- 1Departamento de Medicina Tropical, Instituto de Investigaciones en Ciencias de la Salud-Universidad Nacional de Asunción, Asunción, Paraguay
- 2Departamento de Biología, Facultad de Ciencias Exactas y Naturales-Universidad Nacional de Asunción, Asunción, Paraguay
- 3Laboratorio de Biología de Vectores y Parásitos, Instituto de Zoología y Ecología Tropical, Universidad Central de Venezuela, Caracas, Venezuela
- 4Centro para el Desarrollo de la Investigación Científica (CEDIC), Asunción, Paraguay
- 5Grupo de Química Orgánica Medicinal, Instituto de Química Biológica, Facultad de Ciencias-Universidad de la República, Montevideo, Uruguay
- 6Área de Radiofarmacia, Centro de Investigaciones Nucleares, Facultad de Ciencias-Universidad de la República, Montevideo, Uruguay
For almost one hundred years, the quinoline heterocycle has been recognized as a privileged pharmacophore in anti-Leishmania agents. Early on, the action of compounds with this scaffold, found in some natural alkaloids, was tested against different Leishmania stages and strains. Different structural arrangements containing the quinoline framework have been described in different in vitro and in vivo anti-Leishmania alkaloids, namely, quinoline proper, isoquinoline, and quinolone, among others. In recent years, new quinoline derivatives isolated from nature have been described, in addition to having carried out in-depth in vitro and in vivo biological studies, as well as chemical modifications to obtain new leaders. This review updates the state of the art on naturally occurring quinolines and some synthetic derivatives to provide therapeutic tools and strategies to explore new drugs based on this chemosystem for the treatment of leishmaniasis.
Introduction
Leishmaniasis is a zoonotic vector-borne disease caused by protozoan parasites of the genus Leishmania (Eukarya, Kinetoplastea), transmitted to animals and humans through the bite of infected female phlebotomine sandflies. The disease presents in three main forms: cutaneous leishmaniasis (CL), mucocutaneous leishmaniasis (MCL), and visceral leishmaniasis (VL) (Steverding, 2017). The stigmatization in patients with the visible nature of CL and MCL is a significant social and psychological issue, leading to discrimination, social exclusion, and emotional distress (Wenning et al., 2022). There are approximately 700,000 to 1 million new cases of leishmaniasis every year, with an estimated 20,000 to 40,000 deaths in 98 endemic countries (WHO, 2024). The burden of the disease is concentrated in areas of Africa, the Middle East, South America, and parts of Asia. Efforts are ongoing to improve surveillance, control, and treatment strategies, as well as to develop new interventions such as vaccines and innovative diagnostic tools. There is no vaccine available for use in humans, and control of the disease relies mainly on chemotherapy. Current drugs are outdated, toxic, and unaffordable for most populations, while increasing resistance and treatment failures challenge their efficacy (Monzote, 2009).
The quinoline heterocycle has been acknowledged as a favored pharmacophore in anti-Leishmania agents for nearly 10 decades (Karamchandani, 1927; Varma, 1927; DasGupta and Dikshit, 1929; Devi, 1929). Compounds containing this scaffold, which are present in many natural alkaloids with different structural motives, including quinoline proper, isoquinoline, and quinolone, have shown to be effective in vitro and in vivo against various Leishmania strains and stages early on. Recent years have seen the description of novel quinoline secondary metabolites derived from primary metabolites discovered in nature, as well as extensive in vitro and in vivo biological research and chemical modifications, generating chemodiversity by using moieties not present in nature, to produce new anti-Leishmania leads. In the next section, we expose some recent aspects related to the investigation of new anti-Leishmania drugs based on this chemosystem.
Historical background
In the 20th century, the first descriptions of naturally occurring quinolines date to the 1920s. Thus, berberine was one of the first alkaloids of the isoquinoline group, an ammonium salt with an interesting structure (1, Figure 1A), described as anti-Leishmania (Karamchandani, 1927; Varma, 1927). In the early 1990s, this alkaloid, along with other derivatives (natural and synthetic), was evaluated in vivo in golden hamster models of Leishmania donovani and Leishmania braziliensis panamensis, finding that it was less potent in L. donovani and more toxic (measuring weight loss) than the reference drug, Glucantime (Vennerstrom et al., 1990). Two synthetic derivatives (2 and 3, Figure 1A) emerged, due to their activities or toxicities, as possible leaders to consider in future studies.
