- 1Department of Colorectal Surgery, Xinhua Hospital, Shanghai Jiaotong University School of Medicine, Shanghai, China
- 2Shanghai Colorectal Cancer Research Center, Shanghai, China
Introduction: Over the past few years, multiple observational studies have speculated a potential association between inflammatory bowel disease (IBD), which includes ulcerative colitis (UC) and Crohn’s disease (CD), and osteoporosis. However, no consensus has been reached regarding their interdependence and pathogenesis. Herein, we sought to further explore the causal associations between them.
Methods: We validated the association between IBD and reduced bone mineral density in humans based on genome-wide association studies (GWAS) data. To investigate the causal relationship between IBD and osteoporosis, we performed a two-sample Mendelian randomization study using training and validation sets. Genetic variation data for IBD, CD, UC, and osteoporosis were derived from published genome-wide association studies in individuals of European ancestry. After a series of robust quality control steps, we included eligible instrumental variables (SNPs) significantly associated with exposure (IBD/CD/UC). We adopted five algorithms, including MR Egger, Weighted median, Inverse variance weighted, Simple mode, and Weighted mode, to infer the causal association between IBD and osteoporosis. In addition, we evaluated the robustness of Mendelian randomization analysis by heterogeneity test, pleiotropy test, leave-one-out sensitivity test, and multivariate Mendelian randomization.
Results: Genetically predicted CD was positively associated with osteoporosis risk, with ORs of 1.060 (95% CIs 1.016, 1.106; p = 0.007) and 1.044 (95% CIs 1.002, 1.088; p = 0.039) for CD in the training and validation sets, respectively. However, Mendelian randomization analysis did not reveal a significant causal relationship between UC and osteoporosis (p > 0.05). Furthermore, we found that overall IBD was associated with osteoporosis prediction, with ORs of 1.050 (95% CIs 0.999, 1.103; p = 0.055) and 1.063 (95% CIs 1.019, 1.109; p = 0.005) in the training and validation sets, respectively.
Conclusion: We demonstrated the causal association between CD and osteoporosis, complementing the framework for genetic variants that predispose to autoimmune disease.
Introduction
With an overall increase in human lifespan, aging-related diseases are becoming increasingly prevalent each year (1, 2). Among them, osteoporosis is a common systemic skeletal disease characterized by an imbalance in bone formation and resorption (3). Currently, the gold standard for diagnosing osteoporosis is through bone mineral density (BMD) measurements of the lumbar spine, proximal femur, and distal forearm, using dual-energy X-ray bone absorptiometry (DXA) (4). It is widely believed that age, hormones (glucocorticoids, steroid hormones), nutrition (coffee, alcohol, vitamin D, calcium), and autochthonous diseases (inflammatory diseases, gastrointestinal diseases, blood disorders) are associated with reduced bone density and increased risk of osteoporosis (5). Therefore, it is essential to identify osteoporosis’s causes and associated risk factors.
Several gastrointestinal disorders have been associated with osteoporosis and bone loss, including inflammatory bowel disease, celiac disease, and chronic liver disease (6, 7). Inflammatory bowel disease (IBD) is an idiopathic disease that can be categorized into two major types: Crohn’s disease and ulcerative colitis. IBD’s pathogenesis is thought to stem from the interplay of genetic susceptibility and other risk factors (8). The incidence of osteoporosis and the associated risk of pathological fracture in patients with IBD has increased over the years, potentially caused by a loss of bone minerals due to IBD, an imbalance between osteoblasts and osteoclasts triggered by inflammatory mediators, and the administration of glucocorticoids (9–11). However, establishing a causal association between IBD and osteoporosis from an epidemiological perspective has been challenging, with factors such as gut microbiota, nutritional intake, and hormonal interventions complicating the issue (12, 13). Besides, bone densitometry is relatively rare and not commonly available in general community hospitals. Moreover, clinicians do not generally provide precise guidance on the frequency of DXA scans for IBD patients. Due to differences in study design and ethnicity, a clear causal association between IBD and osteoporosis remains ambiguous.
Mendelian randomization (MR) uses genetic variation to assess causal relationships between exposures and outcomes, similar to randomized controlled studies (14). By including genetically assigned variation obtained through meiosis as an exposure-related instrumental variable (IV), the analysis results are less affected by confounding factors. To determine whether a causal relationship exists between IBD and osteoporosis, we conducted a two-sample MR analysis using publicly accessible genome-wide association studies (GWAS) datasets.
Materials and methods
Data sources and study design
The training set was derived from the most extensive genome-wide association study (GWAS, https://gwas.mrcieu.ac.uk/), where cohorts included IBD (N = 12,882 cases, 21,770 controls; SNPs = 12,716,084), UC (N = 6,968 cases, 20,464 controls; SNPs = 12,255,197), CD (N = 5,956 cases,14,927 controls; SNPs = 12,276,506) (14). The GWAS data used for validation was from the United Kingdom IBD Genetics Consortium (UKIBDGC) and the publicly available International Inflammatory Bowel Disease Genetics Consortium (IIBDGC) pooled studies, including IBD (N = 25,042 cases, 34,915 controls; SNPs = 9,619,016), UC (N = 12,366 cases, 33,609 controls; SNPs = 9,474,559), CD (N = 12,194 cases, 28,072 controls; SNPs = 9,457,998) (15). These include the UK low coverage whole genome sequencing IBD study, the UK HumanCoreExome genotyped IBD study, and the IIBDGC genotyped IBD (including CD and UC) study. The data originates from European populations and has undergone strict quality control to eliminate potential overlap. The osteoporosis cohort included 3,203 cases and 209,575 controls, comprising 16,380,452 SNPs. Population selection was based on the International Classification of Diseases-10 (ICD-10). Currently, given the lack of a gold standard for diagnosing IBD, clinical investigators rely on several factors, including the patient’s clinical presentation, standard diagnoses like endoscopy and histopathology, and excluding infectious and non-infectious colitis. Diagnostic criteria for UC include persistent or recurrent diarrhoea, mucus stools, bloody stools with abdominal pain, and varying degrees of systemic symptoms. Endoscopic examination may reveal diffuse mucosal involvement, loss of vascular texture and mucosal roughness, and multiple vesicles or pseudo-ulcers. Pathological examination during the active phase may show diffuse inflammatory cell infiltration in the mucosal cavity, crypt abscesses, and cupulocytopenia. Diagnostic criteria for CD include similar clinical presentations and endoscopic examination may reveal discontinuous lesions with the mucosa between the lesions completely normal, as well as oval signs and varying degrees of intestinal wall thickening. Pathological examination may show focal chronic inflammation, focal structural abnormalities of the crypt, and non-caseating granulomas. To diagnose osteoporosis, a DXA examination at the femoral neck and lumbar spine is considered the gold standard. All datasets provided in GWAS have been approved by the relevant ethics committees. The data derivation process of this study is shown in Figure 1.
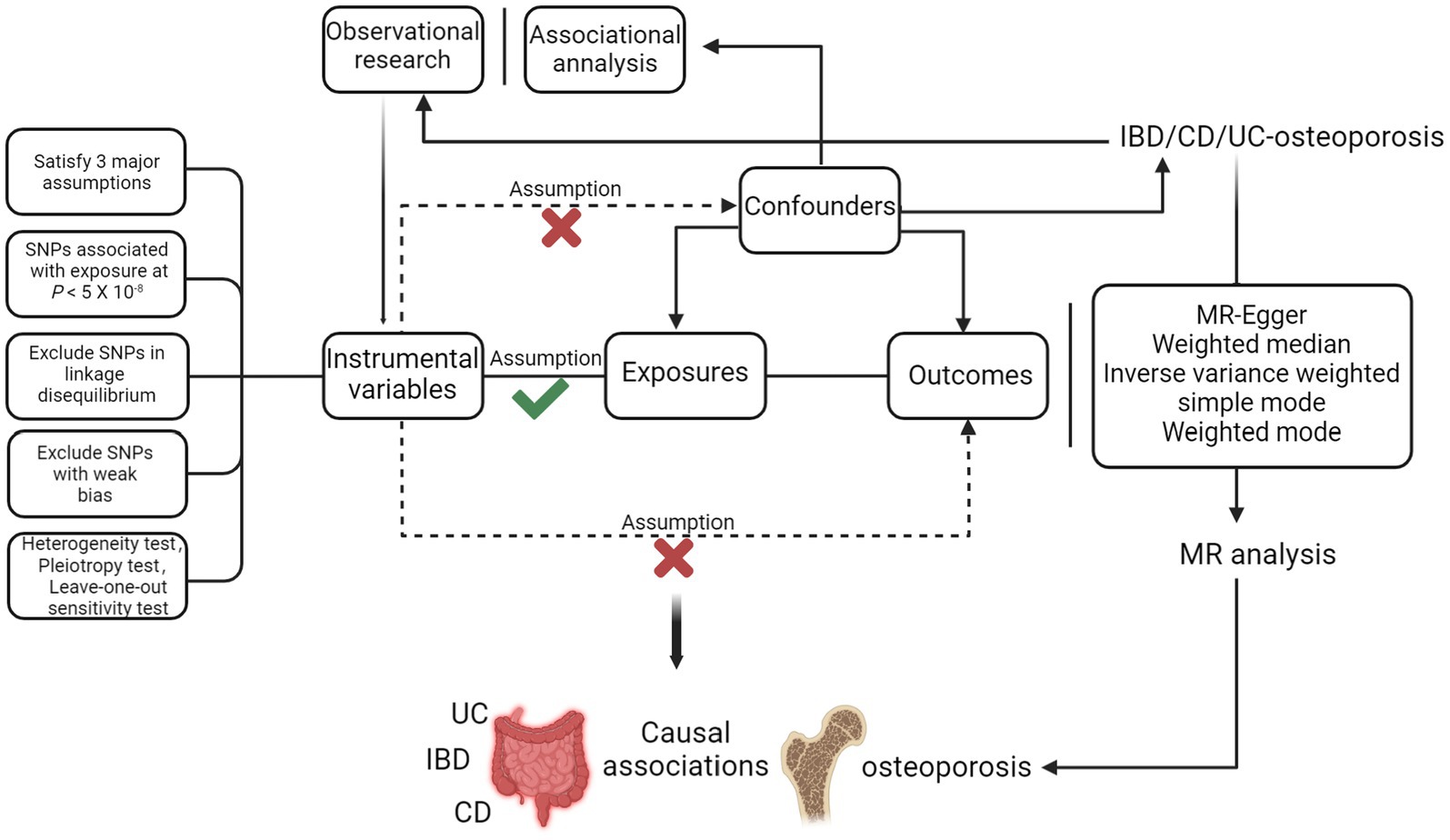
Figure 1. Procedure for an MR analysis of causal associations between IBD (including UC/CD) and osteoporosis. Checks indicate a correlation between IVs and exposure, while crosses suggest no correlation with confounders or outcomes.
