- 1The European Organization for Nuclear Research (CERN), Meyrin, Switzerland
- 2Fakultät 1, Rheinisch-Westfälische Technische Hochschule Aachen, Aachen, Germany
- 3Dipartimento di Fisica “Giuseppe Occhialini”, Universitá degli studi di Milano Bicocca, Milano, Italy
Time resolution of scintillation-based detectors is becoming continuously more important, both for medical applications, especially in positron emission tomography (PET), and in high energy physics. This article is an initial study on exploiting the fast cross-luminescence emission in the inorganic
1. Introduction
Scintillating crystals are widely used for the detection of ionizing particles and gamma radiation in various applications, e.g., in high energy physics and medical imaging, where high time resolution plays an ever more significant role. In medical applications, especially in positron emission tomography (PET), the precise time-of-flight (TOF) information would lead to higher signal-to-noise ratio imaging, thus allowing to reduce the administered radioactive dose to the patient and/or the scanning time. High-luminosity particle physics experiments would benefit from high resolution timing in order to cope with serious pile-up effects, especially important in the search for rare events and new physics.
For this purpose, there is a large effort in many groups focusing on inorganic scintillators for the improvement of time resolution [1–10],1,2 for instance by exploiting intrinsically fast scintillation processes with short rise- and decay times. Due to its fast cross-luminescence emission,
Since the beginning of the 1970s, it is known that
More than 10 years later, a second emission band at 220 nm was discovered [12]. It has been established that, on top of the self-trapped exciton emission at 320 nm,
Cross-luminescence emission is a scintillation process where an electron is excited from the core band to the conduction band. The resulting hole in the core band then recombines with an electron in the valence band. This process is illustrated in Figure 1. Since the valence band contrary to the conduction band, is filled with electrons with which the hole in the core-band can recombine, the recombination probability is large. As such, cross-luminescence is an intrinsically fast scintillation process resulting in a very short light pulse with a typical decay time of the order of nanoseconds or less.
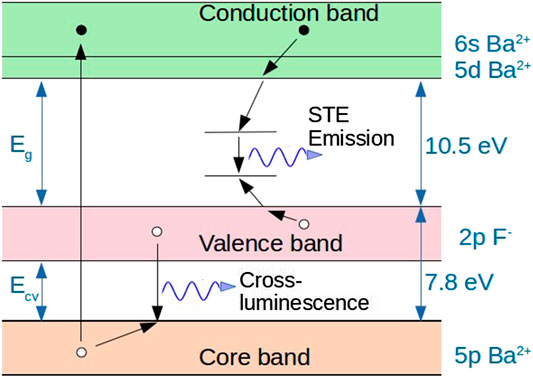
FIGURE 1. Schematic overview of the band-gap diagram of
Cross-luminescence is also referred to as core-valence luminescence [16, 17] and Auger-free luminescence [18]. Cross-luminescence is only exhibited by crystals that have a band gap between the valence band and the top of the core band with an energy difference
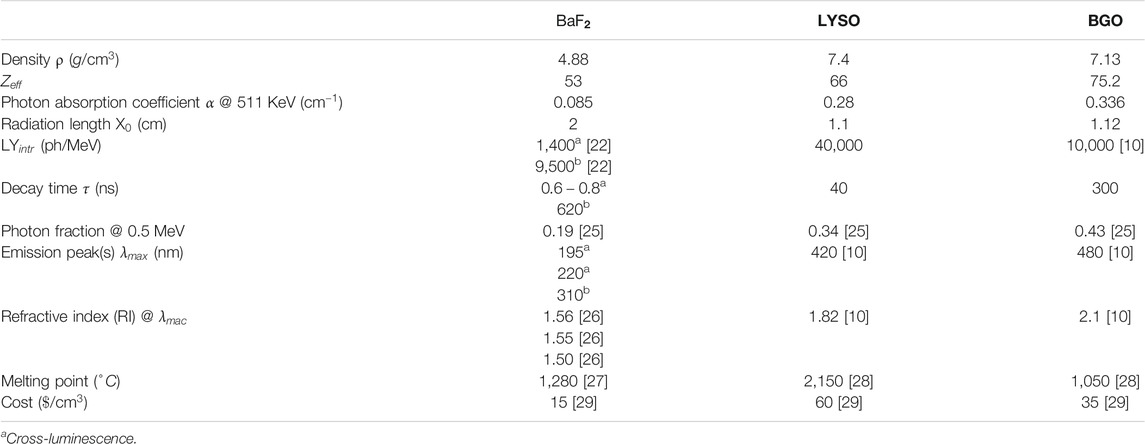
TABLE 1. Overview of the general characteristics of
Owing to its favorable timing characteristics, as also shown in Table 1,
Comparing the properties of
This promising potential in CTR performance, considering also the relatively low production cost of
This paper summarizes initial investigations on the timing potential of cross-luminescence in
Materials and Methods
Crystal Samples
In the study presented in this article,
Optical Coupling Greases
To optimize light extraction from the crystal, inorganic scintillators are generally coupled to a photodetector using an optical coupling agent. The difficulty here is that there are not many materials on the market that are transparent to UV-light with wavelengths below 250–300 nm. Previously Klamra et al. [31] and Zhu [34] have reported their research on this subject. This article presents a study on the suitability of some selected greases as optical coupling agents for BaF2 and also for measurements in conjunction with the specific VUV SiPMs.
