- 1School of Electronic Engineering and Automation, China Guangxi Key Laboratory of Optoelectronic Information Processing, Guilin University of Electronic Technology, Guilin, China
- 2National Research Center of Intelligent Equipment for Agriculture, Beijing Academy of Agriculture and Forestry Sciences, Beijing, China
Accurate monitoring of ammonia has decisive significance for the environmental control of poultry housing. Existing sensors based on semiconductor or electrochemistry have the defects of short life, severe baseline drift and delayed response when facing the harsh environment of poultry housing. In this work, we developed a portable sensor based on tunable diode laser absorption spectroscopy with a micro circular absorption cell for sensitive detection of ammonia in poultry housing. The micro circular absorption cell has a volume of only 25 ml, but the effective absorption path is up to 5 m, which allows the sensor to achieve the ability of less than 15 s response time and 0.2 ppm measurement accuracy. The results of continuous monitoring for 6 days showed that the ammonia concentration in the range of 0–6 ppm was accurately detected in a poultry house with 36 roosters. Through analyzing dynamic changes in ammonia concentration, we successfully identified some abnormal activity caused by humans or weather. Therefore, our sensor has performances of accurate, stable, real-time measurement of ammonia and can provide strong technical support for environmental control of poultry housing.
Introduction
With the development of the economy, the poultry breeding mode has changed from free-ranging to intensive farming mode. However, in intensive farming, fecal decomposition, animal respiration and padding decay produce large quantities of harmful gases such as ammonia [1–3]. Especially during the winter, farmers often close their poultry housing to ensure the proper temperature for the growth of poultry. Ammonia (NH3) concentration in the housing remains high due to the lack of air circulation, and poultry exposed to such high ammonia levels for a long time can become ill or die [4–6]. Livestock farming is the primary source of ammonia emissions. Ammonia diffused from the livestock to the atmosphere will harm the health of breeders and surrounding residents, and induce various respiratory diseases [7–9]. Therefore, quick and accurate detection of ammonia concentration in poultry housing to timely guide ventilation and deodorization is not only the health needs of poultries and people but also the urgent needs of environmental protection and food safety.
Detection methods of ammonia in poultry housing are divided into laboratory methods and sensor methods [10]. The laboratory methods usually use Fourier transform infrared spectrometer, photoacoustic spectrometer and mass spectrometry to detect harmful gas concentration in livestock with a sub-ppm accuracy [11–14]. These instruments have the advantages of qualitative and quantitative accuracy, high sensitivity and multi-component measurement simultaneously, they are generally large, costly and complex in operation, making them difficult to use in the field for long-term monitoring. The sensor methods often integrated semiconductor or electrochemical gas sensors to detect ammonia in poultry housing. Compared with laboratory methods, these sensors have advantages of small size, low cost and easy operation [15–19]. However, these sensors have poor sensitivity, severe baseline drift, cross interference and short lifetime when used in poor poultry housing environments. This is why these sensors are not widely used for long-term monitoring of ammonia in poultry houses.
Tunable diode laser absorption spectroscopy (TDLAS) uses a tunable distributed feedback (DFB) laser which has a narrow band wavelength corresponding to the gas absorption line to detect gas concentration specifically and sensitively. In recent years, TDLAS has been widely used to monitor harmful gases in industry [20–22]. However, existing commercial TDLAS sensors used for industrial gas monitoring usually have a high detection limit and large size with a heavy frontal absorption cell, which makes them unsuitable for high-precision, on-site measurement of trace ammonia in poultry houses. In this paper, we aimed to develop a portable TDLAS sensor for monitoring trace ammonia in poultry houses. For this, a unique and micro circular absorption cell was designed. In addition, the sensor also integrated temperature, humidity, total volatile organic compounds (TVOC), dust and carbon dioxide (CO2) sensors to obtain parameters related to air quality in poultry houses.
