- State Key Laboratory of Transient Optics and Photonics, Xi'an Institute of Optics and Precision Mechanics, Chinese Academy of Sciences, Xi'an, China
We conduct a power scaling study for the femtosecond dissipative soliton generation with a 10-μm core diameter, fiber-based, compact-sized, and low-cost all-normal-dispersion laser configuration. Laser performance, in terms of spectra distribution, temporal characteristic, average power, and stability in 8 h, for different filtering bandwidths has been, respectively, investigated. In the experiment, the obtainable highest output power and shortest pulse duration are 4.5 W and 85 fs, respectively. To our best knowledge, this is the shortest pulse duration among the reported all-fiber pumped Watt-level mode-locked fiber lasers.
Introduction
Benefiting from the extensive applications in fundamental science and application areas [1, 2], mode-locked laser sources have been one of the most important research subjects [3–5]. High-average-power and high-pulse-energy mode-locked oscillators contribute to increase the signal to noise ratio (SNR) and reduce the non-linear dispersion of amplified pulses. Not only that, but the powerful and energetic seed pulses are also beneficial for the extraction of the laser gain and the improvement of the amplification efficiency. In practice, the compact-sized and low-cost, power-scaled dissipative soliton fiber laser can also be widely used for two-photon polymerization, non-linear microscopy, and as pump source of femtosecond optical parametric amplification. Consequently, these research works on carrying out the generation of high-average-power and high-pulse-energy ultrashort pulse mode-locking fiber laser have important research significance. Up to now, in the reported literature, bulk solid-state crystals, and flexible fibers are frequently used gain media. In particular, the mode-locked fiber lasers, due to excellent thermo-optical effect, diffraction-limited beam quality, and broad emission bandwidth, have been extensively studied in different wavelength regimes (1, 1.5, 2, and 2.8 μm) [6–9]. For conventional single-mode fiber-based mode-locked oscillators, the main restrictions for power scaling and energy improvement are limited pump power and excessive non-linear phase accumulation-induced pulse splitting. Nevertheless, in some potential application fields, such as biomedical and optical coherence tomography, femtosecond laser sources with medium power, and moderate pulse energy can effectively degrade the optical damage and increase the tissue penetration depth. Thus, extremely cumbersome multistage fiber amplifiers with precise dispersion management and non-linearity control are frequently used to scale the power of the conventional mode-locked fiber oscillators to the desired level and simultaneously ensure the compressible clean temporal profile [10, 11].
As we know, pulse shaping mechanism plays a crucial role for the generation of ultrashort pulse mode-locking fiber laser, and thus various types of solitons have been generated over the past decades, such as high-order soliton [12], induced soliton [13], and so on. Therefore, a problem arises as to whether a low-cost and compact-sized mode-locking fiber laser scheme used to directly generate Watt-level ultrashort pulses exists rather than the complicated chirped pulse amplification [10, 11]. Compared with other mode-locking schemes (such as soliton formation, stretched-pulse, and similariton evolution), the all-normal-dispersion mode-locking scheme has attracted much attention due to the chirped pulse spectral filtering-based dissipative soliton-forming mechanism and the corresponding extremely large non-linear phase tolerance capacity, which theoretically supports the generation of power-scaled and energetic mode-locked pulses [14, 15]. Meanwhile, due to the large core diameter and the resultant low non-linearity, large-mode-area (LMA) fibers also have important significance in promoting power scaling and energy increment [16]. Therefore, with further study on the mode-locking technique and the soliton buildup theory, large-mode-area photonic crystal fibers (LMA-PCFs) have been successfully utilized in the all-normal-dispersion mode-locked laser configurations, and as a result, researchers have achieved a more powerful output based on the free-space pumping coupling techniques [17–20].