Rhodorsil 47V was one of the first greases tested in conjunction with
Silicon Photomultipliers
Common SiPMs on the market are not compatible with light emitted in the deep UV. There are several reasons for this [35]. One apparent reason is that, in order to protect the wire bonds of the SiPM and the sensor itself, a protection layer is applied on top of the SiPM [35], usually made of glass or epoxy. Both are not transparent to deep UV light. Underneath the protection layer is usually an anti-reflective layer, which is not designed for UV light either.
Furthermore, deep UV light with a wavelength of around 200 nm only penetrates a few nanometers into the silicon layer [35]. This is about 100 times shallower than for light above 400 nm. This has the effect that the electron-hole pairs are effectively created at the surface of the SiPM, making it difficult to retain high photon detection efficiencies (PDEs).
Therefore, in this work a SiPM specifically developed for measurements in the vacuum UV (VUV) was used, i.e., the Hamamatsu S13370-3050CN5 primarily developed for dark matter searches with liquid xenon. This SiPM then does not possess a layer to protect the wire bonds, nor to protect its surface, making it fragile and prone to damage when applying greases on its surface.
According to its datasheet, this SiPM has a photon detection efficiency (PDE) of about 24%5 at 175 nm and is constant up to at least 200 nm. An independent measurement, however, indicates a PDE of only
Characterization Methods
Transmission
Transmission measurements were performed with a Perkin Elmer LAMBDA 650 UV/VIS Spectrometer.6 This device can measure transmission of samples using light with wavelengths between 190 and 900 nm. To measure the transmission of the viscous optical coupling agents, the grease under test was applied as a thin layer between two quartz plates, which had been tested beforehand to be transparent down to 190 nm. This is used to correct the later transmission measurements of the greases for the effect of Fresnel reflection.
Light Output
The light output is measured by exciting the scintillating crystal with a
where
Time Correlated Single Photon Counting
The time correlated single photon counting (TCSPC) measurements were performed with the setup illustrated in Figure 2. A Hamamatsu N5084 pulsed X-ray tube coupled to a picosecond laser with a FWHM of ∼50 ps excites the crystal under test. At the same time the laser driver produces a start signal for the fast time-to-digital converter (Cronologic xTDC4). The light produced by the X-rays traversing the crystal is measured by a hybrid PMT HPM-100-07 from Becker & Hickl and provides the stop to the TDC. Every time a pulse is generated in the X-ray tube, a time measurement is started and the time delays of the detected single photon signals in the hybrid PMT recorded. In this way the scintillation pulse produced by the scintillator is basically sampled one photon at a time. A histogram of the delay times is produced, showing the full kinetics of the scintillation light. The impulse response function (IRF) of the entire setup was measured to be 162 ps FWHM and was taken into account in the data analysis (fit of the decay and rise times). The quantum efficiency of the hybrid PMT is only reported for values
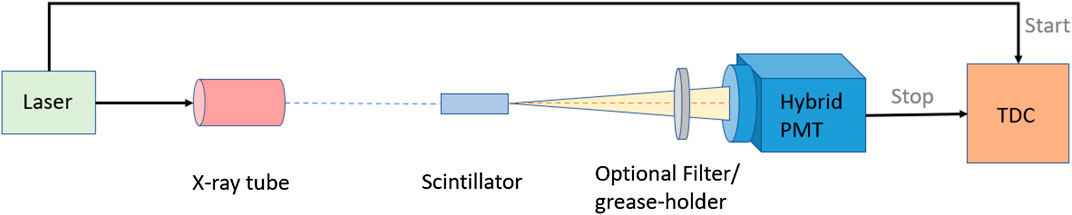
FIGURE 2. Illustration of the time correlated single photon counting (TCSPC) setup, using an X-ray tube excited by a picosecond laser, hybrid-PMT, and a fast TDC. Filters can be placed in front of the hybrid-PMT.