Structure of the sensor and circular absorption cell
Measurement principle
TDLAS mainly uses the property of tunable semiconductor lasers that the narrowband wavelength of the laser change with the injection current and temperature to achieve a high-resolution quantitative analysis of gas concentration. After passing through the gas to be measured, the laser intensity has a specific attenuation. However, direct analysis of the change of laser intensity is easy to be affected by various noises in the environment and equipment, and the measurement accuracy cannot meet the actual needs. Therefore, wavelength modulation spectroscopy (WMS) improves the sensor’s measurement accuracy.
The principle of WMS is summarized as follows: Based on the Lamber-Beer law, when the laser passes through the measured gas, the relationship between the transmitted and incident light intensities at a specific wavenumber is:
Where
WMS is to transfer the detection object of the absorption spectrum from the low-frequency signal to the relatively high-frequency signal. The reason is that the noise in the system, such as 1/f noise or mechanical noise, has a greater impact on the low-frequency signal, while the high-frequency signal is not easily affected. By modulating the injection current of the tunable semiconductor laser, the wavelength and intensity of the output laser can be modulated at high frequency. When the high-frequency sine wave modulates the laser frequency, the instantaneous frequency and intensity of the incident laser can be expressed as:
Where
The amplitude of second harmonic signal extracted by lock-in amplifier can be expressed as:
Where
Structure of the sensor
According to the HITRAN database [23] and commercially available lasers, we selected a tunable laser with the spectral line of 1,512 nm from Wuhan 69 Sensor Technology Co. LTD for specific and sensitive detection of NH3 gas. The structure of the sensor is shown in Figure 1. The 1,512 nm tunable laser works at a constant temperature by a temperature controller. The working temperature is 25°C, and the current scan range is 55–65 mA. Its spectral line will be scanned by a drive circuit combined a high-frequency and small amplitude sine wave superimposed on a low-frequency and large amplitude sawtooth wave. The frequency of the sawtooth wave is 5 Hz, and the sine wave is 5 kHz. This scanning method is usually called wavelength modulation spectroscopy which can extract weak absorption signals from the strong background noise. When the NH3 gas was pumped into the circular absorption cell, the laser beam was absorbed and detected by the photodetector. The type of photodetector is g10899-01k from Hamamatsu Photonics (China) Co. LTD. Then the second harmonic signal for concentration inversion was demodulated by the lock-in amplifier and collected by the microcontroller (MCU). The type of MCU is STM32F103. According to Beer-Lambert law [24], the gas concentration is proportional to the second harmonic amplitude, absorption line strength, optical path and pressure. At normal temperature and pressure, the absorption line strength, optical path and pressure are known and constant. Therefore, the ammonia concentration has a positive proportional relationship with the second harmonic amplitude.
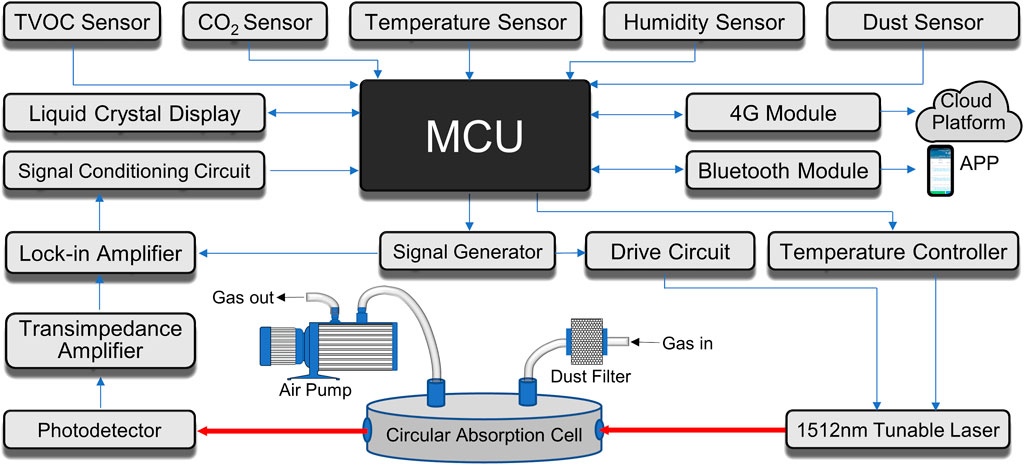
FIGURE 1. Schematic diagram of the sensor for monitoring NH3 gas based on the TDLAS technology and other environmental parameters in the poultry housing.
