inhibition of EGFR phosphorylation.
3.1 EAE and acetone extract shows selectivity for EGFR_T790M mutant kinase
EGFR_TK and EGFR_T790M variant Bacmid clones were used to transfect Sf21 cells, recombinant viruses rescued and plaque-purified. Purified clones of each variant were used to produce recombinant proteins from high density ExpiSf9™ cultures. The 2-stage purification process yielded ∼8 mg/L of kinases (Figure 3). The two recombinant EGFR variants were used to optimize the kinase inhibition assays. Optimal reaction conditions for both variants were determined to be 4 μM enzyme, 200 μM substrate, and 500 μM ATP, incubated at 37°C for 1 hour (Supplementary Figure S1). Next, the kinase inhibitory activity of different solvent extracts against the recombinant EGFR variants was investigated under optimized reaction conditions. All the solvent extracts exhibited notable but variable inhibitory activities against the two EGFR variants compared to the vehicle control. Of note, the EAE exhibited potent inhibitory activity against both the EGFR variants. Importantly, its activity against the EGFR_T790M was at least 3.4-fold higher than that against the EGFR_TK. Acetone extract also exhibited similar, but lesser, inhibitory activity against the two EGFR variants compared to the EAE. While the results from these assays suggested superior selectivity of EAE against the EGFR_T790M, both EAE and acetone extract were considered for further cell-based biological characterization. All other extracts that exhibited higher selectivity against EGFR_TK were excluded from further investigations. Relative inhibition of enzyme activities by different plant extracts against wtEGFR_TK and EGFR_T790M, and their corresponding IC50 values are shown in Figures 4 A-C. The 3.4-fold higher inhibitory effect of EAE against the EGFR_T790M mutant compared to that against the EGFR_TK, which highlights the selectivity of EAE for the mutant EGFR, is presented in Figure 4D.
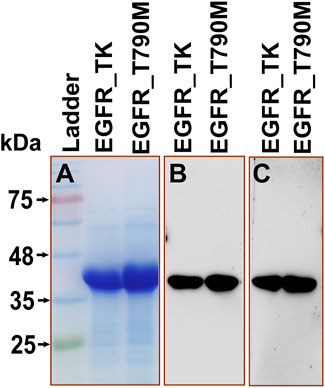
Figure 3. Purified EGFR_TK and EGFR_T790M proteins (A) Coomassie-stained 12% SDS-PAGE (B) Immunoblot with anti-His (1:3,000 dilution) antibody, (C) Immunoblot with anti-EGFR-TK-specific mAb (1:1,000 dilution).
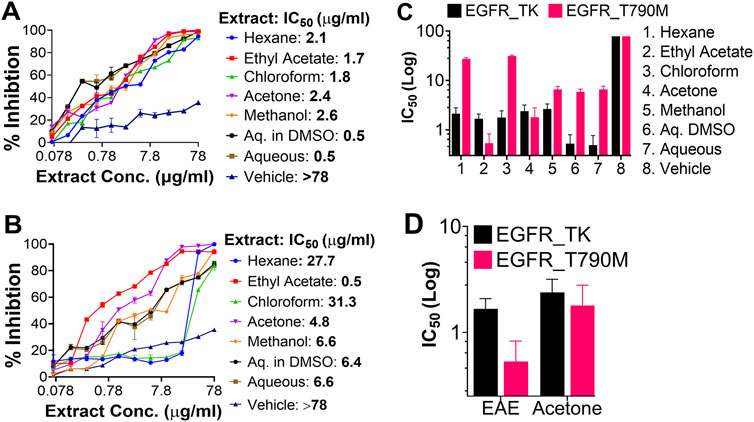
Figure 4. The kinase-inhibitory potential of different solvent extracts against EGFR variants. Dose-response curves were generated from the titration data, and their respective IC50 values were derived using GraphPad Prism v.9.3.1. In each graph, the X-axis represents the concentration of the extract in a linear scale. In contrast, the Y-axis represents the percentage of cell viability (0–100). (A) Inhibition of EGFR_TK activity, (B) Inhibition of EGFR_T790M activity, (C) Bar-graph representation of the IC50-values of each solvent extract against the two EGFR variants. (D) Bar-graph representation of the IC50-values of EAE and Acetone extract. Here, we show the superior selectivity of EAE exhibiting a 3.4-fold difference between the kinase activities of EGFR_TK and EGFR_T790M. The data shown here represents three independent experiments, and the error bars represent the standard deviation. A p-value of <0.05 is considered significant for all statistical analyses. (n = 3).
3.2 EAE is specific against drug-resistant lung cancer cell line
Cell-based assays were performed on different lung cancer cell types and in non-lung cancer cell lines to investigate the biological activity of the selected extracts. EAE and Acetone extract reduced cell viability in a dose-dependent manner in H1975 (EGFR_T790M+), A431 (wtEGFR), H1299 (nRAS-driven Lung Cancer), and A549 (kRAS-driven Lung Cancer) cell lines compared to the non-cancerous EGFR-negative Jurkat cell line (T-lymphocyte) (Figure 5). Notably, EAE demonstrated selective inhibition of EGFR-positive lung cancer cells (Figures 5A–D), and no significant inhibition, even at higher extract concentrations, of the EGFR-negative Jurkat cells was observed (Figure 5E). Like EAE, acetone extract was also effective against all the EGFR-positive cell types except against the A549 cell line. Importantly, with the p-value of < 0.0001, the inhibitory activity of acetone extract on the EGFR_WT-positive A431 cell line (IC50 = 61.9 μg/mL) was significantly superior to that of EAE (IC50 = 196.7 μg/mL) (Figure 5B). However, it was equally effective against the EGFR-negative non-lung cancer Jurkat cell line, suggesting its non-specific cytotoxicity against all cell types (Figure 5E). These results together suggest superior inhibition of EGFR-positive lung cancer cell types by the EAE and its preferential selectivity for the EGFR_T790M mutant phenotype over the acetone extract. Their respective IC50 is given in Figure 5F. EAE exhibited 3-fold more potent inhibitory activity against the EGFR_T790M mutant (IC50: 53.3 μg/mL) compared to EGFR_WT (IC50: 196.7 μg/mL) (Figure 5F), suggesting the presence of an enriched bioactive content in EAE that is more specific against the EGFR_T790M mutant. While acetone extract [IC50: 73.9 μg/mL (EGFR_T790M) vs 61.9 μg/mL (EGFR_WT)] showed inhibitory activities against both EGFR variants, the difference in their effective concentrations was relatively lower compared to EAE. In addition, the acetone extract exhibited nonspecific inhibition in EGFR-negative Jurkat cells. Hence, Acetone extract was excluded, and EAE was selected for further experiments.
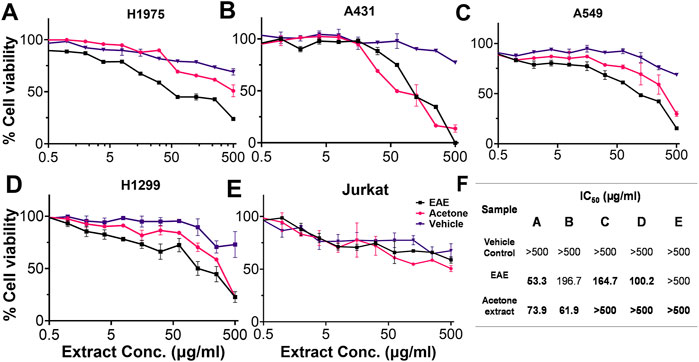
Figure 5. MTT assay showing cytotoxic effects of EAE and Acetone extract on various cell lines. Dose-response curves (X-axis: extract concentration; Y-axis: % cell viability) and IC50 values (calculated using GraphPad Prism v.9.3.1) are shown for (A) H1975, (B) A431, (C) A549, (D) H1299, (E) Jurkat cells. (F) Table summarizes IC50 values. Data are mean ± SD from three experiments (p < 0.05). (n = 3).
3.3 EAE exhibits a strong binding affinity for the mutant EGFR
The MST analysis of ligand-protein interactions provides information on the binding strength between them by analyzing the movement of biomolecules over a temperature gradient. This temperature gradient influences the molecular properties, such as the interacting partners’ charge, size, hydration shell, and conformations. Accordingly, binding affinities of EAE with the purified EGFR_TK and EGFR_T790M were determined. The data from triplicate experiments was used to plot the dose-response curves, and the statistical significance of the differences in binding kinetics was calculated using the unpaired t-test with an error margin of 5% (p < 0.05). Interestingly, EAE showed stronger interaction with the EGFR_T790M variant with a Kd value of 0.670 µg compared to EGFR_TK, which had a Kd value of 1.01 µg (Figure 6). The results obtained here are well aligned with those from the kinase inhibition assay.