To measure the grease-induced absorption for the different decay components, two plates of quartz with a thin layer of optical grease applied between them are placed between the
Coincidence Time Resolution
The coincidence time resolution (CTR) is measured with the setup illustrated in Figure 3. In the setup two scintillators are facing each other in a back-to-back arrangement and are excited by two correlated and co-linear gammas of 511 keV originating from a
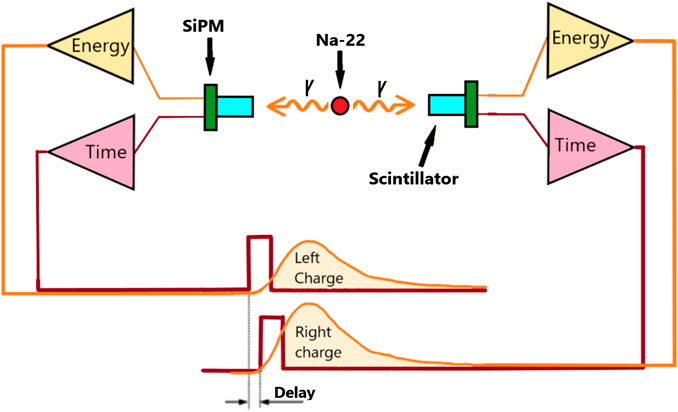
FIGURE 3. Illustration of the coincidence time resolution (CTR) setup, based on ref. [8].
As mentioned before, the
Results
Transmission Measurements
To determine the transparency of the
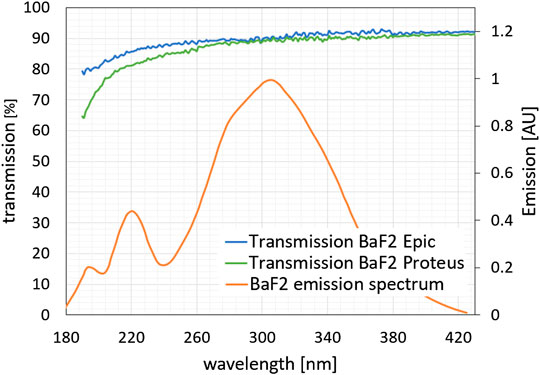
FIGURE 4. Transmission measurements with
Figure 5 shows the transmission of the measured greases. They show that Meltmount is clearly not suitable as a glue for
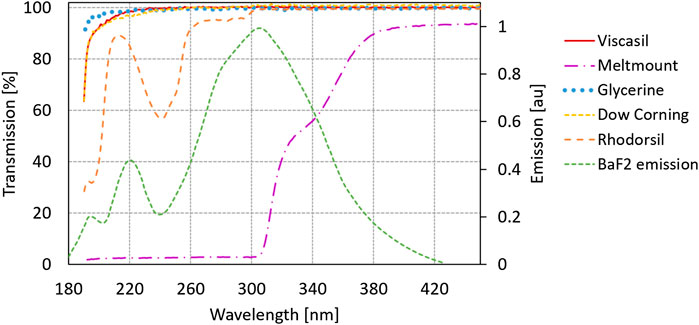
FIGURE 5. Transmission measurements of several optical couplings agents between two quartz plates, performed between 190 and 440 nm, corrected for the absorption by one quartz plate and the Fresnel reflection between the quartz/air interfaces. Also the emission spectrum of
As to Dow Corning and Viscasil, the differences between the two of them are insignificant. Both are close to 100% transparent down to 220 nm, from where on they both start to absorb gradually. They both seem to have a cut-off at ∼190 nm, also observed by Zhu [23] and Dorenbos et al. [22]. All together, Dow Corning 200 and Viscasil look suitable as optical coupling compounds for
In contrast to Dow Corning and Viscasil, glycerine extends its transparency even further down to shorter wavelength before it also rolls off.
Light Output Measurements
Table 2 gives an overview of the light output measurements with the greases that had been investigated, as well as the corresponding gain in light extraction compared to measurements with air coupling.
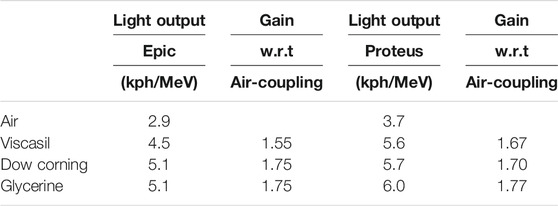
TABLE 2. Light output measurements on
The light output from the Proteus crystal is consistently higher than that from the Epic crystal, on average by about a factor 1.15.