Due to reduced non-linearity and enhanced power tolerance capacity, the LMA-PCF-based mode-locked oscillators allow for significant increment in average power [21]. However, expensive price, environmentally sensitive pump coupling, and bulky size are the most severe defects of such lasers. In contrast, the off-the-shelf flexible double-clad fibers not only support fusion spliced combiner pumping in only fiber format but also ensure the flexibility and very high cost performance. Therefore, mode-locked oscillators with flexible double-clad fibers as gain media have vast development prospects in improving laser performance, reducing cost, and minimizing complexity. Additionally, this kind of mode-locked oscillator can also meet the highest beam quality demand, especially for the 10-μm core diameter, double-clad fibers, due to their easier single-mode operation than that of the LMA-PCFs, making them very favorable for use in practice.
In view of the inherent performance advantages and potential application values of the power-scaled mode-locked fiber lasers, in this paper we implement the power scaling study with a 10-μm core diameter, fiber-based, reliable, and low-cost laser configuration. With different filtering bandwidths, the obtainable highest average power and shortest pulse duration from the main port are 4.5 W and 85 fs, respectively. Moreover, the recorded power fluctuations are all better than 1.5% root mean square (RMS) in 8 h.
Experimental Setup
The mode-locked fiber laser is schematically shown in Figure 1. It mainly consists of a multimode laser diode (LD), a pump-signal combiner, 1.2 m of Yb-doped flexible double-clad fiber (Liekki: Yb1200-10/125DC), a polarizing beam splitter (PBS), a polarization-sensitive isolator (PS-ISO), a half-wave plate (HWP), and two quarter-wave plates (QWP). The LD has 105 μm core diameter and can emit 29 W of output power. The total fiber length utilized in the experiment is ~3 m and the intra-cavity net dispersion is estimated to be 0.06 ps2. The overall optical cavity length is approximately 4.7 m. In the experiment, the pump power from the 976-nm LD is coupled into the highly doped, flexible, double-clad fiber through the pump-signal combiner with 10-μm core diameter and 125-μm cladding diameter. To reduce the intra-cavity losses as much as possible, a pair of low coupling loss fiber collimators is used. Additionally, the PBS, birefringent plate (BFP), and the following PS-ISO realize the chirped pulse spectral filtering-based dissipative soliton-forming mechanism in the presented laser configuration. The operating principle of the all-normal-dispersion mode-locking can be concisely described as follows: In spectrum domain, considering the spectrum-dependent birefringent effect-induced equivalent spectral filter mechanism, the intra-cavity pulses can be effectively spectra-filtered to the desired bandwidths. However, due to positive group velocity dispersion, the corresponding pulse duration in the temporal domain has also been shortened. After amplification in the Yb-doped fiber in a round trip, the pulses accumulate relatively high peak power and generate new frequency components as a result of the enhanced self-phase modulation. Meanwhile, the pulses broaden in temporal domain again due to the increased normal dispersion. As described above, after a number of round trips along the cavity, highly coherent and chirped dissipative soliton pulses can be generated from the initial noise and can reach the steady oscillated state. In the experiment, the former PBS as the main port provides the high-quality output pulses. Simultaneously in combination with the optimization of the thickness of the BFP, which corresponds to a different filtering bandwidth, the laser parameters from the main port, such as average power, spectrum distribution, pulse duration, and power stability in 8 h, are, respectively, investigated. In contrast, the ejection port of the PS-ISO outputs the poor-quality dissipative soliton, which has also been experimentally studied.
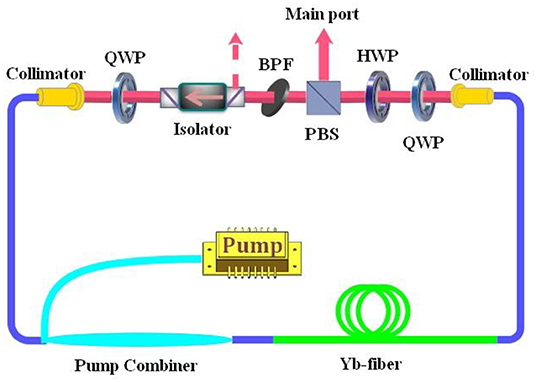
Figure 1. Schematic of the power-scaled dissipative soliton mode-locking fiber laser. QWP, quarter-wave plate; BFP, birefringent plate; PBS, polarizing beam splitter; HWP, half-wave plate; PS-ISO, polarization-sensitive isolator.