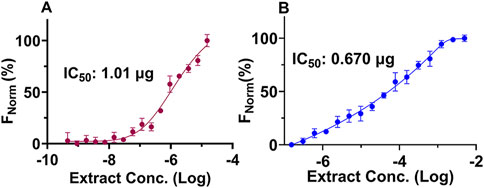
Figure 6. MST analyses of protein-ligand interactions. Two-fold serial dilutions of EAE were incubated with EGFR_TK and EGFR_T790M protein, and the reactions were analyzed on the Monolith NT.115 instrument. The mean of the % normalized fluorescence [FNorm (%), Y-axis] for the two EGFR proteins calculated from triplicate experiments was plotted against the EAE concentrations (X-axis) to generate the dose-response curves and to derive the Kd values. Error bars represent the standard deviation between the three experimental data sets. (A) MST binding curve of EGFR_TK with EAE, and (B) MST binding curve of EGFR_T790M with EAE. (n = 3).
3.4 EAE treatment enhances induction of apoptosis
Since IC50 values for EAE in different cell types ranged between 50–200 μg/mL, all future studies used 100 μg/mL and 200 μg/mL of EAE. Accordingly, different cell types used in this study were treated with/without 100 μg/mL or 200 μg/mL of EAE for 48 h and subsequently stained with Annexin V/PI. Post-staining flow cytometry analysis revealed a higher rate of apoptosis in EAE-treated cells compared to untreated or vehicle-treated cells (Figure 7). Consistent with the preliminary findings in this study, EAE treatment resulted in a higher percentage of H1975 (EGFR_T790M mutant) cells exhibiting the apoptotic phenotype than A431 cells (EGFR_WT) in a dose-dependent manner. Accordingly, ∼39.8% of H1975 cells were positive for the apoptotic phenotype compared to ∼13% of A431 cells at 100 μg/mL EAE treatment, which increased to ∼45.6% in H1975 cells compared to ∼32.1% of A431 cells at 200 μg/mL (Figures 7A,B). Notably, EAE induces apoptosis in EGFR_T790M cells without progressing to necrosis, while higher doses have less effect on H1975 cells, highlighting its selective apoptotic effect. Contrary to its effects on H1975 and A431 cell types, EAE had a variable impact on the other two lung cancer cell lines, A549 and H1299 (Figures 7C,D). Apoptosis was observed in 10% of A549 cells and 44.6% of H1299 cells treated with 100 μg/mL EAE, compared to 41.4% of A549 cells and 49.7% of H1299 cells treated with 200 μg/mL. Interestingly, irrespective of the EAE dosage, no detectable transition from apoptotic to necrotic phenotype was observed in either A549 or H1299 cells (0.42% at 100 μg/mL vs. 0.20% at 100 μg/mL) compared to untreated cells (Figures 7C,D). These results suggest that apoptosis induction was dose-dependent in A549 and H1299 cells. However, only A549 cells exhibited necrotic phenotype, which was also dose-dependent.
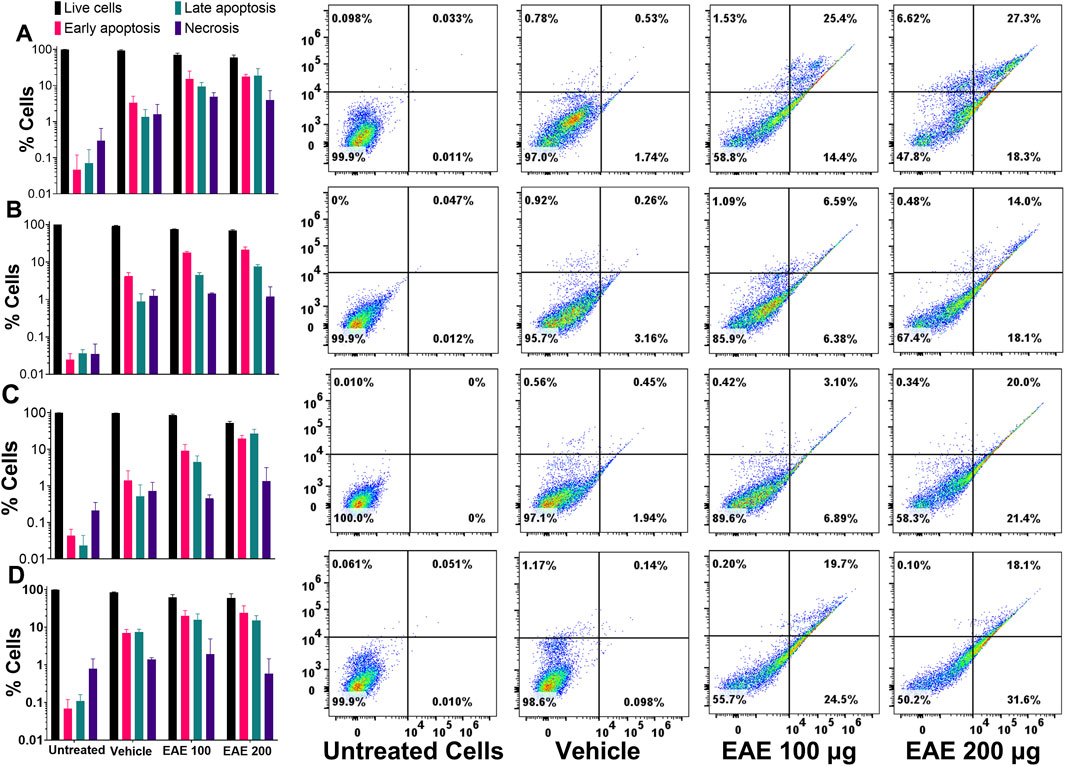
Figure 7. (A) H1975, (B) A431, (C) A549, (D) H1299 Cells. The Bar graph on the left and their corresponding four quadrant on the right side, shows the effects of EAE treatment on the induction of apoptotic phenotype and their progression to the necrotic phenotype in different cell types. Different cell types were treated with/without EAE for 48 h, stained with AnnexinV and PI, and analyzed by flow cytometry. Cells positive for the necrotic phenotype were gated in Q1, those in the late apoptotic state were gated in Q2, those in the early apoptotic state in Q3, and healthy cells in Q4. Data was plotted using the GraphPad Prism v9.3.1. Statistical significance was analyzed using the in-built statistical methods. A p-value of <0.05 was considered significant, indicating a p < 0.0001. The plotted data shown here represent three independent experiments, and the error bars represent the standard deviation between them. (n = 3).
Selective induction of apoptosis in cells expressing EGFR genes after EAE treatment was further confirmed by TUNEL assay. After 48 h EAE treatment with 100 μg/mL and 200 μg/mL, H1975 cells showed a notable increase in staining intensity, indicative of apoptotic activity, compared to untreated and vehicle-treated cells (Figure 8A). Similarly, untreated or vehicle-treated A431 cells hardly showed any staining. However, they showed notable, albeit relatively less compared to that in H1975 cells, TUNEL-positivity after EAE treatment in a dose-dependent manner (Figure 8B). Similar to that in the H1975 and A431 cells, A549 cells too showed an increase in TUNEL-positive cells only after the EAE treatment (Figure 8C). On the other hand, control H1299 cells showed intense staining. This staining increased marginally upon EAE treatment (Figure 8D). Overall, these results in different lung cancer cell types agree with the flow cytometry data discussed above on the apoptotic state.
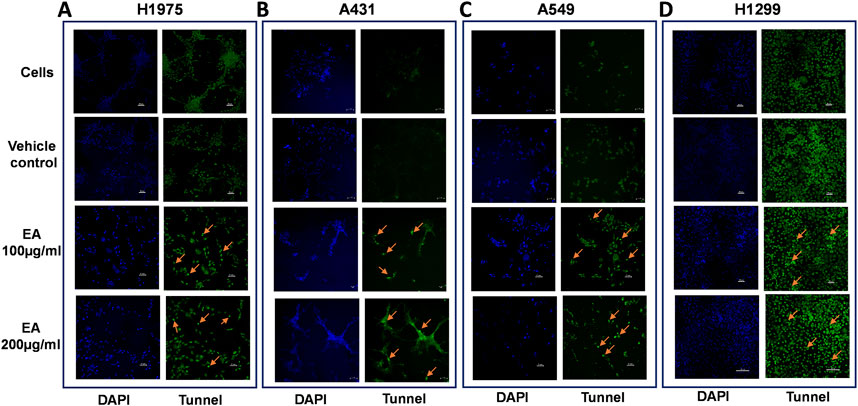
Figure 8. Shows TUNEL staining, indicative of apoptotic state, in different lung cancer cell types. Cells were treated with the selected concentrations (100 and 200 μg) of EAE and stained using the TUNEL assay kit. Different treatment variables are represented on the left. Blue-stained cells in the image represent DAPI staining, and those stained green represent FITC or TUNEL signal. The representative images from one of the three TUNEL assays are shown. TUNEL-positive cells among the fluorescently-stained cells are indicated with an arrow. (A) H1975 cells, (B) A431 cells, (C) A549 cells, (D) H1299 cells. (n = 3).