Table 2 further shows that all greases clearly improve light extraction from the crystals. Comparing the results from the three grease measurements with those obtained from air-coupling measurements, a gain in extracted light of 1.6–1.8 is observed for both crystals.
Time Correlated Single Photon Counting Measurements
In a first round, the kinetics of the
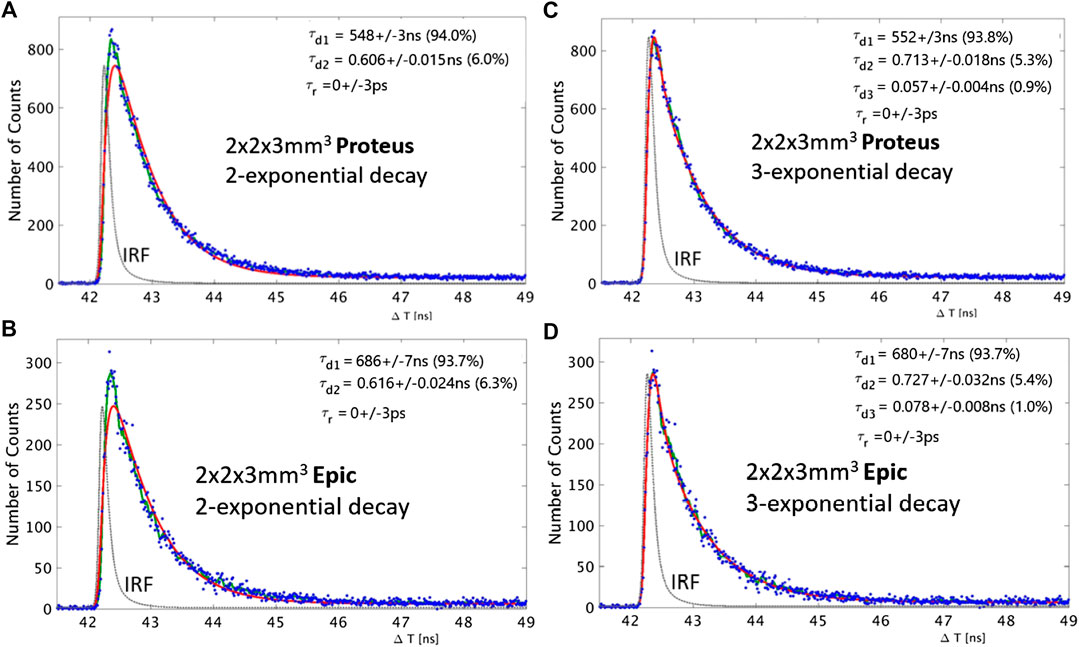
FIGURE 6. Time correlated single photon counting measurements under X-ray excitation, with 3 mm long
Since two decay channels were expected from literature, one for cross-luminescence and one for the STE emission, the data was initially fitted with a double exponential decay function. The results of these fits are shown in the left graphs of Figure 6. Notwithstanding its low
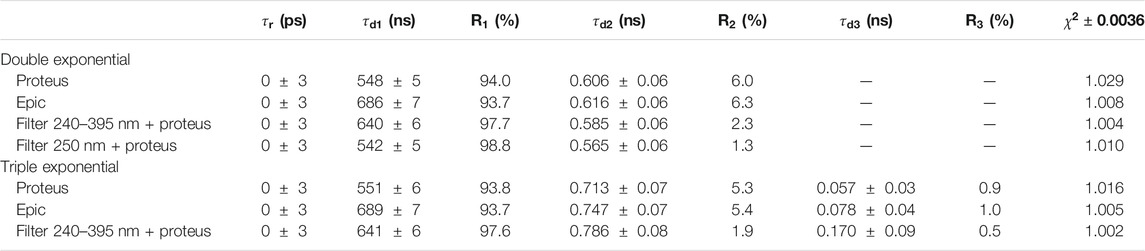
TABLE 3. Parameters of the double and triple exponential used, convolved with the IRF, to fit the data from the TCSPC measurements with a
As to the origin of the very fast decay component, some groups have claimed the existence of hot intraband-luminescence in
The measurements in Figure 6 furthermore show that both crystals have a decay time of 0.7 ns for the component identified as the cross-luminescence emission, with an abundance of ∼5%. The decay times of ∼700 ns and ∼600 ns for Epic and Proteus, respectively, both with an abundance of 94%, were identified as the STE emission. These values are comparable with literature values. The abundance of cross-luminescence emission is lower than expected from literature, which can be attributed to the lower detection efficiency in the deep UV of the hybrid-PMT used in this work. There is no difference in the observed abundance of cross-luminescence between the two crystals.
