An Adverse Outcome Pathway for Decreased Lung Function Focusing on Mechanisms of Impaired Mucociliary Clearance Following Inhalation Exposure
- 1Philip Morris International R&D, Philip Morris Products S.A., Neuchatel, Switzerland
- 2PETA Science Consortium International e.V., Stuttgart, Germany
- 3British American Tobacco (Investments) Ltd., Group Research and Development, Southampton, United Kingdom
- 4Broughton Nicotine Services, Earby, Lancashire, United Kingdom
Adverse outcome pathways (AOPs) help to organize available mechanistic information related to an adverse outcome into key events (KEs) spanning all organizational levels of a biological system(s). AOPs, therefore, aid in the biological understanding of a particular pathogenesis and also help with linking exposures to eventual toxic effects. In the regulatory context, knowledge of disease mechanisms can help design testing strategies using in vitro methods that can measure or predict KEs relevant to the biological effect of interest. The AOP described here evaluates the major processes known to be involved in regulating efficient mucociliary clearance (MCC) following exposures causing oxidative stress. MCC is a key aspect of the innate immune defense against airborne pathogens and inhaled chemicals and is governed by the concerted action of its functional components, the cilia and airway surface liquid (ASL). The AOP network described here consists of sequences of KEs that culminate in the modulation of ciliary beat frequency and ASL height as well as mucus viscosity and hence, impairment of MCC, which in turn leads to decreased lung function.
1 Introduction
Regulatory frameworks are moving towards risk assessment approaches that better protect human health and are not reliant on testing in animals. Therefore, 21st century science is incorporating the use of human-relevant methods that are ethical, scientifically sound, and can accurately predict the toxicity of chemicals. In silico models that consider human-relevant parameters as well as in vitro methods that vary in complexity—spanning from mono-to co-culture systems—are already being used to predict human outcomes. For example, the Organisation for Economic Co-operation and Development (OECD) (OECD, 2021) uses combined information from several sources (e.g. in silico predictions, in chemico, in vitro data) to predict pathological outcomes in humans in response to chemical exposure. Anchored to known mechanisms of human toxicity such mechanism-based approaches enable us to understand whether a chemical will be toxic and through which pathway(s) it may act to cause the adverse outcome (AO) (Clippinger et al., 2018).
Adverse outcome pathways (AOP) are a means to organize known information related to a pathological outcome and understand the mechanism leading to the adverse effect. Starting with a molecular initiating event (MIE) and ending in an AO, AOPs are a sequence of causally linked key events (KE) that span different levels of biological organization—from the molecular to the whole organism level (Ankley et al., 2010). An AOP may not necessarily include every single event that contributes to the development of the AO, but it does include all KEs that are critical for its development (OECD, 2017). In vitro and in silico assays that measure each of the KEs of an AOP can be used to design testing approaches that closely predict human responses and replace the need for in vivo data in order to derive benchmark values for determining the potential adverse health impacts of chemicals. When combined with existing data and physicochemical information related to the test substance, AOP-based testing can help develop integrated approaches that can predict human responses (OECD, 2021). Given the usefulness of AOPs in the risk assessment of chemicals, the OECD launched a program for the development of AOPs in 2012; called the AOP-Wiki, this program is overseen by the Extended Advisory Group on Molecular Screening and Toxicogenomics (EAGMST). Several online resources, including the OECD AOP users’ handbook, are available to aid developers in compiling AOPs on the AOPwiki (OECD, 2018). There are currently more than 300 AOPs online, at various stages of completion. One of these is AOP148 [EGFR Activation Leading to Decreased Lung Function, https://aopwiki.org/aops/148], which is extended and complemented by the AOP network described here.
With a surface area of ∼100 m2 and ventilated by 10,000 to 20,000 L of air per day (National Research Council, 1988; Frohlich et al., 2016), the lungs are a major barrier that protect the body from a host of external factors that enter the respiratory system and may cause lung pathologies. Mucociliary clearance (MCC) is a key aspect of the innate immune defense against airborne pathogens and inhaled particles. MCC is governed by the concerted action of its functional components, the cilia and the airway surface liquid (ASL), where the latter comprises mucus and the periciliary layer (PCL) (Bustamante-Marin and Ostrowski, 2017). Healthy subjects produce >10 ml airway secretions daily (King, 2006), which are continuously transported by the mucociliary escalator. Disturbances in any of the processes that regulate ASL volume, mucus production, mucus viscoelastic properties, or ciliary function can cause MCC dysfunction and are linked to airway diseases such as chronic obstructive pulmonary disease (COPD) and asthma, both of which bear a significant risk of increased morbidity and mortality. The mechanism by which exposure to inhaled toxicants might lead to mucus hypersecretion and thereby impact pulmonary function has already been mapped in AOP148 on decreased lung function. However, whether an exposure-related decline in lung function is solely related to excessive production of mucus is debatable, particularly in light of the close relationship between mucus, cilia function, and efficient MCC. To date, no single event has been attributed to MCC impairment. This AOP work evaluates the major processes known to be involved in ensuring efficient MCC and consists of sequences of KEs that culminate in the modulation of ASL, ciliary beat frequency (CBF), and mucus viscosity. Together, these processes impair MCC, which—when persistent—leads to decreased lung function. Evidence was gathered from the peer-reviewed literature from multiple sources (e.g., PubMed, Web of Science, Scopus) by keyword searches. No publication date limit was applied. Both empirical and quantitative evidence was captured, consolidated and transferred to the corresponding KE and KER pages on the AOPwiki following the recommendations in the AOP User’s Handbook.
2 Summary of Key Events and Mechanisms
The epithelium of the respiratory tract has a powerful defense mechanism against airborne pollutants, owing to the combined performance of mucus-producing goblet cells and ciliated cells that are covered with microtubular projections called cilia. In response to various irritants and pathogens, goblet cells produce and secrete mucus, and the cilia sweep the mucus upward through coordinated beating motions, thus clearing the airways of these substances—a process which is termed MCC. Optimal MCC is dependent on multiple factors, including cilia number and structure, ASL height1, and the physical and chemical properties of mucus. Any disturbances in these factors can lead to impaired MCC. A summary schematic of the AOP network delineating processes that lead from oxidative stress to decreased lung function is presented in Figure 1 and detailed on the AOPwiki (https://aopwiki.org/aops/411, https://aopwiki.org/aops/424, https://aopwiki.org/aops/425).The MIE for this network of AOP is oxidative stress. Oxidative stress is generally regarded as a redox imbalance characterized by the increased production of oxidative species and concurrent depletion of antioxidant defenses. Thus, the overall redox balance of the cell/tissue is tipped in favor of oxidation. Various highly reactive species, collectively referred to as “reactive oxygen species” (ROS) or “reactive nitrogen species” (RNS), are formed continuously at relatively low concentrations during the normal biochemical functioning of cells and tissues. They are highly unstable because they contain unpaired electrons capable of initiating oxidation reactions and include free radicals such as hydroxyl radicals, superoxide anions, oxygen radicals, nitric oxide, and non-free radicals, such as hydrogen peroxide, peroxynitrite and hypochlorous acid (Rahman et al., 2006). However, upon exposure to certain xenobiotics or in the presence of pathogens, cells may form excessive ROS/RNS, which may react with cellular components such as proteins, lipids and nuclear material, leading to the dysfunction of these components and, ultimately, cell death and disease manifestation (Halliwell and Aruoma, 1991; Berlett and Stadtman, 1997). Protective enzymes such as catalase, glutathione peroxidase, superoxide dismutase, and thioredoxin—in combination with radical scavengers such as glutathione, ascorbic acid, uric acid and vitamin E—work in concert to maintain ROS/RNS levels that are not overly damaging to cells and cellular systems (Rahman et al., 2006).
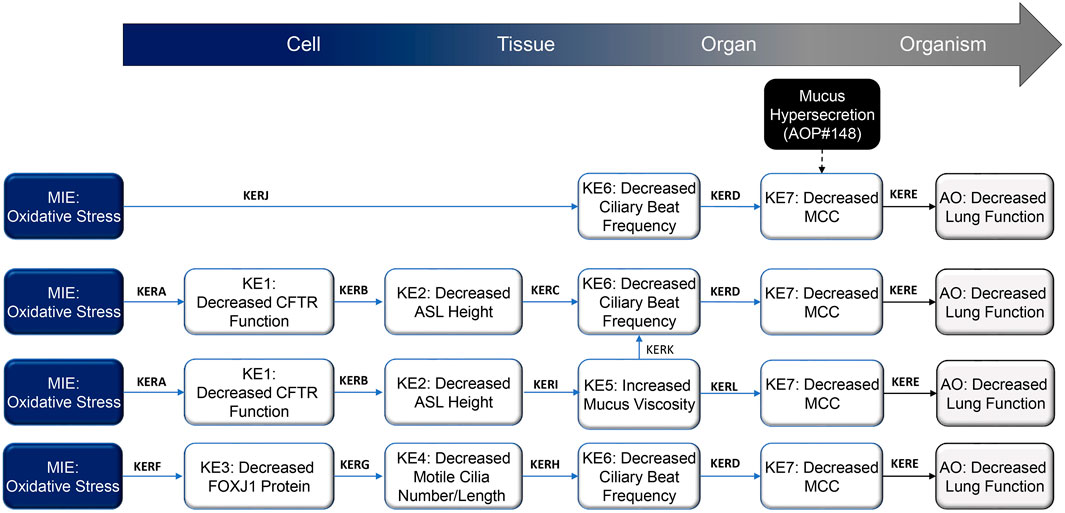
FIGURE 1. Schematic representation of the proposed decreased lung function adverse outcome pathways (AOP), focusing on mechanisms that result in impaired mucociliary clearance (MCC) following inhalation exposure. Abbreviations: AO, adverse outcome; ASL, airway surface liquid; CFTR, cystic fibrosis transmembrane regulator; FOXJ1, forkhead box protein J1; KE, key event; KER; key event relationship; MCC, mucociliary clearance; MIE, molecular initiating event.
In the lungs, free radical species may be endogenously produced or introduced following exposure to exogenous sources, such as air pollutants, inhaled chemicals/therapeutics, and cigarette smoke (Church and Pryor, 1985). The main cellular sources of reactive species in the lungs include neutrophils, eosinophils, alveolar macrophages, alveolar epithelial cells, bronchial epithelial cells, and endothelial cells (Holland et al., 1990; Kinnula et al., 1992; Kinnula et al., 1995); these cells may increase their ROS/RNS production in response to infection or tissue damage. ROS/RNS generally inflict their effects by remodeling extracellular matrix and stimulating mucus secretion and repair responses (Poli and Parola, 1997). Oxidative stress can lead to a variety of respiratory diseases, such as asthma, acute respiratory distress syndrome and COPD (Rahman and MacNee, 1996; Chabot et al., 1998).With respect to this specific AOP, localized oxidative stress in the airways as a result of cigarette smoke exposure, for example, can cause damage to various proteins linked to the regulation of cilia function. Reduced expression of the CFTR (cystic fibrosis transmembrane conductance regulator) transcript, diminished CFTR protein levels, and altered chloride (Cl−) channel gating lead to acquired CFTR dysfunction (Clunes et al., 2012; Braun, 2014), which perturbs the height of the ASL and facilitates cilia collapse. Furthermore, oxidative damage has been reported to decrease the FOXJ1 (forkhead box protein J1) gene and protein expression, a critical protein involved in the assembly of motile cilia (Milara et al., 2012; Brekman et al., 2014; Garcia-Arcos et al., 2016; Valencia-Gattas et al., 2016; Ishikawa and Ito, 2017). Collectively, these perturbations result in decreased MCC from the upper airways.