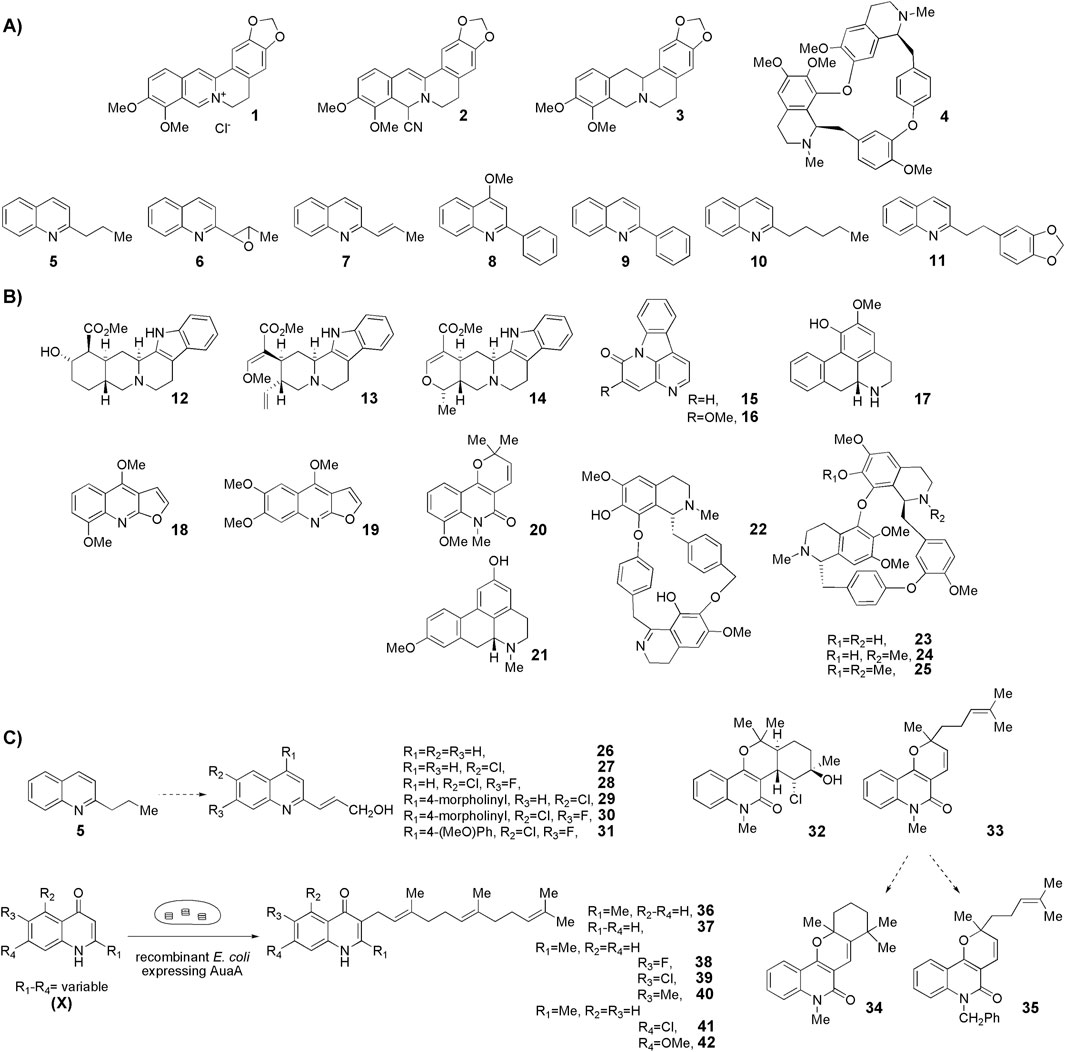
Figure 1. Some relevant natural quinolines with anti-Leishmania activities isolated (A) in the 20th century and (B) in the 21st century. Recent synthetic compounds with anti-Leishmania activities based on natural quinolines (C).