To investigate the effect of the chosen greases on the abundances of the different decay components, it was preferred to use the double exponential decay function for the fitting of the data, thus determining an effective decay time and abundance for the two fast components together. A fit with three decay components would have resulted in a sharing of the statistics of the emission over two more fitting parameters, thus reducing the statistics per parameter such that too many statistical fluctuations are introduced. These fluctuations will affect the fitted values for the abundances and decay times of both the very fast component and the cross-luminescence.
Table 4 shows the results of the measurements with the selected optical coupling greases, fitted with a double exponential decay function. The fits indicate that the fast emission abundance is slightly reduced (0.94–0.92) by the presence of the three coupling compounds, yet this reduction is not significant when compared with the air measurement. This is consistent with the transmission measurements of the greases, which show very little absorption in this wavelength region.
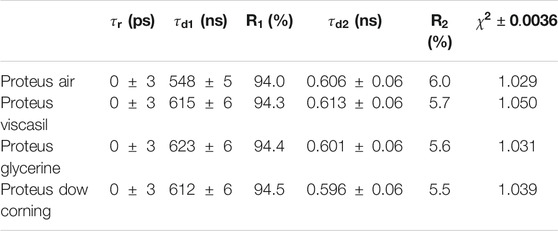
TABLE 4. Parameters of the double exponential fit, convolved with the IRF, used to describe the data from the TCSPC measurements with a
CTR Measurements
Measurements With Air Coupling
Figure 7 shows the CTR measurements with Proteus and Epic crystals. The obtained CTR values are 98
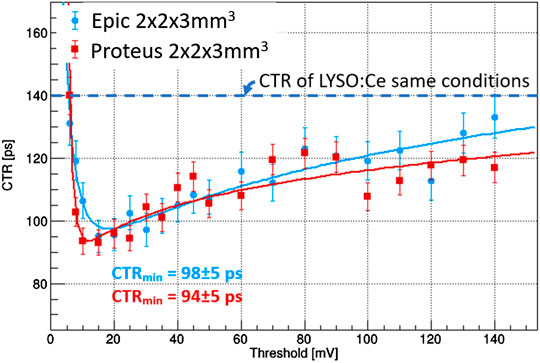
FIGURE 7. CTR measurements of
Measurements With Optical Coupling
The results of the CTR measurements with the selected greases are summarized in Table 5. The SiPM bias used for comparing the different greases in terms of their CTR was 60 V. These results are also visualized in Figure 8. The operating voltage was then further increased to 61 V to assess the limits of the SiPM for highest CTR performance.
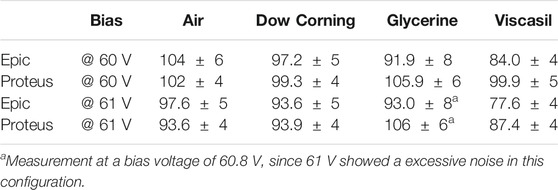
TABLE 5. CTR measurements of
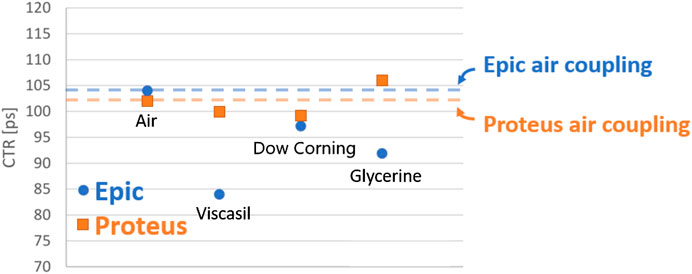
FIGURE 8. Minima of CTR measurements (equals highest resolution) of
Figure 8 shows that, within the statistical limits, the CTR of the Proteus crystal shows no improvement no matter what grease is chosen. Only when pushing the bias voltage to 61 V, Viscasil together with the Proteus crystal shows a slight improvement in CTR, as can been seen in Table 5.
Unlike the Proteus crystal, whose CTR seems to be largely insensitive to any of the applied coupling agents, the Epic’s CTR visibly benefits from these greases, with Viscasil being the best of all, allowing even a CTR of as high as
Discussion
In this work, several characterizations have been performed to analyze and better understand the time resolution of
Transmission measurements show that, compared to Epic, crystals from Proteus absorb more light below ∼270 nm, meaning that predominantly cross-luminescence light is being absorbed. This will have a negative effect on the CTR. Furthermore, as to the three coupling compounds Viscasil, Dow Corning and glycerine, they have been shown to be almost completely transparent down to 220 nm, before they begin to slightly absorb light below that. Among the three, glycerine in fact appeared as the most transparent as it transmits better below 220 nm than the others.