CFTR is a multi-domain membrane protein belonging to the large family of adenine nucleotide-binding cassette transporters (Riordan, 2008). It is an integral membrane glycoprotein which functions as cyclic adenosine monophosphate (cAMP)-activated and phosphorylation-regulated Cl− channel at the apical membrane of epithelial cells (Farinha et al., 2013). In respiratory epithelia, CFTR mediates fluid and electrolyte transport, and its function is critical to ASL homeostasis. Exposure to inhaled oxidants leads to decreased CFTR gene and protein expression as well as CFTR internalization, which reduces protein presentation at the membrane and reduces or abolishes short-circuit currents (Cantin et al., 2006a; Cantin et al., 2006b; Clunes et al., 2012; Sloane et al., 2012; Rasmussen et al., 2014). Decreased CFTR expression (KE1) in airway epithelium has been observed in cystic fibrosis and after hypoxia and cigarette smoke exposure, resulting in reduced Cl− transport and, ultimately, reduced ASL depth (Alexander et al., 2012; Clunes et al., 2012; Rasmussen et al., 2014; Woodworth, 2015; Raju et al., 2016).
The ASL is a liquid layer on the apical side of the respiratory epithelium, reportedly between 5 and 100 μm in depth (Widdicombe and Widdicombe, 1995). It consists of an inner aqueous PCL, which spans the length of the cilia, and an outer gel-like mucus layer. The PCL has a low viscosity and enables ciliary beating, thereby facilitating the movement of the outer mucus layer toward the glottis and, ultimately, its removal by cough or ingestion (Antunes and Cohen, 2007). Both ASL composition and height are considered critical for its function (Fischer and Widdicombe, 2006). Under physiological conditions, ASL composition and height are regulated through vectorial transport of electrolytes, driven by transepithelial transport and apical secretion of Cl− by (predominantly) CFTR, which results in passive water secretion and, consequently, increased ASL height. Absorption of sodium ions (Na+) on the apical side by the epithelial sodium channel (ENaC) and its interaction with the basolateral Na+/K+-ATPase leads to net absorption of Na+, which in turn drives fluid absorption and therefore decreases ASL height (KE2) (Hollenhorst et al., 2011; Althaus, 2013). Impairment of CFTR or ENaC function can lead to the dysfunction of the other ion channel (increased CFTR activity leads to decreased ENaC activity and vice versa) (Hobbs et al., 2013; Munkholm and Mortensen, 2014), resulting in perturbation of ASL height.
The number, structure, and cohesive beating of the motile cilia lining the upper and lower respiratory tract are critical for efficient MCC. Motile cilia are microtubular organelles, 6–7 µm long and 0.2–0.3 µm in diameter (Brooks and Wallingford, 2014; Yaghi and Dolovich, 2016). They protrude from the cell surface and generate directional flow of fluid though coordinated beating. Approximately 50–80% of the human respiratory epithelium is comprised of ciliated cells; each ciliated cell is covered by more than a hundred motile cilia, which move mucus upwards (together with mucus-trapped substances) upward (Yaghi and Dolovich, 2016; Bustamante-Marin and Ostrowski, 2017). Cilia formation is initiated and coordinated by a distinct gene expression program, led by the transcription factor FOXJ1 (Brody et al., 2000; Zhou and Roy, 2015). The multiple motile cilia assembly factors MCIDAS (multiciliate differentiation and DNA synthesis associated cell cycle protein) and GMNC (geminin coiled-coil domain containing) converge in positively regulating FOXJ1 (Stubbs et al., 2012; Arbi et al., 2016; Berta et al., 2016), whereas NOTCH (Notch homolog (Drosophila))-, IL-13 (interleukin-13)- or EGF (epidermal growth factor)-triggered signaling antagonizes FOXJ1-driven multiciliogenesis (Gomperts et al., 2007; Shaykhiev et al., 2013; Gerovac et al., 2014; Gerovac and Fregien, 2016). Although various other factors are involved in multiple motile cilia assembly—including MYB (MYB proto-oncogene), RFX3 (regulatory factor X3), ULK4 (Unc-51 like kinase 4), Wnt signaling, and others—they mostly act upstream or in parallel to FOXJ1 (Tan et al., 2013; Choksi et al., 2014; Liu et al., 2016; Schmid et al., 2017). FOXJ1 appears to be the major factor in multiciliogenesis, whereby its activity is necessary and also sufficient for programming cells to assemble functional motile cilia (Vij et al., 2012; Zhou and Roy, 2015). It is not surprising, therefore, that a decrease in FOXJ1 levels (KE3) inhibits ciliogenesis in multiciliated cells in zebrafish and Xenopus (Stubbs et al., 2008), and knockdown of FOXJ1 results in almost complete absence of cilia in mouse epithelial cells (Chen et al., 1998; Brody et al., 2000). FOXJ1 expression also decreases in cigarette smoke extract-treated human airway epithelial cells, leading to suppression of cilia growth, which can be restored by overexpression of the protein (Brekman et al., 2014).
Because ciliated cell density and the multiple motile cilia length and number per cell correlate with CBF—which is routinely used as a predictor of MCC efficiency (King, 2006)—it follows that, if cilia numbers decrease (KE4), CBF decreases (KE6). Cohesive beating of multiple motile cilia with a specific frequency and pattern propels mucus (and trapped particles or pathogens) upwards, creating a continuous movement (Chilvers and O'Callaghan, 2000). CBF is influenced by several factors, including structural modulation in the cilia and the concentrations of the cyclic nucleotides cAMP and cGMP and intracellular calcium (Ca2+) (Rubin, 2002). CBF also depends on the physical and chemical properties of the ASL. If ASL height decreases following, for example, exposure to cigarette smoke, the cilia cannot extend to their full height, and MCC efficiency will drop. In addition, reduced ASL height results in airway dehydration, which increases mucus viscosity (KE5) (Gheber et al., 1998; Lai et al., 2009; Fahy and Dickey, 2010). Increased mucus viscosity, in turn, decreases CBF and slows the transport of mucus on the mucociliary escalator (i.e., decreases MCC; KE7). In chronic inflammatory states, as seen (for example) in the lungs of cystic fibrosis, asthma, or COPD patients, decreased MCC can lead to mucus impaction, resulting in the formation of mucus plugs, which then in turn obstruct the airways and, consequently, lead to decreased lung function (AO) over time (Wanner et al., 1996; Szczesniak et al., 2017; Dunican et al., 2021).
3 Empirical Evidence for Key Event Relationships
Table 1 presents a summary of supporting evidence for each of the KERs in this AOP. KERs are rated as “strong”, “moderate”, or “weak” on the basis of empirical evidence supporting a change in an upstream KE (KEup) leading to an appropriate change in the immediate downstream KE (KEdown). Other considerations are whether KEups occur at lower doses, earlier time points, and at a higher incidence than KEdowns and if there are any inconsistences in the published data. The experimental evidence for a causal relationship between the KEup and KEdown in this AOP has been provisionally rated as “moderate” or “strong” in most cases.
Exposure to inhaled oxidants, such as cigarette smoke and ozone, leads to decreased CFTR gene and protein expression as well as CFTR internalization (KE1), thereby reducing or abolishing open probabilities, short-circuit currents and subsequently ASL height/volume (KE2) (Kulka et al., 2005; Cantin et al., 2006a; Cantin et al., 2006b; Qu et al., 2009; Clunes et al., 2012; Sloane et al., 2012; Rasmussen et al., 2014). Both reduced mRNA stability (Cantin et al., 2006a) and decreased transcription rates (Bargon et al., 1992a; Bargon et al., 1992b; Rasmussen et al., 2014) reportedly contribute to diminished CFTR mRNA expression. CFTR expression was also modulated by STAT1 (Kulka et al., 2005; Qu et al., 2009) and Nrf2 signaling (Zhang et al., 2015). Additionally, on the post-transcriptional level, CFTR function has been shown to be affected by oxidative stress (Clunes et al., 2012) and ischemia (Brézillon et al., 1997; Bodas et al., 2017).
Serous and glandular secretions of the airway epithelium contribute to the ASL, and epithelial ion channel function is critical to ASL homeostasis. Absorption of liquid to and from the mucus layer serves to maintain ASL depth. The regulation of these reabsorption processes is complex and not fully elucidated (Boucher, 2004). Experimental evidence suggests that the balance between Na+ absorption and Cl− secretion—mediated by ENaC and CFTR, respectively—plays a major role in these processed, with the ion channels affecting each other’s activity (Boucher, 2003; Boucher, 2004; Schmid et al., 2011). Impaired functioning of the CFTR and ENaC ion channels results in enhanced Na+ absorption, reduced Cl− secretion, and consequently, reduced ASL height (KE2). This phenomenon is well known not only from studies in models of cystic fibrosis and acquired CFTR deficiency—even though the exact mechanism of the interaction between these two channels remains to be elucidated (Tarran et al., 2001; Boucher, 2003; Zhang et al., 2013; Hassan et al., 2014; Rasmussen et al., 2014; Woodworth, 2015; Raju et al., 2016)—but also from studies with pharmacological agents that enhance CFTR expression and/or function or perturb the interaction between CFTR and ENaC (Van Goor et al., 2009; Van Goor et al., 2011; Lambert et al., 2014).
Under physiological conditions, ASL height is adjusted to the appropriate height, which helps maintain the PCL depth at approximately the length of the cilia (Antunes and Cohen, 2007). If the airways become “dehydrated” (i.e., the ASL height decreases; KE2), the cilia collapse and ciliary movement is slowed or inhibited (KE6) (Matsui et al., 1998; Tarran et al., 2001; Knowles and Boucher, 2002; Munkholm and Mortensen, 2014). Decreased ASL height also contributes to increased mucus viscosity (KE5), a phenomenon that is well described in cystic fibrosis, where CFTR defect results in decreased ASL height, leading to decreased MCC (KE7) and subsequent mucus plugging (Birket et al., 2014; Birket et al., 2016; Birket et al., 2018).
Free radicals such as super oxides, hydroxyl radicals, and hydrogen peroxides are a common factor in various respiratory diseases, such as acute respiratory distress syndrome, asthma and pneumonia. Oxidative stress (such as that caused by cigarette smoke exposure or irradiation) leads to decreased FOXJ1 gene and protein expression (KE3) as well as to decreased FOXJ1 target gene expression (Milara et al., 2012; Brekman et al., 2014; Garcia-Arcos et al., 2016; Valencia-Gattas et al., 2016; Ishikawa and Ito, 2017). Because FOXJ1 is a key factor of multiple motile cilia assembly in the respiratory airways (Zhou and Roy, 2015), oxidative stress blocks the multiciliogenesis program, which is necessary and also sufficient to program cells to grow functional motile cilia (Hua et al., 2010; Vij et al., 2012). Studies in different model organisms have shown that the loss of FOXJ1 (KE3) results in a loss of multiple motile cilia (KE4) (Chen et al., 1998; Brody et al., 2000; Stubbs et al., 2008; Vij et al., 2012).
Cilia in the respiratory epithelium beat in a coordinated fashion at a frequency of approximately 7–16 Hz, propelling mucus upwards (Joki et al., 1998; Smith et al., 2012; Jing et al., 2017). Many factors have been shown to affect ciliary function, including cilia length, number, structure, orientation, and distribution as well as mucus viscosity, temperature, pH, chemicals, ASL height, and exposure to bacterial and viral pathogens (Kanthakumar et al., 1996; Clary-Meinesz et al., 1998; Joki et al., 1998; Ho et al., 2001; Mall, 2008; Smith et al., 2012; Jing et al., 2017; Snyder et al., 2017). Alterations in normal physiological conditions and healthy cilia number/length/structure (KE4) as well as oxidative stress through exposure to hydrogen peroxide or free radicals typically reduce CBF (KE6) (Burman and Martin, 1986; Clary-Meinesz et al., 1998; Min et al., 1999; Jayathilake et al., 2012).Synchronized ciliary action helps transport mucus from the lungs to the mouth, where it is swallowed or expectorated (Munkholm and Mortensen, 2014). In addition to ASL and mucus properties, the speed of mucus movement—and hence the effectiveness of MCC—is dependent on ciliary amplitude and beat frequency (Rubin, 2002). Aside from genetic defects leading to ciliopathies, there is ample evidence that prolonged exposure to noxious agents, such as cigarette smoke, nitrogen oxide and sulfur dioxide, causes a decrease in CBF (KE6) and, subsequently, MCC (KE7) (Knorst et al., 1994; Yeates et al., 1997; Kakinoki et al., 1998; Cohen et al., 2009; Schmid et al., 2015). CBF also seems to be dependent on mucus viscosity, with the CBF decreasing with increasing viscosity in animal models (Andrade et al., 2005; Kikuchi et al., 2017). This linear correlation between CBF (KE7) and mucus viscosity (KE5) has also been confirmed in mathematical models simulating the two-layer mucociliary transport process (Lee et al., 2011; Sedaghat et al., 2016).