Results and Discussion
Firstly, we conduct an experimental study for the generation of power-scaled dissipative soliton based on the BFP with 4.5 mm thickness and an estimated spectral bandwidth of 13 nm [22].
Under the pump power of 8.77 W, we can obtain the stable and self-started mode-locking operation, which corresponds to ~1.3 W of output power at 63.2 MHz repetition rates. For the maximal 29-W pump power, the obtainable mode-locked output power and single-pulse energy at the main port are 4.5 W and 71 nJ, respectively. In the process of pump power increment, the mode-locking state has always remained stable (Figure 2).
Due to chirped pulse spectral filtering-based dissipative mechanism in all-normal-dispersion-based mode-locked fiber laser, the mode-locked spectrum with steep-edge spectral distribution has been generated and shown in the inset of Figure 3B in green solid line. The mode-locked spectrum is ~27 nm wide and centered at 1053 nm. In the scanning range of 150 ps, the measured chirped pulse duration is 7.39 ps, assuming a sech2 pulse shape, which is shown in Figure 3A in red dashed line. After dispersion compensation via 1000 lines/mm transmission grating-pair, dissipative soliton with an average power of up to 3.6 W and pulse duration as short as 92 fs can be experimentally obtained from the compact-sized and low-cost mode-locking fiber laser. The corresponding compression efficiency is 80%.
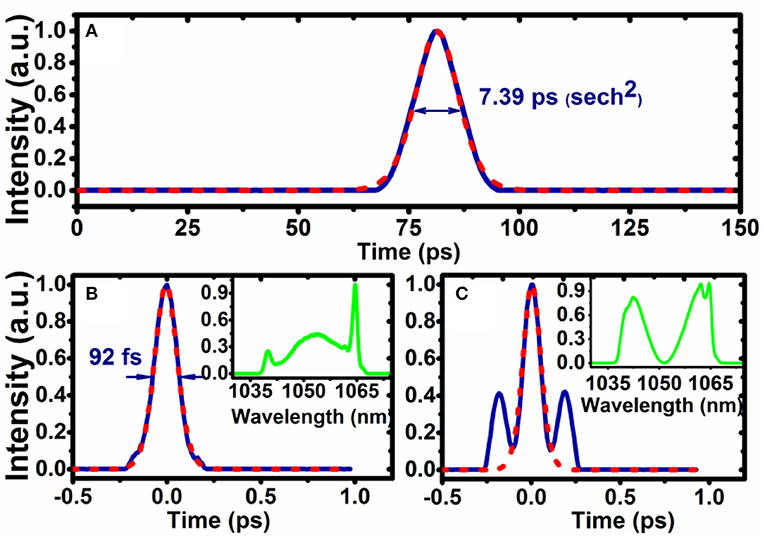
Figure 3. (A) Chirped pulse duration measured directly from the main port of the presented laser configuration. (B) Measured pulse duration after the 1000 lines/mm transmission grating-pair compressor. (C) Measured compressed pulse duration for pulses from the ejection port. The red dashed lines in correspond to the sech2 fitting of pulses. The insets in (B) and (C) correspond to the mode-locked spectra from the main port and the ejection port, respectively.
Beyond that, the laser parameters from the ejection port of the PS-ISO have also been carefully studied in terms of average power, spectrum distribution, and compressed pulse duration. In the experiment, pulses with up to 4.6 W average power and M-like spectral shape can be obtained from the ejection port. However, as shown in Figure 3C, due to the noticeable temporal side lobes around the main pulse, the compressed pulses are not applicable for most of the applications demanding clean pulse quality. Therefore, in this work, we only concentrate on the discussion of the output pulses at the main port.
