- 1One Health Research Group, Universidad de las Américas, Quito, Ecuador
- 2Instituto Nacional de Investigación en Salud Pública “Leopoldo Izquieta Pérez”, Guayaquil, Ecuador
- 3Facultad de Medicina, Universidad Católica Santiago de Guayaquil, Guayaquil, Ecuador
- 4Departamento de Patología Infecciosa, Facultad de Veterinaria, Universidad de Extremadura, Cáceres, Spain
- 5Universidad Espíritu Santo, Guayaquil, Ecuador
Objective: Tuberculosis (TB) is a major public health concern in Ecuador and Colombia, considering that both countries are high-burden TB settings. Molecular epidemiology is crucial to understand the transmission dynamics of Mycobacterium tuberculosis complex (MTBC) and to identify active transmission clusters of regional importance.
Methods: We studied the potential transmission of TB between Colombia and Ecuador through the analysis of the population structure of MTBC lineages circulating in the Ecuadorian province of Esmeraldas at the border with Colombia. A total of 105 MTBC strains were characterized by 24-loci MIRU-VNTR and spoligotyping.
Results: MTBC lineage 4 is only present in Esmeraldas; no MTBC strains belonging to Lineage 2–sublineage Beijing were found despite its presence in other provinces of Ecuador and, in Colombia. Genotyping results revealed a high degree of diversity for MTBC in Esmeraldas: Neither active transmission clusters within this province nor including MTBC strains from Colombia or other provinces of Ecuador were found.
Conclusion: Our data suggest that tuberculosis dynamics in this rural and isolated area may be not related to highly transmitted strains but could be influenced by other health determinants that favor TB relapse such as poverty and poor health system access. Further studies including a larger number of MTBC strains from Esmeraldas are necessary to test this hypothesis.
Introduction
The Mycobacterium tuberculosis complex (MTBC) includes bacteria with specific microbiological characteristics that confer certain properties for host adaptation, virulence, and transmission, thus contributing to the development of tuberculosis (TB), an infectious disease that mainly spreads by close person-to-person contact through expulsion and inhalation of contaminated aerosols, affecting the lungs or other organs (1, 2). Considering the importance of TB as one of the leading causes of death around the world, behind COVID-19 and above HIV-AIDS, the understanding of the genetic diversity of M. tuberculosis (MTB) is critical for TB surveillance and prevention (1, 3). Briefly, the MTBC is comprised of seven highly related lineages that differ in geographical distribution, infectious capabilities, transmission modes, and resistance to antibiotics: Lineage 1 (Indo-Oceanic), Lineage 2 (East Asia), Lineage 3 (India–East Asia), Lineage 4 (Euro-American), Lineage 5 (West African 1), Lineage 6 (West African 2), and Lineage 7 (Ethiopia). The most significant sublineages are lineage 1, MANU and EAI; lineage 2, Beijing; lineage 3, Central Asian (CAS) and Delhi; lineage 4, Haarlem, Latin American Mediterranean (LAM), T, X, S, Ghana, URAL, TUR, Uganda, and H37Rv; and lineage 6, AFRI and West African (3, 4). Therefore, the use of molecular markers present in the genome of M. tuberculosis, such as mycobacterial interspersed repetitive units (MIRU-VNTR typing method) and/or spacer sequences in the direct repeat (DR) region (spoligotyping method), allows the characterization of transmission dynamics and clusters of MTBC strains (5–7).
For 2021, the World Health Organization (WHO) estimated for Ecuador a burden of 8500 TB cases (rate of 48/100,000 population) and 370 cases of multidrug resistance TB (MDR-TB) (rate 2.1 of 100,000 population), 830 deaths of HIV-negative TB patients and 330 deaths of HIV-positive TB patients (TB case fatality ratio: 14%) (8). MTBC molecular epidemiology studies in Ecuador are really scarce (9–12). There is a single study addressing the population structure and genetic diversity of MTBC in the whole country, showing that MTBC lineage 4 sublineage LAM is predominant countrywide, and sublineages X and S are also predominant in the Coastal and Andean regions, respectively (9). Similar results have been reported in other studies with MTBC strains from Quito (10) and Guayaquil (12).
For the same year 2021, the estimates of TB cases for Colombia provided by WHO were 21,000 (rate 41 of 100,000 population) and 1,100 cases of MDR-TB (rate 2.2 of 100,000 population), 1700 deaths of HIV-negative TB individuals, and 840 deaths of HIV-positive TB individuals (TB case fatality ratio: 12%) (13). However, total notified TB cases in both countries are lower than the estimates provided by the WHO, with 5,595 cases notified in Ecuador and 13,659 cases in Colombia, suggesting an underestimation of TB cases in both countries (8, 13, 14). The most predominant MTBC lineage in Colombia is lineage 4 (including sublineages like LAM, Haarlem, X, and S) but also lineage 2–sublineage Beijing (4, 15). Colombia and Peru have a significantly higher presence of MTBC lineage 2–sublineage Beijing than other countries in South America, representing a potential risk for TB control in the region (4, 15–22).
As neighbor countries and members of the Andean Community, Ecuador and Colombia have a historically intense migration flow (9). Considering that both countries are high-burden TB settings, and also that Colombia is a hot spot for MTBC lineage 2-Beijing (11, 23, 24), TB transnational transmission studies could improve strategies for TB control (9, 11). There is a previous study addressing the population structure of MTBC in Ecuador that reported clonal complexes formed by MTBC strains from Ecuador and Colombia, although no active transmission clusters were found (9). However, MTBC strains from the northern provinces of Ecuador on the border with Colombia were underrepresented in this study, so the existence of active transmission clusters could not be totally ruled out (9).
The goal of this retrospective study was to assess the population structure of MTBC in the province of Esmeraldas on the border of Ecuador and Colombia to analyze the TB transmission between those countries.
Materials and methods
Mycobacterium tuberculosis strains included in the study
A collection of 105 MTBC isolates from years 2014 to 2016 stored at “Instituto Nacional de Salud Pública e Investigación Leopoldo Izquieta Pérez” (INSPI) in Guayaquil (Ecuador) was included in the study, distributed in 42, 16, and 47 samples for 2014, 2015, and 2016, respectively. MTBC isolates are routinely processed at INSPI laboratories, where culture and antibiotic resistance profiling for first- and second-line drugs used in TB therapy is performed for MTBC cultures following Pan American Health Organization guidelines (25, 26). The samples were previously inactivated and stored to follow the guidelines from this government center. MTBC culture manipulation prior to inactivation was carried out in a BSL2+ facility.