3.5 EAE selectively induces G1/G0 phase cell cycle arrest in H1975 cells
All four lung cancer cells were treated with DMSO (vehicle) or 100 or 200 μg/mL of EAE for 48 h and analyzed for cell cycle stages by flow cytometry. Data from vehicle-treated cells were compared to those from EAE-treated cells. Irrespective of the cell type, EAE treatment increased the number of cells captured at the G1/G0 phase, which was dose-dependent (Figure 9A). These results suggest that EAE treatment generally causes cell cycle arrest at the G1\G0-phase. However, the effect is relatively more specific to cells expressing the EGFR_T790M mutant phenotype, as none of the other cell types exhibited the trend observed with the H1975 cell line (Figures 9B–D).
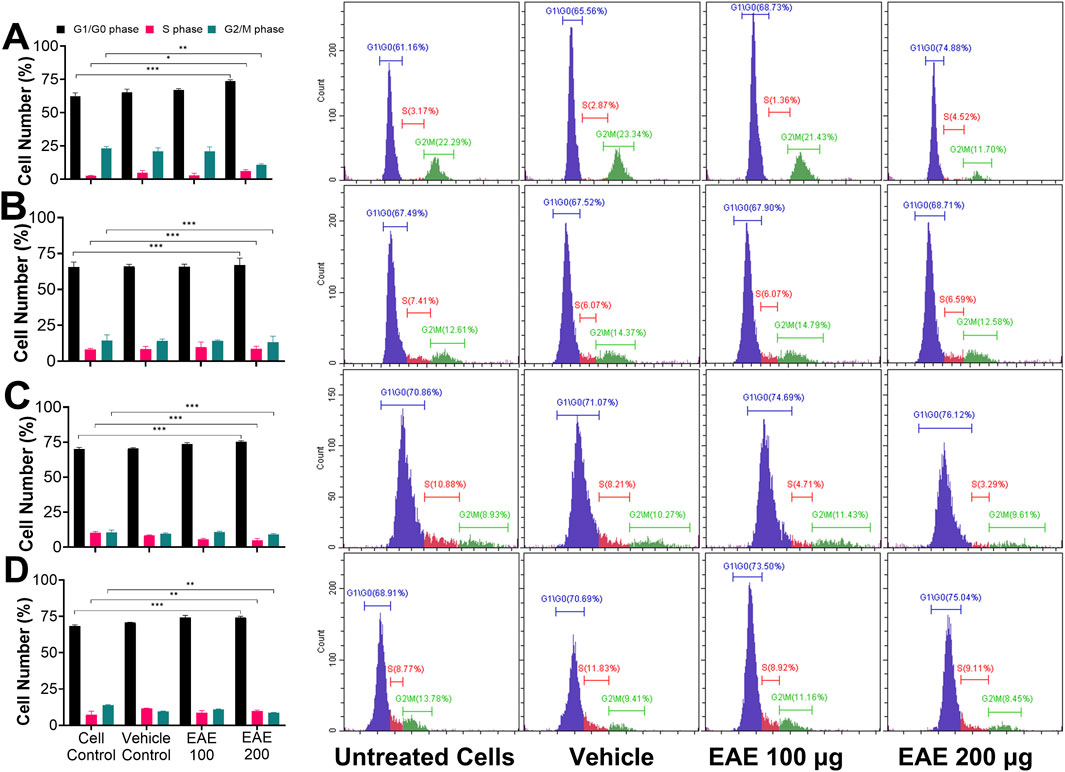
Figure 9. Shows the inhibitory effects of EAE on the cell cycle in different cell types. Cells were left untreated (cell control) or treated with DMSO (vehicle control) or EAE at 100 or 200 μg for 48 h and stained with PI. Cell suspensions were then analyzed on the flow cytometer and quantitated based on the cell cycle phase. These experiments were performed in triplicates, and representative data from one of the replicate experiments is presented here. Cells at the G1/G0, S, and G2/M phases of the cell cycle are colored differently, and their percentages in the total cell population in the sample are indicated. (A) H1975 cells, (B) A431 cells, (C) A549 cells, (D) H1299 cells. Figure (Bar graphs) also shows the mean percentages of cells at different cell cycle phases with their respective standard deviations between the triplicate assay data, plotted as a bar graph against the treatment type using Cytoflex software Cytxpert 2.5. A two-sample t-test was performed to estimate the statistical significance, and a p-value < 0.05 is considered significant. *, p < 0.05; **, p < 0.005; ***, p < 0.0001. (n = 3).
3.6 EAE inhibits EGFR phosphorylation at Tyr1068/1173 and affects EGFR-mediated signaling
Since EGFR phosphorylation is associated with tumor progression, we used Tyr1068/1092 and Tyr1173/1197 as reference sites. These sites were used to study the effect of EAE treatment on phosphorylation and its subsequent effects on the EGFR signaling cascade. Total cell lysates after 48 h EAE treatment were subjected to immunoblotting using mAb specific to unphosphorylated EGFR and phosphorylated EGFR at Tyr1068/1092 and Tyr1173/1197. Phosphorylated and unphosphorylated EGFR levels were normalized to the total EGFR, with basal expression as the reference. All four lung cancer cells consistently expressed the EGFR, but the levels of EGFR phosphorylated at Tyr1068/1092 and Tyr1173/1197 varied between cell types (Figures 10A–D). H1975 cells exhibited a notable reduction in EGFR phosphorylation at both the tyrosine residues in response to both concentrations of EAE (Figure 10A). In contrast, the other three cell types responded differently (Figures 10B–D). However, a general decreasing trend in EGFR phosphorylation is observed across the cell types. It may be noted that inhibition of Tyr1068/1092 phosphorylation leads to the arrest of the EGFR pathway early during the signaling cascade. In contrast, inhibition of Tyr1173/1197 phosphorylation leads to the arrest during the later signaling phases. Accordingly, the more decisive influence of EAE on H1975 cells can be attributed to its dual and more potent effects at both the tyrosine phosphorylation sites in a highly dose-dependent manner. In other cell types, the influence of EAE was weaker, which may be explained by its inhibitory effects predominantly on one of the two tyrosine phosphorylation sites.
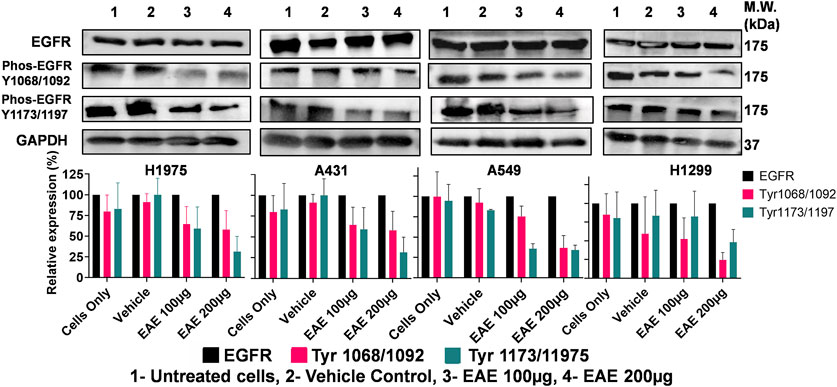
Figure 10. Immunoblot analysis of EGFR phosphorylation inhibition in various cell lines treated with EAE. Panel shows: (A) H1975 cells, (B) A431 cells, (C) A549 cells, (D) H1299 cells. Cells were untreated or treated with DMSO (vehicle control) or EAE (100 and 200 μg) for 48 h. Cell lysates were analyzed for EGFR, phospho-EGFR (Tyr1068/1092 and Tyr1173/1197), and GAPDH (loading control) by immunoblotting. The experiment was performed in triplicates, so the densitometry analysis, represented as bar graphs with error bars, showed significant inhibition of EGFR phosphorylation in all cell lines following EAE treatment compared to control expression of EGFR across all cell lines. (n = 3).
3.7 Binder molecules specific to EGFR_T790M in EAE
To identify the chemical composition of EAE, mass spectrometry analysis was carried out on the whole extract isolated in Ethyl acetate (Supplementary Figures S2A,B). This analysis revealed that EAE comprises several classes of bioactive molecules, such as alkaloids, polyketides, coumarins, terpenoids, flavonoids, quinolines and other classes. The distribution of the top 85 most abundant molecules (Supplementary Mass Spectrometry Data), categorized by molecular class, is illustrated in a pie chart (Figure 11A). To identify specific bioactive molecules within EAE that bind to EGFR_T790M, pull-down assays followed by mass spectrometry analysis were conducted (Supplementary Figures S3A,B), using EGFR_TK (Supplementary Figures S4A,B) as a background control. Following this analysis, molecules common to both the EGFR variants were subtracted to identify binder molecules unique to the EGFR_T790M. From this analysis, we identified 30 molecules having an MS/MS match above 80%, of which 6 molecules were found to have drug-like properties as determined by Swiss-ADME analysis. The chemical structures and the molecular information of these molecules are illustrated in Figure 11B. These include Balticol F, 4,7,8-trimethoxyfuro [2,3-b]quinoline, Communesin A, Talaroenamine D, Thailanstatin A, and Chryxanthone B, which have shown the critical ADME properties and are presented in Supplementary Table S1.
