- 1Laboratório de Biofotônica, Instituto de Física de São Carlos (IFSC/Universidade de São Paulo), São Carlos, Brasil
- 2Hospital Amaral Carvalho, Jaú, Brasil
- 3Hospital Universitário Prof. Alberto Antunes of Universidade Federal de Alagoas, Maceió, Alagoas, Brasil
The photodynamic therapy (PDT) is an alternative technique that can be used for treating superficial basal cell carcinoma (sBCC), Bowen's disease and actinic keratosis with highefficiency. The objective of this study is to present a method where fluorescence imaging together with for PDT as a monitoring guidance in real time. Confirming that the lesion is well prepared and the photosensitizer shows a homogenous distribution, the outcome after few PDT sessions will be more predictable and the recurrence is minimized. Our proposition in this study is use the widefield fluorescence imaging to evaluate the PDT protocol in situ and in real time for each lesion. This evaluation procedure is performed in two steps: first with the monitoring of the production of protoporphyrin IX (PpIX) induced by methyl aminolevulinate (MAL), a derivative of 5-aminolevulinic acid (ALA) and second with the detection of PpIX photobleaching after illumination. The fluorescence images provide information correlated with distinct clinical features and with the treatment outcome. Eight BCC lesions are presented and discussed in this study. Different fluorescence patterns of PpIX production and photobleaching could be correlated with the treatment response. The presented results show the potential of using widefield fluorescence imaging as a guidance tool to customized PDT. This procedure is being incorporated to the main PDT skin cancer protocol applied in Brazil with an excellent outcome.
Introduction
Photodynamic therapy (PDT) is a treatment modality that can be used for several cancer types and pre-cancer lesions, like superficial basal cell carcinoma (sBCC), Bowen's disease and actinic keratosis. The PDT is based on the combination of a photosensitizer (PS), the molecular oxygen within the cells and a suitable light source. The photosensitizer is a molecule, which absorbs light at specific wavelengths, and once the light is absorbed, the molecule passes from the ground state to an excited state. From this excited metastable state, the molecule can transfer energy to other molecules, starting the photodynamic reaction, or the molecule returns to the ground state releasing energy as photons, a irradiative process known as fluorescence [1–7], which identifies the presence of the photo sensible molecules. While the photodynamic route takes to the killing of cell, the fluorescence is on mechanism for diagnostic.
The fluorescence properties of most PSs can be used for photodynamic diagnosis. This type of clinical application has been widely reported in literature [8–10]. This is possible because the photosensitizer molecules have higher affinity to cancer cells compared to normal cells. It has been shown widely in preclinical and clinical studies that PS fluorescence can be useful contrast for improved visualization of cancer region and help surgeons delineating tumor margins during surgical resections [11–13]. By using 5-aminolevulinic acid (ALA) or the methyl aminolevulinate (MAL) as precursor of protoporphyrin IX (PpIX), it is possible to use fluorescence to quantify PpIX content at pre- and post-treatment [6, 14–16].
It has been shown that singlet oxygen is generated and PS is being consumed continuously during PDT (as called photobleaching process) and this consumption can be related to PDT outcome [13, 17, 18]. Thus, one can get information about the PDT efficacy by quantifying the PpIX content before and after PDT. The observation of the accumulated PpIX fluorescence is crucial in quantifying photosensitizer content before PDT. On the other hand if fluorescence signal is decreased significantly after PDT, one can infer that a significant amount of photoreaction has occurred and substantial level of singlet oxygen has been generated due to PDT [3, 19, 20]. Thus, if one measures PpIX fluorescence after PDT, the fluorescence level can indicate that the photosensitizer was consumed and there is a good level of photodynamic reaction. On the other hand, if the PpIX fluorescence is high after PDT, it may imply an insufficient level of photoreaction and a lack of singlet oxygen generation. This fluorescence monitoring has practical benefits for optimizing the treatment at the clinical settings.
Based on previous studies [13, 15, 21], the aim of this work is to implement fluorescence imaging in a clinical protocol to improve the clinical outcome. In our multi-center clinical trial of PDT for skin cancer in Brazil, we have added three fluorescence measurements during PDT implementation. First we measured before the application of the photosensitizer PpIX. In this case the fluorescence originated from tissue itself, as called auto fluorescence. Second time point was 3 h after application of the cream, so that fluorescence provided accumulated PpIX content in the lesion. And finally, we measured at the end of the PDT treatment to assess the PpIX photobleaching due to PDT.