Instrumental variables selection and quality control
In MR, genetic variants are used as IVs to assess the causal effect of exposure on outcomes (16). The fundamental requirements for genetic variation to meet the criteria for instrumental variables were: (1) genetic variation is associated with exposure; (2) genetic variation is not associated with confounders affecting exposure/outcome; (3) genetic variation may only be associated with outcome through exposure. Prior to MR analysis, we performed multi-step quality control of SNPs to ensure that qualified IVs were obtained. First, we ensured that all IVs were not in linkage disequilibrium to avoid bias in the results due to strong linkage disequilibrium. To effectively avoid potential linkage disequilibrium interference between SNPs, the following screening criteria were used to identify SNPs (17): (1) Significant threshold associated with IV: p < 5 × 10−8; (2) r2 = 0.001; (3) The physical distance between genes kb = 10,000.
Statistical analyses
MR analysis was based on genetic variants as IVs to estimate the causal association of exposure on outcome. We combined multiple independent data (beta coefficients and standard errors) and adopted different algorithms to assess causal associations between IBD (including UC/CD) and osteoporosis. We adopted five algorithms of MR Egger (18), Weighted median (19), Inverse variance weighted (IVW) (20), Simple mode, and Weighted mode to estimate the causal association between IBD and osteoporosis. All analyses were adjusted via the Bonferroni method (21).
Correlation test of IVs and exposure
To ensure that IVs and exposure were sufficiently correlated, we incorporated the F-score to prevent any biases from weak IVs (17). Typically, an F-score greater than 10 indicates a lack of weak IV bias. The formulas for calculating the F score and R2 are as follows. “n” represents the sample size, and “k” represents the number of SNPs included. The sample size of this study far exceeds the number of SNPs included, thus avoiding the possibility of weak IV bias.
Heterogeneity test
We used Cochran’s Q statistic to test for heterogeneity of the included IVs (22). A p-value <0.05 indicated significant heterogeneity. The heterogeneity results are shown in Table 1.
Pleiotropy test
We used the intercept term of MR Egger’s method to test whether there was horizontal pleiotropy in IVs (18, 23). A non-zero intercept term indicated the presence of horizontal pleiotropy. If the intercept term equaled 0 or was statistically insignificant, the MR Egger regression’s slope represented the estimated causal effect of exposure and outcome. In addition, we used the MR-PRESSO package (MR-PRESSO outlier test) to identify and remove any SNPs with significant differences to correct for horizontal pleiotropy (24). To avoid potential confounding, investigated possible associations of each instrument SNP with plausible confounders in the PhenoScanner GWAS database (p < 5 × 10−8). While all IVs were not associated with outcomes, residual confounding factors may still exist due to potential horizontal polymorphisms, despite our validation and culling efforts.
Leave-one-out sensitivity test
After sequentially removing each IV using the leave-one-out sensitivity test, we performed IVW MR analysis on the remaining IVs (25). To determine the existence of abnormal IVs that significantly impact the causal effect estimates, we assessed the stability of the effect estimates.
Ethics statement
Our study was based on publicly available GWAS pooled data, the included studies were approved by the institutional ethics review board, and all participants provided written informed consent.
Statistics
All analyses were performed based on R(version 4.1.2) and the TwoSampleMR package. A p-value <0.05 was considered statistically significant.
Results
The findings of our study were based on the radial IVW method, which employs adjusted second-order weights, while the other four methods are merely supplementary resources. Estimates from the MR analysis are detailed in Supplementary data.1
Two-sample MR analysis for the causal association between IBD/CD/UC and osteoporosis
We identified 62, 31, and 51 robust single nucleotide polymorphisms (SNPs) as instrumental variables for IBD, UC, and CD, respectively, after excluding pleiotropic SNPs. The percentage of horizontal pleiotropic outliers ranged from 0 to 3.1%. Low heterogeneity was observed for all diseases using the IVW method, with Cochran’s Q-derived p values of 0.403 for IBD, 0.562 for UC, and 0.487 for CD. Results from MR Egger regression showed no bias due to genetic pleiotropy, with p-values ranging from 0.422 to 0.948. The MR-PRESSO global test revealed no weak IVs bias, with F-scores larger than 10 for all diseases and p-values ranging from 0.371 to 0.539.
Therefore, these IVs were robust and feasible for assessing the causal association between IBD/CD/UC and osteoporosis. We evaluated the causal association between IBD/CD/UC and osteoporosis (mainly IVW) using five algorithms (MR Egger, Weighted median, IVW, Simple mode, and Weighted mode analysis), which yielded ORs of 1.054 (95% CIs 0.913, 1.218; p = 0.472), 1.095 (95% CIs 1.020, 1.176; p = 0.012),1.050 (95% CIs 0.999, 1.103; p = 0.055), 1.113 (95% CIs 0.944, 1.312; p = 0.208) and 1.135 (95% CIs 0.992, 1.297; p = 0.069) for IBD (Figure 2). The corresponding ORs for UC and osteoporosis were 1.100 (95% CIs 0.911, 1.328; p = 0.329), 0.990 (95% CIs 0.907, 1.080; p = 0.820),1.021 (95% CIs 0.963, 1.083; p = 0.484), 0.888 (95% CIs 0.733, 1.076; p = 0.235) and 0.910 (95% CIs 0.739, 1.119; p = 0.375). Finally, the corresponding ORs for CD and osteoporosis were 1.071 (95% CIs 0.964, 1.190; p = 0.206), 1.065 (95% CIs 1.001, 1.132; p = 0.046), 1.060 (95% CIs 1.016, 1.106; p = 0.007), 1.063 (95% CIs 0.946, 1.196; p = 0.308) and 1.085 (95% CIs 0.998, 1.180; p = 0.062), respectively. Collectively, these results illustrate that CD may increase the risk of osteoporosis from a genetic perspective.