This collection of 105 MTBC isolates included all the MTBC strains available at the time this study was carried out for Esmeraldas, located in the Northern Coastal Region of Ecuador, that borders with Colombia. Nevertheless, according to the reports from the Ecuadorian Ministry of Health for TB cases distributions by province, there were 121 and 200 TB cases reported in Esmeraldas for the years 2017 and 2018, respectively.1 If we estimate an average of 200 TB cases per year in this province, the total number of cases for 2014–2016 would be 600, so a collection of 105 MTBC strains would represent 17.5% of the total cases in Esmeraldas province.
Additionally, information from MTBC strains from Ecuador and Colombia was retrieved from the bibliography, as it has been done in similar studies (27, 28). For the phylogenetic analysis described below, 190 MTBC strains from Colombia for the years 2012–2014 (4) and 385 MTBC strains from Ecuador from the years 2012–2016 (9, 26) were included in the study.
Mycobacterium tuberculosis heat inactivation and DNA isolation
A sample from cultures of MTBC was collected and resuspended in TE buffer (10 mM Tris–HCl, 1 mM EDTA, pH 8.0), then inactivated by boiling at 95°C for 45 min. After this process, samples were centrifuged for 5 min at 10000 g and the supernatant was directly used for genotyping (11, 30).
MIRU-VNTR genotyping of MTBC strains
The method is PCR-based and allows the detection of different mycobacterial interspersed repetitive units (MIRU) located at multiple loci in the MTBC genome. Each MIRU allele is identified by a number, thus generating a numerical profile that is used for genotyping studies (5, 31, 32). Amplicons were observed in 2% UltraPure™ Agarose (Invitrogen, California, USA) gels of 15 cm x 10 cm in 0.5X Tris-boric acid-EDTA (TBE) buffer at 100 V for 3 h using a ladder 100-bp Plus Opti-DNA Marker (Cat.No.: G016, Applied Biological Materials Inc., British Columbia, Canada) for size determination. MIRU allele identification was performed according to Supply et al. (33).
Mycobacterium tuberculosis complex strains spoligotyping
Spoligotyping was performed as described elsewhere (34, 35). The results were compared to the databases available in the following free sites: the SITVIT2 website (http://www.pasteur-guadeloupe.fr:8081/SITVIT2/) and the MIRU-VNTRplus (https://www.miru-vntrplus.org/MIRU/index.faces).
Phylogenetic analysis of MTB strains
For phylogenetic analysis, 24 MIRU-VTNR and spoligotyping patterns belonging to the 105 MTBC strains from the years 2014–2016 from Esmeraldas were used. In addition, we retrieved information on MTBC strains from Ecuador and Colombia from the literature: (1) 24 MIRU-VTNR patterns of 385 MTBC isolates for years 2012–2016 from Ecuador (9, 29); (2) 24 MIRU-VTNR and spoligotyping patterns of 190 MTBC strains for years 2012–2014 from Colombia (4).
Genotyping data were analyzed using the MIRU-VNTRplus web application2 (36). Lineage identification was performed by similarity search, using MIRU-VNTR and spoligotyping information, by categorical distance measure (MIRU-VNTR weight: 1, Spoligo weight: 1). Calculation of Neighbor-joining Tree (NJT) and Minimum Spanning Tree (MST) were performed using MIRU-VNTR and/or Spoligotyping information when available.
Results
Drug susceptibility testing of MTBC strains from Esmeraldas Province of Ecuador
Regarding the 105 MTBC strains included in the study, the information for drug susceptibility testing was available for 98 of them: 23 of 98 (23.5%) were resistant to isoniazid (7 of 23 were monoresistant), 10 of 98 (10.2%) were resistant to streptomycin (6 of 10 were monoresistant), 6 of 98 (6.1%) were resistant to ethambutol, 17 of 98 (17.3%) were resistant to rifampicin (1 of 17 was monoresistant), and 5 of 28 (5.1%) were resistant to pyrazinamide. MTBC strains resistant to isoniazid and rifampicin (MDR) were 16 of 98 (16.3%). On the other hand, 71 of 98 (72.4%) MTBC strains were sensible to all the drugs tested (Table 1).
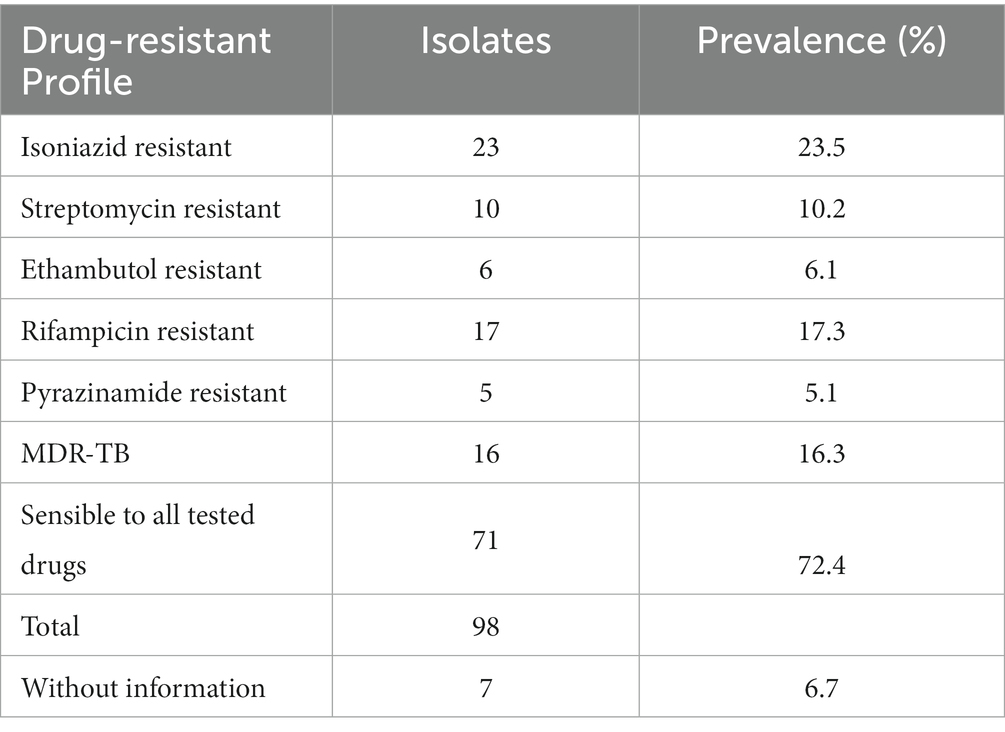
Table 1. Drug resistance profile for MTBC strains from Esmeraldas included in this study (MDR-TB are strains resistant to isoniazid + rifampicin).