Since the 1970s, Fournet et al. described a series of isolations of different quinolines that were studied in vitro and in vivo against Leishmania. In this sense, the bisbenzyltetrahydroisoquinoline isotetrandrine (4, Figure 1A), isolated from the roots, stems, leaves, and fruits of Limaciopsis loangensis Engl., a liana growing in west central tropical Africa to Angola (Cavé et al., 1979), was evaluated in vitro (Fournet et al., 1988) and in vivo with promising results (at 100 mg/kg/day), being better than the reference drug (Glucantime, at 200 mg/kg/day), against Leishmania amazonensis (PH8 and H-142) and Leishmania venezuelensis (VE/74/PM-H3) in Balb/c mice (Fournet et al., 1993a). In 1994, petroleum ether and chloroformic extraction of alkaloidal extracts of stem, leaves, and roots of Galipea longiflora Krause, a tree growing in Bolivia, Brazil, and Perú, were analyzed in vitro and in vivo against Leishmania spp. (Fournet et al., 1994b; Fournet et al., 1994c). The bioguided extract fractionations and purifications revealed 12 active alkaloids, 2-substituted quinolines 5–11, with 5–8 (Figure 1A) having the most in vitro anti-promastigotes activity against different strains of Leishmania braziliensis, L. amazonensis and L. donovani (Fournet et al., 1994a) (IC90 in the range of 25–100 μg/mL with IC90 of 1 μg/mL for pentamidine and >100 μg/mL for Glucantime in all the studied strains). The authors did not mention alkaloids with no biological activity.
The most recent naturally isolated quinolines and derivatives with leishmanicidal activities
In the 21st century, new alkaloids derived from quinoline framework with very varied and more complex structures were isolated and evaluated biologically, in vitro and in vivo, against Leishmania. Thus, in 2000, Staerk et al. (2000) described some alkaloids isolated from the bark of Corynanthe pachyceras K.Schum., a lower-storey forest tree growing in tropical West Africa, that were evaluated against different biosystems. Among the characterized compounds, perhydroisoquinoline 12 (Figure 1B) stood out for its in vitro activity against Leishmania major promastigotes (IC50 = 23.4 µM), nearly 10 times greater than the reference Pentostam (IC50 = 219 µM). The hexahydro-1H-quinolizine 13 (Figure 1B), related to 12, was another secondary metabolite extracted from this botanical species that emerged as excellent anti-Leishmania agent (IC50 = 1.12 µM) together with the alkaloid 14 (ajmalicine, IC50 = 0.57 µM, Figure 1B), previously extracted from other botanical species, which is structural and metabolically related to this system but was not evaluated against this protozoan (Wei, 1965).
Since 2002, Ferreira et al. (2002) described a series of isolations of new quinoline derivatives that were studied in vitro and in vivo as potential anti-Leishmania drugs. From the stem bark of Zanthoxylum chiloperone var. angustifolium (Engl.), a tree growing in central and southern South America, they isolated a pair of azaquinolines, canthin-6-one, 15, and 5-methoxycanthin-6-one, 16 (Figure 1B). These alkaloids were the two most active major constituents of the extract of Z. chiloperone stem bark in vitro. In another study from 2015, the authors studied the best harvesting time and seasonal dynamics to obtain the best yield of 16 from Z. chiloperone leaves (Cebrián-Torrejón et al., 2015). Using information from traditional medicine, Ferreira et al. screened different Paraguayan plants for anti-Leishmania activities and found that the crude extract of Ocotea lancifolia stem bark, a tree growing in Argentina, Brazil, Bolivia, and Paraguay, displayed relevant behaviors against three strains of promastigote of L. braziliensis, L. amazonensis and L. donovani (Fournet et al., 2007). Among the active alkaloids, the study identified 17 ((−)-cadaverine, Figure 1B), which also displayed a discrete selectivity index (SI), IC50,mammal/IC50,protozoan, of 1.6, using HepG2 hepatic cell line. Finally, Ferreira et al. (2010) isolated from the stem bark of Helietta apiculata Benth., a shrub or tree growing in South America (Argentina, Brazil, and Paraguay), a series of