The manuscript structure is as follows: In the next section we introduce the materials and methods where we describe the details related to chemicals, patient and instruments. Then we show our results, and provide discussion and conclusion.
Materials and Methods
Chemicals
The pro-drug used was the commercial methyl aminolevulinate (MAL) obtained from PDT-PHARMA (Cravinhos, São Paulo - Brazil) in 20% concentrations in oil and water emulsion (O/W).
Patients
This study is a part of a Brazilian program for the treatment of skin cancer using PDT [22]. The patients were treated at the Amaral Carvalho Hospital (Jaú, SP, Brazil). The fluorescence monitoring of the PS was performed in seven different patients, ranging from 59 to 77 years old, presenting different skin phototypes (I-IV) and lesions in different anatomical sites. The PDT protocol was approved by Ethics Committee of Fundação Hospital Amaral Carvalho (73th Ethical Committee Meeting on October 28, 2011), and the patients signed an informed consent form before being enrolled in the clinical study. The response to the treatment, classified as complete or partial response, was assessed through histopathology 30 days after the second session of PDT and follow-up of after 6 months.
Lesion Identification
The BCC lesions were identified by clinical evaluation and classified as nodular, superficial and pigmented. The hypothesis confirmation of clinical evaluation was performed by histopathological diagnosis, conducted by the pathology service of Amaral Carvalho Hospital, confirming the presence or absence of sBCC. The criterion of exclusion was the use of only superficial lesions.
Lesion Preparation
Lesions were previously cleaned with chlorhexidine solution and curetted for removal of the skin horny layer. This procedure facilitates the transdermal delivery of the MAL cream and optimizes the light coupling in the tissue. The 20% MAL cream was applied on the lesion with a layer about 3 mm thick and 2 mm beyond the clinical lesion edges. Then the lesions were covered with an occlusive curative using plastic film, aluminum foil and bandage to prevent light exposure. The drug-light interval was 3 h.
Fluorescence Images
The fluorescence images were acquired 3 h after 20% MAL cream application before the first and second PDT session and immediately after the PDT procedure in the first and in the second session (after 7 days). A PDT device (Lince, MMOptics, São Carlos, SP- Brazil) was developed presenting a dual platform: a widefield fluorescence imaging system that is based on 405 nm LED (Light Emitting Diode) arrays that allow observing the fluorescence emission over 450 nm and an illumination probe for PDT treatment constituted with 630 nm LED (Light Emitting Diodes) arrays (Figure 1). Having both functions at the same device (optical diagnosis and PDT treatment light illumination) has made the use of fluorescence guided PDT a simple and more clinically feasible procedure.
Lesions Treatment Illumination
After 3 h of cream application, the illumination procedure was performed with an irradiance of 125 mW/cm2 for about 20 min, resulting in a delivered fluence of about 150 J/cm2. A second PDT session was performed after 7 days, following the same protocol. This light dose was adapted based on fluorescence observations.
Image Processing and Data Analysis
Fluorescence images were analyzed with software aimed for numerical calculation (Matlab, Matrix Laboratory, R2014a version). A routine was created to calculate the average of PpIX fluorescence intensity in each image. The routine read the image and the RGB channels were spitted in order to evaluate only the red (R) channel, corresponding to the PpIX fluorescence. The average value of fluorescence intensity was calculated for each image, and then normalized by maximum pixel intensity (255), which is the maximum value that a pixel in the image may have, resulting in values from 0 to 1. More details about the wide-field fluorescence imaging analyses can be found in our previously papers described by Menezes et al. [23].
The widefield fluorescence imaging of PpIX production and photodegradation, and the average of red fluorescence were compared for different situations: concerning the PDT session number, the anatomical site of the lesion and the tissue response to the therapy.
The comparisons were done before and after illumination in the first and in the second session to evaluate the quantitative differences between the images, which gave information about the formation and degradation or consumption of PpIX.
Evaluation and Treatment Progress
The patients were evaluated in a follow-up exam after 30 days of the second session. Clinical and histopathological analysis was done after a punch biopsy according to complete response (cure) or partial response (not cure). A clinical evaluation was performed after 6 months.
Statistical Analysis
We used the Mann-Whitney U-test, a nonparametric test applied for two independent samples, with the groups: PpIX photobleaching of the first and second PDT session; PpIX formation in two anatomical regions.