In addition, TVOC, CO2, temperature, humidity and dust sensors are also integrated into our sensor system to obtain parameters related to air quality in poultry houses. Various sensor modules integrated into the system have their measurement accuracy, and the system adopted the all-in-one sensor module PTQS1005A from Beijing Planttower Co. LTD. Among them, the temperature range is between −10–55°C, the resolution is 0.1°C; The humidity measurement range is 0–99%, the resolution is 0.1%; The TVOC range is 0–10 ppm, the resolution is 0.01 ppm; The minimum detection limit of dust sensor is 1ppb; The range of CO2 sensor is 400–3000 ppm. The data transmitted through the communication module is the value of the gas content in the environment obtained at the corresponding time, which will be transmitted to the mobile app in real time and automatically saved in the Excel table for the measurement personnel to view and analyze.
Design of the circular absorption cell
The measurement principle of TDLAS is based on Beer-Lambert law [24]. The detection sensitivity is often improved by increasing the effective absorption path at the same absorption line. However, the volume of the absorption cell with a long optical path is usually large. This results in a long response time and inapplicability to develop compact and portable TDLAS sensors [25]. With the trend of miniaturization of TDLAS sensors, more and more attention has been paid to the micro absorption cell with a long optical path [26]. Absorption cells based on several structures have developed to satisfy different application situations [27]. In this paper, by borrowing from published technologies [28–30], we designed a micro circular absorption cell for ammonia detection in poultry housing, just as shown in Figure 2. Compared to the circular absorption cell made up of many small reflectors in the Ref. [31], our cell used an integral ring reflector with the diameter of 60 mm and height of 10 mm to make it easy to install and has a better stability. The ring reflector was coated with an enhanced silver metal film with a reflectivity of more than 99% within the spectral region of 800–2000 nm. Using a cylindrical mirror, the incident beam with a diameter of 1.5 mm was shined on the ring reflector and reflected about 70 times. Therefore, the cell could reach up to a 5 m optical path only within a volume of 30 ml, which ensures high sensitivity and fast response time for NH3 detection.
Sensor performance and application in poultry housing
Sensor performance
Ammonia concentration in poultry housing is regulated to protect worker health and animal productivity in countries and regions worldwide. The European Union limits ammonia in poultry to 20 ppm, America allows a weighted average exposure limit of 50 ppm for humans for 8 h, and The United Egg Producers state indicates that ammonia levels in chicken houses should ideally be less than 10 ppm and not more than 25 ppm [32–34]. We fit the second harmonic peak obtained by measuring standard ammonia according to the variation range of ammonia concentration in poultry housing. The harmonic peak has an excellent linear relationship with the low ammonia concentration in poultry housing.
As shown in Figure 3A, we continuously measured the standard gas to verify the sensor performance and conducted it under laboratory conditions to ensure accuracy. As shown in Figure 3B,C, the standard gas concentration of 2 ppm and 50 ppm were continuously measured at 24 h with intervals of 3 min. The data fluctuated by 0.2 ppm during measured ammonia concentration of 2 ppm and fluctuated by 0.1 ppm when measured ammonia concentration of 50 ppm. The time-corresponding concentration data in Figures 3B–D is obtained by using Origin to draw the data from the mobile app. Prolonged monitoring observed that the signal baseline had no drift, and the measurement of low concentration ammonia also had excellent precision and stability. The response time and recovery time are also essential performance metrics. To demonstrate the reversibility of the sensor, we measured the standard gas several times. Figure 3D shows the signal response when switching from air to 50 ppm ammonia three times. As a result, the sensor response time and recovery time remain within 15 s, and measurements can be quickly restored to air levels, meaning the sensor has excellent reversibility. The data indicated that the sensor could be used to monitor the dynamic changes at low concentrations of ammonia to meet the need for ammonia monitoring in poultry housing.