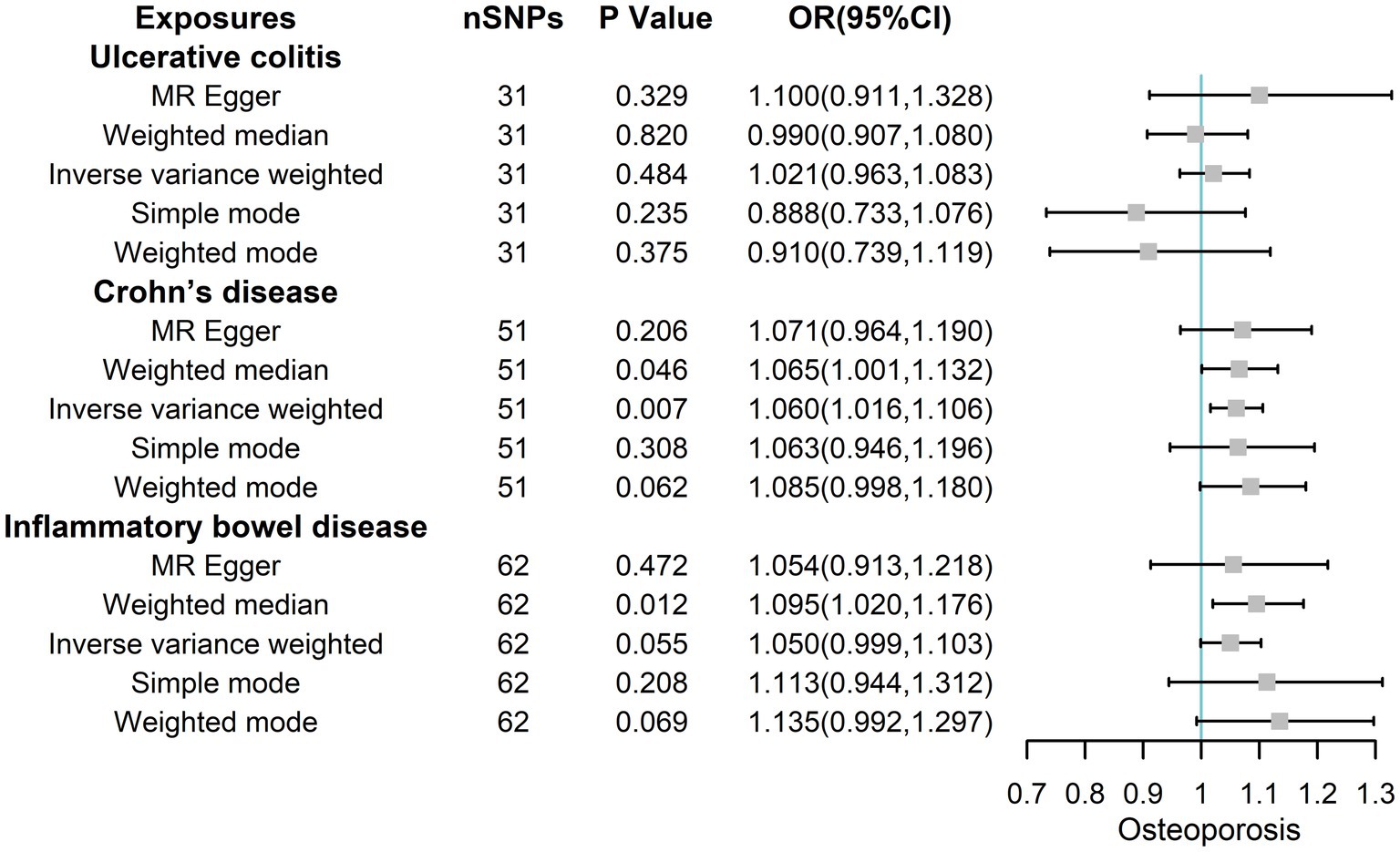
Figure 2. The risk association between IBD (including UC/CD) and osteoporosis in the training set visualized in a forest plot.
The estimated effect sizes of SNPs on IBD/UC/CD and osteoporosis are shown in scatter plots. The selected IBD/CD/UC SNP effects (X-axis) and osteoporosis SNP effects (Y-axis) were plotted, and the results showed that there was a significant positive causal effect on the total effect of IBD/CD/UC and the occurrence of osteoporosis (Figure 3). However, the single SNP IVW analysis and the leave-one-out sensitivity analysis only suggested a causal effect of CD on osteoporosis(Supplementary Figures S1, S2). Taken together, there was a positive correlation between genetically predicted CD and osteoporosis.
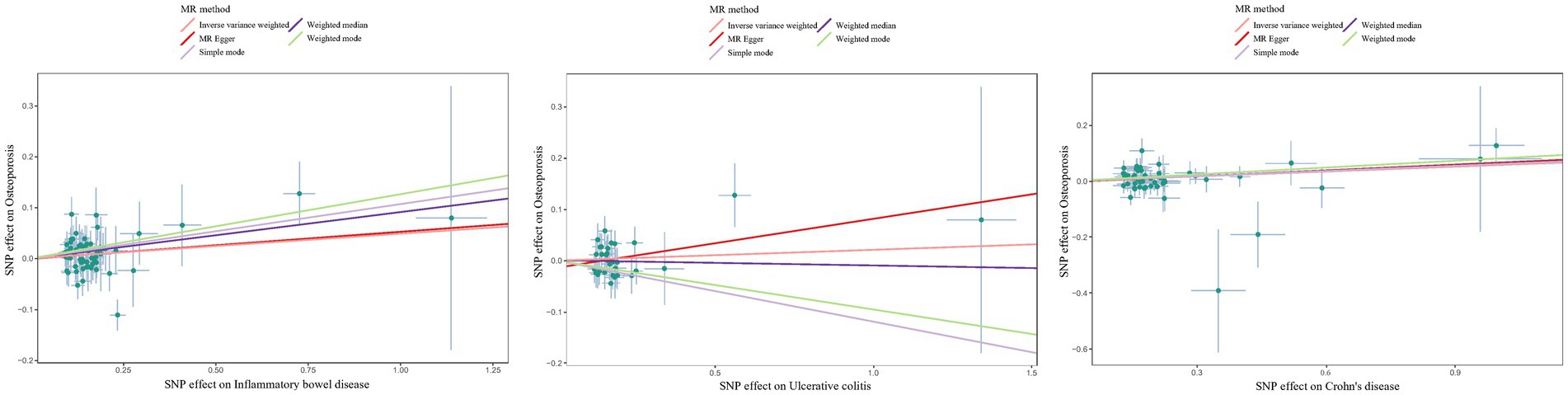
Figure 3. Scatter plots to estimate the genetic risk of osteoporosis in training set IBD (including UC/CD). SNP: Single Nucleotide Polymorphism. The slope of each line represents the effect estimation of different methods using MR.
We selected an IBD dataset with a larger sample size than the training set as the validation set. After removing pleiotropic SNPs, we obtained 102 SNPs for IBD, 48 for UC, and 80 for CD, all of which were robust instrumental variables. The percentages of horizontal polymorphic outliers for IBD/CD/UC were 10.5, 7.0, and 15.8%, respectively. The heterogeneity test by the IVW method showed that the heterogeneity among genes was low (IBD Cochran’s derived p value =0.477; UC Cochran’s Q derived p value =0.341; CD Cochran’s Q derived p value =0.690) (Table 1). The results of MR Egger regression suggested that genetic pleiotropy did not introduce any bias (IBD intercept = 0.007, p = 0.171; UC intercept = −0.027, p = 0.076; CD intercept = 0.013, p = 0.178). The MR-PRESSO global test yielded p-values of 0.458, 0.705, and 0.347 for IBD, CD, and UC, respectively, when tested against osteoporosis. Furthermore, the F-scores were well above 10, with values of 70.034, 64.576, and 80.293, indicating the absence of any weak IV bias.
MR Egger, Weighted median, IVW, Simple mode, and Weighted mode analysis were used to calculate the ORs of IBD, yielding ORs of 1.020 (95% CIs 0.948, 1.096; p = 0.603), 1.003 (95% CIs 0.931, 1.079; p = 0.947), 1.063 (95% CIs 1.019, 1.109; p = 0.005), 1.134 (95% CIs 0.954, 1.349; p = 0.157), and 1.023 (95% CIs 0.953, 1.098; p = 0.531) as shown in Figure 4. The corresponding ORs of UC with osteoporosis were 1.236 (95% CIs 1.012, 1.510; p = 0.044), 0.065 (95% CIs 0.975, 1.163; p = 0.162), 1.035 (95% CIs 0.975, 1.099; p = 0.257), 0.867 (95% CIs 0.702, 1.071; p = 0.193) and 0.885 (95% CIs 0.724, 1.083; p = 0.241). The corresponding ORs of CD with osteoporosis were 0.972 (95% CIs 0.870, 1.086; p = 0.618), 1.074(95% CIs 1.010, 1.141; p = 0.023),1.044 (95% CIs 1.002, 1.088; p = 0.039), 1.037 (95% CIs 0.908, 1.184; p = 0.594) and 1.062 (95% CIs 0.976, 1.156; p = 0.164). Overall, these results showed that both total IBD and CD could increase the risk of osteoporosis from a genetic perspective.