Light output measurements show Proteus having a consistently higher light output than Epic, on average by a factor of 1.15. This is to be seen in contrast to the transmission measurements, where the Proteus crystal was found to absorb more light occurring only in the wavelength range of the fast emission. The light output measurements, on the other hand, are dominated by the more abundant slow STE emission in the crystal, since its light yield is about 7–8 times higher than that from the fast cross-luminescence [22]. This leads to the conclusion that for Proteus at least the amount of extracted STE emission is higher than for Epic. However, considering the higher absorption of Proteus below 270 nm, this does not necessarily mean that the amount of extracted fast cross-luminescence light from Proteus is also a factor 1.15 higher than for Epic.
The outcome of TCSPC measurements shows that the emission kinetics of the Epic and Proteus crystals are the same, so are the abundances of the cross-luminescence emission. This was a priori not expected given the transmission measurements on the one hand, which showed a higher absorption in the cross-luminescence region for Proteus, and the light output measurements on the other hand, showing a higher total light extraction for Proteus. This offset is probably due to the limited quantum efficiency at short wavelengths of the used HPK-100-07 hybrid PMT, making it less sensitive in the region where the Proteus crystal shows a higher absorption than Epic.
Furthermore, a very fast decay component, with a decay-time of ∼0.1 ns has been observed, with an estimated abundance of 1%. This very fast emission was newly discovered by our group [10], its origin still being under investigation. Measurements with filters have demonstrated that the majority of its emission lies below 240 nm. Considering the limitations of the HPM-100-07 hybrid PMT in the setup, the abundance of this emission is most probably underestimated. From the TCSPC measurements one can derive that all the tested greases slightly, by 6–8%, absorb light in the region of the cross-luminescence emission.
As to coincidence time resolution, of prime interest in this study, both Epic and Proteus crystals produced comparable results of 94
However, despite the beneficial effects of the optical coupling greases on the global detection performance, their physical contact with the unprotected SiPM surface can lead to unexpected effects such as those observed here in the case of glycerine. Although being chemically inert and chosen for that purpose, glycerine systematically led to an increase in the SiPM’s dark current, with its obvious negative effects on the CTR.
The scintillators used for the investigation in this article are short 3 mm long pixels. As the main purpose of this paper was to assess the timing potential of cross-luminescence in
In summary, this work has shown that, already with existing technologies,
If the photon detection efficiency of SiPMs working in the deep UV could be improved from the current 15% to values of 60% not an untypical value for SiPMs at larger wavelengths [10], this would have an immediate impact on the coincidence time resolution, ultimately enabling to reach a CTR of
Data Availability Statement
All datasets presented in this study are included in the article.
Author Contributions
RP, EA, and SG developed as a team the idea of using VUV SiPMs for cross-luminescence readout for ultrafast timing. SG designed the readout for the coincidence time resolution measurements. RP wrote the manuscript, perfomed measurements on the transmission, light yield, coincidence time resolution, and corresponding data analysis. SG and RP performed TCSPC measurements and corresponding data analysis. SG and EA reviewed the manuscript.
Funding
This work was performed in the framework of the Crystal Clear Collaboration. It was sponsored by the German Federal Ministry of Education and Research in the framework of the Wolfgang Gentner Program (grant no. 05E15CHA).
Conflict of Interest
The authors declare that the research was conducted in the absence of any commercial or financial relationships that could be construed as a potential conflict of interest.
Acknowledgments
We would like to thank all the members of the Crystal Clear team at The European Organization for Nuclear Research (CERN) for their support.
References
1. Huizenga, J, Seifert, S, Schreuder, F, van Dam, H, Dendooven, P, Löhner, H, et al. A fast preamplifier concept for sipm-based time-of-flight pet detectors. Nucl Instrum Methods Phys Res Sect A Accel Spectrom Detect Assoc Equip (2012). 695:379–84. doi:10.1016/j.nima.2011.11.012
2. Gundacker, S, Auffray, E, Frisch, B, Jarron, P, Knapitsch, A, Meyer, T, et al. Time of flight positron emission tomography towards 100ps resolution with L(y)SO: an experimental and theoretical analysis. J Inst (2013). 8:P07014. doi:10.1088/1748-0221/8/07/p07014
3. Derenzo, SE, Choong, WS, and Moses, WW. Fundamental limits of scintillation detector timing precision. Phys Med Biol (2014). 59:3261–86. doi:10.1088/0031-9155/59/13/3261.