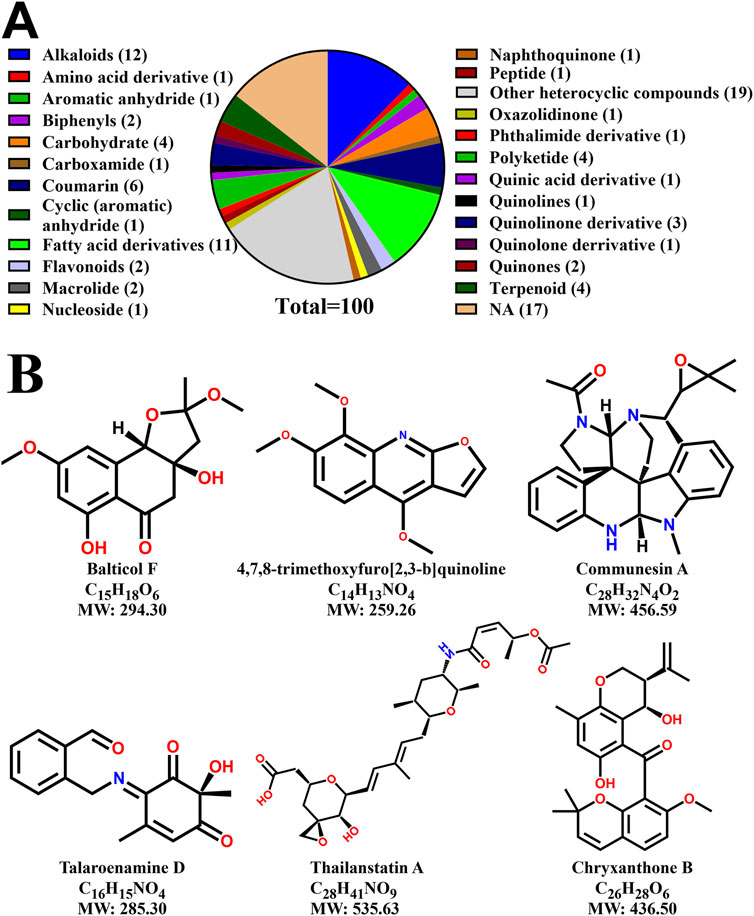
Figure 11. Characterization of EAE and identification of potential EGFR_T790M-specific binders from the mass spectrometry analysis. (A) A pie chart showing the most abundant molecules in the EAE, their respective classes and the number of molecules identified in each class, (B) Chemical structures of unique binder molecules of EGFR_T790M having drug-like properties, as determined by SWISS-ADME analysis.
4 Discussion
The present study investigated the kinase inhibitory activities of different solvent extracts from the leaves of R. graveolens against EGFR_TK and EGFR_T790M mutant. Our results from the kinase-inhibition assays suggested that the EAE was relatively more effective against the mutant, highlighting its selectivity against the drug-resistant NSCLC. Some of the studies in the published literature have explored the therapeutic potential of synthetic molecules targeting the EGFR_T790M(X. Tang et al., 2021; Zhou et al., 2009). Osimertinib, a third generation TKI, have shown such prominent effectiveness (Zhou et al., 2009; Nishino and Hatabu, 2017; Planchard et al., 2015; Song et al., 2016; Goss et al., 2016; Wu et al., 2018). However, the development of resistance and off-target effects made them less effective, necessitating exploring alternative or complementary therapeutic strategies. Unfortunately, while a plethora of information exists on synthetic molecules or purified natural compounds, to the best of our knowledge, studies showing the activity of plant extracts against EGFR_T790M using in vitro kinase inhibition assays are lacking. The specificity of EAE for the mutant EGFR was further established through cell-based experiments. Here, while EAE treatment led to a decrease in the viability across different cell types, the effect was relatively more pronounced in H1975 cells that express the EGFR_T790M mutant. Further, there was dose-dependency in the EAE-mediated cytotoxicity in H1975 cells. This further substantiated our claim that the EAE selectively inhibits drug-resistant NSCLC cells more effectively than their wild-type counterparts. Solvent extracts from medicinal plants rich in various bioactive molecules, like flavonoids, alkaloids, etc., have been shown to modulate EGFR-signalling pathways and subsequently cause cell cycle arrest and/or regression of cancer growth (Chun et al., 2013; Wang et al., 2018). In this context, our findings are in line with the existing literature on the potential ability of the plant extracts to exert selective inhibitory effects on the EGFR_T790M (Tang et al., 2021).
About the mechanism of inhibition, the flow cytometric analysis presented here suggested that the EAE treatment induced cell cycle arrest at the G1 phase. This cell cycle arrest indicates the EAE-mediated antiproliferative effect of EAE and is in complete agreement with the observed reduction in viability. Further, EAE treatment increased apoptosis, as evidenced quantitatively by Annexin/PI assay and qualitatively by TUNEL assay. The whole R. graveolens extract has been used in other cancer cells to understand its anticancer properties and it was found to induce apoptosis by interfering with p53, AKT pathways and causing the cell cycle arrest at G2/M phase (Wu et al., 2022). Studies were conducted on different lung cancer cell lines, including H1975 cells, in which ethanol extract of Scutellaria baicalensis and triterpenes extracted from H. diffusa effectively induced apoptosis by inactivating STAT3 in EGFR-TK-resistant cells (Park et al., 2021). These studies reveal that the active metabolite from plant extracts can combat different cancers, including drug-resistant lung cancer, and cause apoptosis. Our results suggested that the EAE treatment causes cell cycle arrest, inhibiting cell proliferation, and activating programmed cell death in drug-resistant NSCLC cells. This is consistent with previous studies, where alkaloid and polyphenolic compounds in medicinal plant extracts induced increased apoptosis (Ge et al., 2023; Elkady, 2013; Ratheesh, Sindhu, and Helen, 2013; Stalikas, 2007). These findings further support the multi-target therapeutic ability of natural products in cancer therapy. Next, to investigate the molecular basis for EAE-mediated inhibition of cell cycle arrest and/or activation of apoptosis, western blot analyses were performed on lysates prepared from EAE-treated cells. Here, dose-dependent suppression of phosphorylation at Tyr1068 and Tyr1173 residues of EGFR_TK was demonstrated, which was relatively higher and consistent between the experimental replicates in EAE-treated H1975 cells. These results were in order of the previous study by Wang et al. (2018), Liu and Gao (2020), Jiang et al. (2017), Qin et al. (2020), showing EGFR and pEGFR 1068 inhibition, Tang et al. (2016), Perera et al. (2005), van Rosenburgh et al. (2022) shown p1173 inhibition. The inhibition of EGFR phosphorylation at these sites agrees with a better inhibitor of EGFR_T790M.
Previous studies have shown that phosphorylated EGFR at Tyr1068 plays a crucial role in (i) recruitment of growth factor receptor-bound protein 2 (GRB2), an adapter protein (Okutani et al., 1994; Rojas et al., 1996; Yamauchi et al., 1997), which is pivotal in the activation of the Ras-MAPK signaling pathway in response to epidermal growth factor (EGF) (Sebastian et al., 2006), and (ii) serves as a docking site for proteins with the SH2 domain of signal transducer and activator of transcription 3 (STAT3) (Shao et al., 2003). Similarly EGFR phosphorylated at Tyr1173 facilitates the binding of SHC protein (Okabayashi et al., 1994). So it is well-established that the inhibition of phosphorylation at these key sites effectively disrupts EGFR signaling, thereby the viability and proliferation of NSCLC cells (Tang et al., 2021; Wang et al., 2018; Ma et al., 2020; Liu and Gao, 2020; Jiang et al., 2017; Qin et al., 2020; Tang et al., 2016; Perera et al., 2005; van Rosenburgh et al., 2022), Also, inhibition of phosphorylation of EGFR_TK was reported after treatment with plant-derived bioactive metabolites, like curcumin (Starok et al., 2015; Zhen et al., 2014), resveratrol (Wang et al., 2020), etc.
Further, pull-down assays using EGFR_TK and EGFR_T790M in the presence of EAE, followed by mass spectrometric analyses of the metabolic content, were performed to identify metabolite content unique to the mutant EGFR. Analysis of the data suggested the identification of a variety of bioactive metabolites belonging to different classes of phytochemicals, including alkaloids, polyketides, etc. Computational analyses of these metabolites for drug-like properties enabled us to refine the list of metabolites, which have now formed the basis for further investigation and validation of their therapeutic potential. Prior literature has also reported on the selective kinase inhibitory potential of these secondary metabolites, further validating our study outcomes (Gowtham et al., 2024; Marston, 2011). Sambo et al., have shown the effective inhibition of kinase activity and in vitro downregulation of protein kinases in lung cancer cell lines by identifying known anticancer metabolites of Ziziphus mucronata (Sambo et al., 2025).