Finally, the link between decreased MCC and decreased lung function (AO) is well established through observations in patients with ciliary defects (e.g., primary ciliary dyskinesia) and cystic fibrosis. Failure to clear mucus from the lungs causes mucus build up, which can lead to mucus plugging in the airways and, consequently, leads to decreased lung function over time (Mossberg et al., 1978; Regnis et al., 1994; Wanner et al., 1996; Robinson and Bye, 2002; Kerem et al., 2014; Szczesniak et al., 2017). Mucus plugging due to decreased MCC is also considered a major cause of airway obstruction and airflow limitation in COPD patients (Okajima et al., 2020; Dunican et al., 2021) and asthmatics (Maxwell, 1985; Kuyper et al., 2003).
4 Overall Assessment of the Adverse Outcome Pathways
4.1 Key Event Essentiality
The definition of essentiality implies that modulation of upstream KEs impacts the downstream KEs in an expected fashion. When blocked or when they fail to occur, the KEs in the current AOP will not necessarily stop the progression to subsequent KEs. Owing to the complex biology of motile cilia formation and function, ASL homeostasis, mucus properties, and MCC, the KEs and AO may be triggered because of alternative pathways or biological redundancies. However, when exacerbated, the KEs promote the occurrence of downstream events that eventually lead to the AO. The causal pathway starting from exposure to oxidants and leading to decreased lung function involves parallel routes with KEs, each of which is sufficient to cause the downstream KE to occur. Different mechanisms—such as oxidant-induced decreases in ASL height due to CFTR function decline or oxidant-induced decrease in cilia number and length as a result of decreased FOXJ1 levels—lead to decreased CBF and decreased MCC. Each of these pathways contributes to the AO, but their relative contributions are difficult to evaluate. We judge the KEs MIE, KE1, KE3, KE4, KE6, and KE7 as highly essential and suggest moderate essentiality for KE2 and KE5 (Table 1; AOPwiki, https://aopwiki.org/aops/411, https://aopwiki.org/aops/424, https://aopwiki.org/aops/425).
4.2 Key Event Relationship Biological Plausibility
Mechanistic data on the pathways that contribute to oxidative stress-elicited lung damage have varied coverage in current literature. The AOP network we present here starts with an oxidant exposure or exposure-causing oxidative stress leading to decreased CFTR and FOXJ1 mRNA and protein levels as well as decreased protein function. KERA (oxidative stress leading to decreased CFTR function) is supported by multiple studies across different species, which suggest their high biological plausibility (for empirical evidence supporting each KER, refer to Table 1). For a similar inhibitory role of oxidative stress on FOXJ1, the studies are less ample. However, there is credible evidence that oxidative stress has a deteriorating effect on FOXJ1 transcript and protein levels as well as on the function of this transcription factor. Therefore, we judge the plausibility of KERF (oxidative stress leading to decreased FOXJ1 protein) to be moderate.
The biological functions of CFTR and FOXJ1 are extensively studied and established across different test systems, implying the high biological plausibility of both KERB (decreased CFTR function leading to decreased ASL height) and KERG (decreased FOXJ1 protein leading to decreased motile cilia length/number). Specifically, CFTR contributes to healthy lung function by regulating epithelial ion conductance to support ASL height maintenance (Boucher, 2003; Csanady et al., 2019), and FOXJ1 is an essential factor for functional multiple motile cilia assembly (Vij et al., 2012; Choksi et al., 2014). Both decreased ASL height (KE2) and decreased motile cilia length/number (KE4) lead to decreased CBF (KE6), as outlined in KERC and KERH, respectively. Multiple studies describe the link between decreased ASL height and reduced CBF. However, the causality between these KEs is not well-established, prompting us to judge KERC as weakly supported. As for KERH, higher numbers of motile cilia with a healthy length support efficient ciliary beating, and a decrease in cilia number and/or length results in a proportionate reduction in CBF. This causal relationship is logical but is directly tested only in few studies. Therefore, we rank the biological plausibility of KERH as moderate. ASL height is also linked to the physical properties of mucus, and studies in models of or individuals with cystic fibrosis support the link between ASL depletion and increased mucus viscosity (KERI), though the overall evidence is sparse, and causality is not always proven. Because the dependencies between these two KEs were highlighted in different species in vitro and in vivo, and the underlying mechanism is well established, we judge the plausibility of KERI as moderate.
Additionally, we propose a direct relationship between oxidative stress and KE6, decreased CBF (KERJ). A variety of oxidants, such as hydrogen peroxide, nitric dioxide, sulfur dioxide, acetaldehyde, ozone, and cigarette smoke decrease CBF in airway epithelial cells in a dose- and time-dependent manner after exposure. This link is demonstrated in several studies in various species, and we judge the plausibility of KERJ as strong. Synchronized ciliary beating helps transport mucus from the distal airways to the mouth, where it is cleared through ingestion or expectoration. In vivo studies and observations in patients with ciliopathies, respiratory infections, or allergies, and following exposure to inhaled toxicants that compromise ciliary function demonstrate that absent, decreased, or asynchronous cilia beating results in defective mucus clearance. Pharmacological studies have demonstrated that CBF stimulation typically results in MCC stimulation. While some results support both a dose-dependent response and temporal sequence of decreased CBF (KE6) leading to decreased MCC (KE7), most studies evaluate these KEs in parallel, and no clear causal linkage is affirmed. The same is true for increased mucus viscosity. Mucus viscoelastic properties, whether altered by airway dehydration or mucus hypersecretion, directly influence MCC. In fact, there is an inverse relationship between mucus viscosity and CBF (KERK) and mucus transport/MCC (KERL), as demonstrated in several in vivo and ex vivo studies. A large proportion of these studies have employed (bio)polymers or other large organic molecules to mimic the mucus layer in the airways and the increase in its viscosity. In addition, some of these studies have shown that decreased mucus viscosity may also result in impairment of MCC. Therefore, a causal link is only tentatively supported. Because cilia function, ASL height, and mucus properties are intricately linked to each other as evidenced by cystic fibrosis studies, we consider the plausibility of KERD, KERK, and KERL as moderate.
Different routes lead to impaired MCC, such as smoking-related oxidative stress, ciliary defects or CFTR mutations. Regardless of the route that leads to a reduction in MCC, individuals with impaired MCC exhibit decreased lung function. Moreover, many pharmacological treatments that enhance MCC also improve lung function. KE7 and the AO are thus closely related; however, as causal evidence is not always available, we judge the biological plausibility of KERE (decreased MCC leading to decreased lung function) as moderate.
The linear AOPs presented here have certain knowledge gaps; however, overall, we consider the biological plausibility of our AOP network as strong, as the network integrates different plausible pathways from the same MIE, leading to a common AO. For example, while oxidative stress leading to decreased lung function through the branch MIE → KE1 → KE2 → KE5 and/or KE6 → KE7 → AO has a weakly supported link represented by KERC (KE2 → KE6, i.e., decreased ASL height leading to decreased CBF), the oxidative stress can lead to the AO also through MIE → KE6 → KE7 → AO or via decreased FOXJ1 protein levels through MIE → KE3 → KE4→ KE5 and/or KE6 → KE7 → AO.
5 Discussion and Conclusion
Here, we have presented an AOP that links oxidative stress resulting from inhalation exposure to toxicants to impaired lung function via a decrease in MCC. Given the individual and public health burden of the consequences of lung function impairment, gaining a greater understanding of the underlying mechanisms of this pathology is extremely important in the risk assessment of inhaled toxic chemicals. There is strong empirical evidence to support several of the KERs in this AOP, particularly at the cellular level (i.e., oxidative stress leading to decreased CFTR function [KERA], decreased CFTR function leading to decreased ASL height [KERB], and oxidative stress leading to decreased CBF [KERJ]). However, additional evidence on causality is required to attribute stronger plausibility to KERs such as that between decreased ASL height and decreased CBF (KERC), which we evaluated as being weak. Future studies, using in vitro assays such as those outlined in Supplementary Table S1, that directly assess this linkage as well as the KERs we determined to have moderate plausibility (i.e., KERD, KERE, KERF, KERH, KERI, KERK, and KERL) will help greatly strengthen this AOP overall.
An integrated assessment of substances with the potential to be inhaled, either intentionally or unintentionally, could incorporate inhalation exposure and dosimetry modelling to inform an in vitro assessment approach with appropriate exposure techniques and cell systems for assessing the KEs in this AOP (EPA’s Office of Chemical Safety and Pollution Prevention, 2019). Standardization and robustness testing of assays against explicit performance criteria using suitable reference materials can greatly increase the level of confidence in their use for KE assessment (Petersen EJ. et al., 2021; Petersen E. J. et al., 2021). Much of the empirical evidence that supports the KERs in the qualitative AOP described here was obtained from in vitro studies using well-established methodologies for biological endpoint assessment (Supplementary Table S1). Being chemical-agnostic, this AOP can be applied to a variety of substances that share the AO. For example, impaired MCC and decreased lung function have a long-known relationship with smoking, but little is known about the consequences of the long-term use of alternative inhaled nicotine delivery products such as electronic cigarettes and heated tobacco products. This AOP can form the basis of an assessment strategy for evaluating the effects of exposure to aerosol from these products on the basis of the KEs identified here.
AOPs such as this one can play a central role in risk assessment strategies for a wide variety of regulatory purposes by providing mechanistic support to an integrated approach to testing and assessment (IATA; (Clippinger et al., 2018)) or defined approach (DA). IATAs are flexible frameworks that can be adapted to best address the regulatory question or purpose at hand. Unlike the assessment process within IATA that involves some level of expert judgement, DA uses rule-based fixed data interpretation procedure. Both DA and IATAs are a means to integrate existing data on a chemical (e.g., physicochemical properties and ADME [absorption, distribution, metabolism, and excretion] information) with an AOP-based in vitro testing strategy to generate data that does not currently exist (Willett, 2019). An important feature of these approaches is that they should also include a measure of uncertainty to facilitate regulatory decision-making. AOPs can be used in an iterative fashion to identify and reduce or resolve, where possible, areas of uncertainty by generating data to fill those knowledge gaps. Furthermore, a quantitative AOP could provide data that would be translated to prediction models for human risk assessment through the application of in vitro to in vivo extrapolation (IVIVE) approaches.
Data Availability Statement
Publicly available datasets were analyzed in this study. This data can be found here: At the point of submission, evidence has been gathered from the literature and compiled prior to populating the AOPwiki at https://aopwiki.org/aops/411, https://aopwiki.org/aops/424 and https://aopwiki.org/aops/425. Special care was taken to properly cite all original studies that were evaluated during AOP development and assessment.
Ethics Statement
Ethical approval was not provided for this study on human participants because the work presented here cites publicly available data (e.g. reports of clinical study results). No new/additional studies in humans was conducted for the purpose of this work. Ethical review and approval was not required for the animal study because this work is based on the review of publicly available data (e.g. scientific articles), some of which came from animal experimentation. No new/additional animal study was conducted for the purpose of the work presented here.
Author Contributions
All authors listed have made significant contribution to the work (literature review and evaluation, development of AOP, synthesis of evidence, draft and revision of manuscript) and approved it for publication.
Funding
PMI is the sole source of funding and sponsor of this research.
Conflict of Interest
KL and HY are employed by Philip Morris International (PMI). MS is employed by PETA International Science Consortium Ltd. DB is employed by British American Tobacco (Investments) Ltd. FJL is employed by Broughton Nicotine Services LLC.
Publisher’s Note
All claims expressed in this article are solely those of the authors and do not necessarily represent those of their affiliated organizations, or those of the publisher, the editors and the reviewers. Any product that may be evaluated in this article, or claim that may be made by its manufacturer, is not guaranteed or endorsed by the publisher.