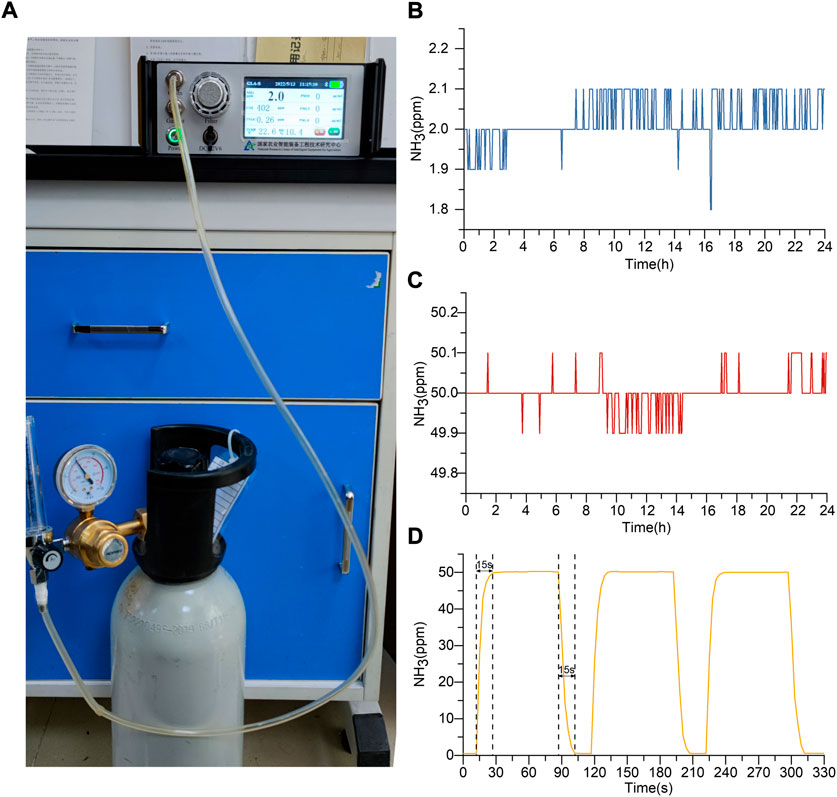
FIGURE 3. (A) Photograph of measuring standard ammonia; (B) Monitoring data for 2 ppm ammonia within 24 h; (C) Monitoring data for 50 ppm ammonia within 24 h; (D) Repeat measurement of 50 ppm ammonia.
Application in poultry housing
We used the sensor to monitor the broiler chicken house environment on the farm for 6 days. There are 36 mature roosters in the monitoring field, staff regularly clean up and sanitize, and fewer external factors influence the data. As shown in Figure 4A, the sensor was placed in the broiler house on September 3 at 10:00 a.m., measurements were taken at intervals of 5 min, and normal production activities continued in the house.
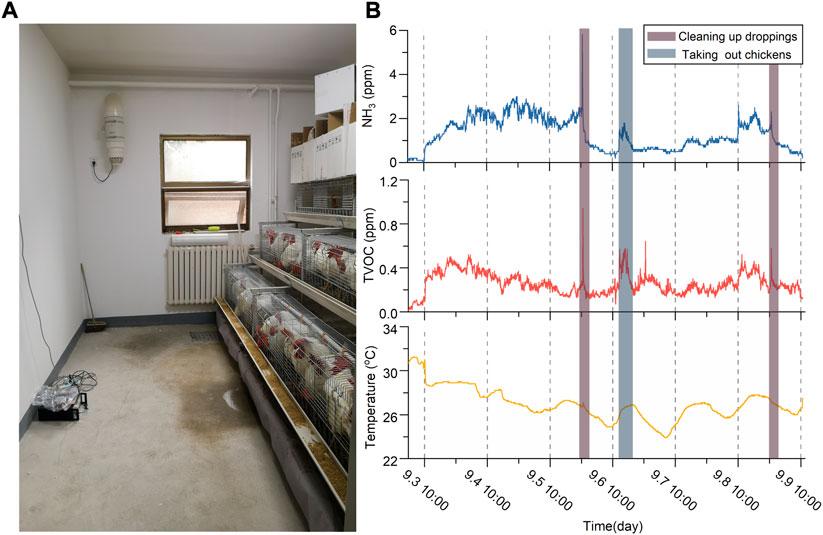
FIGURE 4. (A) Photograph from field measurement at the broiler house; (B) NH3, TVOC and temperature monitoring data in 6 days.