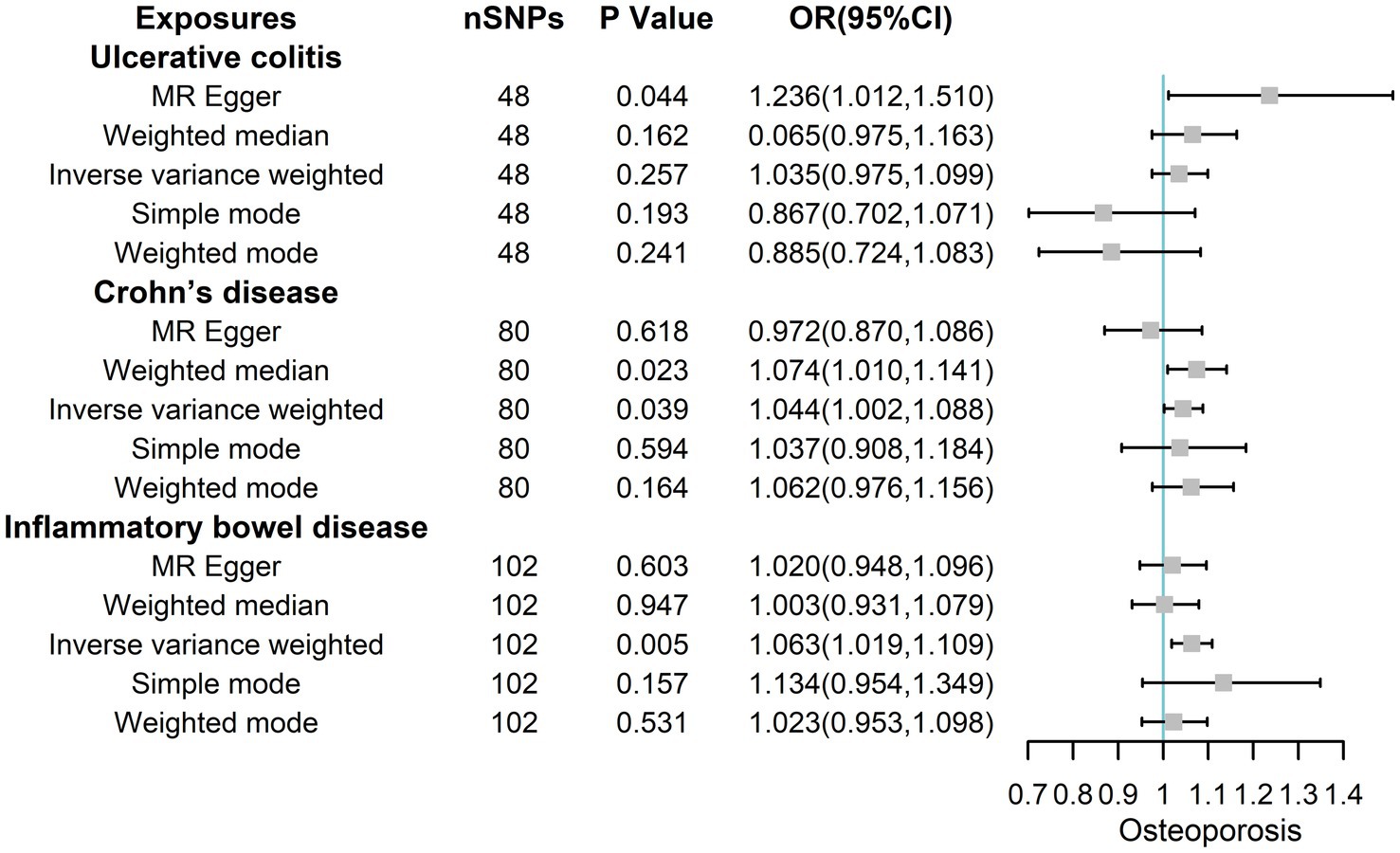
Figure 4. The risk association between IBD (including UC/CD) and osteoporosis in the validation set visualized in a forest plot.
Although the scatter plot revealed that the total effect of IBD/CD/UC yielded a significant positive causal effect on the occurrence of osteoporosis, single SNP IVW analysis and leakage sensitivity analysis suggested that only IBD and CD yielded a positive causal effect on osteoporosis(Figure 5; Supplementary Figures S3, S4).
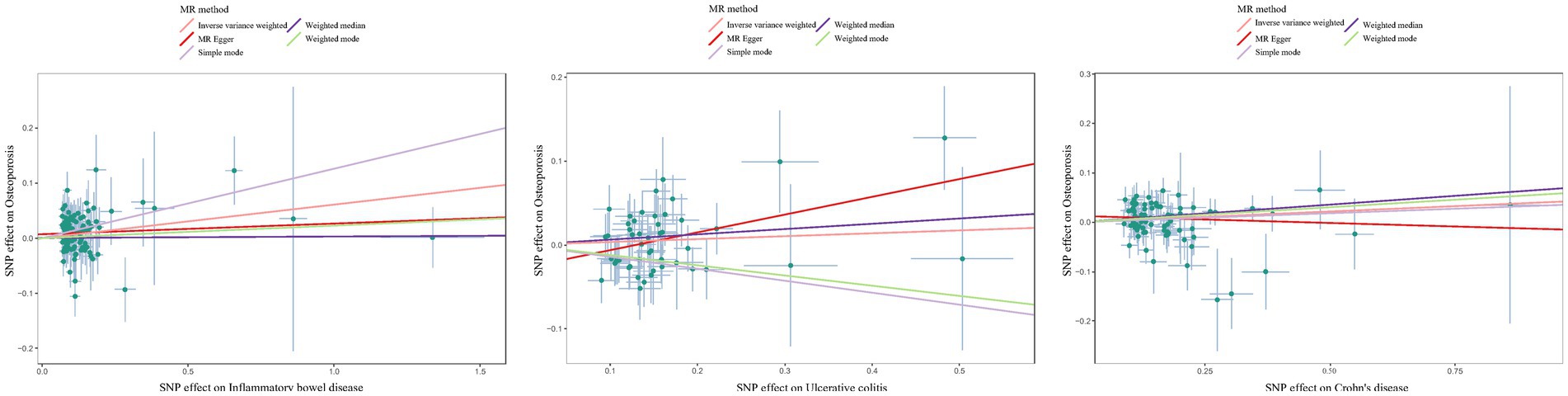
Figure 5. Scatter plot estimation of genetic risk for osteoporosis in the validation set IBD (including UC/CD). SNP: Single Nucleotide Polymorphism. The slope of each line represents the MR estimation effect of the different methods.
Multivariate MR
To eliminate confounding factors related to UC and CD, we conducted a multivariate MR of UC and CD with osteoporosis to confirm our findings (26). Specifically, we identified SNPs associated with UC and CD separately (p < 5 × 10−8) and combined all genetic variants. Following the exclusion of duplicate IVs, we observed a significant causal association between CD and osteoporosis (p = 0.004) but no causal association for UC (p = 0.177). Our results were confirmed in the validation set, with a strong causal association between CD and osteoporosis (p = 0.040) and no significant association for UC (p = 0.755).
Discussion
IBD is an immune-mediated idiopathic chronic digestive tract disease, often accompanied by various extraintestinal manifestations, such as erythema nodosum, bone and joint lesions, and ocular lesions (27). Epidemiological data suggest that individuals with IBD frequently exhibit reduced bone mineral density (with an incidence rate ranging from 30% to 80%) and are at a higher risk of developing osteoporosis (with an incidence rate of almost 50%). In severe cases, these patients may even experience fractures (28, 29).
The increased risk of fracture in patients with IBD, especially non-traumatic fractures, invariably poses a challenge to the management of this patient population. Due to the lack of a gold standard for diagnosing IBD, clinicians depend on a combination of clinical presentation, conventional diagnostic measures like endoscopy and histopathology, and eliminating other potential causes of colitis, infectious or otherwise. In cases of uncertainty regarding the diagnosis, endoscopic or histological evaluation should be conducted within a specific timeframe, typically within 6 months. DXA is widely used to determine BMD in patients because of its simplicity and non-invasiveness (30). The criteria for IBD patients eligible for DXA screening generally include postmenopausal status, ongoing or cumulative 3 months of corticosteroid therapy, history of fractures, and age over 60 years (31). However, these screening criteria are based on the bone physiology of postmenopausal Caucasian women, and their generalization to all ethnic groups still lacks adequate evidence. Additionally, IBD patients often have other risk factors for osteoporosis, and the small sample size of studies may overestimate its prevalence (29). BMD assessment can be quantified using T-score (as per WHO) and Z-score (as per ISCD) (32, 33). The T-score represents the number of standard deviations (SD) that BMD measurements deviate from peak bone mass in healthy individuals aged 30 years. Measurements within 1 SD of peak bone mass are considered normal, while measurements between 1 and 2.49 SD below peak bone mass indicate mild to moderate bone loss. Osteoporosis is defined by measurements that are more than 2.5 SD below peak bone mass (34). On the other hand, the Z-score is the number of SD that BMD measurements deviate from the mean BMD in healthy individuals of the same age group (35).
Various factors, such as daily diet, inflammation levels, low body mass index, advancing age, hypogonadism, reduced physical activity, and corticosteroid therapy, can all contribute to changes in bone structure and density in patients (36–40). When the gut is in a state of inflammation, the rate and volume of mineral loss are increased (41). An increasing body of evidence suggests that intestinal inflammatory cells such as macrophages regulate bone formation and bone resorption through the RANK/RANKL/OPG axis, further affecting bone density and increasing the possibility of osteoporosis (42–44). In a study of 122 patients with IBD, Tsironi et al. found that age (greater than 55 years) (OR 5.08, 95% CI 1.90–13.57, p = 0.001) and low body mass index were independent risk factors for osteoporosis (45). Hamdy et al. found that about 45% of patients who underwent ileectomy had osteopenia, and about 26% had osteoporosis (femoral neck and lumbar spine). Accordingly, ileal resection is a prognostic factor for osteoporosis (46). As an immunomodulator, vitamin D mediates various autoimmune diseases, including IBD (47, 48). Current evidence suggests that Vitamin D deficiency is more common in CD and UC patients than healthy individuals (49, 50). Interestingly, CD patients exhibit seasonal variations in vitamin D levels and bone turnover markers, and the long-term impact of such fluctuations on osteoporosis risk remains uncertain (51). In addition, the vitamin D receptor and its gene polymorphisms have attracted significant interest in recent years. In this regard, Bsml polymorphisms are closely related to osteoporosis (52). A survey study on the relationship between living habits and osteoporosis in Polish IBD patients found that the probability of osteoporosis in CD patients was 48.6%, while that in the UC group was 33.3%. Besides, CD patients diagnosed with osteoporosis were significantly less physically active (p = 0.0335) (29).