Results
PDT Session Number
The effect of the one-and two-session PDT was studied in PpIX fluorescence. Figures 2, 3 display the fluorescence intensity of three BCC lesions of three patients undergoing first and second PDT session, respectively. The PpIX formation was higher in the second session (Figures 3A,C,E) than in the first session (Figures 2A,C,E). The corresponding values of PpIX production and photodegradation are presented in Figure 4. The three patients (Figures 2, 3—P1, P2, and P3) showed complete response to PDT confirmed by histopathology 30 days after second PDT session.
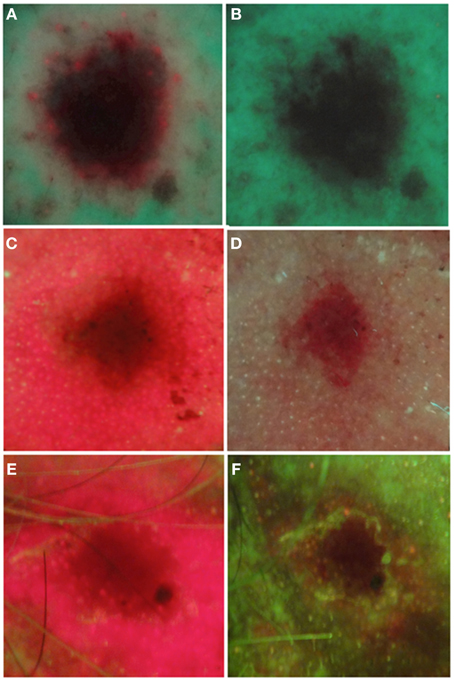
Figure 2. PpIX fluorescence images of BCC lesions after 3 h of MAL topical application (A,C,E) and after the first PDT session (B,D,F).
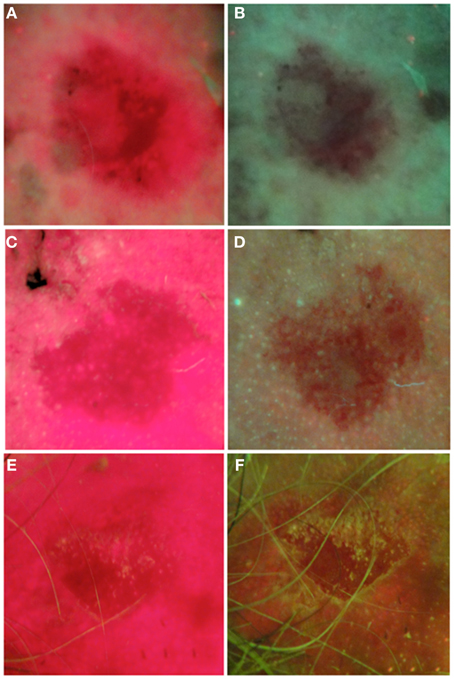
Figure 3. PpIX fluorescence images of BCC lesions before 3 h of MAL topical application (A,C,E) and after the second PDT session (B,D,F).
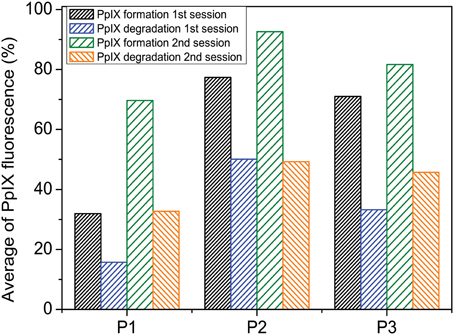
Figure 4. Mean value of red pixels in fluorescence images of different patients (P1, P2, and P3), comparing the formation and degradation in the first and second PDT sessions.
The results show that fluorescence related to PpIX production in the second session was higher than in the first session for all patients, and photodegradation was more pronounced in the first session than in the second session (P < 0.001, two-tailed test).
Lesion Anatomical Site Influence on PpIX Production
The PDT was performed in two lesions of different anatomical sites of the same patient. Figure 5 indicate sit is possible to observe the influence of anatomical body regions in the formation of PpIX during the PDT. The anatomical regions of BCC were near upper lip (Figures 5A,C) and eye regions (Figures 5B,D). Variations between PpIX accumulations in different anatomical regions were observed. The averages of red pixels values are presented in Figure 6. The PpIX fluorescence was higher in the lip region (Figure 6—L1) than in the eyelid region (Figure 6—L2) (P < 0.01, two-tailed test).