Acknowledgments
The authors thank Marja Talikka and Rex FitzGerald for their helpful discussions during AOP development. The authors are also grateful to Manuel C. Peitsch, Rex FitzGerald, Amy J. Clippinger, Andreas O. Stucki, and Holger Behrsing for critical review during the preparation of this article and to Sindhoora Bhargavi Gopala Reddy for editorial assistance.
Supplementary Material
The Supplementary Material for this article can be found online at: https://www.frontiersin.org/articles/10.3389/ftox.2021.750254/full#supplementary-material
Footnotes
1The literature interchangeably refers to ASL height, ASL volume and ASL depth. The data presented in original research articles indicate that these terms are synonymous. To simplify the presentation of evidence, we will refer to “ASL height” here.
References
Abedalthagafi, M. S., Wu, M. P., Merrill, P. H., Du, Z., Woo, T., Sheu, S.-H., et al. (2016). Decreased FOXJ1 Expression and its Ciliogenesis Programme in Aggressive Ependymoma and Choroid Plexus Tumours. J. Pathol. 238 (4), 584–597. doi:10.1002/path.4682
Akaike, T., Ando, M., Oda, T., Doi, T., Ijiri, S., Araki, S., et al. (1990). Dependence on O2- Generation by Xanthine Oxidase of Pathogenesis of Influenza Virus Infection in Mice. J. Clin. Invest. 85 (3), 739–745. doi:10.1172/JCI114499
Alexander, N. S., Hatch, N., Zhang, S., Skinner, D., Fortenberry, J., Sorscher, E. J., et al. (2011). Resveratrol Has Salutary Effects on Mucociliary Transport and Inflammation in Sinonasal Epithelium. Laryngoscope 121 (6), 1313–1319. doi:10.1002/lary.21798
Alexander, N. S., Blount, A., Zhang, S., Skinner, D., Hicks, S. B., Chestnut, M., et al. (2012). Cystic Fibrosis Transmembrane Conductance Regulator Modulation by the Tobacco Smoke Toxin Acrolein. Laryngoscope 122 (6), 1193–1197. doi:10.1002/lary.23278
Alexis, N. E., Bennett, W., and Peden, D. B. (2017). Safety and Benefits of Inhaled Hypertonic saline Following Airway Challenges with Endotoxin and Allergen in Asthmatics. J. Asthma 54 (9), 957–960. doi:10.1080/02770903.2016.1278019
Althaus, M. (2013). ENaC Inhibitors and Airway Re-Hydration in Cystic Fibrosis: State of the Art. Curr. Mol. Pharmacol. 6 (1), 3–12. doi:10.2174/18744672112059990025
Amin, R., Subbarao, P., Lou, W., Jabar, A., Balkovec, S., Jensen, R., et al. (2011). The Effect of Dornase Alfa on Ventilation Inhomogeneity in Patients with Cystic Fibrosis. Eur. Respir. J. 37 (4), 806–812. doi:10.1183/09031936.00072510
Andrade, Y. N., Fernandes, J., Vázquez, E., Fernández-Fernández, J. M., Arniges, M., Sánchez, T. M., et al. (2005). TRPV4 Channel Is Involved in the Coupling of Fluid Viscosity Changes to Epithelial Ciliary Activity. J. Cel Biol. 168 (6), 869–874. doi:10.1083/jcb.200409070
Ankley, G. T., Bennett, R. S., Erickson, R. J., Hoff, D. J., Hornung, M. W., Johnson, R. D., et al. (2010). Adverse Outcome Pathways: a Conceptual Framework to Support Ecotoxicology Research and Risk Assessment. Environ. Toxicol. Chem. 29 (3), 730–741. doi:10.1002/etc.34
Antunes, M. B., and Cohen, N. A. (2007). Mucociliary Clearance - a Critical Upper Airway Host Defense Mechanism and Methods of Assessment. Curr. Opin. Allergy Clin. Immunol. 7 (1), 5–10. doi:10.1097/aci.0b013e3280114eef
Arbi, M., Pefani, D. E., Kyrousi, C., Lalioti, M. E., Kalogeropoulou, A., Papanastasiou, A. D., et al. (2016). GemC1 Controls Multiciliogenesis in the Airway Epithelium. EMBO Rep. 17 (3), 400–413. doi:10.15252/embr.201540882
Åstrand, A. B., Hemmerling, M., Root, J., Wingren, C., Pesic, J., Johansson, E., et al. (2014). Linking Increased Airway Hydration, Ciliary Beating, and Mucociliary Clearance through ENaC Inhibition. Am. J. Physiol. Lung Cel. Mol. Physiol. 308 (1), L22–L32. doi:10.1152/ajplung.00163.2014
Azbell, C., Zhang, S., Skinner, D., Fortenberry, J., Sorscher, E. J., and Woodworth, B. A. (2010). Hesperidin Stimulates Cystic Fibrosis Transmembrane Conductance Regulator-Mediated Chloride Secretion and Ciliary Beat Frequency in Sinonasal Epithelium. Otolaryngol. Head Neck Surg. 143 (3), 397–404. doi:10.1016/j.otohns.2010.05.021
Bargon, J., Trapnell, B. C., Yoshimura, K., Dalemans, W., Pavirani, A., Lecocq, J. P., et al. (1992a). Expression of the Cystic Fibrosis Transmembrane Conductance Regulator Gene Can Be Regulated by Protein Kinase C. J. Biol. Chem. 267 (23), 16056–16060. doi:10.1016/s0021-9258(18)41964-3
Bargon, J., Trapnell, B. C., Chu, C. S., Rosenthal, E. R., Yoshimura, K., Guggino, W. B., et al. (1992b). Down-regulation of Cystic Fibrosis Transmembrane Conductance Regulator Gene Expression by Agents that Modulate Intracellular Divalent Cations. Mol. Cel. Biol. 12 (4), 1872–1878. doi:10.1128/mcb.12.4.1872-1878.1992
Berlett, B. S., and Stadtman, E. R. (1997). Protein Oxidation in Aging, Disease, and Oxidative Stress. J. Biol. Chem. 272 (33), 20313–20316. doi:10.1074/jbc.272.33.20313
Bernard, M. E., Kim, H., Rajagopalan, M. S., Stone, B., Salimi, U., Rwigema, J. C., et al. (2012). Repopulation of the Irradiation Damaged Lung with Bone Marrow-Derived Cells. In Vivo 26 (1), 9–18.
Berta, T., Gabriele, P., Sandra, S.-B., Gabriel, G.-G., Youssef, S. A., Stephan-Otto, A. C., et al. (2016). GEMC1 Is a Critical Regulator of Multiciliated Cell Differentiation. EMBO J. 35 (9), 942–960. doi:10.15252/embj.201592821
Birket, S. E., Chu, K. K., Liu, L., Houser, G. H., Diephuis, B. J., Wilsterman, E. J., et al. (2014). A Functional Anatomic Defect of the Cystic Fibrosis Airway. Am. J. Respir. Crit. Care Med. 190 (4), 421–432. doi:10.1164/rccm.201404-0670oc
Birket, S. E., Chu, K. K., Houser, G. H., Liu, L., Fernandez, C. M., Solomon, G. M., et al. (2016). Combination Therapy with Cystic Fibrosis Transmembrane Conductance Regulator Modulators Augment the Airway Functional Microanatomy. Am. J. Physiol. Lung Cell Mol. Physiol. 310 (10), L928–L939. doi:10.1152/ajplung.00395.2015
Birket, S. E., Davis, J. M., Fernandez, C. M., Tuggle, K. L., Oden, A. M., Chu, K. K., et al. (2018). Development of an Airway Mucus Defect in the Cystic Fibrosis Rat. JCI Insight 3 (1), e97199. doi:10.1172/jci.insight.97199
Bodas, M., Silverberg, D., Walworth, K., Brucia, K., and Vij, N. (2017). Augmentation of S-Nitrosoglutathione Controls Cigarette Smoke-Induced Inflammatory-Oxidative Stress and Chronic Obstructive Pulmonary Disease-Emphysema Pathogenesis by Restoring Cystic Fibrosis Transmembrane Conductance Regulator Function. Antioxid. Redox Signal. 27 (7), 433–451. doi:10.1089/ars.2016.6895
Boek, W. M., Keleş, N., Graamans, K., and Huizing, E. H. (1999). Physiologic and Hypertonic saline Solutions Impair Ciliary Activity In Vitro. Laryngoscope 109 (3), 396–399. doi:10.1097/00005537-199903000-00010
Boek, W. M., Graamans, K., Natzijl, H., van Rijk, P. P., and Huizing, E. H. (2002). Nasal Mucociliary Transport: New Evidence for a Key Role of Ciliary Beat Frequency. Laryngoscope 112 (3), 570–573. doi:10.1097/00005537-200203000-00029
Bottier, M., Thomas, K. A., Dutcher, S. K., and Bayly, P. V. (2019). How Does Cilium Length Affect Beating? Biophys. J. 116 (7), 1292–1304. doi:10.1016/j.bpj.2019.02.012
Boucher, R. (2003). Regulation of Airway Surface Liquid Volume by Human Airway Epithelia. Pflugers Arch. - Eur. J. Physiol. 445 (4), 495–498. doi:10.1007/s00424-002-0955-1
Boucher, R. C. (2004). New Concepts of the Pathogenesis of Cystic Fibrosis Lung Disease. Eur. Respir. J. 23 (1), 146–158. doi:10.1183/09031936.03.00057003
Braun, A. P. (2014). Cigarette Smoke and Calcium Conspire to Impair CFTR Function in Airway Epithelia. Channels 8 (3), 172–173. doi:10.4161/chan.28970
Brézillon, S., Zahm, J.-M., Pierrot, D., Gaillard, D., Hinnrasky, J., Millart, H., et al. (1997). ATP Depletion Induces a Loss of Respiratory Epithelium Functional Integrity and Down-Regulates CFTR (Cystic Fibrosis Transmembrane Conductance Regulator) Expression. J. Biol. Chem. 272 (44), 27830–27838. doi:10.1074/jbc.272.44.27830
Brekman, A., Walters, M. S., Tilley, A. E., and Crystal, R. G. (2014). FOXJ1 Prevents Cilia Growth Inhibition by Cigarette Smoke in Human Airway EpitheliumIn Vitro. Am. J. Respir. Cel Mol. Biol. 51 (5), 688–700. doi:10.1165/rcmb.2013-0363oc
Brody, S. L., Yan, X. H., Wuerffel, M. K., Song, S.-K., and Shapiro, S. D. (2000). Ciliogenesis and Left-Right axis Defects in Forkhead Factor HFH-4-Null Mice. Am. J. Respir. Cel Mol. Biol. 23 (1), 45–51. doi:10.1165/ajrcmb.23.1.4070
Brooks, E. R., and Wallingford, J. B. (2014). Multiciliated Cells. Curr. Biol. 24 (19), R973–R982. doi:10.1016/j.cub.2014.08.047
Burman, W. J., and Martin, W. J. (1986). Oxidant-Mediated Ciliary Dysfunction. Chest 89 (3), 410–413. doi:10.1378/chest.89.3.410
Bustamante-Marin, X. M., and Ostrowski, L. E. (2017). Cilia and Mucociliary Clearance. Cold Spring Harb. Perspect. Biol. 9 (4), a028241. doi:10.1101/cshperspect.a028241
Button, B., Cai, L.-H., Ehre, C., Kesimer, M., Hill, D. B., Sheehan, J. K., et al. (2012). A Periciliary brush Promotes the Lung Health by Separating the Mucus Layer from Airway Epithelia. Science 337 (6097), 937–941. doi:10.1126/science.1223012
Cantin, A. M., Bilodeau, G., Ouellet, C., Liao, J., and Hanrahan, J. W. (2006a). Oxidant Stress Suppresses CFTR Expression. Am. J. Physiology-Cell Physiol. 290 (1), C262–C270. doi:10.1152/ajpcell.00070.2005
Cantin, A. M., Hanrahan, J. W., Bilodeau, G., Ellis, L., Dupuis, A., Liao, J., et al. (2006b). Cystic Fibrosis Transmembrane Conductance Regulator Function Is Suppressed in Cigarette Smokers. Am. J. Respir. Crit. Care Med. 173 (10), 1139–1144. doi:10.1164/rccm.200508-1330OC
Chabot, F., Mitchell, J. A., Gutteridge, J. M., and Evans, T. W. (1998). Reactive Oxygen Species in Acute Lung Injury. Eur. Respir. J. 11 (3), 745–757.