Moreover, the side effects of corticosteroids affect bone density and structure by inhibiting osteoblast activity and inducing apoptosis (53). There is an increasing consensus that BMD in IBD patients is correlated with the dose of corticosteroids administered (54, 55). Interestingly, some patients with IBD who received long-term steroid therapy did not experience significant bone loss. In contrast, at diagnosis, steroid-naive patients already had significantly lower lumbar spine BMD (56), suggesting that additional factors are involved in the pathophysiology of bone loss in IBD. As a result, BMD serves only as one of the risk factors for osteoporosis or fracture and may not always provide a reliable correlation measure. It is well-established that gene regulation plays a significant role in the heightened susceptibility to osteoporosis. At the level of gene transcription, low-density lipoprotein receptor 5 (LRP5) is involved in the transcription of human bone tissue and is thought to enhance bone resorption (57). When LRP5 undergoes deleterious mutations or loss of function, bone tissue develops phenotypic and functional deficits (58). Interestingly, osteoporotic pseudoglioma syndrome is a disease caused by loss-of-function mutations in the LRP5 genome, further supporting the critical role of this gene in bone integrity and function (59).
A prospective study across multiple countries suggested that about 12%–22% of IBD patients had asymptomatic vertebral fractures, including 19.6% (44/224) in Canada, 21.8% (34/156) in Germany, and 12.2% (22/179) in Israel (60–62). It remains unclear whether the reduction in BMD among CD patients is correlated with the occurrence of fractures or osteoporosis. In addition, the decrease in BMD in IBD patients often has no obvious phenotype, and the direct causal association with osteoporosis and fractures is unclear (63). D. Leslie et al. found that IBD only slightly increased the risk of BMD and osteoporosis (64). Univariate and multivariate analysis of CD patients by C. Noble et al. showed that low body mass index (BMI < 18.5) was associated with osteoporosis (p = 0.021, OR 5.83, CI 1.31–25.94) (65). Silva and colleagues conducted a study with 444 participants (case: control = 1:3) in Sri Lanka to investigate whether IBD is a risk factor for osteoporosis in adults. The team performed DXA bone density scans and found that while the overall incidence of osteoporosis was higher in the IBD group compared to the control group (13.5% vs. 4.5%, p = 0.001), there was no significant difference between UC and CD (14.45% vs. 10.7%, p > 0.05) (66). A prospective study on osteoporosis screening strategies in IBD patients found that nearly 66% of patients receiving steroids (>500 mg) were at risk of developing osteoporosis, including 77% for CD and 58% for UC (p < 0.001) (7). Therefore, the incidence of osteoporosis in IBD patients is also subject to confounding factors.
Previous research indicates that the prevalence of osteoporosis (as measured by T-score) in CD and UC is not significantly different (67, 68). However, after controlling for multiple confounding factors (age, hormone therapy, etc.), it was found that CD patients had an increased risk of osteoporosis by about 50% (T < −2.5), but this phenomenon was not observed in UC patients (68). Given that the mean reduction at each measurement point was less than 10%, the study could not assess the overall risk association between IBD and osteoporosis. Additionally, multiple studies acknowledge that CD activity has a more significant effect on BMD and bone physiology than UC. Haschka et al. suggested that, unlike UC, which has an acute onset and is limited to the distal colon, CD patients have a prolonged and systemic disease course and exhibit a more severe bone loss phenotype (69). In addition, CD is characterized by pathological changes in the small intestine, and inflammation or surgical resection of this area can lead to nutrient malabsorption and estrogen deficiency (70). However, Schoon et al. did not observe differences in BMD between CD and UC patients, and BMD was not significantly lower in newly diagnosed IBD patients (67).
Observational studies have indicated a possible direct causal link between IBD and a high prevalence of BMD loss and osteoporosis. However, there is currently limited emphasis placed on screening for osteoporosis in IBD patients. Additionally, due to the low absolute risk of fractures, there is considerable debate over whether all IBD patients should be screened. Therefore, excluding the interference of confounding factors, exploring whether there is a clear causal association between IBD and osteoporosis will benefit the preventive screening and specific treatment of osteoporosis. Genetic markers may assist in screening or identifying potentially high-risk patients for osteoporosis, and MR analysis based on GWAS can be used for causal analysis of IBD and osteoporosis.
Over the past few years, several variants involved in IBD/UC/CD development or immune-related biological processes have been reported, refining our understanding of the underlying pathological mechanisms. Among these, IL-1 and IL-18 are crucial in the inflammatory response and in maintaining intestinal mucosal stability. Nonetheless, their associations with the pathogenesis of IBD are not yet clearly understood. A recent study by Mi et al. revealed a positive causal effect of IL-18 on both IBD/CD/UC with odds ratios of 1.240, 1.199, and 1.274, respectively, in a three-sample MR study. In contrast, the genetically predicted IL-1 receptor antagonist (IL-1Ra) was negatively associated with the risk of IBD/CD/UC with odds ratios of 0.915, 0.902, and 0.899, respectively (71). This study suggests that genetically predicted IL-18 and IL-1Ra correlate with the risk of IBD, increasing the potential for drug development from a genetic perspective. Richards et al. coincidentally discovered three SNPs (rs17229943 (OCLN), rs385076 (NLRC4), and rs71478720 (IL-18)) that exhibited genome-wide significance for IL-18 levels using MR analysis (72). These three SNPs explained 6.8% of the variance in IL-18 levels, and the standard deviation of IL-18 under genetic prediction was positively associated with IBD susceptibility (OR = 1.22, 95% CI = 1.11–1.34, p = 6 × 10−5) (72). rs71478720 is located in the IL-18 intron and affects the expression of IL-18 in tissues, including the lung, thyroid, and skeletal muscle (73). rs385076 is an intron variant that maps to the NLRC4 locus and whose gene product, NLRC4 inflammatory vesicles, responds primarily to bacterial invasion (74). Activation of NLRC4 often induces the production of IL-1β and IL-18 and is also involved in cellular scorching (75). Its missense activating mutations often cause activation of auto-inflammatory disease, but the symptoms are distinctly tissue-specific (76). Besides, rs17229943 is an intron variant near the OCLN gene, primarily involved in encoding a membrane closure protein located at a tight junction (77). Buret et al. found that IL-18 could increase the inflammatory response to IBD by promoting neutrophil migration across the epithelium by selectively breaking the loop of tight junction closure proteins (78). It has been established that rs6062504 (related to TNFRSF6B) acts primarily as a mediator of the intestinal anti-inflammatory response to TNF-α (78). In addition, rs2488389 (associated with DENND1B) is expressed mainly in dendritic cells and natural killer cells and is involved in the synthesis of TNF receptor 1 (79). Moreover, rs2066845 (related to NOD2) is mainly involved in mucosal immunity and microbiota crosstalk (80, 81). Finally, it has been reported that rs11741861 (associated with IRGM) is primarily involved in intestinal mucosal autophagy and cytokine expression. The deletion of its upstream 20 kb copy number variant has been associated with increased or decreased IRGM in a cell lineage-dependent manner and is considered to be a possible causal variant (82).
Herein, we identified a causal association between CD and osteoporosis based on GWAS, enriching the existing framework for genetic variants that predispose to autoimmune disease. The strength of our study is its ability to overcome potential confounding factors present in observational studies, such as clinical heterogeneity arising from disease duration, sample quality, microbiota composition, clinical interventions, and diagnostic criteria. The large sample size ensures greater consistency in the obtained results, making it feasible to evaluate causal effects. However, the generalizability and applicability of the study’s findings to other populations are limited due to the exclusive use of a European sample base.
At present, multiple factors limit the widespread use of MR. Firstly, establishing a reliable genotype-intermediate phenotype-disease association can be challenging in the presence of ethnic or population diversity, which increases the risk of confounding among the three factors. Secondly, while GWAS has enhanced MR application, identifying suitable genetic variants as instrumental variables has become more intricate. Additionally, pleiotropy can introduce bias into MR results, especially if a genetic variant affects multiple phenotypic traits or has multiple biological effects (73). Thirdly, the inclusion of GWAS data from a larger population of middle-aged and older adults in MR studies may lead to amplification or splitting of gene effects due to cumulative environmental influences and interactions, potentially violating the MR assumption (71, 83). Excluding the potential impact of developmental adaptation during the interpretation of MR study results may pose a challenge (72). Finally, genetic variation may only explain a small proportion of exposures or traits in most cases (84).
Therefore, a large sample size is essential to obtain precise risk estimates when applying MR. Additionally, regression MR allows for a simplified assessment of the impact of exposure on outcomes through linear or logistic regression and facilitates the evaluation of effect sizes (85). Establishing a null outcome sample set is crucial for MR, as it links genetic variation and exposure rather than outcome, indicating that exposure does not affect the outcome. In the future, MR studies will aim to predict new biomarkers of disease onset (exposure) and screen for possible therapeutic targets by intersecting multi-omics data. The development of new algorithms represents a future direction to enhance the practicality of MR and minimize the aforementioned inherent limitations.
Conclusion
The use of MR studies not only sheds new light on the causal relationship between inflammatory bowel disease and osteoporosis but also aids in generating new hypotheses regarding the development of osteoporosis. This progress lays the groundwork for future investment in validation intervention trials, exploration of novel therapeutic targets, and guidance for drug design. Although MR has limitations, conducting randomized investigations of biological mechanisms with the necessary genetic data, exposure, and outcome variables can better allocate resources and facilitate clinical trials. In summary, the extension of MR study methods minimizes the influence of confounding factors, strengthens epigenetic causal inference, and serves as a valuable reference for multidisciplinary disease treatment.
Data availability statement
The original contributions presented in the study are included in the article/Supplementary material, further inquiries can be directed to the corresponding authors.