Population structure of MTBC strains from Esmeraldas province in Ecuador
The 105 MTBC strains from Esmeraldas were analyzed using 24-loci MIRU-VNTR and spoligotyping. A neighbor-joining tree (NJT) based on this genotypic information is shown in Figure 1. The lineage distribution obtained for MTBC strains from Esmeraldas revealed a high degree of diversity, and no active transmission clusters without any single loci variation in 24-loci MIRU-VNTR were found (Figure 1). The lineage distribution for the MTBC strains from Esmeraldas province was 100% L4, with sublineages LAM 52/105 (49.5%), Ghana 29/105 (27.6%), Haarlem 12/105 (11.4%), Cameroon 5/105 (4.8%), S 3/105 (2.9%), Uganda I 3/105 (2.9%), and X 1/105 (0.95%) (Figures 1, 2). MTBC LAM strains were more diverse in comparison with Ghana strains, while Haarlem strains were not well defined in a branch (Figure 1).
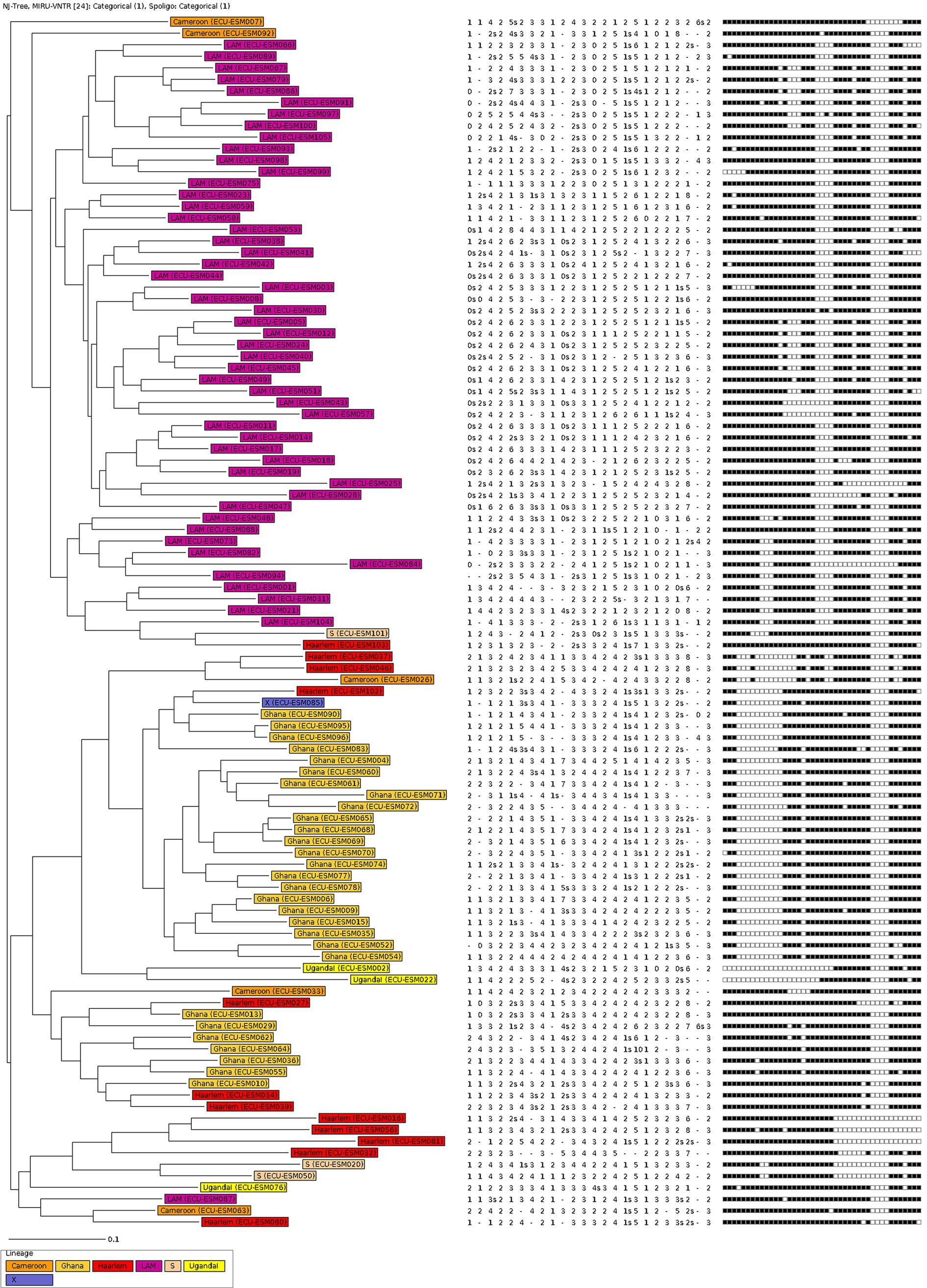
Figure 1. Population structure of MTBC from Esmeraldas province. The neighbor-joining tree was done with 24-loci MIRU-VNTR and spoligotyping data.
