- 1School of Architecture and Civil Engineering, Xi’an University of Science and Technology, Xi’an, China
- 2Department of Civil Engineering, Inha University, Incheon, Korea
To study the deformation behavior of the high rockfill (HRF) embankment during construction and operation, based on more than a 50-m high rockfill embankment of the second-class road reconstruction project from Xunyang to Ankang of the national road 316 in Shaanxi, China, the centrifuge model test was performed to study the deformation laws of HRF embankment. The test results showed the following: (i) the HRF embankment was stable during construction and operation; (ii) during construction, the settlement occurred at the embankment top, and uplift occurred at the slope foot. Moreover, the deformation at the top was greater than that at the slope foot, and the deformations at both the top and the slope toe reached the maximum value at the end of construction; (iii) during operation, the settlement at the embankment top continued, and it changed rapidly at the start of operation. And then, the rate of the settlement slowed down and reached a steady state finally. The deformation of the slope foot was very small. This study can provide basis and reference for the design and construction of a similar project in mountain areas.
Highlights
A model of the HRF embankment was established based on the actual project.
A centrifuge test was performed to simulate the construction and operation of the HRF embankment.
The deformation laws of the HRF embankment during construction and operation were obtained.
Introduction
When building a road in mountainous areas, due to lack of adequate soil fillers, rock ballast generated by excavation of road cutting and tunnels becomes the main roadbed fillings [1]. High rockfill (HRF) embankment that makes full use of the excellent properties of wasted slag, reduces the damage of slags to the ecological environment, and resists the geological disasters to ensure the travel security has become a more common form of the roadbed for high-grade highways in mountainous areas. However, the uneven settlement of the rockfill embankment, in particular the HRF embankment, has been one of the subjects that road builders need to pay attention to.
Therefore, scholars at home and abroad have done a lot of research on the subgrade settlement. Some scholars have shown considerable interest in the laws of roadbed settlement and deformation by means of experiments. The deformation characteristics of high embankment were studied through centrifuge tests with an airport in Southwest China as the engineering background [2]. The centrifuge test was used to study the settlement laws of loess high-filled under different boundary conditions (flexibility and rigidity) [3]. Jing et al. [4] took the 69-m high loess embankment of the Lanzhou–Haishiwan expressway in 109 National Highway as the background of the study, and used centrifuge tests to study the distribution laws of settlement along the height, the longitudinal, and the transverse of the high embankment. A set of centrifugal model tests were used to verify the suitability of the embankment with red-bed soft rock (the general name of Jurassic, Cretaceous purple-red, brown-red sandstone, mudstone, and shale interbedded strata with strength within the soft rock range). Meanwhile, a series of centrifuge tests were carried out on the soil-rock–filled roadbed with different water contents, different degrees of compaction, and different particle compositions to study the settlement and deformation behaviors of the soil-rock–filled roadbed [5]. The settlement deformation characteristics of the high fill embankment were developed under different designed dry densities and water contents through centrifuge tests [6,7]. Kohgo et al. [8] studied the influences of water level variation on the stability of rockfill embankment in reservoir areas by using a centrifugal model. But the results are affected by the rockfill parameters. A series of dynamic centrifugal model tests were conducted to explore the influences of water level, embankment height, slope height, compaction degree, and rockfill on the anti-seismic properties of embankment [9].
At the same time, the subgrade settlement laws had been predicted by some scholars using prediction models. Based on the combined prediction idea, a method of variable weight combination forecasting was proposed for various common prediction models, which can be used to predict the development of subgrade settlement with the limited settlement data [10]. Jing et al. [11] took advantage of the settlement data of the loess high embankment of the Lanzhou–Haishiwan expressway as the study sample, and adopted the GM(1,1) (gray theoretical prediction model) with modified nonuniform filling and unequal step settlement observation time to carry out the settlement prediction of the loess high embankment. Based on the gray theory, an equal-length gray differential equation was established for the settlement and used the optimization theory to predict the settlement of the HRF embankment [12]. With considerations to characteristics of soft soil and various geometric parameters of the embankment, a method was proposed to predict settlement deformation of embankment under loads by using the artificial neutral network system. This approach is highly applicable to soft soil foundation [13]. Miščević et al. [14] developed a calculation model to predict the additional settlement of the embankment caused by alteration of rock-fill and expanded the knowledge range on settlement deformation of the embankment for solving the additional settlement of the embankment caused by the worsening of soft rock-fill. Based on the hardening soil model, the elastic-plastic model of double-yield surfaces was improved and the settlement of the HRF embankment during the construction period was simulated [15].