Ethics statement
All datasets provided in GWAS have been approved by the relevant ethics committees.
Author contributions
ZD, WX, and RD completed the construction of the model and the writing of the article. XP, XS, JS, and PD complete quality control, data analysis, and visualization. ZW and YL completes model guidance, critical review, and funding support. All authors contributed to the article and approved the submitted version.
Funding
This work was supported by the National Natural Science Foundation of China (Nos. 82270549, 82000481, 82002507, and 82073056), the Shanghai Sailing Program (No. 20YF1429400), the Qingfeng Scientific Research Fund of the China Crohn’s & Colitis Foundation (CCCF) (No. CCCF-QF-2022C14-21), and Shanghai Pujiang Talent Program (19PJ1407600 and 20YF1430100).
Acknowledgments
The authors thank all investigators for making available their GWAS data. The authors also acknowledge the participants and investigators of the FinnGen study. The authors thank Home for Researchers editorial team (www.home-for-researchers.com) for their language editing service.
Conflict of interest
The authors declare that the research was conducted in the absence of any commercial or financial relationships that could be construed as a potential conflict of interest.
Publisher’s note
All claims expressed in this article are solely those of the authors and do not necessarily represent those of their affiliated organizations, or those of the publisher, the editors and the reviewers. Any product that may be evaluated in this article, or claim that may be made by its manufacturer, is not guaranteed or endorsed by the publisher.
Supplementary material
The Supplementary material for this article can be found online at: https://www.frontiersin.org/articles/10.3389/fpubh.2023.1151837/full#supplementary-material
Abbreviations
BMD, bone mineral density; CD, Crohn’s disease; DXA, dual-energy X-ray bone absorptiometry; GWAS, genome-wide association studies; IBD, inflammatory bowel disease; ICD-10, International Classification of Diseases-10; IIBDGC, International Inflammatory Bowel Disease Genetics Consortium; IVW, Inverse variance weighted; LRP5, lipoprotein receptor 5; MR, Mendelian randomization; SD, standard deviations; UC, ulcerative colitis; UKIBDGC, United Kingdom IBD Genetics Consortium.
Footnotes
References
1. Levy, G, and Levin, B. An evolution-based model of causation for aging-related diseases and intrinsic mortality: explanatory properties and implications for healthy aging. Front Public Health. (2022) 10:774668. doi: 10.3389/fpubh.2022.774668
2. Schrempft, S, Belsky, DW, Draganski, B, Kliegel, M, Vollenweider, P, Marques-Vidal, P, et al. Associations between life course socioeconomic conditions and the pace of aging. J Gerontol A Biol Sci Med Sci. (2021) 77:2257–64. doi: 10.1093/gerona/glab383
3. Raterman, HG, Bultink, IE, and Lems, WF. Osteoporosis in patients with rheumatoid arthritis: an update in epidemiology, pathogenesis, and fracture prevention. Expert Opin Pharmacother. (2020) 21:1725–37. doi: 10.1080/14656566.2020.1787381
4. Wang, L, Ran, L, Zha, X, Zhao, K, Yang, Y, Shuang, Q, et al. Adjustment of DXA BMD measurements for anthropometric factors and its impact on the diagnosis of osteoporosis. Arch Osteoporos. (2020) 15:155. doi: 10.1007/s11657-020-00833-1
5. Brommage, R, and Ohlsson, C. Translational studies provide insights for the etiology and treatment of cortical bone osteoporosis. Best Pract Res Clin Endocrinol Metab. (2018) 32:329–40. doi: 10.1016/j.beem.2018.02.006
6. Yang, YJ, and Kim, DJ. An overview of the molecular mechanisms contributing to musculoskeletal disorders in chronic liver disease: osteoporosis, sarcopenia, and osteoporotic sarcopenia. Int J Mol Sci. (2021) 22. doi: 10.3390/ijms22052604
7. Lo, B, Holm, JP, Vester-Andersen, MK, Bendtsen, F, Vind, I, and Burisch, J. Incidence, risk factors and evaluation of osteoporosis in patients with inflammatory bowel disease: a Danish population-based inception cohort with 10 years of follow-up. J Crohns Colitis. (2020) 14:904–14. doi: 10.1093/ecco-jcc/jjaa019
8. Jakobsen, C, Cleynen, I, Andersen, PS, Vermeire, S, Munkholm, P, Paerregaard, A, et al. Genetic susceptibility and genotype-phenotype association in 588 Danish children with inflammatory bowel disease. J Crohns Colitis. (2014) 8:678–85. doi: 10.1016/j.crohns.2013.12.010
9. Adriani, A, Pantaleoni, S, Luchino, M, Ribaldone, DG, Reggiani, S, Sapone, N, et al. Osteopenia and osteoporosis in patients with new diagnosis of inflammatory bowel disease. Panminerva Med. (2014) 56:145–9.
10. Summary for patients: fracture risk during osteoporosis drug holidays. Ann Intern Med. (2022) 175:I23. doi: 10.7326/P21-0013
11. Jiang, Y, Lu, Y, Jiang, X, Hu, J, Li, R, Liu, Y, et al. Glucocorticoids induce osteoporosis mediated by glucocorticoid receptor-dependent and -independent pathways. Biomed Pharmacother. (2020) 125:109979. doi: 10.1016/j.biopha.2020.109979
12. Wang, N, Ma, S, and Fu, L. Gut microbiota dysbiosis as one cause of osteoporosis by impairing intestinal barrier function. Calcif Tissue Int. (2022) 110:225–35. doi: 10.1007/s00223-021-00911-7
13. Papadopoulou, SK, Papadimitriou, K, Voulgaridou, G, Georgaki, E, Tsotidou, E, Zantidou, O, et al. Exercise and nutrition impact on osteoporosis and sarcopenia-the incidence of osteosarcopenia: a narrative review. Nutrients. (2021) 13. doi: 10.3390/nu13124499
14. Liu, JZ, van Sommeren, S, Huang, H, Ng, SC, Alberts, R, Takahashi, A, et al. Association analyses identify 38 susceptibility loci for inflammatory bowel disease and highlight shared genetic risk across populations. Nat Genet. (2015) 47:979–86. doi: 10.1038/ng.3359
15. de Lange, KM, Moutsianas, L, Lee, JC, Lamb, CA, Luo, Y, Kennedy, NA, et al. Genome-wide association study implicates immune activation of multiple integrin genes in inflammatory bowel disease. Nat Genet. (2017) 49:256–61. doi: 10.1038/ng.3760
16. Pierce, BL, and Burgess, S. Efficient design for Mendelian randomization studies: subsample and 2-sample instrumental variable estimators. Am J Epidemiol. (2013) 178:1177–84. doi: 10.1093/aje/kwt084
17. Pritchard, JK, and Przeworski, M. Linkage disequilibrium in humans: models and data. Am J Hum Genet. (2001) 69:1–14. doi: 10.1086/321275
18. Bowden, J, Del Greco, MF, Minelli, C, Davey Smith, G, Sheehan, NA, and Thompson, JR. Assessing the suitability of summary data for two-sample Mendelian randomization analyses using MR-egger regression: the role of the I2 statistic. Int J Epidemiol. (2016) 45:1961–74. doi: 10.1093/ije/dyw220
19. Bowden, J, Davey Smith, G, Haycock, PC, and Burgess, S. Consistent estimation in Mendelian randomization with some invalid instruments using a weighted median estimator. Genet Epidemiol. (2016) 40:304–14. doi: 10.1002/gepi.21965
20. Lee, CH, Cook, S, Lee, JS, and Han, B. Comparison of two meta-analysis methods: inverse-variance-weighted average and weighted sum of Z-scores. Genom Inform. (2016) 14:173–80. doi: 10.5808/GI.2016.14.4.173
21. Ranstam, J. Multiple P-values and bonferroni correction. Osteoarthr Cartil. (2016) 24:763–4. doi: 10.1016/j.joca.2016.01.008
22. Kulinskaya, E, and Dollinger, MB. An accurate test for homogeneity of odds ratios based on Cochran's Q-statistic. BMC Med Res Methodol. (2015) 15:49. doi: 10.1186/s12874-015-0034-x
23. Verbanck, M, Chen, CY, Neale, B, and Do, R. Detection of widespread horizontal pleiotropy in causal relationships inferred from Mendelian randomization between complex traits and diseases. Nat Genet. (2018) 50:693–8. doi: 10.1038/s41588-018-0099-7
24. Ong, JS, and MacGregor, S. Implementing MR-PRESSO and GCTA-GSMR for pleiotropy assessment in Mendelian randomization studies from a practitioner's perspective. Genet Epidemiol. (2019) 43:609–16. doi: 10.1002/gepi.22207