Chen, J., Knowles, H. J., Hebert, J. L., and Hackett, B. P. (1998). Mutation of the Mouse Hepatocyte Nuclear Factor/forkhead Homologue 4 Gene Results in an Absence of Cilia and Random Left-Right Asymmetry. J. Clin. Invest. 102 (6), 1077–1082. doi:10.1172/JCI4786
Chilvers, M. A., and O'Callaghan, C. (2000). Analysis of Ciliary Beat Pattern and Beat Frequency Using Digital High Speed Imaging: Comparison with the Photomultiplier and Photodiode Methods. Thorax 55 (4), 314–317. doi:10.1136/thorax.55.4.314
Chinnapaiyan, S., Dutta, R., Bala, J., Parira, T., Agudelo, M., Nair, M., et al. (2018). Cigarette Smoke Promotes HIV Infection of Primary Bronchial Epithelium and Additively Suppresses CFTR Function. Sci. Rep. 8, 7984. doi:10.1038/s41598-018-26095-z
Choksi, S. P., Lauter, G., Swoboda, P., and Roy, S. (2014). Switching on Cilia: Transcriptional Networks Regulating Ciliogenesis. Development 141 (7), 1427–1441. doi:10.1242/dev.074666
Church, D. F., and Pryor, W. A. (1985). Free-radical Chemistry of Cigarette Smoke and its Toxicological Implications. Environ. Health Perspect. 64, 111–126. doi:10.1289/ehp.8564111
Clary-Meinesz, C., Mouroux, J., Cosson, J., Huitorel, P., and Blaive, B. (1998). Influence of External pH on Ciliary Beat Frequency in Human Bronchi and Bronchioles. Eur. Respir. J. 11 (2), 330–333. doi:10.1183/09031936.98.11020330
Clippinger, A. J., Allen, D., Behrsing, H., BéruBé, K. A., Bolger, M. B., Casey, W., et al. (2018). Pathway-based Predictive Approaches for Non-animal Assessment of Acute Inhalation Toxicity. Toxicol. Vitro 52, 131–145. doi:10.1016/j.tiv.2018.06.009
Clunes, L. A., Davies, C. M., Coakley, R. D., Aleksandrov, A. A., Henderson, A. G., Zeman, K. L., et al. (2012). Cigarette Smoke Exposure Induces CFTR Internalization and Insolubility, Leading to Airway Surface Liquid Dehydration. FASEB J. 26 (2), 533–545. doi:10.1096/fj.11-192377
Cohen, N. A., Zhang, S., Sharp, D. B., Tamashiro, E., Chen, B., Sorscher, E. J., et al. (2009). Cigarette Smoke Condensate Inhibits Transepithelial Chloride Transport and Ciliary Beat Frequency. Laryngoscope 119 (11), 2269–2274. doi:10.1002/lary.20223
Conger, B. T., Zhang, S., Skinner, D., Hicks, S. B., Sorscher, E. J., Rowe, S. M., et al. (2013). Comparison of Cystic Fibrosis Transmembrane Conductance Regulator (CFTR) and Ciliary Beat Frequency Activation by the CFTR Modulators Genistein, VRT-532, and UCCF-152 in Primary Sinonasal Epithelial Cultures. JAMA Otolaryngol. Head Neck Surg. 139 (8), 822–827. doi:10.1001/jamaoto.2013.3917
Courville, C. A., Tidwell, S., Liu, B., Accurso, F. J., Dransfield, M. T., and Rowe, S. M. (2014). Acquired Defects in CFTR-dependent β-Adrenergic Sweat Secretion in Chronic Obstructive Pulmonary Disease. Respir. Res. 15 (1), 25. doi:10.1186/1465-9921-15-25
Csanády, L., Vergani, P., and Gadsby, D. C. (2019). Structure, Gating, and Regulation of the Cftr Anion Channel. Physiol. Rev. 99 (1), 707–738. doi:10.1152/physrev.00007.2018
Denizoglu Kulli, H., Gurses, H. N., Zeren, M., Ucgun, H., and Cakir, E. (2020). Do pulmonary and Extrapulmonary Features Differ Among Cystic Fibrosis, Primary Ciliary Dyskinesia, and Healthy Children? Pediatr. Pulmonol. 55 (11), 3067–3073. doi:10.1002/ppul.25052
Derichs, N. (2013). Targeting a Genetic Defect: Cystic Fibrosis Transmembrane Conductance Regulator Modulators in Cystic Fibrosis. Eur. Respir. Rev. 22 (127), 58–65. doi:10.1183/09059180.00008412
Donaldson, S. H., Laube, B. L., Corcoran, T. E., Bhambhvani, P., Zeman, K., Ceppe, A., et al. (2018). Effect of Ivacaftor on Mucociliary Clearance and Clinical Outcomes in Cystic Fibrosis Patients with G551D-CFTR. JCI Insight 3 (24), e122695. doi:10.1172/jci.insight.122695
Dransfield, M. T., Wilhelm, A. M., Flanagan, B., Courville, C., Tidwell, S. L., Raju, S. V., et al. (2013). Acquired Cystic Fibrosis Transmembrane Conductance Regulator Dysfunction in the Lower Airways in COPD. Chest 144 (2), 498–506. doi:10.1378/chest.13-0274
Dunican, E. M., Elicker, B. M., Henry, T., Gierada, D. S., Schiebler, M. L., Anderson, W., et al. (2021). Mucus Plugs and Emphysema in the Pathophysiology of Airflow Obstruction and Hypoxemia in Smokers. Am. J. Respir. Crit. Care Med. 203 (8), 957–968. doi:10.1164/rccm.202006-2248oc
Elkins, M. R., Robinson, M., Rose, B. R., Harbour, C., Moriarty, C. P., Marks, G. B., et al. (2006). A Controlled Trial of Long-Term Inhaled Hypertonic Saline in Patients with Cystic Fibrosis. N. Engl. J. Med. 354 (3), 229–240. doi:10.1056/NEJMoa043900
EPA’s Office of Chemical Safety and Pollution Prevention (2019). FIFRA Scientific Advisory Panel Meeting Minutes and Final Report No. 2019-01 Peer Review on Evaluation of a Proposed Approach to Refine the Inhalation Risk Assessment for Point of Contact Toxicity: A Case Study Using a New Approach Methodology (NAM) December 4 and 6, 2018 FIFRA Scientific Advisory Panel Meeting. Washington, DC: U.S. Environmental Protection Agency.
Fahy, J. V., and Dickey, B. F. (2010). Airway Mucus Function and Dysfunction. N. Engl. J. Med. 363 (23), 2233–2247. doi:10.1056/NEJMra0910061
Farinha, C. M., Matos, P., and Amaral, M. D. (2013). Control of Cystic Fibrosis Transmembrane Conductance Regulator Membrane Trafficking: Not Just from the Endoplasmic Reticulum to the Golgi. FEBS J. 280 (18), 4396–4406. doi:10.1111/febs.12392
Feldman, C., Anderson, R., Kanthakumar, K., Vargas, A., Cole, P. J., and Wilson, R. (1994). Oxidant-mediated Ciliary Dysfunction in Human Respiratory Epithelium. Free Radic. Biol. Med. 17 (1), 1–10. doi:10.1016/0891-5849(94)90002-7
Fernandez-Petty, C. M., Hughes, G. W., Bowers, H. L., Watson, J. D., Rosen, B. H., Townsend, S. M., et al. (2019). A Glycopolymer Improves Vascoelasticity and Mucociliary Transport of Abnormal Cystic Fibrosis Mucus. JCI Insight 4 (8), e125954. doi:10.1172/jci.insight.125954
Ferreira, A. D., Ramos, E. M. C., Trevisan, I. B., Leite, M. R., Proença, M., de Carvalho-Junior, L. C. S., et al. (2018). Função pulmonar e depuração mucociliar nasal de cortadores de cana-de-açúcar brasileiros expostos à queima de biomassa. Rev. Bras. Saúde Ocup. 43, e6. doi:10.1590/2317-6369000004217
Fischer, H., and Widdicombe, J. H. (2006). Mechanisms of Acid and Base Secretion by the Airway Epithelium. J. Membr. Biol. 211 (3), 139–150. doi:10.1007/s00232-006-0861-0
Fló-Neyret, C., Lorenzi-Filho, G., Macchione, M., Garcia, M. L., and Saldiva, P. H. (2001). Effects of Formaldehyde on the Frog's Mucociliary Epithelium as a Surrogate to Evaluate Air Pollution Effects on the Respiratory Epithelium. Braz. J. Med. Biol. Res. 34, 639–643. doi:10.1590/s0100-879x2001000500012
Fröhlich, E., Mercuri, A., Wu, S., and Salar-Behzadi, S. (2016). Measurements of Deposition, Lung Surface Area and Lung Fluid for Simulation of Inhaled Compounds. Front. Pharmacol. 7, 181. doi:10.3389/fphar.2016.00181
Garcia-Arcos, I., Geraghty, P., Baumlin, N., Campos, M., Dabo, A. J., Jundi, B., et al. (2016). Chronic Electronic Cigarette Exposure in Mice Induces Features of COPD in a Nicotine-dependent Manner. Thorax 71 (12), 1119–1129. doi:10.1136/thoraxjnl-2015-208039
Geiler, J., Michaelis, M., Naczk, P., Leutz, A., Langer, K., Doerr, H.-W., et al. (2010). N-acetyl-L-cysteine (NAC) Inhibits Virus Replication and Expression of Pro-inflammatory Molecules in A549 Cells Infected with Highly Pathogenic H5N1 Influenza A Virus. Biochem. Pharmacol. 79 (3), 413–420. doi:10.1016/j.bcp.2009.08.025
Gerber, V., King, M., Schneider, D. A., and Robinson, N. E. (2000). Tracheobronchial Mucus Viscoelasticity during Environmental challenge in Horses with Recurrent Airway Obstruction. Equine Vet. J. 32 (5), 411–417. doi:10.2746/042516400777591183
Gerovac, B. J., and Fregien, N. L. (2016). IL-13 Inhibits Multicilin Expression and Ciliogenesis via Janus Kinase/signal Transducer and Activator of Transcription Independently of Notch Cleavage. Am. J. Respir. Cel Mol. Biol. 54 (4), 554–561. doi:10.1165/rcmb.2015-0227oc
Gerovac, B. J., Valencia, M., Baumlin, N., Salathe, M., Conner, G. E., and Fregien, N. L. (2014). Submersion and Hypoxia Inhibit Ciliated Cell Differentiation in a Notch-dependent Manner. Am. J. Respir. Cel Mol. Biol. 51 (4), 516–525. doi:10.1165/rcmb.2013-0237OC
Gheber, L., Korngreen, A., and Priel, Z. (1998). Effect of Viscosity on Metachrony in Mucus Propelling Cilia. Cell Motil. Cytoskeleton 39 (1), 9–20.