Nevertheless, based on the existing theoretical and constitutive models, the subgrade settlement methods and laws have been studied more widely. Based on the stratified summation method, the settlement calculation of the subgrade and HRF embankment were analyzed separately [16], and with the help of the settlement observation data of the project, they proposed a calculation method for post-construction settlement of HRF embankment. The settlement laws, lateral displacement, and stability of HRF embankment during construction of the Mazhu Expressway were studied by numerical simulation [17]. A three-parameter constitutive model was proposed to describe the creep properties of rock-fills by regarding the fillers as a viscoelastic medium. On this basis, the genetic algorithm and finite element analysis was introduced, and an optimized inversion method was established to determine the parameters of the three-parameter constitutive model. The post-construction settlement analysis was carried out with an engineering example [18]. Based on the monitoring results of post-construction settlement of Lüliang Airport high fill foundation, Zhu et al. [19] analyzed the post-construction settlement components of the original foundation and building body and the causes of the uneven settlement and proposed a method of recursive analysis of the post-construction settlement based on the strain rate.
The settlement mechanism of the HRF embankment was developed based on the deformation characteristics of fillings [20]. A modified Duncan–Chang model was proposed that can consider both shear deformation and compression deformation to calculate subgrade settlement [21]. Zhang et al. [22] proposed a practical method to predict the subgrade settlement with long-term traffic loadings, based on the theory of the equivalent viscoelastic-plastic model.
As a result, it is clear that the current research findings of the subgrade settlement primarily focused on soil embankments using theoretical derivation, numerical simulation, and settlement prediction models. In order to analyze the stability of the HRF embankment during the construction and the long-term operation, and broaden the knowledge range of the HRF embankment, this study based on the section from K21 + 350 to K21 + 541.751 of the HRF embankment of the second-class road reconstruction project from Xunyang to Ankang of the national road 316 in Shaanxi, China, the centrifugal model test was carried out to explore the deformation behavior of the HRF embankment during the construction and operation. This study would provide reference for the construction and stability analysis of HRF embankment in the Qinba mountain district.
Research Background
Engineering Background
There were two initial spoil grounds designed in the second-class highway reconstruction project from Xunyang to Ankang of national highway 316 in Hanbin District. The initial design capacity of waste slag was about 28.6 km3, of which 91.837 km3 was spoil and 188.728 km3 was abandoned stone, which resulted in the need of ground of 47.69 acres to stack up the dregs temporarily. There are many house-intensive villages along the engineering. Meanwhile, there are mountains on the right and the Han Jiang River and the Xiangyu Railway on the left of the engineering, respectively.
However, the original construction drawings of spoil ground were narrow and steep, and the annual runoff and land occupied were large in the region. Therefore, the construction units proposed to turn the Zhoujiahe Bridge at K21 + 443 into the HRF embankment with lots of waste slags, which could make well use of the terrain to reduce the damage caused by slags. And including the following advantages (i) save land effectively, which reduce the difficulty of land acquisition, (ii) protect water quality of the Han River to ensure the safety of residents, and (iii) the potential risks in the railway operation to speed up progress of the project. It is worth noted that the roadbed width of the project from K21 + 350 to K21 + 541.751 designed HRF embankment is only 8.5 m in the original design. Given that the subgrade will be widened in the future, so the width of roadbed was being built up to 12 m by wide each side of the road about 1.75 m. Consequently, related parameters of the project from K21 + 350 to K21 + 541.751 are listed as the following: the maximum filling height of the subgrade center was 37.2 m, and the maximum height of the slope was 50.6 m. Three slopes were built with the slope rate 1:1.5, 1:1.75, and 1:2, respectively, at the height of 8, 12, and 20 m, respectively, and the width of all platforms was 3 m. The slope rate of the fourth slope was 1:2. Simultaneously, the lithologic nature of the foundation base from upper to lower is silty clay, strong weathered quartz schist, and middle weathered quartz schist, respectively, according to the geological survey. Based on the engineering background, settlement of the HRF embankment will be further studied in the following section.