25. Cheng, H, Garrick, DJ, and Fernando, RL. Efficient strategies for leave-one-out cross validation for genomic best linear unbiased prediction. J Anim Sci Biotechnol. (2017) 8:38. doi: 10.1186/s40104-017-0164-6
26. Xu, F, Zhang, Q, Wang, LK, Tang, QX, Sun, CQ, and Deng, HW. Estimates of the effects of physical activity on osteoporosis using multivariable Mendelian randomization analysis. Osteoporos Int. (2021) 32:1359–67. doi: 10.1007/s00198-020-05786-2
27. Rogler, G, Singh, A, Kavanaugh, A, and Rubin, DT. Extraintestinal manifestations of inflammatory bowel disease: current concepts, treatment, and implications for disease management. Gastroenterology. (2021) 161:1118–32. doi: 10.1053/j.gastro.2021.07.042
28. Even Dar, R, Mazor, Y, Karban, A, Ish-Shalom, S, and Segal, E. Risk factors for low bone density in inflammatory bowel disease: use of glucocorticoids, low body mass index, and smoking. Dig Dis. (2019) 37:284–90. doi: 10.1159/000496935
29. Rychter, AM, Ratajczak, AE, Szymczak-Tomczak, A, Michalak, M, Eder, P, Dobrowolska, A, et al. Associations of lifestyle factors with osteopenia and osteoporosis in polish patients with inflammatory bowel disease. Nutrients. (2021) 13. doi: 10.3390/nu13061863
30. Yen, CC, Lin, WC, Wang, TH, Chen, GF, Chou, DY, Lin, DM, et al. Pre-screening for osteoporosis with calcaneus quantitative ultrasound and dual-energy X-ray absorptiometry bone density. Sci Rep. (2021) 11:15709. doi: 10.1038/s41598-021-95261-7
31. Bak-Drabik, K, Adamczyk, P, Chobot, A, and Pluskiewicz, W. Skeletal status assessed by quantitative ultrasound and dual-energy X-ray absorptiometry in children with inflammatory bowel disease: a 2-year prospective study. Clin Res Hepatol Gastroenterol. (2020) 44:768–77. doi: 10.1016/j.clinre.2019.09.004
32. Johansson, H, Azizieh, F, Al Ali, N, Alessa, T, Harvey, NC, McCloskey, E, et al. T-score-based intervention thresholds for osteoporosis. Osteoporos Int. (2017) 28:3099–105. doi: 10.1007/s00198-017-4160-7
33. Yung, CK, Fook-Chong, S, and Chandran, M. The prevalence of recognized contributors to secondary osteoporosis in south east Asian men and post-menopausal women. Are Z score diagnostic thresholds useful predictors of their presence? Arch Osteoporos. (2012) 7:49–56. doi: 10.1007/s11657-012-0078-z
34. Dimai, HP. Use of dual-energy X-ray absorptiometry (DXA) for diagnosis and fracture risk assessment; WHO-criteria, T- and Z-score, and reference databases. Bone. (2017) 104:39–43. doi: 10.1016/j.bone.2016.12.016
35. McKiernan, FE, Berg, RL, and Linneman, JG. The utility of BMD Z-score diagnostic thresholds for secondary causes of osteoporosis. Osteoporos Int. (2011) 22:1069–77. doi: 10.1007/s00198-010-1307-1
36. Leslie, WD, Metge, C, and Ward, L. Contribution of clinical risk factors to bone density-based absolute fracture risk assessment in postmenopausal women. Osteoporos Int. (2003) 14:334–8. doi: 10.1007/s00198-003-1375-6
37. Melton, LJ 3rd, Crowson, CS, O'Fallon, WM, Wahner, HW, and Riggs, BL. Relative contributions of bone density, bone turnover, and clinical risk factors to long-term fracture prediction. J Bone Miner Res. (2003) 18:312–8. doi: 10.1359/jbmr.2003.18.2.312
38. Lucas, JA, Lucas, PR, Vogel, S, Gamble, GD, Evans, MC, and Reid, IR. Effect of sub-elite competitive running on bone density, body composition and sexual maturity of adolescent females. Osteoporos Int. (2003) 14:848–56. doi: 10.1007/s00198-003-1455-7
39. Chandran, M, Hoeck, HC, Wong, HC, Zhang, RF, and Dimai, HP. Vitamin D status and its relationship with bone mineral density and parathyroid hormone in southeast Asian adults with low bone density. Endocr Pract. (2011) 17:226–34. doi: 10.4158/EP10202.OR
40. Fuggle, NR, Westbury, LD, Syddall, HE, Duggal, NA, Shaw, SC, Maslin, K, et al. Relationships between markers of inflammation and bone density: findings from the Hertfordshire cohort study. Osteoporos Int. (2018) 29:1581–9. doi: 10.1007/s00198-018-4503-z
41. Du, YN, Chen, YJ, Zhang, HY, Wang, X, and Zhang, ZF. Inverse association between systemic immune-inflammation index and bone mineral density in postmenopausal women. Gynecol Endocrinol. (2021) 37:650–4. doi: 10.1080/09513590.2021.1885642
42. Ko, Y, Lee, G, Kim, B, Park, M, Jang, Y, and Lim, W. Modification of the RANKL-RANK-binding site for the immunotherapeutic treatment of osteoporosis. Osteoporos Int. (2020) 31:983–93. doi: 10.1007/s00198-019-05200-6
43. Zheng, H, Wang, C, He, JW, Fu, WZ, and Zhang, ZL. OPG, RANKL, and RANK gene polymorphisms and the bone mineral density response to alendronate therapy in postmenopausal Chinese women with osteoporosis or osteopenia. Pharmacogenet Genomics. (2016) 26:12–9. doi: 10.1097/FPC.0000000000000181
44. Wolski, H, Drews, K, Bogacz, A, Kaminski, A, Barlik, M, Bartkowiak-Wieczorek, J, et al. The RANKL/RANK/OPG signal trail: significance of genetic polymorphisms in the etiology of postmenopausal osteoporosis. Ginekol Pol. (2016) 87:347–52. doi: 10.5603/GP.2016.0014
45. Tsironi, E, Hadjidakis, D, Mallas, E, Tzathas, C, Karamanolis, DG, and Ladas, SD. Comparison of T- and Z-score in identifying risk factors of osteoporosis in inflammatory bowel disease patients. J Musculoskelet Neuronal Interact. (2008) 8:79–84.
46. van Hogezand, RA, Banffer, D, Zwinderman, AH, McCloskey, EV, Griffioen, G, and Hamdy, NA. Ileum resection is the most predictive factor for osteoporosis in patients with Crohn's disease. Osteoporos Int. (2006) 17:535–42. doi: 10.1007/s00198-005-0016-7
47. Murdaca, G, Tonacci, A, Negrini, S, Greco, M, Borro, M, Puppo, F, et al. Emerging role of vitamin D in autoimmune diseases: an update on evidence and therapeutic implications. Autoimmun Rev. (2019) 18:102350. doi: 10.1016/j.autrev.2019.102350
48. Triantos, C, Aggeletopoulou, I, Mantzaris, GJ, and Mouzaki, A. Molecular basis of vitamin D action in inflammatory bowel disease. Autoimmun Rev. (2022) 21:103136. doi: 10.1016/j.autrev.2022.103136
49. Limketkai, BN, Singla, MB, Rodriguez, B, Veerappan, GR, Betteridge, JD, Ramos, MA, et al. Levels of vitamin D are low after Crohn's disease is established but not before. Clin Gastroenterol Hepatol. (2020) 18:1769–1776.e1. doi: 10.1016/j.cgh.2019.09.034
50. Rizvi, A, Trivedi, P, Bar-Mashiah, A, Plietz, M, Khaitov, S, Sylla, P, et al. Vitamin D deficiency is common in patients with ulcerative colitis after total proctocolectomy with Ileal pouch anal anastomosis. Inflamm Bowel Dis. (2022) 28:1924–6. doi: 10.1093/ibd/izac093
51. McCarthy, D, Duggan, P, O'Brien, M, Kiely, M, McCarthy, J, Shanahan, F, et al. Seasonality of vitamin D status and bone turnover in patients with Crohn's disease. Aliment Pharmacol Ther. (2005) 21:1073–83. doi: 10.1111/j.1365-2036.2005.02446.x
52. Liao, JL, Qin, Q, Zhou, YS, Ma, RP, Zhou, HC, Gu, MR, et al. Vitamin D receptor Bsm I polymorphism and osteoporosis risk in postmenopausal women: a meta-analysis from 42 studies. Genes Nutr. (2020) 15:20. doi: 10.1186/s12263-020-00679-9
53. Egeberg, A, Schwarz, P, Harslof, T, Andersen, YMF, Pottegard, A, Hallas, J, et al. Association of potent and very potent topical corticosteroids and the risk of osteoporosis and major osteoporotic fractures. JAMA Dermatol. (2021) 157:275–82. doi: 10.1001/jamadermatol.2020.4968
54. Jackson, RD. Topical corticosteroids and glucocorticoid-induced osteoporosis-cumulative dose and duration matter. JAMA Dermatol. (2021) 157:269–70. doi: 10.1001/jamadermatol.2020.4967
55. Chedid, VG, and Kane, SV. Bone health in patients with inflammatory bowel diseases. J Clin Densitom. (2020) 23:182–9. doi: 10.1016/j.jocd.2019.07.009
56. Sakellariou, GT, Moschos, J, Berberidis, C, Mpoumponaris, A, Kadis, S, Molyvas, E, et al. Bone density in young males with recently diagnosed inflammatory bowel disease. Joint Bone Spine. (2006) 73:725–8. doi: 10.1016/j.jbspin.2006.01.017