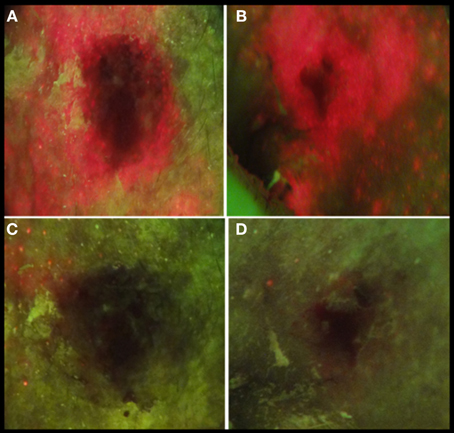
Figure 5. PpIX fluorescence images for comparison of PPIX formation (A,C—before PDT) and degradation (B,D—after PDT) in different lesions of the same patient in second session of PDT.
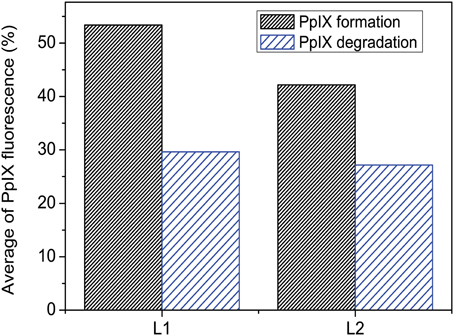
Figure 6. Mean value of red pixels in fluorescence images of different lesions (L1 and L2), comparing the formation and degradation of PPIX in different anatomical sites in the same patient.
Influences of PpIX Intensity on BCC Lesion Response
The negative response to the PDT treatment by BCC lesions showed a direct relation with the PpIX formation. The three BCC lesions that did not present complete response to PDT are shown in Figures 7, 8. The averages of PpIX formation calculated for each lesion of three patients (P1, P2, and P3) is presented in Figure 9. The results were significant (p = 0.0153).
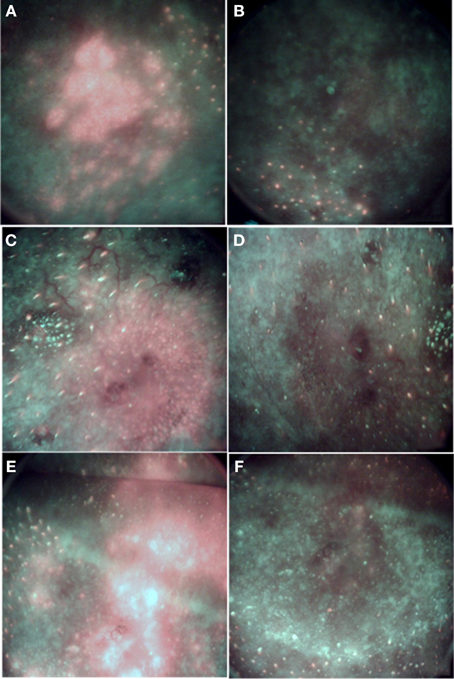
Figure 7. PpIX fluorescence images of BCC lesions after 3 h of MAL topical application (A,C,E) and after the first PDT session (B,D,F).
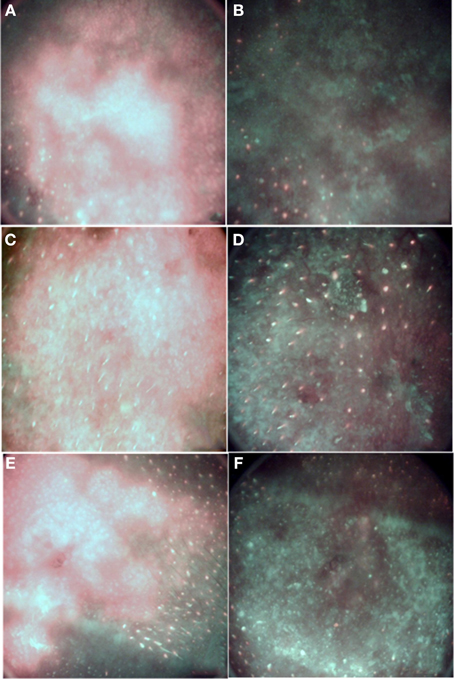
Figure 8. PpIX fluorescence images of BCC lesions before 3 h of MAL topical application (A,C,E) and after the second PDT session (B,D,F).