In the applications of ultrashort pulse fiber lasers, power stability is also one of the most important performance metrics which determines the usability and reliability of fiber lasers to a large extent. Therefore, in order to quantify the operation performance of the compact, fiber-based, power-scaled dissipative soliton mode-locked fiber laser, the power fluctuations in 8 h have also been recorded, with sampling rates of 15 per second, and the RMS fluctuations are <1.5%. Figure 4 shows the measured result. The inset in Figure 4 represents the beam profile of the generated dissipative soliton and implies the excellent single-mode beam quality.
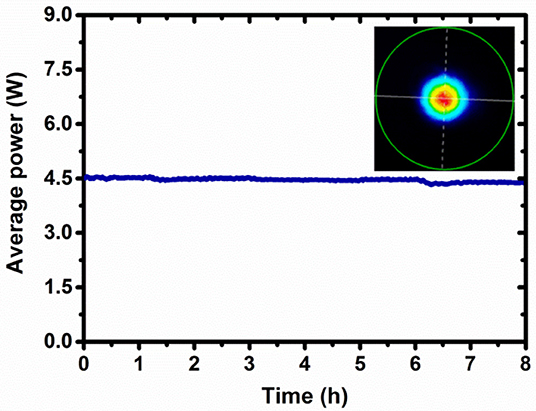
Figure 4. Measured power fluctuations over 8 h under the maximum output power of 4.5 W with sampling rates of 15 per second. The inset represents the beam profile of the generated dissipative soliton and implies the excellent single-mode beam quality.
In addition, BFP with 5.5 mm thicknesses have also been utilized in the power-scaled dissipative soliton mode-locking fiber laser in order to explore the influence of the filtering bandwidth on the laser performance. The BFP correspond to the estimated 11-nm spectral bandwidth [22].
In addition to the spectral bandwidth, the rest of the laser cavity parameters, such as fiber length and maximum pump power, remain constant. Figure 5 shows the variation tendency of the average output power corresponding to the pump power. The blue line represents the pump-signal function of the mode-locking laser cavity with 11 nm spectral shaping bandwidth. The experimentally measured mode-locking threshold is nearly 5.9 W. Under the maximum pump power of 29 W, the obtainable highest output power is 4 W from the main port.
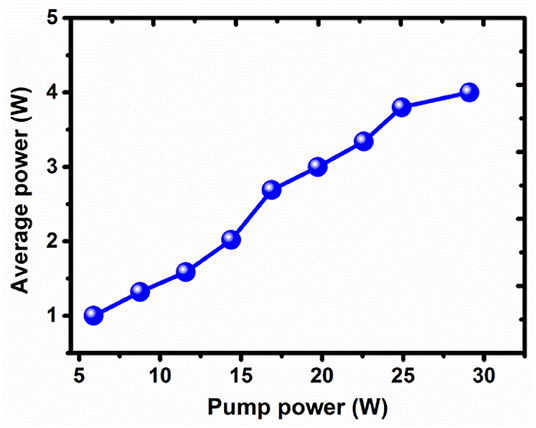
Figure 5. Variation of average power for the mode-locked dissipative soliton fiber laser as a function of pump power.
For the mode-locking laser experiment with 5.5-mm thickness BFP as pulse-shaper, the stable mode-locked spectrum is centered at 1044 nm, with width from 1023.5 to 1064.5 nm. The measured shortest compressed pulse duration is 85 fs, assuming a sech2 pulse shape, which is shown in Figure 6A. The red dashed line in Figure 6A corresponds to the sech2 fitting of the pulse. At the same time, the experimentally recorded power fluctuations in 8 h are better than 1.1% RMS, which are shown in Figure 6B. In comparison with the mode-locked spectrum shown in the inset of Figure 3B, the 5.5-mm thickness BFP-based dissipative soliton laser presents a more pronounced spectral modulation and a much shorter pulse duration as a result of a much wider spectrum distribution. The spectral characteristics are attributed to the narrower spectral filtering and the resultant enhanced self-phase modulation-based broader highly coherent non-linear spectral broadening. In addition, due to the flexible double-clad fibers with 10 μm core diameter providing easier single-mode operation than LMA-PCFs, the presented laser oscillator can thus meet the highest beam quality demand, which has also been measured by the Spiricon M2-200 s and shown in Figure 7. The measured M2 factors are 1.11 and 1.28 in both the horizontal and the perpendicular directions, respectively.