Ghosh, A., Abdelwahab, S. H., Reeber, S. L., Reidel, B., Marklew, A. J., Garrison, A. J., et al. (2017). Little Cigars Are More Toxic Than Cigarettes and Uniquely Change the Airway Gene and Protein Expression. Sci. Rep. 7, 46239. doi:10.1038/srep46239
Gomperts, B. N., Gong-Cooper, X., and Hackett, B. P. (2004). Foxj1 Regulates Basal Body Anchoring to the Cytoskeleton of Ciliated Pulmonary Epithelial Cells. J. Cel Sci. 117 (Pt 8), 1329–1337. doi:10.1242/jcs.00978
Gomperts, B. N., Kim, L. J., Flaherty, S. A., and Hackett, B. P. (2007). IL-13 Regulates Cilia Loss and Foxj1 Expression in Human Airway Epithelium. Am. J. Respir. Cel Mol. Biol. 37 (3), 339–346. doi:10.1165/rcmb.2006-0400OC
González, C., Droguett, K., Rios, M., Cohen, N. A., and Villalón, M. (2016). TNFα Affects Ciliary Beat Response to Increased Viscosity in Human Pediatric Airway Epithelium. Biomed. Res. Int. 2016, 3628501. doi:10.1155/2016/3628501
Halliwell, B., and Aruoma, O. I. (1991). DNA Damage by Oxygen-Derived Species. Its Mechanism and Measurement in Mammalian Systems. FEBS Lett. 281 (1-2), 9–19. doi:10.1016/0014-5793(91)80347-6
Hassan, F., Xu, X., Nuovo, G., Killilea, D. W., Tyrrell, J., Da Tan, C., et al. (2014). Accumulation of Metals in GOLD4 COPD Lungs Is Associated with Decreased CFTR Levels. Respir. Res. 15 (1), 69. doi:10.1186/1465-9921-15-69
Ho, J. C., Chan, K. N., Hu, W. H., Lam, W. K., Zheng, L., Tipoe, G. L., et al. (2001). The Effect of Aging on Nasal Mucociliary Clearance, Beat Frequency, and Ultrastructure of Respiratory Cilia. Am. J. Respir. Crit. Care Med. 163 (4), 983–988. doi:10.1164/ajrccm.163.4.9909121
Hobbs, C. A., Blanchard, M. G., Alijevic, O., Tan, C. D., Kellenberger, S., Bencharit, S., et al. (2013). Identification of the SPLUNC1 ENaC-Inhibitory Domain Yields Novel Strategies to Treat Sodium Hyperabsorption in Cystic Fibrosis Airway Epithelial Cultures. Am. J. Physiology-Lung Cell Mol. Physiol. 305 (12), L990–L1001. doi:10.1152/ajplung.00103.2013
Holland, J. A., Pritchard, K. A., Pappolla, M. A., Wolin, M. S., Rogers, N. J., and Stemerman, M. B. (1990). Bradykinin Induces Superoxide Anion Release from Human Endothelial Cells. J. Cel. Physiol. 143 (1), 21–25. doi:10.1002/jcp.1041430104
Hollenhorst, M. I., Richter, K., and Fronius, M. (2011). Ion Transport by Pulmonary Epithelia. Biomed. Res. Int. 2011, 174306. doi:10.1155/2011/174306
Hua, X., Zeman, K. L., Zhou, B., Hua, Q., Senior, B. A., Tilley, S. L., et al. (2010). Noninvasive Real-Time Measurement of Nasal Mucociliary Clearance in Mice by Pinhole Gamma Scintigraphy. J. Appl. Physiol. 108 (1), 189–196. doi:10.1152/japplphysiol.00669.2009
Ishikawa, S., and Ito, S. (2017). Repeated Whole Cigarette Smoke Exposure Alters Cell Differentiation and Augments Secretion of Inflammatory Mediators in Air-Liquid Interface Three-Dimensional Co-culture Model of Human Bronchial Tissue. Toxicol. Vitro 38, 170–178. doi:10.1016/j.tiv.2016.09.004
Ito, J. T., Ramos, D., Lima, F. F., Rodrigues, F. M., Gomes, P. R., Moreira, G. L., et al. (2015). Nasal Mucociliary Clearance in Subjects with COPD after Smoking Cessation. Respir. Care 60 (3), 399–405. doi:10.4187/respcare.03266
Jayathilake, P. G., Tan, Z., Le, D. V., Lee, H. P., and Khoo, B. C. (2012). Three-dimensional Numerical Simulations of Human Pulmonary Cilia in the Periciliary Liquid Layer by the Immersed Boundary Method. Comput. Fluids 67, 130–137. doi:10.1016/j.compfluid.2012.07.016
Jayathilake, P. G., Le, D. V., Tan, Z., Lee, H. P., and Khoo, B. C. (2015). A Numerical Study of Muco-Ciliary Transport under the Condition of Diseased Cilia. Comput. Methods Biomech. Biomed. Eng. 18 (9), 944–951. doi:10.1080/10255842.2013.864285
Jing, J. C., Chen, J. J., Chou, L., Wong, B. J. F., and Chen, Z. (2017). Visualization and Detection of Ciliary Beating Pattern and Frequency in the Upper Airway Using Phase Resolved Doppler Optical Coherence Tomography. Sci. Rep. 7 (1), 8522. doi:10.1038/s41598-017-08968-x
Johansen, H. K., Nir, M., Koch, C., Schwartz, M., and Høiby, N. (1991). Severity of Cystic Fibrosis in Patients Homozygous and Heterozygous for ΔF508 Mutation. Lancet 337 (8742), 631–634. doi:10.1016/0140-6736(91)92449-c
Joki, S., Toskala, E., Saano, V., and Nuutinen, J. (1998). Correlation between Ciliary Beat Frequency and the Structure of Ciliated Epithelia in Pathologic Human Nasal Mucosa. Laryngoscope 108 (3), 426–430. doi:10.1097/00005537-199803000-00021
Kakinoki, Y., Tanaka, A., Washio, Y., Yamada, K., Nakai, Y., Morimoto, K., et al. (1998). Nitrogen Dioxide Compromises Defence Functions of the Airway Epithelium. Acta Oto-Laryngol. 118 (538), 221–226. doi:10.1080/00016489850182972
Kanthakumar, K., Taylor, G. W., Cundell, D. R., Dowling, R. B., Johnson, M., Cole, P. J., et al. (1996). The Effect of Bacterial Toxins on Levels of Intracellular Adenosine Nucleotides and Human Ciliary Beat Frequency. Pulm. Pharmacol. 9 (4), 223–230. doi:10.1006/pulp.1996.0028
Kerem, E., Corey, M., Kerem, B.-s., Rommens, J., Markiewicz, D., Levison, H., et al. (1990). The Relation between Genotype and Phenotype in Cystic Fibrosis - Analysis of the Most Common Mutation (ΔF508). N. Engl. J. Med. 323 (22), 1517–1522. doi:10.1056/nejm199011293232203
Kerem, E., Viviani, L., Zolin, A., MacNeill, S., Hatziagorou, E., Ellemunter, H., et al. (2014). Factors Associated with FEV1 Decline in Cystic Fibrosis: Analysis of the ECFS Patient Registry. Eur. Respir. J. 43 (1), 125–133. doi:10.1183/09031936.00166412
Kikuchi, K., Haga, T., Numayama-Tsuruta, K., Ueno, H., and Ishikawa, T. (2017). Effect of Fluid Viscosity on the Cilia-Generated Flow on a Mouse Tracheal Lumen. Ann. Biomed. Eng. 45 (4), 1048–1057. doi:10.1007/s10439-016-1743-y
Kilgour, E., Rankin, N., Ryan, S., and Pack, R. (2004). Mucociliary Function Deteriorates in the Clinical Range of Inspired Air Temperature and Humidity. Int. Care Med. 30 (7), 1491–1494. doi:10.1007/s00134-004-2235-3
King, M. (1979). Interrelation between Mechanical Properties of Mucus and Mucociliary Transport: Effect of Pharmacologic Interventions. Biorheology 16 (1-2), 57–68. doi:10.3233/bir-1979-161-210
King, M. (2006). Physiology of Mucus Clearance. Paediatric Respir. Rev. 7 (Suppl. 1), S212–S214. doi:10.1016/j.prrv.2006.04.199
Kinnula, V. L., Chang, L., Everitt, J. I., and Crapo, J. D. (1992). Oxidants and Antioxidants in Alveolar Epithelial Type II Cells: In Situ, Freshly Isolated, and Cultured Cells. Am. J. Physiol.-Lung Cell Mol. Physiol. 262 (1 Pt 1), L69–L77. doi:10.1152/ajplung.1992.262.1.L69
Kinnula, V. L., Crapo, J. D., and Raivio, K. O. (1995). Generation and Disposal of Reactive Oxygen Metabolites in the Lung. Lab. Invest. 73 (1), 3–19.
Knorst, M. M., Kienast, K., Riechelmann, H., Müller-Quernheim, J., and Ferlinz, R. (1994). Effect of Sulfur Dioxide on Mucociliary Activity and Ciliary Beat Frequency in guinea Pig Trachea. Int. Arch. Occup. Environ. Heath 65 (5), 325–328. doi:10.1007/BF00405697
Knowles, M. R., and Boucher, R. C. (2002). Mucus Clearance as a Primary Innate Defense Mechanism for Mammalian Airways. J. Clin. Invest. 109 (5), 571–577. doi:10.1172/jci0215217
Kulka, M., Dery, R., Nahirney, D., Duszyk, M., and Befus, A. D. (2005). Differential Regulation of Cystic Fibrosis Transmembrane Conductance Regulator by Interferon γ in Mast Cells and Epithelial Cells. J. Pharmacol. Exp. Ther. 315 (2), 563–570. doi:10.1124/jpet.105.087528
Kuyper, L. M., Paré, P. D., Hogg, J. C., Lambert, R. K., Ionescu, D., Woods, R., et al. (2003). Characterization of Airway Plugging in Fatal Asthma. Am. J. Med. 115 (1), 6–11. doi:10.1016/s0002-9343(03)00241-9
Lai, S. K., Wang, Y.-Y., Wirtz, D., and Hanes, J. (2009). Micro- and Macrorheology of Mucus. Adv. Drug Deliv. Rev. 61 (2), 86–100. doi:10.1016/j.addr.2008.09.012
Lai, Y., Dilidaer, D., Chen, B., Xu, G., Shi, J., Lee, R. J., et al. (2014). In Vitro studies of a Distillate of Rectified Essential Oils on Sinonasal Components of Mucociliary Clearance. Am. J. Rhinol Allergy 28 (3), 244–248. doi:10.2500/ajra.2014.28.4036
Lambert, J. A., Raju, S. V., Tang, L. P., McNicholas, C. M., Li, Y., Courville, C. A., et al. (2014). Cystic Fibrosis Transmembrane Conductance Regulator Activation by Roflumilast Contributes to Therapeutic Benefit in Chronic Bronchitis. Am. J. Respir. Cel Mol. Biol. 50 (3), 549–558. doi:10.1165/rcmb.2013-0228oc
Laube, B. L., Auci, R. M., Shields, D. E., Christiansen, D. H., Lucas, M. K., Fuchs, H. J., et al. (1996). Effect of rhDNase on Airflow Obstruction and Mucociliary Clearance in Cystic Fibrosis. Am. J. Respir. Crit. Care Med. 153 (2), 752–760. doi:10.1164/ajrccm.153.2.8564129
Lee, W. L., Jayathilake, P. G., Tan, Z., Le, D. V., Lee, H. P., and Khoo, B. C. (2011). Muco-ciliary Transport: Effect of Mucus Viscosity, Cilia Beat Frequency and Cilia Density. Comput. Fluids 49 (1), 214–221. doi:10.1016/j.compfluid.2011.05.016
Lin, V. Y., Kaza, N., Birket, S. E., Kim, H., Edwards, L. J., LaFontaine, J., et al. (2020). Excess Mucus Viscosity and Airway Dehydration Impact COPD Airway Clearance. Eur. Respir. J. 55 (1), 1900419. doi:10.1183/13993003.00419-2019
Liu, M., Guan, Z., Shen, Q., Lalor, P., Fitzgerald, U., O'Brien, T., et al. (2016). Ulk4 Is Essential for Ciliogenesis and CSF Flow. J. Neurosci. 36 (29), 7589–7600. doi:10.1523/jneurosci.0621-16.2016
Macchione, M., Oliveira, A. P., Gallafrio, C. T., Muchão, F. P., Obara, M. T., Guimarães, E. T., et al. (1999). Acute Effects of Inhalable Particles on the Frog Palate Mucociliary Epithelium. Environ. Health Perspect. 107 (10), 829–833. doi:10.1289/ehp.99107829
Mall, M., Grubb, B. R., Harkema, J. R., O'Neal, W. K., and Boucher, R. C. (2004). Increased Airway Epithelial Na+ Absorption Produces Cystic Fibrosis-like Lung Disease in Mice. Nat. Med. 10 (5), 487–493. doi:10.1038/nm1028
Mall, M. A. (2008). Role of Cilia, Mucus, and Airway Surface Liquid in Mucociliary Dysfunction: Lessons from Mouse Models. J. Aerosol Med. Pulm. Drug Deliv. 21 (1), 13–24. doi:10.1089/jamp.2007.0659
Mata, M., Sarrion, I., Armengot, M., Carda, C., Martinez, I., Melero, J. A., et al. (2012). Respiratory Syncytial Virus Inhibits Ciliagenesis in Differentiated Normal Human Bronchial Epithelial Cells: Effectiveness of N-Acetylcysteine. PloS one 7 (10), e48037. doi:10.1371/journal.pone.0048037
Matsui, H., Grubb, B. R., Tarran, R., Randell, S. H., Gatzy, J. T., Davis, C. W., et al. (1998). Evidence for Periciliary Liquid Layer Depletion, Not Abnormal Ion Composition, in the Pathogenesis of Cystic Fibrosis Airways Disease. Cell 95 (7), 1005–1015. doi:10.1016/s0092-8674(00)81724-9
Maxwell, G. M. (1985). The Problem of Mucus Plugging in Children with Asthma. J. Asthma 22 (3), 131–137. doi:10.3109/02770908509073131
McCoy, K., Hamilton, S., and Johnson, C. (1996). Effects of 12-Week Administration of Dornase Alfa in Patients with Advanced Cystic Fibrosis Lung Disease. Chest 110 (4), 889–895. doi:10.1378/chest.110.4.889
Milara, J., Armengot, M., Bañuls, P., Tenor, H., Beume, R., Artigues, E., et al. (2012). Roflumilast N-Oxide, a PDE4 Inhibitor, Improves Cilia Motility and Ciliated Human Bronchial Epithelial Cells Compromised by Cigarette Smoke In Vitro. Br. J. Pharmacol. 166 (8), 2243–2262. doi:10.1111/j.1476-5381.2012.01929.x
Min, Y.-G., Ohyama, M., Lee, K. S., Rhee, C.-S., Ha Oh, S., Sung, M.-W., et al. (1999). Effects of Free Radicals on Ciliary Movement in the Human Nasal Epithelial Cells. Auris Nasus Larynx 26 (2), 159–163. doi:10.1016/s0385-8146(98)00073-x
Morgan, K. T., Patterson, D. L., and Gross, E. A. (1984). Frog Palate Mucociliary Apparatus: Structure, Function, and Response to Formaldehyde Gas. Toxicol. Sci. 4 (1), 58–68. doi:10.1093/toxsci/4.1.58
Morgan, K. T., Patterson, D. L., and Gross, E. A. (1986). Responses of the Nasal Mucociliary Apparatus of F-344 Rats to Formaldehyde Gas. Toxicol. Appl. Pharmacol. 82 (1), 1–13. doi:10.1016/0041-008x(86)90431-x
Mossberg, B., Afzelius, B. A., Eliasson, R., and Camner, P. (1978). On the Pathogenesis of Obstructive Lung Disease. A Study on the Immotile-Cilia Syndrome. Scand. J. Respir. Dis. 59 (2), 55–65.