4. Brunner, SE, Gruber, L, Marton, J, Suzuki, K, and Hirtl, A. Studies on the cherenkov effect for improved time resolution of tof-pet. IEEE Trans Nucl Sci (2014). 61:443–7. doi:10.1109/tns.2013.2281667
5. Omelkov, S, Nagirnyi, V, Vasil׳ev, A, and Kirm, M. New features of hot intraband luminescence for fast timing. J Lumin (2016). 176:309–17. doi:10.1016/j.jlumin.2016.03.039
6. Lecoq, P. Pushing the limits in time-of-flight pet imaging. IEEE Trans Radiat Plasma Med Sci (2017). 1:473–85. doi:10.1109/trpms.2017.2756674
7. Cates, JW, Gundacker, S, Auffray, E, Lecoq, P, and Levin, CS. Improved single photon time resolution for analog SiPMs with front end readout that reduces influence of electronic noise. Phys Med Biol (2018). 63:185022. doi:10.1088/1361-6560/aadbcd
8. Gundacker, S, Turtos, RM, Auffray, E, Paganoni, M, and Lecoq, P. High-frequency SiPM readout advances measured coincidence time resolution limits in TOF-PET. Phys Med Biol (2019). 64:055012. doi:10.1088/1361-6560/aafd52
9. Lecoq, P, Morel, C, Prior, J, Visvikis, D, Gundacker, S, Auffray, E, et al. Roadmap toward the 10 ps time-of-flight pet challenge. Phys Med Biol (2020). [Epub ahead of print]. doi:10.1088/1361-6560/ab9500
10. Gundacker, S, Turtos, RM, Kratochwil, N, Pots, RH, Paganoni, M, Lecoq, P, et al. Experimental time resolution limits of modern SiPMs and TOF-PET detectors exploring different scintillators and cherenkov emission. Phys Med Biol. (2019). 65:025001. doi:10.1088/1361-6560/ab63b4
11. Farukhi, MR, and Swinehart, CF. Barium fluoride as a gamma ray and charged particle detector. IEEE Trans Nucl Sci (1971). 18:200–4. doi:10.1109/TNS.1971.4325864
12. Ershov, NN, Zakharov, NG, and Rodnyi, PA. Spectral-kinetic study of the intrinsic-luminescence characteristics of a fluorite-type crystal. Optic Spectrosc (1982). 53:51–4.
13. Andriessen, J, Dorenbos, P, and Eijk, CV. Electronic structure and transition probabilities in pure and Ce3+doped BaF2, an explorative study. Mol Phys (1991). 74:535–46. doi:10.1080/00268979100102401
14. Laval, M, Moszyński, M, Allemand, R, Cormoreche, E, Guinet, P, Odru, R, et al. Barium fluoride—inorganic scintillator for subnanosecond timing. Nucl Instrum Methods Phys Res (1983). 206:169–76. doi:10.1016/0167-5087(83)91254-1
16. Rodnyi, PA. Core-to-valence transitions. In: Physical processes in inorganic scintillators. Chap. 3.3. Cleveland, OH: CRC Press LLC (1997). p. 111–29.
17. Rodnyi, PA. Core–valence luminescence in scintillators. Radiat Meas (2004). 38:343–52. doi:10.1016/j.radmeas.2003.11.003
18. Ikeda, T, Kobayashi, H, Ohmura, Y, Nakamatsu, H, and Mukoyama, T. Electronic structure of alkaline-earth fluorides studied by model clusters. ii. auger-free luminescence. J Phys Soc Jpn (1997). 66:1079–87. doi:10.1143/JPSJ.66.1079
19. Lecoq, P, Gektin, A, and Korzhik, M. Inorganic scintillators for detector systems. Physical principles and crystal engineering. 2nd ed. Cham, Switzerland: Springer (2017).
20. Valbis, YA, Rachko, ZA, and Yansons, YL. Short-wave UV luminescence of BaF2 crystals caused by crossover transitions. Soviet J Exp Theor Phys Lett (1985). 42:172.