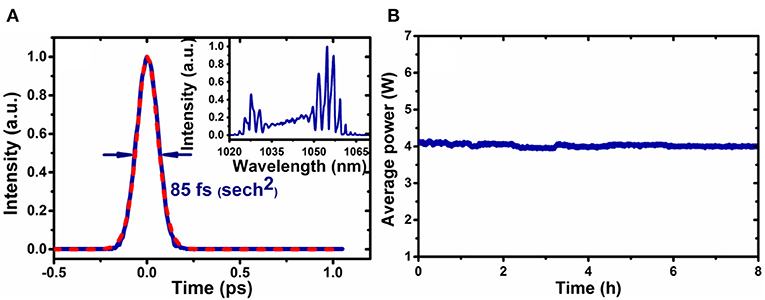
Figure 6. (A) Measured compressed pulse duration and (B) power fluctuations under the maximum output power of 4 W. The red dashed line and the inset in (A) correspond to the sech2 fitting of the pulse and the stable mode-locking spectrum, respectively.
Conclusion
In this paper, power-scaled dissipative soliton generation has been experimentally studied with a compact fiber-based all-normal-dispersion mode-locking laser configuration. Through the optimization of the spectrum-dependent birefringent effect-induced shaping bandwidths, the average power of up to 4.5 W and the pulse duration as short as 85 fs have been experimentally generated, respectively. To our best knowledge, this is the shortest pulse duration ever achieved directly from this kind of compact fiber-based Watt-level mode-locked fiber oscillators. Additionally, it should be noted that advances in materials science also significantly promote the development of the mode-locked fiber lasers, which benefit from the availability of the novel saturable absorbers (SAs) [23–27]. For example, compared with the non-linear polarization rotation technique used here, the single-wall carbon nanotube and graphene can also serve as excellent SAs to realize mode-locking [28–32]. However, in practice, especially for the high-average-power mode-locking lasers, the applications of SAs are prone to thermal damage. With the further development and advancements in materials science, the novel SAs-based high-power mode-locked fiber lasers may be possible.
Data Availability Statement
The datasets generated for this study are available on request to the corresponding author.
Author Contributions
All authors listed have made a substantial, direct and intellectual contribution to the work, and approved it for publication.
Funding
This work was supported by the National Natural Science Foundation of China (Grant Nos. 61805274 and 61690222), the Shaanxi Key R&D Program (Grant No. 2019GY-095), the National Key R&D Program of China (Grant No. 2018YFB1108000), and the Guangdong Key R&D Program (Grant No. 2018B090904003).
Conflict of Interest
The authors declare that the research was conducted in the absence of any commercial or financial relationships that could be construed as a potential conflict of interest.