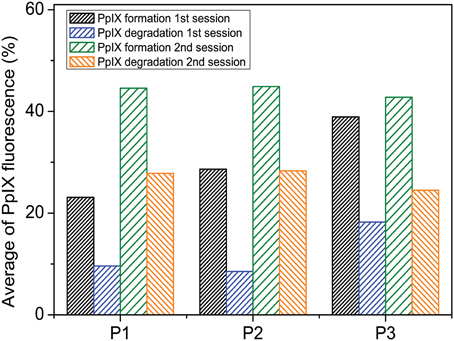
Figure 9. Mean value of red pixels in fluorescence images of different patients (P1, P2, and P3), comparing the formation and degradation in the first and second PDT sessions.
Discussion
Photodynamic diagnosis has been widely used for cancer detection, mainly skin cancer, since photosensitizers can be used as contrast agent for fluorescence imaging in the visible spectral region improving the cancer/normal tissue discrimination [24]. The metabolic activity and the structural and biochemical changes of the skin cancer influences its autofluorescence [17]. Also, the production of PpIX is increased at malignant cells, so it can work as a cancer marker. The photodynamic diagnosis is not only used to detect cancerous tissue but also bacteria, which when synthesizing porphyrin, the biofilm shows an intense red autofluorescence in tooth surfaces [18]. ALA-PpIX derivative is one of the most used markers for this purpose.
In this paper the correlation between the production and photodegradation of PpIX during PDT and its clinical response were assessed. Factors like, PDT session number, lesion site and intrinsic characteristics of the lesion are correlated with PpIX fluorescence. All fluorescence images of PpIX production and photodegradation were performed 3 h after 20% MAL topical administration.
As expected, there was a substantial image contrast between normal and abnormal tissues. The normal tissue shows an intense green autofluorescence, mostly related to collagen under the common blue excitation wavelength (e.g., 405 nm). BCC lesions exhibit decreased autofluorescence due to the chronic inflammation, resulting in a higher absorption by higher hemoglobin content, as well as the decreased collagen emission associated to the breakdown of the crosslinks between its fibers [1]. PpIX ALA derivative accumulates preferentially in abnormal cells. The increase of PpIX formation in the lesions was observed in this study. This can be explained by the increased uptake of MAL by the abnormal tissue. This occurs due to the cell changes in BCC as suggested by Martin et al. [13]. Differences in the PpIX intensity may also be due to cell type from different tissues as observed by Inada et al. [25]. PpIX production did not differ significantly between different lesions of the same tissue of BCC, squamous cell carcinoma (SCC) and psoriasis [26].
The PpIX excited by blue light emits an intense red fluorescence and thus PpIX fluorescence can be used as a contrast for tumor demarcation and PDT therapy response assessment. Therefore, this study demonstrates the effectiveness of this tool in assessing the success of treatment. The total amount of PpIX in tumor and its distribution in tissue determines the type of PDT response rate. On the other hand, PpIX photobleaching is dependent on the illuminations parameters [27]. The enzymatic levels involved in the metabolism of porphyrin expression are altered during PDT [28].
Human and animals studies showed a maximum PpIX production 3 h after ALA or MAL cream application at skin. Since the elimination of PpIX from skin is quite completed within 24 h, performing topical PDT using these ALA and MAL pro drugs is very advantageous compared to hematoporphyrin derivatives that causes prolonged skin photosensitivity for 1–2 months.
The diffuse fluorescence in the areas surrounding to tumor is explained by curettage carried out before application of the cream. Reactive oxygen species formed by PDT cause irreversible cellular damage. Necrotic tissue is removed in second session of PDT, improving the cream and light penetration. However, the PpIX formation in the second session is greater when compared to the first session of PDT. This may be explained by the alteration of metabolism during the inflammatory process. The inflammatory process can change the cellular metabolism during an acute response and the cellular metabolism of porphyrin in the inflammatory process can change the accumulation of PpIX in epithelial tissue. We also note that a lack of improved specificity for photodynamic detection of malignant tissue was also observed at the second session.