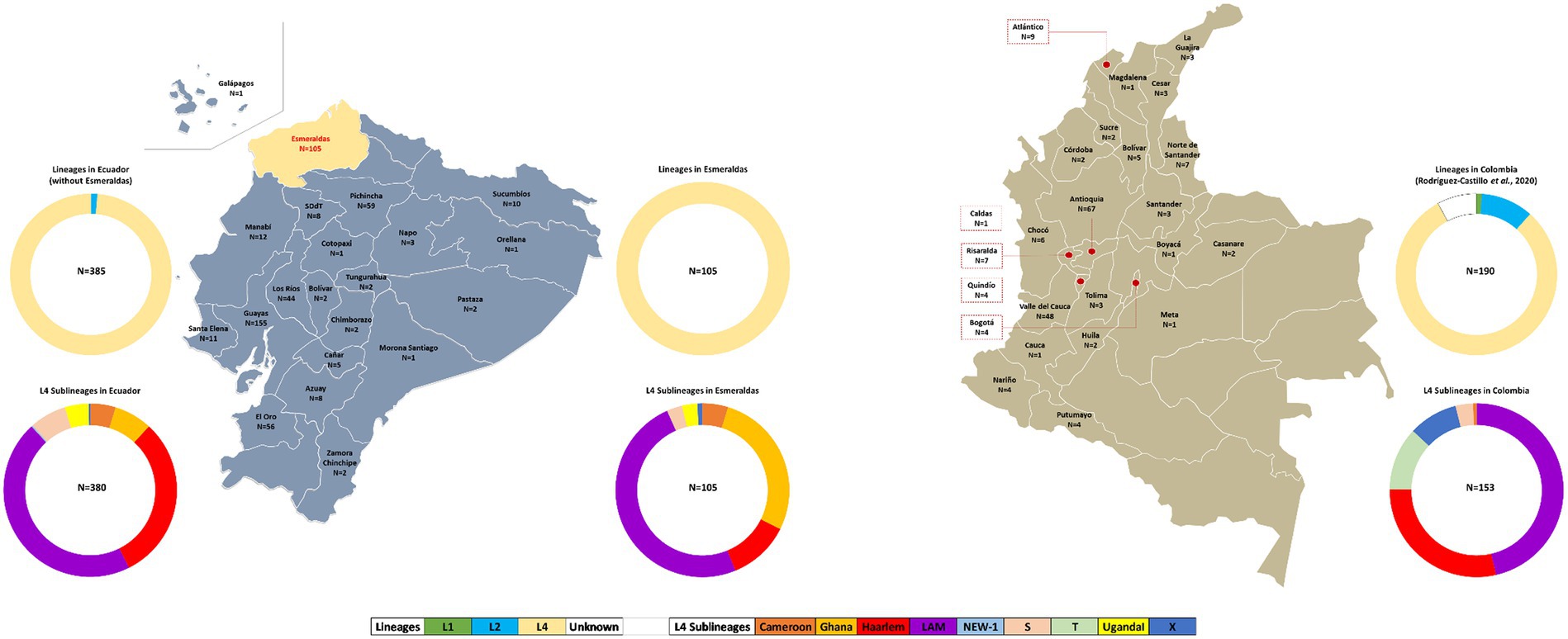
Figure 2. Geographical and sublineage distribution of MTBC strains from Esmeraldas (our study), Ecuador (9), and Colombia (20). Ring charts represent the occurrence of MTBC lineages in Esmeraldas province, the rest of Ecuador, and Colombia.
Comparison of population structure of MTBC strains from Esmeraldas province, Ecuador, and Colombia
The geographic distribution of MTBC strains and lineage distribution for Esmeraldas province, Ecuador, and Colombia are detailed in Figure 2.
The 24-loci MIRU-VNTR patterns of 385 MTBC strains from Ecuador from the years 2012–2016 were retrieved from our previous studies (9, 29) and included in this analysis (Figure 2). The lineage distribution for the MTBC strains from Ecuador (excluding Esmeraldas province) was as follows: 5 of 385 Lineage 2–sublineage Beijing (1.3%) and 380 of 385 Lineage 4 (98.7%). Within Lineage 4: 173 of 385 LAM (44.9%), 117 of 385 Haarlem (30.4%), 27 of 385 Ghana (7%), 26 of 385 S (6.7%), 18 of 385 Cameroon (4.7%), 17of 385 Uganda I (4.4%), 1 of 385 NEW-1 (0.26%), and 1 of 385 (0.26%).
Spoligotyping and 24-loci MIRU-VNTR information of 190 MTBC strains from Colombia from years 2012–2014 was retrieved from reference 4, obtaining the following MTBC lineage distribution: 2 of 190 Lineage 1–Sublineage Manu (1.1%); 20 of 190 Lineage 2–Beijing (10.5%); 158 of 190 Lineage 4 (83.2%). Within Lineage 4: 71 of 190 LAM (37.4%); 44 of 190 Haarlem (25.8%); 18 of 190 T (9.5%); 14 of 190 X (7.4%); 5 of 190 S (2.6%); 1 of 190 Cameroon (0.52%); and 15 of 190 were of unknown lineage (Figure 2).
Figure 3 represents the Minimum Spanning Tree (MST) for MTBC strains from Ecuador (2012–2016) and Esmeraldas (2012–2016) using 24-loci MIRU-VNTR information. This analysis included a total number of 495 MTBC strains, including the 105 MTBC strains for Esmeraldas province (See also de NJT in Supplementary Figure S1). This province is highly represented in this analysis as the number of MTBC strains from the most populated provinces of Pichincha and Guayas were 155 and 59, respectively. A very strong segregation of 99 out of the 105 MTBC strains from Esmeraldas compared to the rest of the provinces of Ecuador was observed. Within those 99 strains, two big and clearly delimitated groups of MTBC strains belonging to sublineage LAM and Ghana were found. Only one clonal complex was observed from MTBC strains from Esmeraldas, including exclusively 2 MTBC strains sublineage Ghana from Esmeraldas, with a maximum of 2 loci difference in the 24 MIRU-VNTR pattern (Figure 3). On the other hand, six MTBC strains from Esmeraldas are clearly dispersed within the MTBC strains from the rest of Ecuador. However, none of the 19 clonal complexes that occur in different groups of lineages of MTBC strains from Ecuador included MTBC strains from Esmeraldas.