Model Design
The actual profile of K21 + 460 as the objective in this study was shown in Figure 1. In view of the actual K24 + 460 profile is too complex for experimental research, some simplification was listed as the following: i) the small steps on the surface of the basement under the embankment were merged, ii) the boundary on the right of the model was extended to the shoulder of the old road, and iii) the footwall on the left of the embankment was removed. Figure 2 shows the final experimental model.
According to “specification for geotechnical centrifuge model test techniques [23],” the geometric similarity scale of the model was determined to be 1/200 because the interface span is too large. Meanwhile, the NHRI-400 gt centrifuge centrifugal acceleration was determined to be the maximum centrifugal acceleration of 200 g. Table 1 shows the similarity rates of other physical quantities.
Materials and Methods
Raw Materials
The fillings of the HRF embankment consisted of sericite quartz schist and loess, which are introduced as follows. Figure 3 shows the raw materials of rockfill embankment fillers.
Sericite Quartz Schist
The engineering was built along the natural slope of 15–60° on the left bank of the Han Jiang River, and the physiognomy belongs to the first terrace of the Han Jiang River. The overburden mainly consists of Quaternary alluvial deposits and residual slope deposits. And the underlying bedrock is sericite quartz schist, limestone, and siliceous slate of the Silurian Meiziya Formation. Therefore, the fillers of the project from K21 + 350 to K21 + 541.751 are sericite quartz schist with quality grade class of III and not easily softened. The space between the rocks was filled with loess. According to the report of engineering geological exploration, uniaxial saturation compressive strength of the rock meets the requirements of subgrade filling [24]. As a result, the rock can be used as roadbed fillings on the premise of crushing and rolling. Table 2 presented detailed material mechanical parameters. Based on the sizes of the model box, the maximum size of the stone was 40 mm.
Loess
The loess was taken from the construction site and was disposed by paving, drying, rolling, and sifting.
Water
Tap water was used as the mixing water.
Fillers Preparation
The raw materials of the embankment fillers were homogeneously mixed using a mixer (Figure 3). Based on the main purpose, contents of stone were identified as accounting for 30% of the total mass. Based on experience, the moisture content of 13.2% is the optimum moisture content for this soil, when water was added to the clay for mixing. Simultaneously, at this moisture content, the soil is most compacted. Finally, the filler was sealed for about 24 h.
According to the mass and volume of the model, the compaction density of the mixture was determined at about 2.32 g/cm3. The moisture content was about 9.74% obtained by conversion of the proportion of soil to stone. The dry density was obtained by conversion of the compaction density and moisture content, which was about 2.11 g/cm3.
Experimental Process
(1) The fillings were spread evenly in the model box and compacted in layers; the layer between layers was treated with napping, and the thickness of the paving layer was not more than 50 mm (Figure 4).
(2) The model was hoisted into the centrifuge hanging basket platform, and the designed gravity acceleration of 200 g was applied to it for about 0.5 h to recover the self-weight stress field of the soil.
(3) According to the design data, the model was cut to form a model of the HRF embankment, and the deformation meshes were drawn in the model section (Figures 5, 6). Figure 5 shows, according to the design data, that the model was cut to form a model of the HRF embankment before the test. In order to prevent the surface of the soil from cracking due to water loss during a long-term test, a layer of silicone oil was brushed on the surface of the soil. Then, an organic glass and a laser displacement sensor were installed.