Munkholm, M., and Mortensen, J. (2014). Mucociliary Clearance: Pathophysiological Aspects. Clin. Physiol. Funct. Imaging 34 (3), 171–177. doi:10.1111/cpf.12085
National Research Council (1988). Air Pollution, the Automobile, and Public Health. Washington, DC: The National Academies Press.
OECD (2017). “Revised Guidance Document on Developing and Assessing Adverse Outcome Pathways,” in Series on Testing and Assessment (Paris: Organisation for Economic Cooperation and Devleopment).
OECD (2018). “Users' Handbook Supplement to the Guidance Document for Developing and Assessing Adverse Outcome Pathways,” in OECD Series on Adverse Outcome Pathways (Paris: OECD).
OECD (2021). Guideline No. 497: Defined Approaches on Skin Sensitisation. Paris, France: OECD Publishing.
Okajima, Y., Come, C. E., Nardelli, P., Sonavane, S. K., Yen, A., Nath, H. P., et al. (2020). Luminal Plugging on Chest CT Scan. Chest 158 (1), 121–130. doi:10.1016/j.chest.2019.12.046
Petersen, E. J., Nguyen, A., Brown, J., Elliott, J. T., Clippinger, A., Gordon, J., et al. (2021a). Characteristics to Consider when Selecting a Positive Control Material for an In Vitro Assay. Altex 38 (2), 365–376. doi:10.14573/altex.2102111
Petersen, E. J., Sharma, M., Clippinger, A. J., Gordon, J., Katz, A., Laux, P., et al. (2021b). Use of Cause-And-Effect Analysis to Optimize the Reliability of In Vitro Inhalation Toxicity Measurements Using an Air–Liquid Interface. Chem. Res. Toxicol. 34 (6), 1370–1385. doi:10.1021/acs.chemrestox.1c00080
Poli, G., and Parola, M. (1997). Oxidative Damage and Fibrogenesis. Free Radic. Biol. Med. 22 (1-2), 287–305. doi:10.1016/s0891-5849(96)00327-9
Puchelle, E., Bajolet, O., and Abély, M. (2002). Airway Mucus in Cystic Fibrosis. Paediatric Respir. Rev. 3 (2), 115–119. doi:10.1016/S1526-0550(02)00005-7
Qu, F., Qin, X.-Q., Cui, Y.-R., Xiang, Y., Tan, Y.-R., Liu, H.-J., et al. (2009). Ozone Stress Down-Regulates the Expression of Cystic Fibrosis Transmembrane Conductance Regulator in Human Bronchial Epithelial Cells. Chemico-Biol. Interact. 179 (2–3), 219–226. doi:10.1016/j.cbi.2008.10.059
Quan, J. M., Tiddens, H. A. W. M., Sy, J. P., McKenzie, S. G., Montgomery, M. D., Robinson, P. J., et al. (2001). A Two-Year Randomized, Placebo-Controlled Trial of Dornase Alfa in Young Patients with Cystic Fibrosis with Mild Lung Function Abnormalities. J. Pediatr. 139 (6), 813–820. doi:10.1067/mpd.2001.118570
Rahman, I., and MacNee, W. (1996). Role of Oxidants/antioxidants in Smoking-Induced Lung Diseases. Free Radic. Biol. Med. 21 (5), 669–681. doi:10.1016/0891-5849(96)00155-4
Rahman, I., Biswas, S. K., and Kode, A. (2006). Oxidant and Antioxidant Balance in the Airways and Airway Diseases. Eur. J. Pharmacol. 533 (1-3), 222–239. doi:10.1016/j.ejphar.2005.12.087
Raju, S. V., Jackson, P. L., Courville, C. A., McNicholas, C. M., Sloane, P. A., Sabbatini, G., et al. (2013). Cigarette Smoke Induces Systemic Defects in Cystic Fibrosis Transmembrane Conductance Regulator Function. Am. J. Respir. Crit. Care Med. 188 (11), 1321–1330. doi:10.1164/rccm.201304-0733oc
Raju, S. V., Lin, V. Y., Liu, L., Mcnicholas, C. M., Karki, S., Sloane, P. A., et al. (2016). The Cftr Potentiator Ivacaftor Augments Mucociliary Clearance Abrogating Cftr Inhibition by Cigarette Smoke. Am. J. Respir. Cel Mol. Biol. 56 (1), 99–108. doi:10.1165/rcmb.2016-0226OC
Rasmussen, J. E., Sheridan, J. T., Polk, W., Davies, C. M., and Tarran, R. (2014). Cigarette Smoke-Induced Ca2+ Release Leads to Cystic Fibrosis Transmembrane Conductance Regulator (CFTR) Dysfunction. J. Biol. Chem. 289 (11), 7671–7681. doi:10.1074/jbc.M113.545137
Regnis, J. A., Robinson, M., Bailey, D. L., Cook, P., Hooper, P., Chan, H. K., et al. (1994). Mucociliary Clearance in Patients with Cystic Fibrosis and in normal Subjects. Am. J. Respir. Crit. Care Med. 150 (1), 66–71. doi:10.1164/ajrccm.150.1.8025774
Rennolds, J., Butler, S., Maloney, K., Boyaka, P. N., Davis, I. C., Knoell, D. L., et al. (2010). Cadmium Regulates the Expression of the CFTR Chloride Channel in Human Airway Epithelial Cells. Toxicol. Sci. 116 (1), 349–358. doi:10.1093/toxsci/kfq101
Riordan, J. R. (2008). CFTR Function and Prospects for Therapy. Annu. Rev. Biochem. 77 (1), 701–726. doi:10.1146/annurev.biochem.75.103004.142532
Robinson, M., and Bye, P. T. B. (2002). Mucociliary Clearance in Cystic Fibrosis. Pediatr. Pulmonol. 33 (4), 293–306. doi:10.1002/ppul.10079
Robinson, M., Hemming, A. L., Regnis, J. A., Wong, A. G., Bailey, D. L., Bautovich, G. J., et al. (1997). Effect of Increasing Doses of Hypertonic saline on Mucociliary Clearance in Patients with Cystic Fibrosis. Thorax 52 (10), 900–903. doi:10.1136/thx.52.10.900
Rompolas, P., Patel-King, R. S., and King, S. M. (2009). Schmidtea Mediterranea. Methods Cel. Biol. 93, 81–98. doi:10.1016/S0091-679X(08)93004-1
Rowe, S. M., Heltshe, S. L., Gonska, T., Donaldson, S. H., Borowitz, D., Gelfond, D., et al. (2014). Clinical Mechanism of the Cystic Fibrosis Transmembrane Conductance Regulator Potentiator Ivacaftor in G551D-Mediated Cystic Fibrosis. Am. J. Respir. Crit. Care Med. 190 (2), 175–184. doi:10.1164/rccm.201404-0703OC
Rutland, J., and Cole, P. J. (1981). Nasal Mucociliary Clearance and Ciliary Beat Frequency in Cystic Fibrosis Compared with Sinusitis and Bronchiectasis. Thorax 36 (9), 654–658. doi:10.1136/thx.36.9.654
Schaedel, C., de Monestrol, I., Hjelte, L., Johannesson, M., Kornfält, R., Lindblad, A., et al. (2002). Predictors of Deterioration of Lung Function in Cystic Fibrosis*. Pediatr. Pulmonol. 33 (6), 483–491. doi:10.1002/ppul.10100
Schmid, A., Clunes, L. A., Salathe, M., Verdugo, P., Dietl, P., Davis, C. W., et al. (2011). “Nucleotide-mediated Airway Clearance,” in Purinergic Regulation of Respiratory Diseases (Springer), 95–138. doi:10.1007/978-94-007-1217-1_5
Schmid, A., Baumlin, N., Ivonnet, P., Dennis, J. S., Campos, M., Krick, S., et al. (2015). Roflumilast Partially Reverses Smoke-Induced Mucociliary Dysfunction. Respir. Res. 16 (1), 135. doi:10.1186/s12931-015-0294-3
Schmid, A., Sailland, J., Novak, L., Baumlin, N., Fregien, N., and Salathe, M. (2017). Modulation of Wnt Signaling Is Essential for the Differentiation of Ciliated Epithelial Cells in Human Airways. FEBS Lett. 591 (21), 3493–3506. doi:10.1002/1873-3468.12851
Schwarzer, C., Fischer, H., Kim, E.-J., Barber, K. J., Mills, A. D., Kurth, M. J., et al. (2008). Oxidative Stress Caused by Pyocyanin Impairs CFTR Cl− Transport in Human Bronchial Epithelial Cells. Free Radic. Biol. Med. 45 (12), 1653–1662. doi:10.1016/j.freeradbiomed.2008.09.011
Sears, P. R., Yin, W.-N., and Ostrowski, L. E. (2015). Continuous Mucociliary Transport by Primary Human Airway Epithelial Cells In Vitro. Am. J. Physiol.-Lung Cell Mol. Physiol. 309 (2), L99–L108. doi:10.1152/ajplung.00024.2015
Sedaghat, M. H., Shahmardan, M. M., Norouzi, M., and Heydari, M. (2016). Effect of Cilia Beat Frequency on Muco-Ciliary Clearance. J. Biomed. Phys. Eng. 6 (4), 265–278.