Figure 4B shows that ammonia concentration in the broiler house varied over time. In 6 days, the staff grabbed 16 chickens from the house (grey shadow) and cleaned up manure twice (brown shadow). As the manure accumulated, ammonia concentration continued to rise in the house and cleaning the manure caused a precipitous drop in ammonia concentration. The data confirmed that manure is the main source of ammonia in poultry housing [35]. Ammonia evaporated rapidly while cleaning manure, which caused the ammonia concentration to fluctuate significantly in a short period [36]. The concentration curve in Figure 4B is obtained using Origin to draw the data from the mobile app.
Similarly, measurements changed when some chickens were grabbed because of fluctuation in ammonia concentration by shaking the coop. The rapid rise and fall of ammonia caused by human activities demonstrated the rapid response of the sensor to monitor ammonia change in poultry housing. According to the Environmental Quality Standard For The Livestock And Poultry Farm of the People’s Republic of China, the concentration of ammonia in adult poultry housing is less than 15 ppm, and the measurement results meet the standards.
The environmental requirements of modern poultry housing are not limited to harmful gas concentration but also include temperature and humidity, dust and CO2 concentration. Abnormal temperature, humidity and other environmental factors affect the development of animals. The TVOC concentration and temperature in Figure 4B reflect the broiler house environment from the side. TVOC levels suddenly increased when cleaning the house and grabbing chickens, similar to the change in ammonia concentration. The temperature changed with the day and night rule and never exceeded 30°C. Upon completion of the measurement, the device was re-measured for standard ammonia and found to be unchanged, indicating that the measured concentration in the broiler house was accurate.
Conclusion
The experimental data shows a good ability to monitor the ammonia of the sensor in poultry housing. The performance of this sensor and some previous sensors is shown in Table 1. The volume of this sensor in Table 1 only includes the part of measure ammonia, excluding other integrated sensor modules. The sensor designed in this paper can quickly measure ammonia. As the volume is reduced, the accuracy remains at an excellent level.
Based on the TDLAS and circular absorption cell, the portable sensor of low monitor concentration of ammonia was studied. WMS reduces environmental noise interference and detects fainter signals. The sensor has an incredible increase in performance using the circular absorption cell. The small size of the absorption cell makes the chamber highly efficient for gas exchange at a fixed velocity. The low exposure area reduces ammonia memory effects on the inner surface. Experimental data shows that the sensor has the advantages of high sensitivity, high stability and fast response time in long-term monitoring. The system realized the multiple component measurement in a complex environment using integrated sensor modules. As an effective low concentration ammonia monitoring system, the sensor has considerable application potential in poultry housing.
Data availability statement
The original contributions presented in the study are included in the article/supplementary material, further inquiries can be directed to the corresponding author.
Author contributions
KW, RG, YZ, LJ, and DD contributed to the conception and design of the study. RG and LJ performed the simulation analysis and performed the experimental verification. KW and YZ wrote the first draft of the manuscript. LJ and DD presented the revision of the manuscript. All authors have read and agreed to the published version of the manuscript vision.
Funding
This research was funded by the Science and Technology Innovation 2030- Key Project of China (2021ZD0113801), National Natural Science Foundation of China (32101609), Innovation Capacity Building Project of Beijing Academy of Agriculture and Forestry Sciences (KJCX20200417), Beijing Innovation Consortium of Agriculture Research System (BAIC08-2022), and National and Local Joint Engineering Laboratory For Agricultural Internet of Things (PT2022-23).