57. Abdel-Hamid, MS, Elhossini, RM, Otaify, GA, Abdel-Ghafar, SF, and Aglan, MS. Osteoporosis-pseudoglioma syndrome in four new patients: identification of two novel LRP5 variants and insights on patients' management using bisphosphonates therapy. Osteoporos Int. (2022) 33:1501–10. doi: 10.1007/s00198-022-06313-1
58. Rocca, MS, Minervini, G, Di Nisio, A, Merico, M, Bueno Marinas, M, De Toni, L, et al. Identification of rare LRP5 variants in a cohort of males with impaired bone mass. Int J Mol Sci. (2021) 22. doi: 10.3390/ijms221910834
59. Pekkinen, M, Grigelioniene, G, Akin, L, Shah, K, Karaer, K, Kurtoglu, S, et al. Novel mutations in the LRP5 gene in patients with osteoporosis-pseudoglioma syndrome. Am J Med Genet A. (2017) 173:3132–5. doi: 10.1002/ajmg.a.38491
60. Siffledeen, JS, Siminoski, K, Jen, H, and Fedorak, RN. Vertebral fractures and role of low bone mineral density in Crohn's disease. Clin Gastroenterol Hepatol. (2007) 5:721–8. doi: 10.1016/j.cgh.2007.02.024
61. Stockbrugger, RW, Schoon, EJ, Bollani, S, Mills, PR, Israeli, E, Landgraf, L, et al. Discordance between the degree of osteopenia and the prevalence of spontaneous vertebral fractures in Crohn's disease. Aliment Pharmacol Ther. (2002) 16:1519–27. doi: 10.1046/j.1365-2036.2002.01317.x
62. Klaus, J, Armbrecht, G, Steinkamp, M, Bruckel, J, Rieber, A, Adler, G, et al. High prevalence of osteoporotic vertebral fractures in patients with Crohn's disease. Gut. (2002) 51:654–8. doi: 10.1136/gut.51.5.654
63. Etzel, JP, Larson, MF, Anawalt, BD, Collins, J, and Dominitz, JA. Assessment and management of low bone density in inflammatory bowel disease and performance of professional society guidelines. Inflamm Bowel Dis. (2011) 17:2122–9. doi: 10.1002/ibd.21601
64. Bernstein, CN, and Leslie, WD. Review article: osteoporosis and inflammatory bowel disease. Aliment Pharmacol Ther. (2004) 19:941–52. doi: 10.1111/j.1365-2036.2004.01876.x
65. Noble, CL, McCullough, J, Ho, W, Lees, CW, Nimmo, E, Drummond, H, et al. Low body mass not vitamin D receptor polymorphisms predict osteoporosis in patients with inflammatory bowel disease. Aliment Pharmacol Ther. (2008) 27:588–96. doi: 10.1111/j.1365-2036.2008.03599.x
66. de Silva, AP, Karunanayake, AL, Dissanayaka, TG, Dassanayake, AS, Duminda, HK, Pathmeswaran, A, et al. Osteoporosis in adult Sri Lankan inflammatory bowel disease patients. World J Gastroenterol. (2009) 15:3528–31. doi: 10.3748/wjg.15.3528
67. Schoon, EJ, Blok, BM, Geerling, BJ, Russel, MG, Stockbrugger, RW, and Brummer, RJ. Bone mineral density in patients with recently diagnosed inflammatory bowel disease. Gastroenterology. (2000) 119:1203–8. doi: 10.1053/gast.2000.19280
68. Targownik, LE, Bernstein, CN, Nugent, Z, and Leslie, WD. Inflammatory bowel disease has a small effect on bone mineral density and risk for osteoporosis. Clin Gastroenterol Hepatol. (2013) 11:278–85. doi: 10.1016/j.cgh.2012.10.022
69. Haschka, J, Hirschmann, S, Kleyer, A, Englbrecht, M, Faustini, F, Simon, D, et al. High-resolution quantitative computed tomography demonstrates structural defects in cortical and trabecular bone in IBD patients. J Crohns Colitis. (2016) 10:532–40. doi: 10.1093/ecco-jcc/jjw012
70. Garcia-Villatoro, EL, and Allred, CD. Estrogen receptor actions in colitis. Essays Biochem. (2021) 65:1003–13. doi: 10.1042/EBC20210010
71. Elliott, P, Chambers, JC, Zhang, W, Clarke, R, Hopewell, JC, Peden, JF, et al. Genetic loci associated with C-reactive protein levels and risk of coronary heart disease. JAMA. (2009) 302:37–48. doi: 10.1001/jama.2009.954
72. Collaboration CCG. Collaborative pooled analysis of data on C-reactive protein gene variants and coronary disease: judging causality by Mendelian randomisation. Eur J Epidemiol. (2008) 23:531–40. doi: 10.1007/s10654-008-9249-z
73. Relton, CL, and Davey Smith, G. Mendelian randomization: applications and limitations in epigenetic studies. Epigenomics. (2015) 7:1239–43. doi: 10.2217/epi.15.88
74. Ference, BA, Majeed, F, Penumetcha, R, Flack, JM, and Brook, RD. Effect of naturally random allocation to lower low-density lipoprotein cholesterol on the risk of coronary heart disease mediated by polymorphisms in NPC1L1, HMGCR, or both: a 2 x 2 factorial Mendelian randomization study. J Am Coll Cardiol. (2015) 65:1552–61. doi: 10.1016/j.jacc.2015.02.020
75. Rees, JMB, Foley, CN, and Burgess, S. Factorial Mendelian randomization: using genetic variants to assess interactions. Int J Epidemiol. (2020) 49:1147–58. doi: 10.1093/ije/dyz161
76. Sasaki, Y, Otsuka, K, Arimochi, H, Tsukumo, SI, and Yasutomo, K. Distinct roles of IL-1beta and IL-18 in NLRC4-induced autoinflammation. Front Immunol. (2020) 11:591713. doi: 10.3389/fimmu.2020.591713
77. Lapointe, TK, and Buret, AG. Interleukin-18 facilitates neutrophil transmigration via myosin light chain kinase-dependent disruption of occludin, without altering epithelial permeability. Am J Physiol Gastrointest Liver Physiol. (2012) 302:G343–51. doi: 10.1152/ajpgi.00202.2011
78. Barrett, JC, Hansoul, S, Nicolae, DL, Cho, JH, Duerr, RH, Rioux, JD, et al. Genome-wide association defines more than 30 distinct susceptibility loci for Crohn's disease. Nat Genet. (2008) 40:955–62. doi: 10.1038/ng.175
79. Rosenfeld, G, Brown, J, Vos, PM, Leipsic, J, Enns, R, and Bressler, B. Prospective comparison of standard- versus low-radiation-dose CT Enterography for the quantitative assessment of Crohn disease. AJR Am J Roentgenol. (2018) 210:W54–62. doi: 10.2214/AJR.17.18296
80. Liu, Z, Zhang, Y, Jin, T, Yi, C, Ocansey, DKW, and Mao, F. The role of NOD2 in intestinal immune response and microbiota modulation: a therapeutic target in inflammatory bowel disease. Int Immunopharmacol. (2022) 113:109466. doi: 10.1016/j.intimp.2022.109466
81. Sidiq, T, Yoshihama, S, Downs, I, and Kobayashi, KS. Nod2: a critical regulator of Ileal microbiota and Crohn's disease. Front Immunol. (2016) 7:367. doi: 10.3389/fimmu.2016.00367
82. Prescott, NJ, Dominy, KM, Kubo, M, Lewis, CM, Fisher, SA, Redon, R, et al. Independent and population-specific association of risk variants at the IRGM locus with Crohn's disease. Hum Mol Genet. (2010) 19:1828–39. doi: 10.1093/hmg/ddq041
83. Thanassoulis, G, and O'Donnell, CJ. Mendelian randomization: nature's randomized trial in the post-genome era. JAMA. (2009) 301:2386–8. doi: 10.1001/jama.2009.812
84. Kobylecki, CJ, Afzal, S, Davey Smith, G, and Nordestgaard, BG. Genetically high plasma vitamin C, intake of fruit and vegetables, and risk of ischemic heart disease and all-cause mortality: a Mendelian randomization study. Am J Clin Nutr. (2015) 101:1135–43. doi: 10.3945/ajcn.114.104497
Keywords: inflammatory bowel disease, osteoporosis, Mendelian randomization, causal association, GWAS
Citation: Dai Z, Xu W, Ding R, Peng X, Shen X, Song J, Du P, Wang Z and Liu Y (2023) Two-sample Mendelian randomization analysis evaluates causal associations between inflammatory bowel disease and osteoporosis. Front. Public Health. 11:1151837. doi: 10.3389/fpubh.2023.1151837
Edited by:
Jindong Ni, Guangdong Medical University, ChinaReviewed by:
Vivek P. Chavda, L M College of Pharmacy, IndiaMing-Li Chen, Harvard University, United States
Copyright © 2023 Dai, Xu, Ding, Peng, Shen, Song, Du, Wang and Liu. This is an open-access article distributed under the terms of the Creative Commons Attribution License (CC BY). The use, distribution or reproduction in other forums is permitted, provided the original author(s) and the copyright owner(s) are credited and that the original publication in this journal is cited, in accordance with accepted academic practice. No use, distribution or reproduction is permitted which does not comply with these terms.
*Correspondence: Zhongchuan Wang, d2FuZ3pob25nY2h1YW5AeGluaHVhbWVkLmNvbS5jbg==; Yun Liu, bGl1eXVuQHhpbmh1YW1lZC5jb20uY24=
†These authors have contributed equally to this work and share first authorship