(4) After the silicone oil was completely dried, the model was weighed and hoisted into the centrifuge hanging basket platform; the laser displacement sensor was connected to the data acquisition system, and the centrifuge counterweight was adjusted.
(5) The centrifuge was turned on, and the centrifugal acceleration reached to 200 g in 396 s and continued to operate for about 3 h and 20 min. During the test, the laser displacement sensor was used to record the deformation of slope foot and pavement of the HRF embankment.
(6) The centrifuge was stopped and the coordinates of the deformed grid nodes were measured (Figure 7), and the test ended. The data acquisition system was working until the end of the test. The construction period of 6 months and the operation period of 15 years were simulated in this experiment.
Test Setup
As illustrated in Figure 8, a 400 gt geotechnical centrifuge of the Nanjing Hydraulic Research Institute (NHRI) was used to perform the centrifuge test. Table 3 shows the main technical indexes of it. However, the geotechnical centrifuge setup also included a model box, a system of data acquisition, and sensors.
The type of measuring instrument has a great influence on the results of the laboratory and field test [25]. The sensors that can be used to measure embankment displacement include strain gages and LVDTs, which are used in displacement measurement with high-precision requirements [26,27]. Considering the large running speed of the centrifuge, the sensor (Figure 9) used in the centrifuge model test is an ideal noncontact high-precision laser displacement sensor (Wenglor YP11MGVL80), with a range of 50 mm and an accuracy of 20 μm. Sadeghi and Esmaeili et al. [28–30] gave a detailed introduction to the specifications of more test measuring instruments.
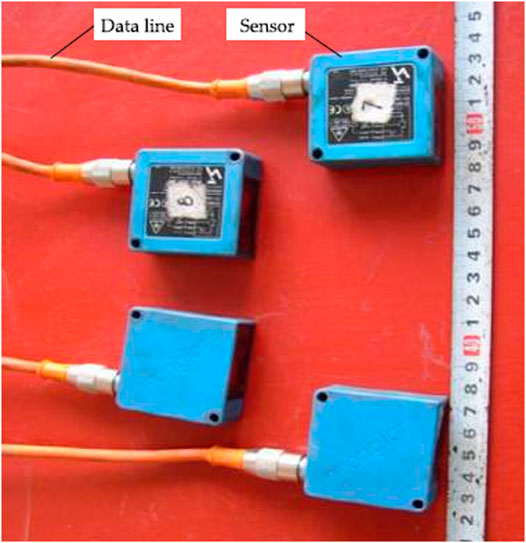
FIGURE 9. High-precision laser displacement sensors used to measure the top of the embankment deformation.
Results and Discussion
Results of Test
During construction and operation, the overall deformation of the slope foot and pavement of the HRF embankment was presented in Figure 10. During the operation period, the deformation of the slope foot and pavement was presented in Figure 11. Before and after the test, the coordinates of the deformation grid node in Figures 8, 9 were measured, and the deformation vector field of the soil was drawn, as shown in Figure 12.
Analysis of Results
It can be clearly seen from Figure 10 that 1) for the HRF embankment, the settlement of top was not only considered in the construction period but also in the operation period; the deformation of the slope foot was mainly in the construction period, and the deformation of post-construction was small; 2) during the construction period, the settlement of the top occurred, at the same time, uplift of the slope foot occurred, and the settlement was greater than the uplift. At the end of the construction period, the deformation both at the top and at the slope foot has reached the maximum value, and 3) during the operation period, the settlement at the top continued, while the deformation of slope foot was small, but the law of deformation was complicated.
During the construction period (Figure 10), the settlement of the top was increasing with the increase in the filling height. When the progress of filling was completed, the settlement deformation at the top was about 212 mm, comparatively, the deformation law of the slope foot was a bit complicated, but it basically showed the law that increases with the settlement development of the top; at the end of filling, when the settlement of the top become the maximum, the uplift of the slope foot reached the maximum and was about 169 mm. The uplift at the slope foot of the embankment was less than the settlement at the top.