Ruta graveolens is a well-studied plant against various ailments such as infectious diseases, neurological disorders, inflammatory diseases, cardiovascular diseases, gastrointestinal diseases, metabolic diseases, dermatological conditions, etc .,(Ratheesh et al., 2013; Jinous, 2012; Eickhorst et al., 2007). It is rich in alkaloids, flavonoids, coumarins, and essential oils, which are known to exert antimicrobial, anti-inflammatory, antioxidant, and anticancer properties (Jinous, 2012). With specific regard to cancer treatment, extracts from at least four of the above-cited Ruta species have been investigated for their potential against colon cancer, breast cancer, lung cancer, leukemia, etc., (Fadlalla et al., 2011; Gentile et al., 2015; Varamini, Soltani, and Ghaderi, 2009; Richardson et al., 2016; Pathak et al., 2003; Arora and Tandon, 2015; Pushpa et al., 2015; Schelz et al., 2016; Fuselier et al., 2023). Mechanistic studies attributed their anticancer potential to the efficient induction of apoptosis, inhibition of cell proliferation, and induction of oxidative stress (Gentile et al., 2015; Freyer et al., 2014). Among Ruta species, R. graveolens has been extensively investigated as cancer therapeutics, including in clinical trials (Gentile et al., 2015; Freyer et al., 2014). In one such trial, Ruta was given with Ca3(PO4)2 to treat brain cancers, particularly gliomas. As part, respective brain cancer cells were treated with the Ruta extracts ex vivo and injected into human patients. This resulted in complete regression of tumors in six out of seven glioma patients (Pathak et al., 2003).
The present study’s findings strongly supported the therapeutic potential of EAE from R. graveolens in treating NSCLC, particularly in those carrying the EGFR_T790M mutation. The multiple inhibitory mechanisms exerted by EAE from R. graveolens, including cell cycle disruption, apoptosis induction, and direct inhibition of EGFR phosphorylation, highlight its promise as a natural anticancer agent. Future studies should focus on isolating and characterizing the active metabolites from the EAE preparation from the leaves of R. graveolens to refine and enhance their efficacy as EGFR_T790M mutant-specific NSCLC therapeutics. Additionally, in vivo studies and clinical evaluations are warranted to translate the findings of this study into potential treatment strategies for patients with drug-resistant NSCLC cancer. The alignment of our results with prior research on plant-based kinase inhibitors highlights the growing relevance of natural products in anticancer drug discovery.
Data availability statement
The raw data supporting the conclusions of this article will be made available by the authors, without undue reservation.
Ethics statement
Ethical approval was not required for the studies on humans in accordance with the local legislation and institutional requirements because only commercially available established cell lines were used. Ethical approval was not required for the studies on animals in accordance with the local legislation and institutional requirements because only commercially available established cell lines were used.
Author contributions
VK: Conceptualization, Investigation, Methodology, Project administration, Validation, Visualization, Writing – original draft, Writing – review and editing, Data curation, Formal Analysis, Software. LC: Investigation, Methodology, Writing – review and editing, Resources. DS: Investigation, Data curation, Formal Analysis, Writing – review and editing. AK: Data curation, Formal Analysis, Investigation, Writing – review and editing, Methodology. RK: Data curation, Investigation, Methodology, Resources, Writing – review and editing. TK: Writing – review and editing, Data curation, Formal Analysis, Software. KB: Data curation, Formal Analysis, Validation, Writing – review and editing. RS: Data curation, Formal Analysis, Funding acquisition, Investigation, Methodology, Resources, Software, Visualization, Writing – review and editing. SK: Funding acquisition, Investigation, Resources, Project administration, Supervision, Writing – review and editing. SG: Funding acquisition, Resources, Conceptualization, Validation, Writing – review and editing. PH: Resources, Formal Analysis, Investigation, Methodology, Writing – original draft. SD: Investigation, Writing – original draft. JR: Investigation, Data curation, Formal Analysis, Methodology, Writing – original draft. DS: Investigation, Conceptualization, Data curation, Funding acquisition, Methodology, Project administration, Resources, Supervision, Validation, Visualization, Writing – original draft, Writing – review and editing. MBA: Data curation, Funding acquisition, Supervision, Validation, Visualization, Writing – review and editing, Formal Analysis, Writing – original draft. Dr. Krishna Kishore KI: Formal Analysis, Supervision, Writing – original draft, Writing – review and editing, Conceptualization, Data curation, Funding acquisition, Investigation, Methodology, Project administration, Resources, Software, Validation, Visualization.
Funding
The author(s) declare that financial support was received for the research and/or publication of this article. KI: Financially supported by grants from DST_SERB (Ref. # CRG/2019/003546) to KI, SG and DS, and ICMR (Ref. # ISRM/12(39)/2019) to KI and DS. Science and Engineering Research Board (# EEQ/2022/000,170), and Indian Council of Medical Research (IIRP-2023-4174/F1) to KI and (IIRP-2023-1172/F1) to KI and DS. DS: School of Natural Sciences, Shiv Nadar Institute of Eminence, Gautam Buddha Nagar, for supporting financially and providing other research facilities. MA: Grant-in-Aid support from BIRAC-NBM (Ref # BT/COVID0035/01/20), core R&D grants from M/s Srikara Biologicals Pvt. Ltd. (SBPL) and Grant-in-Aid support from DBT (# BT/PR24406/MED/15/175/2017) RS: DST-FIST Confocal Microscope Facility [Department of Science and Technology (Grant No. SR/FST/LS-1/2017/59(c)], DS, SNIoE University Noida.
Conflict of interest
The authors declare that the research was conducted in the absence of any commercial or financial relationships that could be construed as a potential conflict of interest.
Generative AI statement
The author(s) declare that no Generative AI was used in the creation of this manuscript.
Publisher’s note
All claims expressed in this article are solely those of the authors and do not necessarily represent those of their affiliated organizations, or those of the publisher, the editors and the reviewers. Any product that may be evaluated in this article, or claim that may be made by its manufacturer, is not guaranteed or endorsed by the publisher.
Supplementary material
The Supplementary Material for this article can be found online at: https://www.frontiersin.org/articles/10.3389/fphar.2025.1570108/full#supplementary-material
Abbreviations
EAE, Ethyl Acetate Extract; EGFR, Epidermal Growth Factor Receptor; EGFR_TK, EGFR Tyrosine Kinase (wild-type); EGFR_T790M, Substitution of threonine (T) with a methionine (M) at position 790 of exon 20 of EGFR; SCLC, Small-Cell Lung Cancer; NSCLC, Non-Small Cell Lung Cancer; TKI, Tyrosine Kinase Inhibitors; FDA, Food and Drug Administration; DMSO, Dimethyl Sulfoxide; TUNEL, Terminal deoxynucleotidyl transferase [TdT] dUTP Nick-End Labeling.