Seybold, Z. V., Mariassy, A. T., Stroh, D., Kim, C. S., Gazeroglu, H., and Wanner, A. (1990). Mucociliary Interaction In Vitro: Effects of Physiological and Inflammatory Stimuli. J. Appl. Physiol. 68 (4), 1421–1426. doi:10.1152/jappl.1990.68.4.1421
Shaykhiev, R., Zuo, W.-L., Chao, I., Fukui, T., Witover, B., Brekman, A., et al. (2013). EGF Shifts Human Airway Basal Cell Fate toward a Smoking-Associated Airway Epithelial Phenotype. Proc. Natl. Acad. Sci. 110 (29), 12102–12107. doi:10.1073/pnas.1303058110
Sloane, P. A., Shastry, S., Wilhelm, A., Courville, C., Tang, L. P., Backer, K., et al. (2012). A Pharmacologic Approach to Acquired Cystic Fibrosis Transmembrane Conductance Regulator Dysfunction in Smoking Related Lung Disease. PloS one 7 (6), e39809. doi:10.1371/journal.pone.0039809
Smith, C. M., Djakow, J., Free, R. C., Djakow, P., Lonnen, R., Williams, G., et al. (2012). ciliaFA: a Research Tool for Automated, High-Throughput Measurement of Ciliary Beat Frequency Using Freely Available Software. Cilia 1 (1), 14. doi:10.1186/2046-2530-1-14
Snyder, R. J., Hussain, S., Tucker, C. J., Randell, S. H., and Garantziotis, S. (2017). Impaired Ciliogenesis in Differentiating Human Bronchial Epithelia Exposed to Non-cytotoxic Doses of Multi-Walled Carbon Nanotubes. Part. Fibre Toxicol. 14 (1), 1–14. doi:10.1186/s12989-017-0225-1
Song, Y., Namkung, W., Nielson, D. W., Lee, J.-W., Finkbeiner, W. E., and Verkman, A. S. (2009). Airway Surface Liquid Depth Measured in Ex Vivo Fragments of Pig and Human Trachea: Dependence on Na+ and Cl− Channel Function. Am. J. Physiol.-Lung Cell Mol. Physiol. 297 (6), L1131–L1140. doi:10.1152/ajplung.00085.2009
Stanek, A., Brambrink, A. M., Latorre, F., Bender, B., and Kleemann, P. P. (1998). Effects of Normobaric Oxygen on Ciliary Beat Frequency of Human Respiratory Epithelium. Br. J. Anaesth. 80 (5), 660–664. doi:10.1093/bja/80.5.660
Stubbs, J. L., Oishi, I., Izpisúa Belmonte, J. C., and Kintner, C. (2008). The Forkhead Protein Foxj1 Specifies Node-like Cilia in Xenopus and Zebrafish Embryos. Nat. Genet. 40 (12), 1454–1460. doi:10.1038/ng.267
Stubbs, J. L., Vladar, E. K., Axelrod, J. D., and Kintner, C. (2012). Multicilin Promotes Centriole Assembly and Ciliogenesis during Multiciliate Cell Differentiation. Nat. Cel Biol. 14 (2), 140–147. doi:10.1038/ncb2406
Szczesniak, R., Heltshe, S. L., Stanojevic, S., and Mayer-Hamblett, N. (2017). Use of FEV1 in Cystic Fibrosis Epidemiologic Studies and Clinical Trials: A Statistical Perspective for the Clinical Researcher. J. Cystic Fibrosis 16 (3), 318–326. doi:10.1016/j.jcf.2017.01.002
Tan, F. E., Vladar, E. K., Ma, L., Fuentealba, L. C., Hoh, R., Espinoza, F. H., et al. (2013). Myb Promotes Centriole Amplification and Later Steps of the Multiciliogenesis Program. Development 140 (20), 4277–4286. doi:10.1242/dev.094102
Tarran, R., Grubb, B. R., Parsons, D., Picher, M., Hirsh, A. J., Davis, C. W., et al. (2001). The CF Salt Controversy: in vivo observations and therapeutic approaches. Mol. Cel. 8 (1), 149–158. doi:10.1016/s1097-2765(01)00286-6
Tarran, R. (2004). Regulation of Airway Surface Liquid Volume and Mucus Transport by Active Ion Transport. Proc. Am. Thorac. Soc. 1 (1), 42–46. doi:10.1513/pats.2306014
Thomas, B., Rutman, A., and O'Callaghan, C. (2009). Disrupted Ciliated Epithelium Shows Slower Ciliary Beat Frequency and Increased Dyskinesia. Eur. Respir. J. 34 (2), 401–404. doi:10.1183/09031936.00153308
Toskala, E., Nuutinen, J., Rautiainen, M., and Torkkeli, T. (1995). The Correlation of Mucociliary Transport and Scanning Electron Microscopy of Nasal Mucosa. Acta Oto-Laryngol. 115 (1), 61–65. doi:10.3109/00016489509133348
Tuggle, K. L., Birket, S. E., Cui, X., Hong, J., Warren, J., Reid, L., et al. (2014). Characterization of Defects in Ion Transport and Tissue Development in Cystic Fibrosis Transmembrane Conductance Regulator (CFTR)-Knockout Rats. PloS one 9 (3), e91253. doi:10.1371/journal.pone.0091253
Valencia-Gattas, M., Conner, G. E., and Fregien, N. L. (2016). Gefitinib, an EGFR Tyrosine Kinase Inhibitor, Prevents Smoke-Mediated Ciliated Airway Epithelial Cell Loss and Promotes Their Recovery. PloS one 11 (8), e0160216. doi:10.1371/journal.pone.0160216
Van Goor, F., Hadida, S., Grootenhuis, P. D. J., Burton, B., Cao, D., Neuberger, T., et al. (2009). Rescue of CF Airway Epithelial Cell Function In Vitro by a CFTR Potentiator, VX-770. Proc. Natl. Acad. Sci. 106 (44), 18825–18830. doi:10.1073/pnas.0904709106
Van Goor, F., Hadida, S., Grootenhuis, P. D. J., Burton, B., Stack, J. H., Straley, K. S., et al. (2011). Correction of the F508del-CFTR Protein Processing Defect In Vitro by the Investigational Drug VX-809. Proc. Natl. Acad. Sci. 108 (46), 18843–18848. doi:10.1073/pnas.1105787108
Vij, S., Rink, J. C., Ho, H. K., Babu, D., Eitel, M., Narasimhan, V., et al. (2012). Evolutionarily Ancient Association of the FoxJ1 Transcription Factor with the Motile Ciliogenic Program. Plos Genet. 8 (11), e1003019. doi:10.1371/journal.pgen.1003019
Wanner, A., Salathé, M., and O'Riordan, T. G. (1996). Mucociliary Clearance in the Airways. Am. J. Respir. Crit. Care Med. 154 (6 Pt 1), 1868–1902. doi:10.1164/ajrccm.154.6.8970383
Widdicombe, J. H., and Widdicombe, J. G. (1995). Regulation of Human Airway Surface Liquid. Respiration Physiol. 99 (1), 3–12. doi:10.1016/0034-5687(94)00095-h
Willett, C. (2019). “The Use of Adverse Outcome Pathways (AOPs) to Support Chemical Safety Decisions within the Context of Integrated Approaches to Testing and Assessment (IATA),” in ALTEX (Springer Singapore), 83–90.
Wilson, R., Pitt, T., Taylor, G., Watson, D., MacDermot, J., Sykes, D., et al. (1987). Pyocyanin and 1-hydroxyphenazine Produced by Pseudomonas aeruginosa Inhibit the Beating of Human Respiratory Cilia In Vitro. J. Clin. Invest. 79 (1), 221–229. doi:10.1172/jci112787
Woodworth, B. A. (2015). Resveratrol Ameliorates Abnormalities of Fluid and Electrolyte Secretion in a Hypoxia-Induced Model of Acquired CFTR Deficiency. Laryngoscope 125 (S7), S1–S13. doi:10.1002/lary.25335
Xie, S., Jin, J., Xu, Z., Huang, Y., Zhang, W., Zhao, L., et al. (2019). Centrosomal Protein FOR20 Is Essential for Cilia‐dependent Development in Zebrafish Embryos. FASEB J. 33 (3), 3613–3622. doi:10.1096/fj.201801235RR
Xu, X., Balsiger, R., Tyrrell, J., Boyaka, P. N., Tarran, R., and Cormet-Boyaka, E. (2015). Cigarette Smoke Exposure Reveals a Novel Role for the MEK/ERK1/2 MAPK Pathway in Regulation of CFTR. Biochim. Biophys. Acta (Bba) - Gen. Subj. 1850 (6), 1224–1232. doi:10.1016/j.bbagen.2015.02.004
Yaghi, A., and Dolovich, M. (2016). Airway Epithelial Cell Cilia and Obstructive Lung Disease. Cells 5 (4), 40. doi:10.3390/cells5040040
Yeates, D. B., Katwala, S. P., Daugird, J., Daza, A. V., and Wong, L. B. (1997). Excitatory and Inhibitory Neural Regulation of Tracheal Ciliary Beat Frequency (CBF) Activated by Ammonia Vapour and SO2. Ann. Occup. Hyg. 41, 736–744.
Yoshitsugu, M., Matsunaga, S., Hanamure, Y., Rautiainen, M., Ueno, K., Miyanohara, T., et al. (1995). Effects of Oxygen Radicals on Ciliary Motility in Cultured Human Respiratory Epithelial Cells. Auris Nasus Larynx 22 (3), 178–185. doi:10.1016/s0385-8146(12)80056-3
Yu, X., Ng, C. P., Habacher, H., and Roy, S. (2008). Foxj1 Transcription Factors Are Master Regulators of the Motile Ciliogenic Program. Nat. Genet. 40 (12), 1445–1453. doi:10.1038/ng.263
Zhang, S., Blount, A. C., McNicholas, C. M., Skinner, D. F., Chestnut, M., Kappes, J. C., et al. (2013). Resveratrol Enhances Airway Surface Liquid Depth in Sinonasal Epithelium by Increasing Cystic Fibrosis Transmembrane Conductance Regulator Open Probability. PloS one 8 (11), e81589. doi:10.1371/journal.pone.0081589
Zhang, Z., Leir, S.-H., and Harris, A. (2015). Oxidative Stress RegulatesCFTRGene Expression in Human Airway Epithelial Cells through a Distal Antioxidant Response Element. Am. J. Respir. Cel Mol. Biol. 52 (3), 387–396. doi:10.1165/rcmb.2014-0263OC
Keywords: adverse outcome pathway, AOP, mucociliary clearance, ciliary beat frequency, lung function, new approach methodologies, NAMs, inhalation toxicity
Citation: Luettich K, Sharma M, Yepiskoposyan H, Breheny D and Lowe FJ (2021) An Adverse Outcome Pathway for Decreased Lung Function Focusing on Mechanisms of Impaired Mucociliary Clearance Following Inhalation Exposure. Front. Toxicology 3:750254. doi: 10.3389/ftox.2021.750254
Received: 30 July 2021; Accepted: 11 November 2021;
Published: 14 December 2021.
Edited by:
Claire Terry, Corteva Agriscience™, United StatesReviewed by:
Hilmi Orhan, Ege University, TurkeySatyanarayana “Satya” Achanta, Duke University, United States
Copyright © 2021 Luettich, Sharma, Yepiskoposyan, Breheny and Lowe. This is an open-access article distributed under the terms of the Creative Commons Attribution License (CC BY). The use, distribution or reproduction in other forums is permitted, provided the original author(s) and the copyright owner(s) are credited and that the original publication in this journal is cited, in accordance with accepted academic practice. No use, distribution or reproduction is permitted which does not comply with these terms.
*Correspondence: Karsta Luettich, Karsta.Luettich@pmi.com