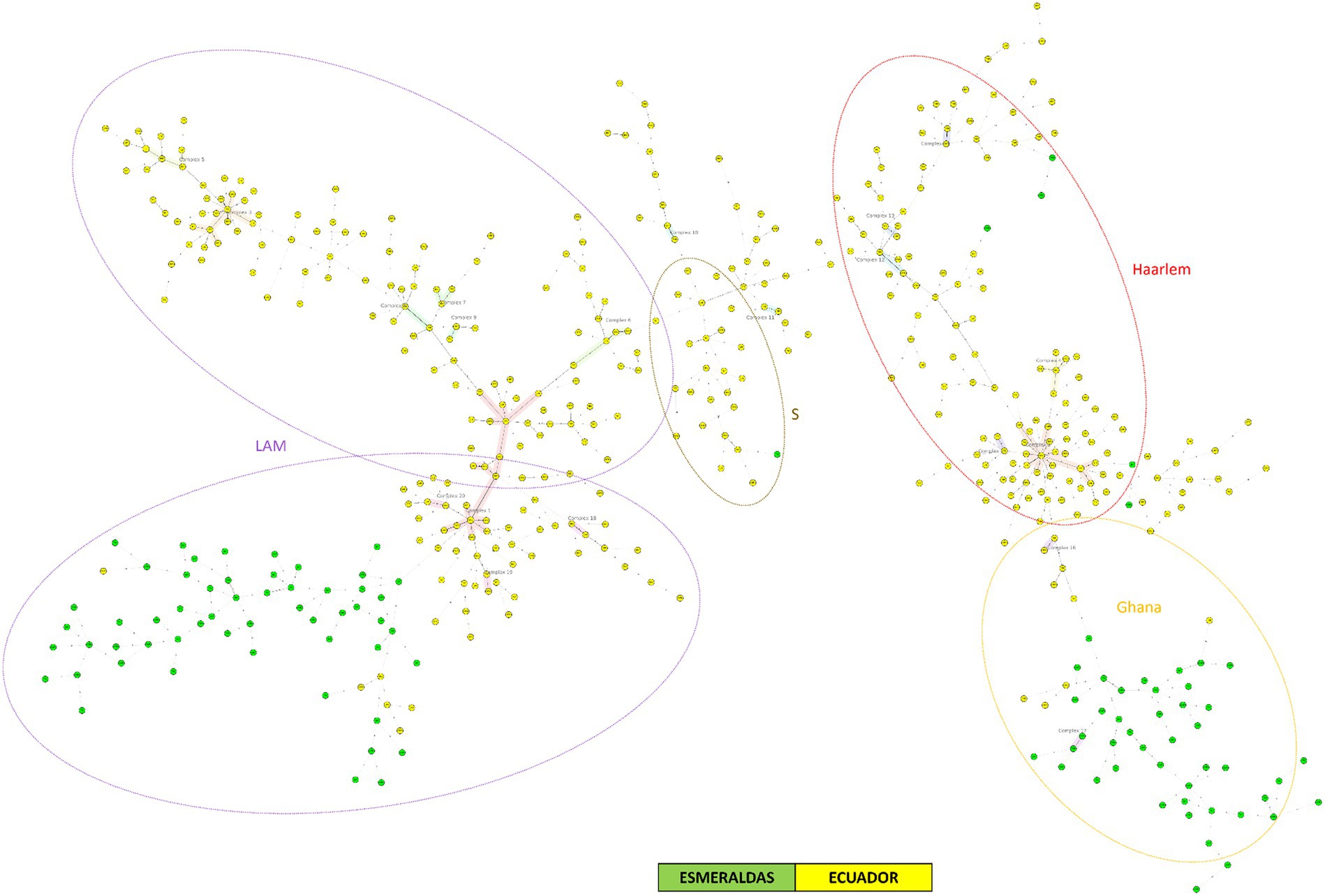
Figure 3. Minimum spanning tree of MTBC strains from Ecuador and Esmeraldas included in this study using 24-loci MIRU-VNTR data. Distinction of genotypic lineages is shown by dotted circles of different colors. The maximum locus difference within a clonal complex is 2.
Figure 4A represents the minimum spanning tree (MST) for MTBC strains from Colombia (2012–2014) and Esmeraldas (2012–2016) using 24-loci MIRU-VNTR information. This analysis included a total number of 190 MTBC strains from Colombia and 105 strains from Esmeraldas province. There is a strong segregation between all the MTBC strains of Esmeraldas and Colombia in the MST, without any clonal complex including strains from both locations (Figure 3). A consistent result was obtained for the NJT (Supplementary Figure S2) where only 2 MTBC strains from Esmeraldas belonging to sublineage Haarlem and LAM were found more phylogenetically related to MTBC strains from Colombia than to other strains from Esmeraldas, both not clustering in clonal complexes.
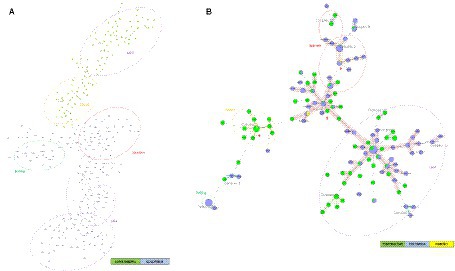
Figure 4. Minimum spanning trees of MTBC strains from Esmeraldas and Colombia included in this study: (A) using 24-loci MIRU-VNTR data and (B) using Spoligotyping data. Distinction of genotypic lineages is shown by dotted circles of different colors. Red arrows indicate clonal complexes formed between MTBC strains from Esmeraldas province and Colombia (Maximum locus difference within a clonal complex is 2). MTBC strains from the Colombian Department of Nariño, on the border with Esmeraldas, are labeled in yellow.
Figure 4B represents the Minimum Spanning Tree (MST) for MTBC strains from Colombia (2012–2015) and Esmeraldas (2012–2016) using only spoligotyping information. Within the reduced segregation capacity of the spoligotyping method for active transmission events, five well-defined clonal complexes (CC1, CC2, CC3, CC7, and CC9) of L4 sublineages including MTBC strains from Esmeraldas and Colombia were observed. Interestingly, three of those clonal complexes (CC1, CC2, and CC3) included MTBC strains from the department of Nariño in the south of Colombia on the border with Esmeraldas and Carchi provinces in Ecuador; isolates reported from this department share three clonal complexes with strains from Esmeraldas (CC1, CC2, and CC3).
Discussion
The rapid identification of a highly active transmission complex of MTBC is crucial to reinforce TB surveillance and control programs. Colombia is one of the countries in Latin America that reports a high burden of MTB of lineages commonly associated with antibiotic resistance and increased transmission (4, 7, 13, 15, 23, 24). We addressed the potential transmission of high-risk MTBC strains from Colombia to Ecuador through the identification of the dominant MTBC genotypes in the border province of Esmeraldas.