References
Abraham, R. T., and Arthur, W. (2004). Jurkat T cells and development of the T-cell receptor signalling paradigm. Nat. Rev. Immunol. 4 (4), 301–308. doi:10.1038/nri1330
Aherne, S. A., and O’Brien, N. M. (2000). Mechanism of protection by the flavonoids, quercetin and rutin, against tert-butylhydroperoxide- and menadione-induced DNA single strand breaks in caco-2 cells. Free Radic. Biol. Med. 29 (6), 507–514. doi:10.1016/S0891-5849(00)00360-9
Aiebchun, T., Mahalapbutr, P., Auepattanapong, A., Khaikate, O., Seetaha, S., Tabtimmai, L., et al. (2021). Identification of vinyl sulfone derivatives as EGFR tyrosine kinase inhibitor: in vitro and in silico studies. Molecules 26 (8), 2211. doi:10.3390/molecules26082211
Arora, S., and Tandon, S. (2015). DNA fragmentation and cell cycle arrest: a hallmark of apoptosis induced by Ruta graveolens in human colon cancer cells. Homeopathy 104 (1), 36–47. doi:10.1016/j.homp.2014.10.001
Baier, A., and Szyszka, R. (2020). Compounds from natural sources as protein kinase inhibitors. Biomolecules 10 (11), 1546. doi:10.3390/biom10111546
Bajaj, M., Waterfield, M. D., Schlessinger, J., Taylor, W. R., and Blundell, T. (1987). On the tertiary structure of the extracellular domains of the epidermal growth factor and insulin receptors. Biochim. Biophys. Acta 916 (2), 220–226. doi:10.1016/0167-4838(87)90112-9
Baker, D. H. A., Ibrahim, E. A., Ahmed, K., and El Baz, F. K. (2017). Sterols bioactivity of Ruta graveolens L. and Murraya paniculata L. Int. J. Pharm. Pharm. Sci. 9, 103–108. doi:10.22159/ijpps.2017v9i2.15790
Bartholomew, C., Eastlake, L., Dunn, P., and Yiannakis, D. (2017). EGFR targeted therapy in lung cancer; an evolving story. Respir. Med. Case Rep. 20, 137–140. doi:10.1016/j.rmcr.2017.01.016
Bernas, T., and Dobrucki, J. (2002). Mitochondrial and nonmitochondrial reduction of MTT: interaction of MTT with TMRE, JC-1, and NAO mitochondrial fluorescent probes. Cytometry 47 (4), 236–242. doi:10.1002/cyto.10080
Bray, F., Laversanne, M., Sung, H., Ferlay, J., Siegel, R. L., Soerjomataram, I., et al. (2024). Global cancer statistics 2022: GLOBOCAN estimates of incidence and mortality worldwide for 36 cancers in 185 countries. CA A Cancer J. Clin. 74 (3), 229–263. doi:10.3322/caac.21834
Brignola, P. S., Lackey, K., Kadwell, S. H., Hoffman, C., Horne, E., Luke Carter, H., et al. (2002). Comparison of the biochemical and kinetic properties of the type 1 receptor tyrosine kinase intracellular domains. Demonstration of differential sensitivity to kinase inhibitors. J. Biol. Chem. 277 (2), 1576–1585. doi:10.1074/jbc.M105907200
Chen, Ya, Liu, H., Hu, N., Wang, Y., Yang, Z., Zhang, J., et al. (2023). Survival benefit of anlotinib in T790M-positive non-small-cell lung cancer patients with acquired osimertinib resistance: a multicenter retrospective study and exploratory in vitro study. Cancer Med. 12 (15), 15922–15932. doi:10.1002/cam4.6232
Chun, J., Joo, E. J., Kang, M., and Kim, Y. S. (2013). Platycodin D induces anoikis and caspase-mediated apoptosis via P38 MAPK in AGS human gastric cancer cells. J. Cell. Biochem. 114 (2), 456–470. doi:10.1002/jcb.24386
De Rie, M. A., VAN Eendenburg, J. P., Vkrsnick, A. C., Stolk, L. M. L., Bos, J. D., and Westerhof, W. (1995). A new Psoralen-containing gel for topical PUVA therapy: development, and treatment results in patients with palmoplantar and plaque-type psoriasis, and hyperkeratotic eczema. Br. J. Dermatol. 132 (6), 964–969. doi:10.1111/j.1365-2133.1995.tb16956.x
Eickhorst, K., Vincent, D. L., and Joan, C. (2007). Rue the herb: Ruta graveolens-associated phytophototoxicity. DERM 18 (1), 52–55. doi:10.2310/6620.2007.06033
El Kadi, N., Wang, L., Davis, A., Korkaya, H., Cooke, A., Vadnala, V., et al. (2018). The EGFR T790M mutation is acquired through AICDA-mediated deamination of 5-methylcytosine following TKI treatment in lung cancer. Cancer Res. 78 (24), 6728–6735. doi:10.1158/0008-5472.CAN-17-3370
Elkady, A. I. (2013). Crude alkaloid extract of rhazya stricta inhibits cell growth and sensitizes human lung cancer cells to cisplatin through induction of apoptosis. Genet. Mol. Biol. 36 (1), 12–21. doi:10.1590/S1415-47572013005000009
Engel, K., Sasaki, T., Qi, W., and Kuriyan, J. (2013). A highly efficient peptide substrate for EGFR activates the kinase by inducing aggregation. Biochem. J. 453 (3), 337–344. doi:10.1042/BJ20130537
Fadlalla, K., Watson, A., Yehualaeshet, T., Turner, T., and Samuel, T. (2011). Ruta graveolens extract induces DNA damage pathways and blocks Akt activation to inhibit cancer cell proliferation and survival. Anticancer Res. 31, 233–241.
Freyer, G., You, B., Villet, S., Tartas, S., Fournel-Federico, C., Trillet-Lenoir, V., et al. (2014). Open-label uncontrolled pilot study to evaluate complementary therapy with Ruta graveolens 9c in patients with advanced cancer. Homeopathy 103 (4), 232–238. doi:10.1016/j.homp.2014.06.001
Fuselier, C., Dufay, E., Berquand, A., Terryn, C., Bonnomet, A., Molinari, M., et al. (2023). Dynamized ultra-low dilution of Ruta graveolens disrupts plasma membrane organization and decreases migration of melanoma cancer cell. Cell Adhesion Migr. 17 (1), 1–13. doi:10.1080/19336918.2022.2154732
Ge, Z., Xu, M., Ge, Y., Huang, G., Chen, D., Ye, X., et al. (2023). Inhibiting G6PD by quercetin promotes degradation of EGFR T790M mutation. Cell Rep. 42 (11), 113417. doi:10.1016/j.celrep.2023.113417
Gentile, M. T., Ciniglia, C., Reccia, M. G., Volpicelli, F., Gatti, M., Thellung, S., et al. (2015). Ruta graveolens L. Induces death of glioblastoma cells and neural progenitors, but not of neurons, via ERK 1/2 and AKT activation. PLoS One 10 (3), e0118864. doi:10.1371/journal.pone.0118864
Giard, D. J., Aaronson, S. A., Todaro, G. J., Arnstein, P., Kersey, J. H., Harvey, D., et al. (1973). In vitro cultivation of human tumors: establishment of cell lines derived from a series of solid tumors. J. Natl. Cancer Inst. 51 (5), 1417–1423. doi:10.1093/jnci/51.5.1417
Goss, G., Tsai, C.-M., Shepherd, F. A., Bazhenova, L., Lee, J. S., Chang, G.-C., et al. (2016). Osimertinib for pretreated EGFR Thr790Met-positive advanced non-small-cell lung cancer (AURA2): a multicentre, open-label, single-arm, phase 2 study. Lancet. Oncol. 17 (12), 1643–1652. doi:10.1016/S1470-2045(16)30508-3
Gowtham, H. G., Revanasiddappa, P. D., Murali, M., Singh, S. B., Abhilash, M. R., Pradeep, S., et al. (2024). Secondary metabolites of trichoderma spp. as EGFR tyrosine kinase inhibitors: evaluation of anticancer efficacy through computational approach. PloS One 19 (1), e0296010. doi:10.1371/journal.pone.0296010
Greulich, H., Chen, T.-H., Feng, W., Jänne, P. A., Alvarez, J. V., Zappaterra, M., et al. (2005). Oncogenic transformation by inhibitor-sensitive and -resistant EGFR mutants. PLoS Med. 2 (11), e313. doi:10.1371/journal.pmed.0020313
Guo, G.-G., Luo, X., Zhu, K., Ling-Ling, L. I., and Yang-Fan, O. U. (2023). Fatal ventricular arrhythmias after osimertinib treatment for lung adenocarcinoma: a case report. J. Geriatric Cardiol. 20 (3), 242–246. doi:10.26599/1671-5411.2023.03.009
Hale, A. L., Meepagala, K. M., Oliva, A., Aliotta, G., and Duke, S. O. (2004). Phytotoxins from the leaves of Ruta graveolens. J. Agric. Food Chem. 52 (11), 3345–3349. doi:10.1021/jf0497298
Herst, P. M., and Berridge, M. V. (2013). Cell hierarchy, metabolic flexibility and systems approaches to cancer treatment. Curr. Pharm. Biotechnol. 14 (3), 289–299. doi:10.2174/1389201011314030005
Hirsch, F. R., and Bunn, P. A. (2009). EGFR testing in lung cancer is ready for prime time. Lancet Oncol. 10 (5), 432–433. doi:10.1016/S1470-2045(09)70110-X
Hodoglugil, U., Carrillo, M. W., Hebert, J. M., Karachaliou, N., Rosell, R. C., Altman, R. B., et al. (2013). PharmGKB summary: very important pharmacogene information for the epidermal growth factor receptor. Pharmacogenetics Genomics 23 (11), 636–642. doi:10.1097/FPC.0b013e3283655091