21. Novotny, R, Riess, R, Hingmann, R, Ströher, H, Fischer, R, Koch, G, et al. Detection of hard photons with baf2 scintillators. Nucl Instrum Methods Phys Res Sect A Accel Spectrom Detect Assoc Equip (1987). 262:340–6. doi:10.1016/0168-9002(87)90871-0
22. Dorenbos, P, de Haas, J, Visser, R, Eijk, CWV, and Hollander, R. Absolute light yield measurements on baf/sub 2/ crystals and the quantum efficiency of several photomultiplier tubes. IEEE Trans Nucl Sci (1993). 40:424–30. doi:10.1109/23.256593
23. Zhu, R-y. A barium fluoride crystal calorimeter for the ssc. Berlin, Germany: Springer (1991). p. 437–55. doi:10.1007/978-1-4615-3746-5_44
24. Kirm, M, Lushchik, A, Lushchik, C, Nepomnyashikh, AI, and Savikhin, F. Dependence of the efficiency of various emissions on excitation density in baf2 crystals. Radiat Meas (2001). 33:515–9. doi:10.1016/s1350-4487(01)00044-0
25. Derenzo, S. The quest for new radiation detector materials (2008). Available at: https://www-group.slac.stanford.edu/ais/publicDocs/presentation88.pdf
26. Li, HH. Refractive index of alkaline earth halides and its wavelength and temperature derivatives. J Phys Chem Ref Data (1980). 9:161–290. doi:10.1063/1.555616
27. Zhu, RY. The next generation of crystal detectors. J Phys Conf Ser (2015). 587:012055. doi:10.1088/1742-6596/587/1/012055
28. Sarukura, N, Nawata, T, Ishibashi, H, Ishii, M, and Fukuda, T. Czochralski growth of oxides and fluorides. In: P Rudolph, editor Handbook of crystal growth. 2nd ed. Chap. 4. Boston, MA: Elsevier (2015). p. 131–68.
29 Bell, Z. Scintillation counters. In: Handbook of particle detection and imaging. Chap. 15. Berlin, Germany: Springer-Verlag (2012). p. 349–75.
30. Jansons, JL, Krumins, VJ, Rachko, ZA, and Valbis, JA. Luminescence due to radiative transitions between valence band and upper core band in ionic crystals (crossluminescence). Phys Status Solidi B (1987). 144:835–44. doi:10.1002/pssb.2221440244
31. Klamra, W, Lindblad, T, Moszyński, M, and Norlin, L. Properties of optical greases for BaF2 scintillators. Nucl Instrum Methods Phys Res Sect A Accel Spectrom Detect Assoc Equip (1987). 254:85–7. doi:10.1016/0168-9002(87)90486-4
32. Jamil, A, Ziegler, T, Hufschmidt, P, Li, G, Lupin-Jimenez, L, Michel, T, et al. Vuv-sensitive silicon photomultipliers for xenon scintillation light detection in nexo. IEEE Trans Nucl Sci (2018). 65:2823–33. doi:10.1109/TNS.2018.2875668
33. Gallina, G, Giampa, P, Retière, F, Kroeger, J, Zhang, G, Ward, M, et al. Characterization of the hamamatsu vuv4 mppcs for nexo. Nucl Instrum Methods Phys Res Sect A Accel Spectrom Detect Assoc Equip (2019). 940:371–9. doi:10.1016/j.nima.2019.05.096
34. Zhu, R-y. On quality requirements to the barium fluoride crystals. Nucl Instrum Methods Phys Res Sect A Accel Spectrom Detect Assoc Equip (1994). 340:442–57. doi:10.1016/0168-9002(94)90125-2
35. Gola, A, Acerbi, F, Capasso, M, Marcante, M, Mazzi, A, Paternoster, G, et al. Nuv-sensitive silicon photomultiplier technologies developed at fondazione bruno kessler. Sensors (2019). 19:308. doi:10.3390/s19020308
36. Gundacker, S, Knapitsch, A, Auffray, E, Jarron, P, Meyer, T, and Lecoq, P. Time resolution deterioration with increasing crystal length in a tof-pet system. Nucl Instrum Methods Phys Res Sect A Accel Spectrom Detect Assoc Equip (2014). 737:92–100. doi:10.1016/j.nima.2013.11.025
Keywords: coincidence time resolution, scintillator, cross-luminescence, silicon photomultiplier, deep ultraviolet, positron emission tomography, high energy physics,
Citation: Pots RH, Auffray E and Gundacker S (2020) Exploiting Cross-Luminescence in
Received: 08 August 2020; Accepted: 22 September 2020;
Published: 29 October 2020.
Edited by:
Paul Sellin, University of Surrey, United KingdomReviewed by:
Yadong Xu, Northwestern Polytechnical University, ChinaLodovico Ratti, University of Pavia, Italy
Copyright © 2020 Pots, Auffray and Gundacker. This is an open-access article distributed under the terms of the Creative Commons Attribution License (CC BY). The use, distribution or reproduction in other forums is permitted, provided the original author(s) and the copyright owner(s) are credited and that the original publication in this journal is cited, in accordance with accepted academic practice. No use, distribution or reproduction is permitted which does not comply with these terms.
*Correspondence: Rosalinde Hendrika Pots, rosalinde.hendrika.pots@cern.ch