The population structure of MTBC in the province of Esmeraldas showed a high genetic variability itself, as no MTBC active transmission clusters were found in the collection of 105 strains for 2014–2016. Additionally, most MTBC strains from Esmeraldas were not phylogenetically closely related to strains from the other Ecuadorian provinces, as no mixed clonal complexes were found. A high level of variability within a reduced rural and isolated geographical area has been also described in Panama and Ecuador (29, 37), and the lack of active transmission clusters despite the high burden of TB could be explained as a consequence of relapse of latent tuberculosis cases (9, 29, 37). Interestingly, the MTBC lineage distribution in Esmeraldas is exclusively composed of MTBC lineage 4 strains with no presence of Lineage 2–sublineage Beijing. Despite the close proximity to Colombia where Lineage 2–sublineage Beijing represents 5% of the MTBC population (4, 24), we could not find evidence of recent transnational transmission of this lineage. These results confirm previous findings reporting circulation of Beijing lineage only in limited locations of the Americas like Cuba, Colombia, and Peru (24). Nevertheless, permanent genetic surveillance should be implemented within the Ecuadorian national TB surveillance program to identify active transmission clusters, as has been described, for instance, in Panama (37) or Tunisia (27, 28). This is especially relevant considering the presence of hot spots for active transmission clusters like prisons in Latin American region (38).
Regarding drug resistance results, we found a 16.3% prevalence of MDR-TB in our study population. This value is much higher than the 4.35% MDR-TB prevalence estimated for Ecuador by the WHO (1, 8). However, similar results of higher prevalence of MDR-TB that WHO estimation was also found in the previous study for the whole country (9). This difference could be explained by a bias in the TB samples that are received by the National Reference Laboratory in Ecuador. In this sense, not all TB patient samples are processed but those defined by a triage protocol that includes all the samples with drug treatment failure, increasing the probability of detecting MDR-TB.
This study has some limitations. First, the 105 MTBC strains included in the study represented less than 20% percent of total TB cases in Esmeraldas (see details in methods), so we cannot totally rule out the presence of Beijing lineage or active transmission cluster due to sampling bias. Second, as we could only access MTBC strains from 2014 to 2016, the current TB transmission scenario could have changed, especially considering the high level of migration back and for across the Ecuador–Colombia border in recent years due to the huge humanitarian and migratory crisis following the economic collapse of Venezuela (39). Third, the phylogenetic analysis had different resolutions depending on the data available: While all the MTBC strains from Esmeraldas and Colombia had 24-loci MIRU-VNTR and spoligotyping data available, the MTBC strains from other provinces of Ecuador lacked spoligotyping information. Fourth, either MTBC strains collections from Colombia and Ecuador could also have geographical bias, as most of those strains came from the Department of Valle del Cauca in Colombia and from the main cities of Ecuador (Quito and Guayaquil) (4, 9). Moreover, the lack of MTBC strains from the other Ecuadorian border provinces with Colombia represents another source of potential sample bias.
In conclusion, cheap and easy molecular epidemiology tools are still useful for middle to high-burden TB settings in Latin America where whole-genome sequencing is still an expensive approach. Further studies with greater MTBC sample sizes from recent years in this region and others in Ecuador are needed to confirm the main findings in our study, so they could be considered for improvements in the TB surveillance program in high-burden rural settings like Esmeraldas.
Data availability statement
The original contributions presented in the study are included in the article/Supplementary materials, further inquiries can be directed to the corresponding author.
Ethics statement
This study was authorized by the Institutional Review Board of Universidad de Las Américas (code 2024-EXC-001). Written informed consent for participation was not required from the participants or the participants’ legal guardians/next of kin in accordance with the national legislation and institutional requirements.
Author contributions
BC-R: Conceptualization, Data curation, Formal analysis, Investigation, Methodology, Validation, Writing – original draft, Writing – review & editing. SE-A: Data curation, Formal analysis, Investigation, Methodology, Writing – review & editing. GF-S: Conceptualization, Funding acquisition, Investigation, Project administration, Resources, Validation, Writing – review & editing. JB-M: Data curation, Methodology, Validation, Writing – review & editing. NJ-P: Formal analysis, Investigation, Methodology, Validation, Writing – review & editing. CC-F: Data curation, Formal analysis, Investigation, Methodology, Validation, Writing – review & editing. JG: Data curation, Formal analysis, Investigation, Methodology, Writing – review & editing. JJ: Funding acquisition, Resources, Validation, Writing – review & editing. JH: Conceptualization, Funding acquisition, Investigation, Project administration, Resources, Writing – review & editing. MG-B: Conceptualization, Data curation, Formal analysis, Funding acquisition, Investigation, Project administration, Resources, Supervision, Validation, Writing – original draft, Writing – review & editing. SO: Conceptualization, Resources, Writing – review & editing.
Funding
The author(s) declare financial support was received for the research, authorship, and/or publication of this article. This study was funded by Universidad de Las Américas (MED.MGB.23.01).
Conflict of interest
The authors declare that the research was conducted in the absence of any commercial or financial relationships that could be construed as a potential conflict of interest.
Publisher’s note
All claims expressed in this article are solely those of the authors and do not necessarily represent those of their affiliated organizations, or those of the publisher, the editors and the reviewers. Any product that may be evaluated in this article, or claim that may be made by its manufacturer, is not guaranteed or endorsed by the publisher.
Supplementary material
The Supplementary material for this article can be found online at: https://www.frontiersin.org/articles/10.3389/fpubh.2024.1343350/full#supplementary-material
Footnotes
References
1. World Health Organization. Global Tuberculosis Report 2022. Geneva: World Health Organization (2022).
2. Murray, PR, Rosenthal, KS, and Pfaller, MA. Medical microbiology. 9th Edn., Edinburgh: Elsevier (2021).
3. Brites, D, and Gagneux, S. The nature and evolution of genomic diversity in the Mycobacterium tuberculosis complex In: S Gagneux, editor. Strain variation in the Mycobacterium tuberculosis complex: Its role in biology, epidemiology and control. Cham: Springer International Publishing (2017). 1–26.