The anatomical site influence on PDT efficacy was also monitored by PpIX fluorescence. The treated lesions showed some changes in response to treatment. The red pixel values showed that the photodegradation of PpIX in the eyelid was approximately 37% lower than that in the upper lip region. The eye lesions were in anatomical regions with irregular surfaces, resulting in limitation on the optimal, uniform illumination of the whole lesion. These areas might have received a lower light dose than the desired dose. A real-time and noninvasive way to evaluate this type of sub dose treatment is highly attractive, since it allows the clinician to correct the illumination planning at the same session, after the evaluation of the PpIX photodegradation.
Conclusion
In this study we focused on the use of fluorescence PDT optimization in skin cancer. PDT provides lower risks and better cosmetic results than surgery. However, there are variations in clinical PDT outcome, partially due to limited utilization of quantitative imaging tools. Utilizing PpIX fluorescence can allow PDT optimization and improve its efficacy. In this respect, we implemented the analysis of the wide-field fluorescence of PpIX, relating the PpIX production and photodegradation with the PDT clinical response. Our results indicate that the fluorescence monitoring of PpIX production and photodegradation can be an indicative of the PDT outcome in the treatment of BCC.
Conflict of Interest Statement
The authors declare that the research was conducted in the absence of any commercial or financial relationships that could be construed as a potential conflict of interest.
Acknowledgments
The authors acknowledge the financial support of The Brazilian Development Bank (BNDES, grant n. (09.2.1458.1), the Funding Authority for Studies and Projects (FINEP) and São Paulo Research Foundation (FAPESP/CEPID/CEPOF), National Counsel of Technological and Scientific Development (CNPq) and Coordination of Superior Level Staff Improvement (Capes).
References
1. Celli JP, Spring BQ, Rizvi I, Evans CL, Samkoe KS, Verma S, et al. Imaging and photodynamic therapy: mechanisms, monitoring, and optimization. Chem Rev. (2010) 12:2795–838. doi: 10.1021/cr900300p
PubMed Abstract | Full Text | CrossRef Full Text | Google Scholar
2. Golub AL, Dickson EFG, Kennedy JC, Marcus SL, Park Y, Pottier RH. The Monitoring of ALA-induced protoporphyrin IX accumulation and clearance in patients with skin lesions by in vivo surface-detected fluorescence spectroscopy. Lasers Med Sci. (1999) 14:112–22. doi: 10.1007/s101030050032
PubMed Abstract | Full Text | CrossRef Full Text | Google Scholar
3. Juzeniene A, Peng Q, Moan J. Milestones in the development of photodynamic therapy and fluorescence diagnosis. Photochem Photobiol Sci. (2007) 6:1234–45. doi: 10.1039/b705461k
PubMed Abstract | Full Text | CrossRef Full Text | Google Scholar
4. Orenstein A, Kostenich G, Malik Z. The kinetics of protoporphyrin fluorescence during ALA-PDT in human malignant skin tumors. (1997) 120:229–34.
5. Robinson DJ, de Bruijn HS, van der Veen N, Stringer MR, Brown SB, Star WM. Fluorescence photobleaching of ala-induced protoporphyrin ix during photodynamic therapy of normal hairless mouse skin?: the effect of light dose and lrradiance and the resulting biological effect. (1998) 67:140–9.
6. Enejder AMK, Wang I, Andersson-engels S, Svanberg S, Svanberg K. Kinetic fluorescence studies of 5-aminolaevulinic acid-induced protoporphyrin IX accumulation in basal cell carcinomas. J Photochem Photobiol B Biol. (1999). 49:120–8.