Conflict of interest
The authors declare that the research was conducted in the absence of any commercial or financial relationships that could be construed as a potential conflict of interest.
Publisher’s note
All claims expressed in this article are solely those of the authors and do not necessarily represent those of their affiliated organizations, or those of the publisher, the editors and the reviewers. Any product that may be evaluated in this article, or claim that may be made by its manufacturer, is not guaranteed or endorsed by the publisher.
References
1. Webb J, Menzi H, Pain BF, Misselbrook TH, Dammgen U, Hendriks H, et al. Managing ammonia emissions from livestock production in europe. Environ Pollut (2005) 135(3):399–406. doi:10.1016/j.envpol.2004.11.013
2. Meda B, Hassouna M, Aubert C, Robin P, Dourmad JY. Influence of rearing conditions and manure management practices on ammonia and greenhouse gas emissions from poultry houses. World's Poult Sci J (2011) 67(3):441–56. doi:10.1017/s0043933911000493
3. Kupper T, Bonjour C, Menzi H. Evolution of farm and manure management and their influence on ammonia emissions from agriculture in Switzerland between 1990 and 2010. Atmos Environ (2015) 103:215–21. doi:10.1016/j.atmosenv.2014.12.024
4. Shah SWA, Chen D, Zhang J, Liu Y, Ishfaq M, Tang Y, et al. The effect of ammonia exposure on energy metabolism and mitochondrial dynamic proteins in chicken thymus: Through oxidative stress, apoptosis, and autophagy. Ecotoxicol Environ Saf (2020) 206:111413. doi:10.1016/j.ecoenv.2020.111413
5. Wei FX, Hu XF, Xu B, Zhang MH, Li SY, Sun QY, et al. Ammonia concentration and relative humidity in poultry houses affect the immune response of broilers. Genet Mol Res (2015) 14(2):3160–9. doi:10.4238/2015.April.10.27
6. Kocaman B, Esenbuga N, Yildiz A, Lacin E, Macit M. Effect of environmental conditions in poultry houses on the performance of laying hens. Int J Poult Sci (2005) 5(1):26–30. doi:10.3923/ijps.2006.26.30
7. Kilic I, Yaslioglu E. Ammonia and carbon dioxide concentrations in a layer house. Asian-australas J Anim Sci (2014) 27(8):1211–8. doi:10.5713/ajas.2014.14099
8. Naseem S, King AJ. Ammonia production in poultry houses can affect health of humans, birds, and the environment-techniques for its reduction during poultry production. Environ Sci Pollut Res (2018) 25(16):15269–93. doi:10.1007/s11356-018-2018-y
9. Green AR, Wesley I, Trampel DW, Xin H. Air quality and bird health status in three types of commercial Egg layer houses. J Appl Poult Res (2009) 18(3):605–21. doi:10.3382/japr.2007-00086
10. Kwak D, Lei Y, Maric R. Ammonia gas sensors: A comprehensive review. Talanta (2019) 204:713–30. doi:10.1016/j.talanta.2019.06.034
11. Mohan T, Sheik Farid K. V. S., Sustainable biological system for the removal of high strength ammoniacal nitrogen and organic pollutants in poultry waste processing industrial effluent. J Air Waste Manag Assoc (2020) 70(12):1236–43. doi:10.1080/10962247.2020.1731013
12. Van Huffel K, Heynderickx PM, Dewulf J, Van Langenhove HJCE. Measurement of odorants in livestock buildings: Sift-ms and td-gc-ms. Chemical Engneering (2012) 30. 978-88. doi:10.3303/CTE1230012
13. Ngwabie NM, Jeppsson KH, Gustafsson G, Nimmermark S. Effects of animal activity and air temperature on methane and ammonia emissions from a naturally ventilated building for dairy cows. Atmos Environ (2011) 45(37):6760–8. doi:10.1016/j.atmosenv.2011.08.027
14. Li H, Zhang C, Xin H. Performance of an infrared photoacoustic single gas analyzer in measuring ammonia from poultry houses. Appl Eng Agric (2015) 31(3):471–7. doi:10.13031/aea.31.10826
15. Lin T, Shah SB, Wang-Li L, Oviedo-Rondón EO, Post J. Development of mos sensor-based Nh3 monitor for use in poultry houses. Comput Elect Agric (2016) 127:708–15. doi:10.1016/j.compag.2016.07.033
16. Gates RS, Xin H, Casey KD, Liang Y, Wheeler EF. Method for measuring ammonia emissions from poultry houses. J Appl Poult Res (2005) 14(3):622–34. doi:10.1093/japr/14.3.622
17. Wheeler E. F., Casey K. D., Zajacz kowski J., Topper P. A., Ammonia emissions from U.S. Poultry houses: Part Iii – broiler houses. Third International Conference on Air Pollution from Agricultural Operations, Research Triangle Park, NC, USA, (October, 2003) (2003).