4. Rodríguez-Castillo, JG, Llerena, C, Argoty-Chamorro, L, Guerra, J, Couvin, D, Rastogi, N, et al. Population structure of multidrug-resistant Mycobacterium tuberculosis clinical isolates in Colombia. Tuberculosis. (2020) 125:102011. doi: 10.1016/j.tube.2020.102011
5. Bull, TJ, Sidi-Boumedine, K, McMinn, EJ, Stevenson, K, Pickup, R, and Hermon-Taylor, J. Mycobacterial interspersed repetitive units (MIRU) differentiate Mycobacterium avium subspecies paratuberculosis from other species of the Mycobacterium avium complex. Mol Cell Probes. (2003) 17:157–64. doi: 10.1016/S0890-8508(03)00047-1
6. Coll, P, and García de Viedma, D. Epidemiología molecular de la tuberculosis. Enferm Infecc Microbiol Clin. (2018) 36:233–40. doi: 10.1016/j.eimc.2018.01.001
7. Woodman, M, Haeusler, I, and Grandjean, L. Tuberculosis genetic epidemiology: a Latin American perspective. Genes (Basel). (2019) 10:53. doi: 10.3390/genes10010053
8. World Health Organization. Tuberculosis profile: Ecuador. (2023). Available at: https://worldhealthorg.shinyapps.io/tb_profiles/?_inputs_&entity_type=%22country%22&lan=%22EN%22&iso2=%22EC%22 (Accessed August 14, 2023).
9. Garzon-Chavez, D, Garcia-Bereguiain, MA, Mora-Pinargote, C, Granda-Pardo, JC, Leon-Benitez, M, Franco-Sotomayor, G, et al. Population structure and genetic diversity of Mycobacterium tuberculosis in Ecuador. Sci Rep. (2020) 10:6237. doi: 10.1038/s41598-020-62824-z
10. Zurita, J, Espinel, N, Barba, P, Ortega-Paredes, D, Zurita-Salinas, C, Rojas, Y, et al. Genetic diversity and drug resistance of Mycobacterium tuberculosis in Ecuador. Int J Tuberc Lung Dis. (2019) 23:166–73. doi: 10.5588/ijtld.18.0095
11. Garzon-Chavez, D, Zurita, J, Mora-Pinargote, C, Franco-Sotomayor, G, Leon-Benitez, M, Granda-Pardo, JC, et al. Prevalence, drug resistance, and genotypic diversity of the Mycobacterium tuberculosis Beijing family in Ecuador. Microb Drug Resist. (2019) 25:931–7. doi: 10.1089/mdr.2018.0429
12. Morey-León, G, Andrade-Molina, D, Fernández-Cadena, JC, and Berná, L. Comparative genomics of drug-resistant strains of Mycobacterium tuberculosis in Ecuador. BMC Genomics. 23:844. doi: 10.1186/s12864-022-09042-1
13. World Health Organization. Tuberculosis profile: Colombia. Avaialble at: https://worldhealthorg.shinyapps.io/tb_profiles/?_inputs_&entity_type=%22country%22&lan=%22EN%22&iso2=%22CO%22 (Accessed August 14, 2023).
14. Ranzani, OT, Pescarini, JM, Martinez, L, and Garcia-Basteiro, AL. Increasing tuberculosis burden in Latin America: an alarming trend for global control efforts. BMJ Glob Health. (2021) 6:e005639. doi: 10.1136/bmjgh-2021-005639
15. Castro, C, Ricardo, A, Zabaleta, A, Llerena, C, and Puerto, G. Caracterización de aislamientos clínicos de Mycobacterium tuberculosis obtenidos de individuos positivos para HIV en Colombia, 2012. Biomedica. (2017) 37:86–95. doi: 10.7705/biomedica.v37i1.3112
16. World Health Organization. Tuberculosis profile: Peru. https://worldhealthorg.shinyapps.io/tb_profiles/?_inputs_&entity_type=%22country%22&iso2=%22PE%22&lan=%22EN%22 (Accessed August 13, 2023).
17. del Castillo, H, Mendoza-Ticona, A, Saravia, JC, and Somocurcio, JG. Epidemia de Tuberculosis multidrogo resistente y extensivamente resistente a drogas (TB MDR/XDR) en el Perú: Situación y propuestas para su control. Rev Peru de Med Exp y Salud Pública. (2009) 26:380–386. doi: 10.17843/rpmesp.2009.263.1391
18. Wong, P, Puray, M, Gonzales, A, and Sevilla, CR. Epidemiología molecular de la tuberculosis en el Peru. Rev Peru Epidemiol. (2011) 15:6–16. Available at: http://www.redalyc.org/articulo.oa?id=203119644002
19. Iwamoto, T, Grandjean, L, Arikawa, K, Nakanishi, N, Caviedes, L, Coronel, J, et al. Genetic diversity and transmission characteristics of Beijing family strains of Mycobacterium tuberculosis in Peru. PLoS One. 7:e49651. doi: 10.1371/journal.pone.0049651
20. Cáceres, O, Rastogi, N, Bartra, C, Couvin, D, Galarza, M, Asencios, L, et al. Characterization of the genetic diversity of extensively-drug resistant Mycobacterium tuberculosis clinical isolates from pulmonary tuberculosis patients in Peru. PLoS One. (2014) 9:e112789. doi: 10.1371/journal.pone.0112789
21. Santos-Lazaro, D, Gavilan, RG, Solari, L, Vigo, AN, and Puyen, ZM. Whole genome analysis of extensively drug resistant Mycobacterium tuberculosis strains in Peru. Sci Rep. 11:9493. doi: 10.1038/s41598-021-88603-y
22. Tarazona, D, Galarza, M, Levano, KS, and Guio, H. Comparative genomic analysis of Peruvian strains of Mycobacterium tuberculosis. Rev Peru Med Exp Salud Publica. (2016) 33:256–63. doi: 10.17843/rpmesp.2016.332.2192
23. Nieto Ramirez, LM, Ferro, BE, Diaz, G, Anthony, RM, de Beer, J, and van Soolingen, D. Genetic profiling of Mycobacterium tuberculosis revealed “modern” Beijing strains linked to MDR-TB from southwestern Colombia. PLoS One. (2020) 15:e0224908. doi: 10.1371/journal.pone.0224908
24. Cerezo-Cortés, MI, Rodríguez-Castillo, JG, Hernández-Pando, R, and Murcia, MI. Circulation of M. tuberculosis Beijing genotype in Latin America and the Caribbean. Pathog Glob Health. (2019) 113:336–51. doi: 10.1080/20477724.2019.1710066
25. Franco-Sotomayor, G, Rivera-Olivero, IA, Leon-Benitez, M, Uruchima-Campoverde, SE, Cardenas-Franco, G, Perdomo-Castro, ME, et al. Fast, simple, and cheap: the Kudoh-Ogawa swab method as an alternative to the Petroff–Lowenstein-Jensen method for culturing of Mycobacterium tuberculosis. J Clin Microbiol. (2020) 58. doi: 10.1128/JCM.01424-19
26. Franco-Sotomayor, G, Garzon-Chavez, D, Leon-Benitez, M, de Waard, JH, and Garcia-Bereguiain, MA. A first insight into the katg and rpob gene mutations of multidrug-resistant Mycobacterium tuberculosis strains from Ecuador. Microb Drug Resist. (2019) 25:524–7. doi: 10.1089/mdr.2018.0203
27. Skhairia, MA, Dekhil, N, and Mardassi, H. Evolutionary history and spread of the Mycobacterium tuberculosis Latin American and Mediterranean (L4.3/LAM) sublineage, Tunisia. Tuberculosis (Edinb). (2023) 138:102297. doi: 10.1016/j.tube.2022.102297
28. Skhairia, MA, Dekhil, N, Mhenni, B, Fradj, SB, and Mardassi, H. Successful expansion of Mycobacterium tuberculosis Latin American and Mediterranean sublineage (L4.3/LAM) in Tunisia mainly driven by a single, long-established clonal complex. Int J Infect Dis. (2021) 103:220–5. doi: 10.1016/j.ijid.2020.11.195
29. Castro-Rodriguez, B, León-Ordoñez, K, Franco-Sotomayor, G, Benítez-Medina, JM, Cárdenas-Franco, G, Granda, JC, et al. A first insight into tuberculosis transmission between Ecuador and Peru through the population structure of Mycobacterium tuberculosis in the border province of “El Oro”. J Infection Public Health. (In press).