Inamura, K., Ninomiya, H., Ishikawa, Y., and Matsubara, O. (2010). Is the epidermal growth factor receptor status in lung cancers reflected in clinicopathologic features? Arch. Pathol. Lab. Med. 134, 66–72. doi:10.5858/2008-0586-rar1.1
Jiang, X.-M., Xu, Y.-L., Huang, M.-Y., Zhang, L.-L., Su, M.-X., Chen, X., et al. (2017). Osimertinib (AZD9291) decreases programmed death ligand-1 in EGFR-mutated non-small cell lung cancer cells. Acta Pharmacol. Sin. 38 (11), 1512–1520. doi:10.1038/aps.2017.123
Jinous, A. (2012). Phytochemistry and pharmacological properties of Ruta graveolens L. J. Med. Plants Res. 6 (23). doi:10.5897/JMPR12.040
Kuzovkina, I., Al’terman, I., and Schneider, B. (2004). Specific accumulation and revised structures of acridone alkaloid glucosides in the tips of transformed roots of Ruta graveolens. Phytochemistry 65 (8), 1095–1100. doi:10.1016/j.phytochem.2004.03.003
Liu, K., Chen, X., Wu, L., Chen, S., Fang, N., Cai, L., et al. (2021). ID1 mediates resistance to osimertinib in EGFR T790M-positive non-small cell lung cancer through epithelial–mesenchymal transition. BMC Pulm. Med. 21 (1), 163. doi:10.1186/s12890-021-01540-4
Liu, X., Jiang, T., Li, X., Zhao, C., Li, J., Zhou, F., et al. (2020). Exosomes transmit T790M mutation-induced resistance in EGFR-mutant NSCLC by activating PI3K/AKT signalling pathway. J. Cell. Mol. Med. 24 (2), 1529–1540. doi:10.1111/jcmm.14838
Liu, Z., and Gao, W. (2020). Synergistic effects of Bcl-2 inhibitors with AZD9291 on overcoming the acquired resistance of AZD9291 in H1975 cells. Archives Toxicol. 94 (9), 3125–3136. doi:10.1007/s00204-020-02816-0
Ma, Q., Wang, J., Ren, Y., Meng, F., and Zeng, L. (2020). Pathological mechanistic studies of osimertinib resistance in non-small-cell lung cancer cells using an integrative metabolomics-proteomics analysis. J. Oncol. 2020, 6249829. doi:10.1155/2020/6249829
Marston, A. (2011). Natural products as a source of protein kinase activators and inhibitors. Curr. Top. Med. Chem. 11 (11), 1333–1339. doi:10.2174/156802611795589575
Merlino, G. T., Xu, Y. H., Ishii, S., Clark, A. J., Semba, K., Toyoshima, K., et al. (1984). Amplification and enhanced expression of the epidermal growth factor receptor gene in A431 human carcinoma cells. Sci. (New York, N.Y.) 224 (4647), 417–419. doi:10.1126/science.6200934
Nan, X., Xie, C., Yu, X., and Liu, J. (2017). EGFR TKI as first-line treatment for patients with advanced EGFR mutation-positive non-small-cell lung cancer. Oncotarget 8 (43), 75712–75726. doi:10.18632/oncotarget.20095
Nishino, M., and Hatabu, H. (2017). Osimertinib in EGFR T790M-positive lung cancer. The New England Journal of Medicine. 376 (20), 1992–1993. doi:10.1056/nejmc1703339
Ohashi, K., Sequist, L. V., Arcila, M. E., Lovly, C. M., Chen, Xi, Rudin, C. M., et al. (2013). Characteristics of lung cancers harboring NRAS mutations. Clin. Cancer Res. 19 (9), 2584–2591. doi:10.1158/1078-0432.CCR-12-3173
Okabayashi, Y., Kido, Y., Okutani, T., Sugimoto, Y., Sakaguchi, K., and Kasuga, M. (1994). Tyrosines 1148 and 1173 of activated human epidermal growth factor receptors are binding sites of shc in intact cells. J. Biol. Chem. 269 (28), 18674–18678. doi:10.1016/s0021-9258(17)32363-3
Okutani, T., Okabayashi, Y., Kido, Y., Sugimoto, Y., Sakaguchi, K., Matuoka, K., et al. (1994). Grb2/Ash binds directly to tyrosines 1068 and 1086 and indirectly to tyrosine 1148 of activated human epidermal growth factor receptors in intact cells. J. Biol. Chem. 269 (49), 31310–31314. doi:10.1016/s0021-9258(18)47424-8
Park, H.-J., Park, S.-H., Choi, Y.-H., and Chi, G.-Y. (2021). The root extract of Scutellaria baicalensis induces apoptosis in EGFR TKI-resistant human lung cancer cells by inactivation of STAT3. Int. J. Mol. Sci. 22 (10), 5181. doi:10.3390/ijms22105181
Pathak, S., Multani, A., Banerji, P., and Banerji, P. (2003). Ruta 6 selectively induces cell death in brain cancer cells but proliferation in normal peripheral blood lymphocytes: a novel treatment for human brain cancer. Int. J. Oncol. 23, 975–982. doi:10.3892/ijo.23.4.975
Perera, R. M., Narita, Y., Furnari, F. B., Gan, H. K., Murone, C., Ahlkvist, M., et al. (2005). Treatment of human tumor xenografts with monoclonal antibody 806 in combination with a prototypical epidermal growth factor receptor–specific antibody generates enhanced antitumor activity. Clin. Cancer Res. 11 (17), 6390–6399. doi:10.1158/1078-0432.CCR-04-2653
Planchard, D., Loriot, Y., André, F., Gobert, A., Auger, N., Lacroix, L., et al. (2015). EGFR-independent mechanisms of acquired resistance to AZD9291 in EGFR T790M-positive NSCLC patients. Ann. Oncol. 26 (10), 2073–2078. doi:10.1093/annonc/mdv319
Poh, M. E., Balakrishnan, S., Tan, S. N., Abidin, M. A. Z., Liam, C. K., Tan, J. L., et al. (2024). Real-world efficacy of low dose osimertinib as second-line treatment in patients with epidermal growth factor receptor-mutated advanced non-small cell lung cancer. Transl. Lung Cancer Res. 13 (7), 1649–1659. doi:10.21037/tlcr-24-243
Politi, K., and Lynch, T. J. (2012). Two sides of the same coin: EGFR exon 19 deletions and insertions in lung cancer. Clin. Cancer Res. 18 (6), 1490–1492. doi:10.1158/1078-0432.CCR-11-3282
Pushpa, H., Shree, N. R., Shetty, S., and Ramesh, D. (2015). Screening of antimicrobial, antioxidant and anticancer activity of Ruta graveolens. Advances in Biological Research 9 (4), 257–264. doi:10.5829/idosi.abr.2015.9.94234
Qin, Q., Li, X., Liang, X., Zeng, L., Wang, J., Sun, L., et al. (2020). CDK4/6 inhibitor palbociclib overcomes acquired resistance to third-generation EGFR inhibitor osimertinib in non-small cell lung cancer (NSCLC). Thorac. Cancer 11 (9), 2389–2397. doi:10.1111/1759-7714.13521
Ratheesh, M., Sindhu, G., and Helen, A. (2013). Anti-inflammatory effect of quinoline alkaloid skimmianine isolated from Ruta graveolens L. Inflamm. Res. 62 (4), 367–376. doi:10.1007/s00011-013-0588-1
Réthy, B., Zupkó, I., Minorics, R., Hohmann, J., Ocsovszki, I., and George, F. (2006). Investigation of cytotoxic activity on human cancer cell lines of arborinine and furanoacridones isolated from Ruta graveolens. Planta Medica 73 (01), 41–48. doi:10.1055/s-2006-951747
Richardson, J. S. M., Sethi, G., Lee, G. S., and Malek, S. N. A. (2016). Chalepin: isolated from Ruta angustifolia L. Pers induces mitochondrial mediated apoptosis in lung carcinoma cells. BMC Comple. Altern. Med. 16 (1), 389. doi:10.1186/s12906-016-1368-6
Rojas, M., Yao, S., and Lin, Y. Z. (1996). Controlling epidermal growth factor (EGF)-Stimulated Ras activation in intact cells by a cell-permeable peptide mimicking phosphorylated EGF receptor. J. Biol. Chem. 271 (44), 27456–27461. doi:10.1074/jbc.271.44.27456
Sambo, T., Mathe, E., Shai, L., Mapfumari, S., and Gololo, S. (2025). Inhibition of kinase activity and in vitro downregulation of the protein kinases in lung cancer and cervical cancer cell lines and the identified known anticancer compounds of Ziziphus mucronata. Plants 14 (3), 395. doi:10.3390/plants14030395
Schelz, Z., Ocsovszki, I., Bózsity, N., Hohmann, J., and Zupkó, I. (2016). Antiproliferative effects of various furanoacridones isolated from Ruta graveolens on human breast cancer cell lines. Anticancer Res. 36 (6), 2751–2758.
Sebastian, S., Jeffrey, S., Reshkin, S. J., Azzariti, A., Bellizzi, A., and Paradiso, A. (2006). The complexity of targeting EGFR signalling in cancer: from expression to turnover. Biochim. Biophys. Acta 1766 (1), 120–139. doi:10.1016/j.bbcan.2006.06.001
Shah, R., and Lester, J. F. (2020). Tyrosine kinase inhibitors for the treatment of EGFR mutation-positive non–small-cell lung cancer: a clash of the generations. Clin. Lung Cancer 21 (3), e216–e228. doi:10.1016/j.cllc.2019.12.003
Shao, H., Cheng, H. Y., Cook, R. G., and Tweardy, D. J. (2003). Identification and characterization of signal transducer and activator of transcription 3 recruitment sites within the epidermal growth factor receptor. Cancer Res. 63 (14), 3923–3930.