18. Aunsa-Ard W, Pobkrut T, Kerdcharoen T, Prombaingoen N, Kijpreedaborisuthi O. Electronic nose for monitoring of livestock farm odors (poultry farms). 2021 13th international Conference on Knowledge and smart technology. KST. (January, 2021). Bangsaen, Chonburi, Thailand. Thailand: IEEE (2021). 176–80.
19. Lahav O, Mor T, Heber AJ, Molchanov S, Ramirez JC, Li C, et al. A new approach for minimizing ammonia emissions from poultry houses. Water Air Soil Pollut (2008) 191(1-4):183–97. doi:10.1007/s11270-008-9616-0
20. Li G, Dong E, Ji W. H. A near-infrared trace Co2 detection system based on an 1, 580 Nm tunable diode laser using a cascaded integrator comb (cic) filter-assisted wavelength modulation technique and a digital lock-in amplifier. Front Phys (2019) 7. 9, doi:10.3389/fphy.2019.00199
21. Guo Y, Qiu X, Li N, Feng S, Cheng T, Liu Q, et al. A portable laser-based sensor for detecting H2s in domestic natural gas. Infrared Phys Tech (2020) 105:103153. doi:10.1016/j.infrared.2019.103153
22. Li G, Jiang Q, Hua C, Ma K, Jiao Y, Ji W. Temperature and pressure insensitive spectroscopic method for measuring 13ch4 during oil and natural gas drilling operations. Front Phys (2021) 9:9. doi:10.3389/fphy.2021.633012
23. Rothman LS, Gordon IE, Babikov Y, Barbe A, Chris Benner D, Bernath PF, et al. The Hitran2012 molecular spectroscopic database. J Quantitative Spectrosc Radiative Transfer (2013) 130:4–50. doi:10.1016/j.jqsrt.2013.07.002
24. Lin S, Chang J, Sun J, Xu P. Improvement of the detection sensitivity for tunable diode laser absorption spectroscopy: A review. Front Phys (2022) 10:10. doi:10.3389/fphy.2022.853966
25. Guo X, Zheng F, Li C, Yang X, Li N, Liu S, et al. A portable sensor for in-situ measurement of ammonia based on near-infrared laser absorption spectroscopy. Opt Lasers Eng (2019) 115:243–8. doi:10.1016/j.optlaseng.2018.12.005
26. Liu K, Wang L, Tan T, Wang G, Zhang W, Chen W, et al. Highly sensitive detection of methane by near-infrared laser absorption spectroscopy using a compact dense-pattern multipass cell. Sensors Actuators B: Chem (2015) 220:1000–5. doi:10.1016/j.snb.2015.05.136
27. Kong R, Sun T, Liu P, Zhou X. Optical design and analysis of a two-spherical-mirror-based multipass cell. Appl Opt (2020) 59(6):1545–52. doi:10.1364/AO.381632
28. Chang H, Feng S, Qiu X, Meng H, Guo G, He X, et al. Implementation of the toroidal absorption cell with multi-layer patterns by a single ring surface. Opt Lett (2020) 45(21):5897–900. doi:10.1364/OL.404198
29. Rothbart N, Schmalz K, Hubers H-W. A compact circular multipass cell for millimeter-wave/terahertz gas spectroscopy. IEEE Trans Terahertz Sci Technol (2020) 10(1):9–14. doi:10.1109/tthz.2019.2950123
30. Mangold M., Tuzson B., Hundt M., Jagerska J., Looser H., Emmenegger L. Circular paraboloid reflection cell for laser spectroscopic trace gas analysis. J Opt Soc Am A (2016) 33(5):913–9. doi:10.1364/JOSAA.33.000913
31. Graf M., Emmenegger L., Tuzson B. Compact, circular, and optically stable multipass cell for mobile laser absorption spectroscopy. Opt Lett (2018) 43(11):2434–7. doi:10.1364/OL.43.002434
32. Santonja G. G., Georgitzikis K., Scalet B. M., Montobbio P., Roudier S., Sancho L. D. J. E. E. Best available techniques (bat) reference document for the intensive rearing of poultry or pigs. European IPPC Bureau, (2017) 11:898.