7. Warren CB, Lohser S, Wene LC, Pogue BW, Bailin PL, Maytin E V. Noninvasive fluorescence monitoring of protoporphyrin IX production and clinical outcomes in actinic keratoses following short-contact application of 5-aminolevulinate. J Biomed Opt. (2014) 15:051607. doi: 10.1117/1.3484255
PubMed Abstract | Full Text | CrossRef Full Text | Google Scholar
8. Fritsch C, Backer-Wegerich PM, Menke H, Ruzicka T, Goerz G, Olbrisch RR. Successful surgery of multiple recurrent basal cell carcinomas guided by photodynamic diagnosis. Aesthet Plast Surg. (1997) 21:437–9. doi: 10.1007/s002669900153
PubMed Abstract | Full Text | CrossRef Full Text | Google Scholar
9. Friesen SA, Hjortland GO, Madsen SJ, Hirschberg H, Engebraten O, Nesland JM, et al. 5-Aminolevulinic acid-based photodynamic detection and therapy of brain tumors (review). Int J Oncol. (2002) 21:577–82. doi: 10.3892/ijo.21.3.577
PubMed Abstract | Full Text | CrossRef Full Text | Google Scholar
10. Stummer W, Novotny A, Stepp H, Goetz C, Bise K, Reulen HJ. Fluorescence-guided resection of glioblastoma multiforme by using 5-aminolevulinic acid-induced porphyrins: a prospective study in 52 consecutive patients. J Neurosurg. (2000) 93:1003–13. doi: 10.3171/jns.2000.93.6.1003
PubMed Abstract | Full Text | CrossRef Full Text | Google Scholar
11. Hewett J, McKechnie T, Sibbett W, Ferguson J, Clark C, Padgett M. Fluorescence detection of superficial skin cancers. J Mod Opt. (2000) 47:2021–7. doi: 10.1080/09500340008232454
12. Galletly NP, McGinty J, Dunsby C, Teixeira F, Requejo-Isidro J, Munro I, et al. Fluorescence lifetime imaging distinguishes basal cell carcinoma from surrounding uninvolved skin. Br J Dermatol. (2008) 159:152–61. doi: 10.1111/j.1365-2133.2008.08577.x
PubMed Abstract | Full Text | CrossRef Full Text | Google Scholar
13. Tope WD, Martin A, Grevelink JM, Starr JC, Fewkes JL, Flotte TJ, et al. Lack of selectivity of protoporphyrin IX fluorescence for basal cell carcinoma after topical application of 5-aminolevulinic acid: implications for photodynamic treatment. Arch Dermatol Res. (1995) 287:665–74. doi: 10.1007/BF00371740
PubMed Abstract | Full Text | CrossRef Full Text | Google Scholar
14. Cottrell WJ, Oseroff AR, Foster TH. Portable instrument that integrates irradiation with fluorescence and reflectance spectroscopies during clinical photodynamic therapy of cutaneous disease. Rev Sci Instrum. (2006) 77:064302. doi: 10.1063/1.2204617
15. Sheng C, Jack Hoopes P, Hasan T, Pogue BW. Photobleaching-based dosimetry predicts deposited dose in ALA-PpIX PDT of rodent esophagus. Photochem Photobiol. (2007) 83:738–48. doi: 10.1562/2006-09-07-RA-1033
PubMed Abstract | Full Text | CrossRef Full Text | Google Scholar
16. Robinson DJ, de Bruijn HS, de Wolf W, Sterenborg HJCM, Star WM. Topical 5-aminolevulinic acid-photodynamic therapy of hairless mouse skin using two-fold illumination schemes: PpIX fluorescence kinetics, photobleaching and biological effect. Photochem Photobiol. (2000) 72:794–802.
17. Cholewka A, Stanek A, Kwiatek S, Sieroñ A, Drzazga Z. Does the temperature gradient correlate with the photodynamic diagnosis parameter numerical colour value (NCV)? Photodiagnosis Photodyn Ther. (2013) 10:33–8. doi: 10.1016/j.pdpdt.2012.07.001
PubMed Abstract | Full Text | CrossRef Full Text | Google Scholar
18. Ebihara A, Kawashima N, Saegusa H, Anjo T, Suda H. Autofluorescence of healthy and inflamed pulpal tissues -Photodynamic diagnosis of pulpal tissue-. (2005) 5687:163–9.