Siegel, R. L., Miller, K. D., Wagle, N. S., and Jemal, A. (2023). Cancer statistics, 2023. CA A Cancer J. Clin. 73 (1), 17–48. doi:10.3322/caac.21763
Simon, G. R., and Wagner, H. (2003). Small Cell Lung Cancer*. Chest 123 (1, Supplement): 259S-271S. doi:10.1378/chest.123.1_suppl.259S
Song, H.-Na, Sun Jung, Ki, Yoo, K. H., Cho, J., Lee, Ji Y., Lim, S. H., et al. (2016). Acquired C797S mutation upon treatment with a T790M-specific third-generation EGFR inhibitor (HM61713) in non–small cell lung cancer. J. Thorac. Oncol. 11 (4), e45–e47. doi:10.1016/j.jtho.2015.12.093
Spence, C., and Spence, S. (2023). Weeds from the yard’: a seasonal salad. Int. J. Gastron. Food Sci. 33, 100776. doi:10.1016/j.ijgfs.2023.100776
Spira, A. (2004). Multidisciplinary management of lung cancer. N. Engl. J. Med. 350, 379–392. doi:10.1056/NEJMra035536
Stalikas, C. D. (2007). Extraction, separation, and detection methods for phenolic acids and flavonoids. J. Sep. Sci. 30 (18), 3268–3295. doi:10.1002/jssc.200700261
Starok, M., Preira, P., Vayssade, M., Haupt, K., Salomé, L., and Rossi, C. (2015). EGFR inhibition by curcumin in cancer cells: a dual mode of action. Biomacromolecules 16 (5), 1634–1642. doi:10.1021/acs.biomac.5b00229
Tang, X., Cheng, L., Li, G., Yan, Y.-M., Su, F., Huang, D.-L., et al. (2021). A small-molecule compound D6 overcomes EGFR-T790M-mediated resistance in non-small cell lung cancer. Commun. Biol. 4 (1), 1391–1413. doi:10.1038/s42003-021-02906-4
Tang, Z.-H., Jiang, X.-M., Guo, X., Fong, C. M. V., Chen, X., and Lu, J.-J. (2016). Characterization of osimertinib (AZD9291)-Resistant non-small cell lung cancer NCI-H1975/OSIR cell line. Oncotarget 7 (49), 81598–81610. doi:10.18632/oncotarget.13150
Thress, K. S., Paweletz, C. P., Felip, E., Cho, B. C., Stetson, D., Dougherty, B., et al. (2015). Acquired EGFR C797S mutation mediates resistance to AZD9291 in non-small cell lung cancer harboring EGFR T790M. Nat. Med. 21 (6), 560–562. doi:10.1038/nm.3854
Ting, C.-H., Lee, K.-Y., Wu, S.-M., Feng, P.-H., Chan, Y.-F., Chen, Y.-C., et al. (2019). FOSB–PCDHB13 axis disrupts the microtubule network in non-small cell lung cancer. Cancers 11 (1), 107. doi:10.3390/cancers11010107
van Rosenburgh, A., van, I. K., Lu, D. M., Grant, M. J., Stayrook, S. E., Phadke, M., et al. (2022). Biochemical and structural basis for differential inhibitor sensitivity of EGFR with distinct exon 19 mutations. Nat. Commun. 13 (1), 6791. doi:10.1038/s41467-022-34398-z
Varamini, P., Soltani, M., and Ghaderi, A. (2009). Cell cycle analysis and cytotoxic potential of Ruta graveolens against human tumor cell lines. Neoplasma 56 (6), 490–493. doi:10.4149/neo_2009_06_490
Vogelsang, G. B., Wolff, D., Altomonte, V., Farmer, E., Morison, W. L., Corio, R., et al. (1996). Treatment of chronic graft-versus-host disease with ultraviolet irradiation and psoralen (PUVA). Bone Marrow Transplant. 17 (6), 1061–1067.
Walter, A. O., Sjin, R. T. T., Haringsma, H. J., Ohashi, K., Sun, J., Lee, K., et al. (2013). Discovery of a mutant-selective covalent inhibitor of EGFR that overcomes T790M-mediated resistance in NSCLC. Cancer Discov. 3 (12), 1404–1415. doi:10.1158/2159-8290.CD-13-0314
Wang, A., Li, X., Wu, H., Zou, F., Yan, X.-E., Chen, C., et al. (2017). Discovery of (R)-1-(3-(4-Amino-3-(3-Chloro-4-(Pyridin-2-Ylmethoxy)Phenyl)-1 H -Pyrazolo[3,4- d ]Pyrimidin-1-Yl)Piperidin-1-Yl)Prop-2-En-1-One (CHMFL-EGFR-202) as a novel irreversible EGFR mutant kinase inhibitor with a distinct binding mode. J. Med. Chem. 60 (7), 2944–2962. doi:10.1021/acs.jmedchem.6b01907
Wang, J., Wang, B., Chu, H., and Yao, Y. (2016). Intrinsic resistance to EGFR tyrosine kinase inhibitors in advanced non-small-cell lung cancer with activating EGFR mutations. OncoTargets Ther. 9, 3711–3726. doi:10.2147/OTT.S106399
Wang, Y., Lai, H., Fan, X., Luo, L., Duan, F., Jiang, Z., et al. (2018). Gossypol inhibits non-small cell lung cancer cells proliferation by targeting EGFRL858R/T790M. Front. Pharmacol. 9, 728. doi:10.3389/fphar.2018.00728
Wang, Y., Wang, W., Wu, X., Li, C., Huang, Y., Zhou, H., et al. (2020). Resveratrol sensitizes colorectal cancer cells to cetuximab by connexin 43 upregulation-induced Akt inhibition. Front. Oncol. 10, 383. doi:10.3389/fonc.2020.00383
Wu, K., Wu, X., Liang, Y., Wang, T., Wu, D., Li, L., et al. (2022). Inhibitory effects of total triterpenoids isolated from the hedyotis diffusa willd on H1975 cells. Front. Pharmacol. 13, 922477. doi:10.3389/fphar.2022.922477
Wu, Y.-L., Ahn, M.-J., Chiara Garassino, M., Han, J.-Y., Katakami, N., Kim, H. R., et al. (2018). CNS efficacy of osimertinib in patients with T790M-positive advanced non-small-cell lung cancer: data from a randomized phase III trial (AURA3). J. Clin. Oncol. 36 (26), 2702–2709. doi:10.1200/JCO.2018.77.9363
Yamauchi, T., Ueki, K., Tobe, K., Tamemoto, H., Sekine, N., Wada, M., et al. (1997). Tyrosine phosphorylation of the EGF receptor by the kinase Jak2 is induced by growth hormone. Nature 390 (6655), 91–96. doi:10.1038/36369
Zhang, J., Vokes, N., Li, M., Xu, J., Bai, H., Wang, J., et al. (2024). Overcoming EGFR-TKI resistance by targeting the tumor microenvironment. Chin. Med. J. Pulm. Crit. Care Med. 2 (3), 151–161. doi:10.1016/j.pccm.2024.08.002
Zhao, B.-X., Wang, J., Song, B., Wei, H., Lv, W.-P., Tian, L.-M., et al. (2015). Establishment and biological characteristics of acquired gefitinib resistance in cell line NCI-H1975/gefinitib-resistant with epidermal growth factor receptor T790M mutation. Mol. Med. Rep. 11 (4), 2767–2774. doi:10.3892/mmr.2014.3058
Zhen, L., Fan, D., Yi, X., Cao, X., Chen, D., and Wang, L. (2014). Curcumin inhibits oral squamous cell carcinoma proliferation and invasion via EGFR signaling pathways. Int. J. Clin. Exp. Pathol. 7 (10), 6438–6446.
Zhou, W., Ercan, D., Chen, L., Yun, C.-H., Li, D., Capelletti, M., et al. (2009). Novel mutant-selective EGFR kinase inhibitors against EGFR T790M. Nature 462 (7276), 1070–1074. doi:10.1038/nature08622
Keywords: Lung cancer, NSCLC, EGFR_T790M mutation, Ruta graveolens, ethyl acetate extract
Citation: Kumar V, Chauhan L, Singh D, Kumar A, Kulandaisamy R, Kushwaha T, Baswal K, Singh R, Kumar S, Gholap SL, Hariprasad P, Dadinaboyina SB, Thota JR, Sehgal D, Appaiahgari MB and Inampudi KK (2025) Ethyl acetate extract of Ruta graveolens: a specific and potent inhibitor against the drug-resistant EGFR_T790M mutant in NSCLC. Front. Pharmacol. 16:1570108. doi: 10.3389/fphar.2025.1570108
Received: 02 February 2025; Accepted: 14 April 2025;
Published: 29 April 2025.
Edited by:
Li-She Gan, Zhejiang Chinese Medical University, ChinaReviewed by:
Olha Mykhailenko, University College London, United KingdomKwanchayanawish Machana, Nakhonratchasima College, Thailand
Copyright © 2025 Kumar, Chauhan, Singh, Kumar, Kulandaisamy, Kushwaha, Baswal, Singh, Kumar, Gholap, Hariprasad, Dadinaboyina, Thota, Sehgal, Appaiahgari and Inampudi. This is an open-access article distributed under the terms of the Creative Commons Attribution License (CC BY). The use, distribution or reproduction in other forums is permitted, provided the original author(s) and the copyright owner(s) are credited and that the original publication in this journal is cited, in accordance with accepted academic practice. No use, distribution or reproduction is permitted which does not comply with these terms.
*Correspondence: Krishna K. Inampudi, krishna.inampudi@aiims.edu