33. Producers U. E. United Egg Producers animal husbandry guidelines for us Egg laying flocks (2006) Alpharetta, Georgia: United Egg Producers.
34. Safety O, Gov H. A. J. O. (2007). Occupational Safety and Health Administration Ammonia in workplace atmospheres—solid sorbent United States: OSHA.
35. Ti C., Xia L., Chang S. X., Yan X. Potential for mitigating global agricultural ammonia emission: A meta-analysis. Environ Pollut (2019) 245:141–8. doi:10.1016/j.envpol.2018.10.124
36. Zhang X., Li J., Shao L., Huan H., Qin F., Zhai P., et al. Effects of manure removal frequencies and deodorants on ammonia and ghg concentrations in livestock house. Atmosphere (2022) 13(7):1033. doi:10.3390/atmos13071033
37. Qi Q., Wang P.-P, Zhao J., Feng L.-L, Zhou L.-J, Xuan R.-F, et al. Sno2 nanoparticle-coated In2o3 nanofibers with improved Nh3 sensing properties. Sensors Actuators B: Chem (2014) 194:440–6. doi:10.1016/j.snb.2013.12.115
38. Liu W., Liu Y.-Y, Do J.-S, Li J. Highly sensitive room temperature ammonia gas sensor based on Ir-doped Pt porous ceramic electrodes. Appl Surf Sci (2016) 390:929–35. doi:10.1016/j.apsusc.2016.08.121
39. Lu H., Zheng C., Zhang L., Liu Z., Song F., Li X., et al. A remote sensor system based on tdlas technique for ammonia leakage monitoring. Sensors (Basel) (2021) 21(7):2448. doi:10.3390/s21072448
Keywords: tunable diode laser absorption spectroscopy, circular absorption cell, ammonia, environment, poultry housing
Citation: Wang K, Guo R, Zhou Y, Jiao L and Dong D (2022) Detection of NH3 in poultry housing based on tunable diode laser absorption spectroscopy combined with a micro circular absorption cell. Front. Phys. 10:1051719. doi: 10.3389/fphy.2022.1051719
Received: 23 September 2022; Accepted: 19 October 2022;
Published: 02 November 2022.
Edited by:
Rui Min, Beijing Normal University, ChinaReviewed by:
Diming Zhang, Zhejiang Lab, ChinaZhuo Wang, Beijing Normal University, China
Hongyi Bai, Heilongjiang University, China
Copyright © 2022 Wang, Guo, Zhou, Jiao and Dong. This is an open-access article distributed under the terms of the Creative Commons Attribution License (CC BY). The use, distribution or reproduction in other forums is permitted, provided the original author(s) and the copyright owner(s) are credited and that the original publication in this journal is cited, in accordance with accepted academic practice. No use, distribution or reproduction is permitted which does not comply with these terms.
*Correspondence: Leizi Jiao, amlhb2xlaXppQDEyNi5jb20=