References
1. Udem T, Reichert J, Holzwarth R, Hänsch TW. Absolute optical frequency measurement of the cesium D1 line with a mode-locked laser. Phys Rev Lett. (2016) 82:3568–71. doi: 10.1103/PhysRevLett.82.3568
2. Zong W, Wu R, Li M, Hu Y, Li Y, Li J, et al. Fast high-resolution miniature two-photon microscopy for brain imaging in freely behaving mice. Nat Methods. (2017) 14:713–9. doi: 10.1038/nmeth.4305
3. Tian W, Peng Y, Zhang Z, Yu Z, Zhu J, Xu X, et al. Diode-pumped power scalable Kerr-lens mode-locked Yb:CYA laser. Photon Res. (2018) 6:127–31. doi: 10.1364/PRJ.6.000127
4. Ilday FÖ, Kesim DK, Hoffmann M, Saraceno CJ. Discrete similariton and dissipative soliton mode locking for energy scaling ultrafast thin-disk laser oscillators. IEEE J Sel Top Quan Electron. (2018) 24:1102712. doi: 10.1109/JSTQE.2018.2832651
5. Tian W, Yu C, Zhu J, Zhang D, Wei Z, Xu X, et al. Diode-pumped high-power sub-100 fs kerr-lens mode-locked Yb:CaYAlO4 laser with 1.85 MW peak power. Opt Express. (2019) 27:21448–54. doi: 10.1364/ASSL.2019.JTh3A.21
6. Lv Z, Yang Z, Song D, Li F, Yang Y, Yang X, et al. Enhanced self-phase modulation effect: an effective method of generating high average and peak power femtosecond laser pulses. Laser Phys Lett. (2019) 16:035110. doi: 10.1088/1612-202X/aaff49
7. Zhang H, Jin L, Xu Y, Zhang H, Shi L, Wang T, et al. C-band wavelength tunable mode-locking fiber laser based on CD-SMS structure. Appl Optics. (2019) 58:5788–93. doi: 10.1364/AO.58.005788
8. Tokurakawa M, Sagara H, Tünnermann H. All-normal-dispersion nonlinear polarization rotation mode-locked Tm:ZBLAN fiber laser. Opt Express. (2019) 27:19530–5. doi: 10.1364/OE.27.019530
9. Duval S, Gauthier J-C, Robichaud L-R, Paradis P, Olivier M, Fortin V, et al. Watt-level fiber-based femtosecond laser source tunable from 2.8 to 3.6 μm, Opt Lett. (2016) 41:5294–7. doi: 10.1364/OL.41.005294
10. Abdelalim MA, Kotb HE, Anis H, Tchouragoulov S. Power-scaled dissipative soliton using double-cladding-pumped Yb-doped all-fiber amplifier. Photon Res. (2016) 4:277–80. doi: 10.1364/PRJ.4.000277
11. Liu W, Chia S-H, Chung H-Y, Greinert R, Kärtner FX, Chang G. Energetic ultrafast fiber laser sources tunable in 1030-1215 nm for deep tissue multi-photon microscopy. Opt Express. (2017) 25:6822–31. doi: 10.1364/OE.25.006822
12. Tang DY, Zhang H, Zhao LM, Wu X. Observation of high-order polarization-locked vector solitons in a fiber laser. Phys Rev Lett. (2008) 101:153904. doi: 10.1103/PhysRevLett.101.153904
13. Zhang H, Tang DY, Zhao LM, Tam HY. Induced solitons formed by cross-polarization coupling in a birefringent cavity fiber laser. Opt Lett. (2008) 33:2317–9. doi: 10.1364/OL.33.002317
14. Chong A, Renninger WH, Wise FW. All-normal-dispersion femtosecond fiber laser with pulse energy above 20 nJ. Opt Lett. (2007) 32:2408–10. doi: 10.1364/OL.32.002408
15. Chong A, Renninger WH, Wise FW. Properties of normal-dispersion femtosecond fiber lasers. J Opt Soc Am B. (2008) 25:140–8. doi: 10.1364/JOSAB.25.000140
16. Röser F, Rothhard J, Ortac B, Liem A, Schmidt O, Schreiber T, et al. 131 W 220 fs fiber laser system. Opt Lett. (2005) 30:2754–6. doi: 10.1364/OL.30.002754
17. Lecaplain C, Chédot C, Hideur A, Ortaç B, Limpert J. High-power all-normal-dispersion femtosecond pulse generation from a Yb-doped large-mode-area microstructure fiber laser. Opt Lett. (2007) 32:2738–40. doi: 10.1364/OL.32.002738
18. Lecaplain C, Ortaç B, Machinet G, Boullet J, Baumgartl M, Schreiber T, et al. High-energy femtosecond photonic crystal fiber laser. Opt Lett. (2010) 35:3156–8. doi: 10.1364/OL.35.003156