During the operation period (Figure 11), the law of the settlement at the top of the embankment was obvious: 1) in the initial period of operation, the variation of settlement was large and about 106 mm that occurred in two years; 2) from the second year to the fifth year, the settlement changed from about 108 mm to about 154 mm and increased by about 46 mm in 3 years, which should be seen as the rate of settlement had slowed down significantly; 3) from the fifth year to the fifteenth year, the rate of settlement with time was basically stable. The settlement changed from about 154 mm to about 253 mm and the average annual settlement was about 9.8 mm; 4) on the whole, after operation of fifteen years, the settlement at the top of the embankment was about 253 mm, of which about 42% occurred in the first two years, and the settlement in the first five years accounted for about 61% of the total, and in the following 10 years, the rate of settlement deformation tended to be stable. For the slope toe, the law of deformation was complicated. And from official operation to the fifth year and six months, the performance was settlement deformation and the maximum was about 21 mm; subsequently, the tendency of deformation showed as repeated uplift and settlement, but the overall trend was uplift and the maximum was about 34 mm. The data would indicate that the HRF embankment was stable during the long-term operation. Those findings in this study have a good agreement with [2, 4] in which the total settlement during the operation period increased with time, but the rate of change gradually decreased.
It should be noted that the simulation of filling progress in centrifuge tests is different from the construction of actual engineering. In centrifuge tests, the progress of embankment filling is simulated by increasing the centrifugal acceleration step by step, and at the end of construction, the settlement results in the elevation of the top of embankment lower than the design value. Therefore, the test results of the settlement of the top of embankment can be used as a basis to calculate the extra earthwork in the practical project, and accordingly, the results of uplift of the slope foot can be used as a basis to calculate the extra excavated volume in practical engineering.
Through the deformation vector field of the model (Figure 12), we can see intuitively that for the deformation of the slope foot, the impact of construction and long-term operation of the HRF embankment, both from the value of deformation and the scope of deformation, was very limited, and it would not affect the stability of the slope foot and the adjacent railway and water area.
Conclusion
To analyze the stability of the HRF embankment during the construction and the long-term operation, and broaden the knowledge range of the HRF embankment, the centrifuge model test was carried out based on the actual project to explore the deformation behavior of the HRF embankment during the construction and operation. Although there is a similarity to a certain extent for centrifuge tests, it still plays an important role in guiding practical engineering. According to the above result analyses, the following conclusions can be drawn:
(1) For the HRF embankment, the settlement at the top of embankment had always existed both in the construction period and long-term operation period. While the deformation of the slope foot occurred mainly during the construction period, and the deformation of post-construction was small.
(2) During the construction period, the deformation of embankment was the settlement and the deformation of slope foot was uplift, and at the end of the construction, the settlement at the top of the embankment reached the maximum value, and the uplift of the slope toe reached the maximum value.
(3) During the long-term operation period, the settlement at the top of the embankment kept on increasing, and at the initial stage of operation the settlement changed faster. And then, the rate of settlement slowed down gradually and tended to stability. For the slope foot, the law of deformation was complicated, which showed the trend of settlement-uplift-settlement-uplift-settlement. However, the deformation of slope foot during operation was only in the range of about -21 mm (settlement)∼34 mm (uplift), indicating that the operation of the engineering has a small impact on the stability of the slope foot.
Data Availability Statement
The raw data supporting the conclusions of this article will be made available by the authors, without undue reservation.
Author Contributions
M-jG and Y-qZ: writing—original draft preparation; H-jJ: methodology; and K-iS: validation. All authors have read and agreed to the published version of the manuscript.
Funding
The authors are grateful for the support provided by the National Natural Science Foundation of China (Grant No. 51378090) and School of Architecture and Civil Engineering, Xi’an University of Science and Technology.
Conflict of Interest
The authors declare that the research was conducted in the absence of any commercial or financial relationships that could be construed as a potential conflict of interest.