30. Mora-Pinargote, C, Garzon-Chavez, D, Franco-Sotomayor, G, Leon-Benitez, M, Granda-Pardo, JC, Trueba, G, et al. Country-wide rapid screening for the Mycobacterium tuberculosis Beijing sublineage in Ecuador using a single-nucleotide polymorphism-polymerase chain reaction method. Int J Mycobacteriol. (2019) 8:366–70. doi: 10.4103/ijmy.ijmy_132_19
31. Supply, P, Allix, C, Lesjean, S, Cardoso-Oelemann, M, Rüsch-Gerdes, S, Willery, E, et al. Proposal for standardization of optimized mycobacterial interspersed repetitive unit-variable-number tandem repeat typing of Mycobacterium tuberculosis. J Clin Microbiol. (2006) 44:4498–510. doi: 10.1128/JCM.01392-06
32. Alarcón, V, Alarcón, E, Figueroa, C, and Mendoza-Ticona, A. Tuberculosis in Peru: epidemiological situation, progress and challenges for its control. Rev Peru Med Exp Salud Publica. (2017) 34:299–310. doi: 10.17843/rpmesp.2017.342.2384
33. Supply, P. Multilocus variable number tandem repeat genotyping of Mycobacterium tuberculosis technical guide. (2014). Available at: https://www.researchgate.net/publication/265990159.
34. Kamerbeek, J, Schouls, L, Kolk, A, van Agterveld, M, van Soolingen, D, Kuijper, S, et al. Simultaneous detection and strain differentiation of Mycobacterium tuberculosis for diagnosis and epidemiology. J Clin Microbiol. (1997) 35:907–14. doi: 10.1128/jcm.35.4.907-914.1997
35. García-Jiménez, WL, Cortés, M, Benítez-Medina, JM, Hurtado, I, Martínez, R, García-Sánchez, A, et al. Spoligotype diversity and 5-year trends of bovine tuberculosis in Extremadura, southern Spain. Trop Anim Health Prod. (2016) 48:1533–40. doi: 10.1007/s11250-016-1124-4
36. Allix-Béguec, C, Harmsen, D, Weniger, T, Supply, P, and Niemann, S. Evaluation and strategy for use of MIRU-VNTRplus, a multifunctional database for online analysis of genotyping data and phylogenetic identification of Mycobacterium tuberculosis complex isolates. J Clin Microbiol. (2008) 46:2692–9. doi: 10.1128/JCM.00540-08
37. Acosta, F, Saldaña, R, Miranda, S, Candanedo, D, Sambrano, D, Morán, M, et al. Heterogeneity of Mycobacterium tuberculosis strains circulating in Panama’s Western region. Am J Trop Med Hyg. 109:740–7. doi: 10.4269/ajtmh.23-0039
38. Islam, Z, Thada, PK, Rahmat, ZS, Akhtar, S, Ahmad, S, Costa, ACDS, et al. Tuberculosis behind bars in Latin America and Caribbean: a growing public health crisis. Infect Control Hosp Epidemiol. (2022) 43:2000–2. doi: 10.1017/ice.2021.424
Keywords: Mycobacterium tuberculosis , MIRU-VNTR, spoligotyping, migration, Ecuador
Citation: Castro-Rodriguez B, Espinoza-Andrade S, Franco-Sotomayor G, Benítez-Medina JM, Jiménez-Pizarro N, Cárdenas-Franco C, Granda JC, Jouvin JL, Orlando SA, Hermoso de Mendoza J and García-Bereguiain M& (2024) A first insight into tuberculosis transmission at the border of Ecuador and Colombia: a retrospective study of the population structure of Mycobacterium tuberculosis in Esmeraldas province. Front. Public Health. 12:1343350. doi: 10.3389/fpubh.2024.1343350
Edited by:
Hai-Feng Pan, Anhui Medical University, ChinaReviewed by:
Victoria Pando-Robles, National Institute of Public Health (Mexico), MexicoEustachio Cuscianna, University of Bari Aldo Moro, Italy
Copyright © 2024 Castro-Rodriguez, Espinoza-Andrade, Franco-Sotomayor, Benítez-Medina, Jiménez-Pizarro, Cárdenas-Franco, Granda, Jouvin, Orlando, Hermoso de Mendoza and García-Bereguiain. This is an open-access article distributed under the terms of the Creative Commons Attribution License (CC BY). The use, distribution or reproduction in other forums is permitted, provided the original author(s) and the copyright owner(s) are credited and that the original publication in this journal is cited, in accordance with accepted academic practice. No use, distribution or reproduction is permitted which does not comply with these terms.
*Correspondence: Miguel Ángel García-Bereguiain, bWFnYmVyZWd1aWFpbkBnbWFpbC5jb20=