19. Lefrançois S, Kieu K, Deng Y, Kafka JD, Wise FW. Scaling of dissipative soliton fiber lasers to megawatt peak powers by use of large-area photonic crystal fiber. Opt Lett. (2010) 35:1569–71. doi: 10.1364/OL.35.001569
20. Baumgartl M, Jansen F, Stutzki F, Jauregui C, Ortaç B, Limpert J, et al. High average and peak power femtosecond large-pitch photonic-crystal-fiber laser. Opt Lett. (2011) 36:244–6. doi: 10.1364/OL.36.000244
21. Baumgartl M, Lecaplain C, Hideur A, Limpert J, Tünnermann A. 66 W average power from a microjoule-class sub-100 fs fiber oscillator. Opt. Lett. (2012) 37:1640–2. doi: 10.1364/OL.37.001640
23. Ma J, Lu S, Guo Z, Xu X, Zhang H, Tang D, et al. Few-layer black phosphorus based saturable absorber mirror for pulsed solid-state lasers. Opt Express. (2015) 23:22643–8. doi: 10.1364/OE.23.022643
24. Wang ZT, Chen Y, Zhao CJ, Zhang H, Wen SC. Switchable dual-wavelength synchronously Q-switched erbium-doped fiber laser based on graphene saturable absorber. IEEE Photon J. (2012) 4:869–76. doi: 10.1109/JPHOT.2012.2199102
25. Guo B, Xiao Q, Wang S, Zhang H. 2D layered materials: synthesis, nonlinear optical properties, and device applications. Laser Photon Rev. (2019) 12:1700221. doi: 10.1002/lpor.201800327
26. Cui Y, Liu X. Graphene and nanotube mode-locked fiber laser emitting dissipative and conventional solitons. Opt Express. (2013) 21:18969–74. doi: 10.1364/OE.21.018969
27. Cui Y, Lu F, Liu X. Nonlinear saturable and polarization-induced absorption of rhenium disulfide. Sci Rep. (2017) 7:40080. doi: 10.1038/srep40080
28. Liu X, Popa D, Akhmediev N. Revealing the transition dynamics from Q switching to mode locking in a soliton laser. Phys Rev Lett. (2019) 123:093901. doi: 10.1103/PhysRevLett.123.093901
29. Liu X, Pang M. Revealing the buildup dynamics of harmonic mode-locking states in ultrafast lasers. Laser Photon Rev. (2019) 13:1800333. doi: 10.1002/lpor.201800333
30. Liu X, Yao X, Cui Y. Real-time observation of the buildup of soliton molecules. Phys Rev Lett. (2018) 121:023905. doi: 10.1103/PhysRevLett.121.023905
31. Liu X, Cui Y. Revealing the behavior of soliton buildup in a mode-locked laser. Adv Photon. (2019) 1:016003. doi: 10.1117/1.AP.1.1.016003
Keywords: power-scaling, mode-locking, dissipative soliton, pulse-shaping, laser performance
Citation: Lv Z, Yang Z, Li F, Li Q, Yang X, Wang Y and Zhao W (2020) Experimental Investigation of Power-Scaled Dissipative Soliton Generation. Front. Phys. 8:48. doi: 10.3389/fphy.2020.00048
Received: 24 October 2019; Accepted: 20 February 2020;
Published: 20 March 2020.
Edited by:
Meng Pang, Max Planck Institute for the Science of Light, GermanyReviewed by:
Wenbin He, Max Planck Institute for the Science of Light, GermanyHan Zhang, Shenzhen University, China
Yudong Cui, Zhejiang University, China
Chengbo Mou, Shanghai University, China
Copyright © 2020 Lv, Yang, Li, Li, Yang, Wang and Zhao. This is an open-access article distributed under the terms of the Creative Commons Attribution License (CC BY). The use, distribution or reproduction in other forums is permitted, provided the original author(s) and the copyright owner(s) are credited and that the original publication in this journal is cited, in accordance with accepted academic practice. No use, distribution or reproduction is permitted which does not comply with these terms.
*Correspondence: Zhiguo Lv, bHZ6aGlndW9Ab3B0LmFjLmNu