Publisher’s Note
All claims expressed in this article are solely those of the authors and do not necessarily represent those of their affiliated organizations, or those of the publisher, the editors and the reviewers. Any product that may be evaluated in this article, or claim that may be made by its manufacturer, is not guaranteed or endorsed by the publisher.
References
1. Zhang YQ, Jing HJ, Dai J, Li SF, Zhang Z. Stability of High Rockfill Embankment Based on Orthogonal Test and Numerical Simulation. Tehnički vjesnik (2020) 27(1):191–9. doi:10.17559/tv-20191216092415
2. Liu H, Zhang ZY, Han WX. Centrifugal Model Tests for Settlement of High Embankment. J Southwest Jiao Tong Univ (2003) 38(3):323–6.
3. Cao J, Zheng JG, Zhang JW. Centrifuge Tests of Loess High-Filled Settlement under Different Boundary Conditions. J China Water Resour Hydropower Res Inst (2017) 15(4):256–61.
4. Jing HJ, Hu CS, Wang BG. Study on Settlement and Deformation Laws of High Loess-Fill Embankment. Chin J Rock Mech Eng (2005) 24(S2):5845–50.
5. Qing SH, Huang RQ, Cao XW. Centrifugal Model Test of Red and Soft Rock-Filled Embankment. J Railway Eng Soc (2007)(2) 41–6.
6. Dong Y, Chai HJ, Yan ZL. Centrifugal Model Test Study on the Settlement Character of the Rock-Soil Filled Roadbed. J Highw Transportation Res Develop (2007) 24(3):25–9.
7. Jiang Y, Chai HJ. Study on Centrifugal Model Settlement experiment of High Filling Embankments. Mod Transportation Techn (2007) 4(6):5–8.
8. Kohgo Y, Takahashi A, Suzuki T. Centrifuge Model Tests of a Rockfill Dam and Simulation Using Consolidation Analysis Method. Soils and Foundations (2010) 50(2):227–44. doi:10.3208/sandf.50.227
9. Enomoto T, Sasaki T. Several Factors Affecting Seismic Behaviour of Embankments in Dynamic Centrifuge Model Tests. Soils and Foundations (2015) 55(4):813–28. doi:10.1016/j.sandf.2015.06.013
10. Zhao MH, Liu JB, Yu Y. Study on Variable-Weight Combined Forecasting Method of the Settlement in High Rock-Filled Embankment. J Hunan Univ Sci Techn (Natural Science) (2005) 20(4):53–7.
11. Jing HJ, Yu MH. Model Study for Prediction of Settlement of Loess-Fill High Embankments. China Civil Eng J (2006) 39(8):113–7.
12. Zhao MH, Zeng GX, Xiang ZF. Gray Optimizing-Markov Predicating Model on Settlement of High-Enrockment Embankment. J Highw Transportation Res Develop (2006) 23(11):19–22.
13. Chik Z, Aljanabi QA. Intelligent Prediction of Settlement Ratio for Soft clay with Stone Columns Using Embankment Improvement Techniques. Neural Comput Applic (2014) 25(1):73–82. doi:10.1007/s00521-013-1449-0
14. Miščević P, Vlastelica G. Estimation of Embankment Settlement Caused by Deterioration of Soft Rock Grains. Bull Eng Geology Environ (2019) 78(3):1843–53.
15. Sukkarak R, Pramthawee P, Jongpradist P. A Modified Elasto-Plastic Model with Double Yield Surfaces and Considering Particle Breakage for the Settlement Analysis of High Rockfill Dams. KSCE J Civ Eng (2017) 21(3):734–45. doi:10.1007/s12205-016-0867-9
16. Cao XR, Zhong SB, Yu YH, Li L. Study on Settlement of High Fill Rock Embankment after Construction and its Engineering Algorithm. J Hunan Univ (Natural Science) (2002) 29(6):112–7.