19. Liu B, Farrell TJ, Patterson MS. A dynamic model for ALA-PDT of skin: simulation of temporal and spatial distributions of ground-state oxygen, photosensitizer and singlet oxygen. Phys Med Biol. (2010) 55:5913–32. doi: 10.1088/0031-9155/55/19/019
PubMed Abstract | Full Text | CrossRef Full Text | Google Scholar
20. Castano AP, Demidova TN, Hamblin MR. Mechanisms in photodynamic therapy: part one—photosensitizers, photochemistry and cellular localization. Photodiagnosis Photodyn Ther. (2004) 1:279–93. doi: 10.1016/S1572-1000(05)00007-4
21. Wiegell SR, Skiveren J, Philipsen PA, Wulf HC. Pain during photodynamic therapy is associated with protoporphyrin IX fluorescence and fluence rate. Br J Dermatol. (2008) 158:727–33. doi: 10.1111/j.1365-2133.2008.08451.x
PubMed Abstract | Full Text | CrossRef Full Text | Google Scholar
22. Ramirez DP, Kurachi C, Inada NM, Moriyama LT, Salvio AG, Vollet Filho JD, et al. Experience and BCC subtypes as determinants of MAL-PDT response: preliminary results of a national Brazilian project. Photodiagnosis Photodyn Ther. (2014) 11:22–6. doi: 10.1016/j.pdpdt.2013.11.001
PubMed Abstract | Full Text | CrossRef Full Text | Google Scholar
23. Menezes PFC, Requena MB, Bagnato VS. Optimization of photodynamic therapy using negative pressure. Photomed Laser Surg. (2014) 32:1–6. doi: 10.1089/pho.2013.3670
PubMed Abstract | Full Text | CrossRef Full Text | Google Scholar
24. Edell E, Lam S, Pass H, Miller YE, Sutedja T, Kennedy T, et al. Detection and localization of intraepithelial neoplasia and invasive carcinoma using fluorescence-reflectance bronchoscopy: an international, multicenter clinical trial. J Thorac Onco. (2009) 4:49–54. doi: 10.1097/JTO.0b013e3181914506
PubMed Abstract | Full Text | CrossRef Full Text | Google Scholar
25. Inada NM, da Costa MM, Guimarães OCC, da Silva Ribeiro E, Kurachi C, Quintana SM, et al. Photodiagnosis and treatment of condyloma acuminatum using 5-aminolevulinic acid and homemade devices. Photodiagnosis Photodyn Ther. (2012) 9:60–8. doi: 10.1016/j.pdpdt.2011.09.001
PubMed Abstract | Full Text | CrossRef Full Text | Google Scholar
26. Fritsch C, Lehmann P, Stahl W, Schulte KW, Blohm E, Lang K, et al. Optimum porphyrin accumulation in epithelial skin tumours and psoriatic lesions after topical application of delta-aminolaevulinic acid. Br J Cancer (1999) 79:1603–8. doi: 10.1038/sj.bjc.6690255
PubMed Abstract | Full Text | CrossRef Full Text | Google Scholar
27. Smits T, Robles CA, van Erp PEJ, van de Kerkhof PCM, Gerritsen MP. Correlation between macroscopic fluorescence and protoporphyrin IX content in psoriasis and actinic keratosis following application of aminolevulinic acid. J Invest Dermatol. (2005) 125:833–9. doi: 10.1111/j.0022-202X.2005.23843.x
PubMed Abstract | Full Text | CrossRef Full Text | Google Scholar
28. Ishikawa T, Takahashi K, Ikeda N, Kajimoto Y, Hagiya Y, Ogura S-I, et al. Transporter-mediated drug interaction strategy for 5-aminolevulinic acid (ALA)-based photodynamic diagnosis of malignant brain tumor: molecular design of ABCG2 inhibitors. Pharmaceutics (2011) 3:615–35. doi: 10.3390/pharmaceutics3030615
PubMed Abstract | Full Text | CrossRef Full Text | Google Scholar
Keywords: PpIX fluorescence, skin cancer, wide-field imaging, photodynamic therapy, photodiagnosis
Citation: Blanco KC, Moriyama LT, Inada NM, Sálvio AG, Menezes PFC, Leite EJS, Kurachi C and Bagnato VS (2015) Fluorescence guided PDT for optimization of the outcome of skin cancer treatment. Front. Phys. 3:30. doi: 10.3389/fphy.2015.00030
Received: 20 November 2014; Accepted: 14 April 2015;
Published: 30 April 2015.
Edited by:
Ulas Sunar, University at Buffalo, USAReviewed by:
Edite Figueiras, Tampere University of Technology, FinlandAna L. Ribeiro De Souza, Thayer School, USA
Copyright © 2015 Blanco, Moriyama, Inada, Sálvio, Menezes, Leite, Kurachi and Bagnato. This is an open-access article distributed under the terms of the Creative Commons Attribution License (CC BY). The use, distribution or reproduction in other forums is permitted, provided the original author(s) or licensor are credited and that the original publication in this journal is cited, in accordance with accepted academic practice. No use, distribution or reproduction is permitted which does not comply with these terms.
*Correspondence: Kate C. Blanco, Grupo de Óptica, Laboratório de Biofotônica, Instituto de Física de São Carlos, Universidade de São Paulo, Av. Trabalhador São carlense, No 400, São Carlos 13566-590, Brazil,YmxhbmNva2F0ZUBnbWFpbC5jb20=