17. Yang B, Wang B, Zhu JB, Li C. Computational Analysis of Settlement and Stability of a High Rockfill Embankment in Construction Period of Ma-Zhu High Way. Transportation Sci Techn (2016)(1) 79–82.
18. Cao WG, Li P, Cheng Y. Research of Creep FEM in Calculating Settlement of High Rock- Filled Embankment after Construction and its Back Analysis. Rock Soil Mech (2005) 26(S):175–8.
19. Zhu CH, Li N, Liu MZ, Wei YF. Spatiotemporal Laws of Post-Construction Settlement of Loess-Filled Foundation of Lüliang Airport. Chin J Geotechnical Eng (2013) 35(2):293–301.
20. Xie YZ, Yang CZ, Xia XQ, Cheng YP, Ren WB. Study on Settlement Mechanism of High Rockfill Embankment. J Highw Transportation Res Develop (Applied Technology) (2008)(3) 28–31.
21. Cao XR, Zhao ZY, Zhao MH. Study on Settlement Calculation Method for Subgrade of High Rockfill Embankment. J Highw Transportation Res Develop (2005) 22(6):38–42.
22. Zhang XX, Zhang JM, Wen YF. Practical Method to Predict Settlement of Subgrade Induced by Long-TermTraffic Loads. Chin J Geotechnical Eng (2015) 37(11):2067–72.
23.Specification for Geotechnical Centrifuge Model Test T. echniques (DL/T 5102-2013). Beijing: China Electric Power Press (2014)5–16.
24. Wu YH. Highway Subgrade Design Code" JTG D30-2015 Phase I Evangelist Will Be Successfully Held in Guangzhou. Standardization Eng Construction (2015)(5) 70.
25. Zakeri J, Hassan Esmaeili M, Mazraeh A, Shadfar M. Impact of Heavy Urban Rail Vehicles Running over Light Rail Turnouts. Proc Inst Mech Eng F: J Rail Rapid Transit. (2021) 235(3):300–12. doi:10.1177/0954409720924308
26. Sadeghi J, Liravi H, Esmaeili MH. Experimental Investigation on Loading Pattern of Railway concrete Slabs. Construction Building Mater (2017) 153:481–95. doi:10.1016/j.conbuildmat.2017.07.025
27. Sadeghi J, Esmaeili MH, Akbari M. Reliability of FTA General Vibration Assessment Model in Prediction of Subway Induced Ground Borne Vibrations. Soil Dyn Earthquake Eng (2019) 117:300–11. doi:10.1016/j.soildyn.2018.11.002
28. Sadeghi J, Esmaeili MH, Shadfar M, Ashari Ghomi A. Influences of Railway Track Geometry Parameters on Ground-Borne Vibration. Int J Railway Res (2014) 1(1):11–8.
29. Sadeghi J, Esmaeili MH. Safe Distance of Cultural and Historical Buildings from Subway Lines. Soil Dyn Earthquake Eng (2017) 96:89–103. doi:10.1016/j.soildyn.2017.02.008
Keywords: road engineering, high rockfill embankment, centrifuge tests, settlement, long term deformation
Citation: Jing HJ, Gou MJ, Zhang YQ and Song K-I (2021) Investigation on the Settlement of High Rockfill Embankment Using Centrifuge Tests. Front. Phys. 9:547991. doi: 10.3389/fphy.2021.547991
Received: 01 April 2020; Accepted: 31 May 2021;
Published: 09 August 2021.
Edited by:
Guoyang Fu, Monash University, AustraliaCopyright © 2021 Jing, Gou, Zhang and Song. This is an open-access article distributed under the terms of the Creative Commons Attribution License (CC BY). The use, distribution or reproduction in other forums is permitted, provided the original author(s) and the copyright owner(s) are credited and that the original publication in this journal is cited, in accordance with accepted academic practice. No use, distribution or reproduction is permitted which does not comply with these terms.
*Correspondence: Hong Jun Jing, amluZ2hvbmdqdW5AeHVzdC5lZHUuY24=