secondary metabolites and evaluated in vitro against L. amazonensis, Leishmania infantum, and L. braziliensis promastigotes and in vivo in a model of L. amazonensis infection in Balb/c mice. Among these metabolites, they found the furoquinoline 18 (γ-fagarine, Figure 1B) (Ahsan et al., 1994) as the most active alkaloid in vitro (IC50 between 17.3 and 26.5 µM), with higher activity than the chloroformic extract of Helietta apiculata (IC50 between 28.5 µM and 39.4 µM) but with much lower activity than the in vitro references (miltefosine, IC50 between 7.5 and 10.4 µM, and amphotericin B, IC50 between 0.01 and 0.05 µM). At that time, Coy Barrera et al. (2011) also described the isolation of furoquinolines, among other secondary metabolites, from stem bark or leaves of Raputia heptaphylla Pittier, a shrub or tree growing in the wet tropical locations of Bolivia, Colombia, Costa Rica, Panamá, Perú, and Venezuela. However, the furoquinolines (for example, 19, Figure 1B) displayed poor in vitro activities against promastigotes and amastigotes of Leishmania (V.) panamensis. From this botanic species, the 2-quinolinone 20 (Figure 1B) emerged as a promising anti-promastigotes agent (IC50 = 52.7 µM, with IC50 = 2.5 µM for pentamidine and IC50 > 1098 µM for sodium stibogluconate).
In 2012, Mollataghi et al. (2012) described the isolation of a new alkaloid (21, Figure 1B), among others previously described in other botanical species, from the acidic percolate of the bark of Beilschmiedia alloiophylla (Rusby) Kosterm, a tree growing in Colombia, Costa Rica, Ecuador, Panamá, and Venezuela. This polyhydroquinoline, a methylated positional isomer of 17, was the best anti-Leishmania alkaloid evaluated in this study (IC50 = 10.5 µM with an IC50 = 0.4 µM for miltefosine), although, unfortunately, the authors did not specify which strain and stage of the protozoan were used.
da Silva et al. (2012) evaluated the previously isolated bisbenzylisoquinoline warifteine (22, Figure 1B) against promastigotes of Leishmania chagasi in vitro. This alkaloid, purified from leaves of Cissampelos sympodialis Eichl., a climbing shrub growing in Brazil, was 50 times more active (IC50 = 135 µM) than the reference Glucantime. Regrettably, 22 had cytotoxicity against mammalian cells HEp-2 and NCI-H292 with SIs less than 1. Similarly, Naman et al. (2015), in a study that involved in vitro screening against L. donovani promastigotes of 234 isolated natural products, identified three bisbenzyltetrahydroisoquinolines (23, 24, and 25, Figure 1B) with protozoan IC50 lower than 10 µM. These alkaloids, which were purified previously from roots of Thalictrum alpinum, a perennial herb growing in the arctic and alpine regions of North America and Eurasia, displayed different SIs (using human colorectal cancer HT-29 cells as mammalian system) depending on the N- and O-methylation at SI23 = 29.3; SI24 = 3.6; and SI25 = 0.5.
Some recent structural modifications based on the naturally isolated quinolines in the search for leishmanicidal agents
Leishmaniasis is not an exception to the long-standing practice of employing natural products as structural templates to produce novel bioactive compounds. In this regard, the active isolated 2-substituted quinolines (for example, 5, Figure 1A) (Fournet et al., 1994a) were used as a structural reference to generate new synthetic derivatives. In 2003, Fakhfakh et al. (2003) synthesized approximately 50 quinolines by modifying them mainly in heterocycle position 2. In general, the 2-alkenyl derivatives displayed good anti-parasite activities, and the authors highlighted synthetic-quinoline 26 (Figure 1C) for its double antileishmanial and antiviral activity (Fakhfakh et al., 2003) that could be proposed as a drug for coinfection of HIV-Leishmania. A few years later, Gopinath et al. (2013), with the help of the Drug for Neglected Diseases initiative (DNDi, 2024), prepared some quinolines by modifying position 2 but also investigating modifications at positions 4, 5, 6, and 7 (for example, 27, 28, 29, 30, and 31, Figure 1C). Approximately 25 quinolines with such characteristics were reported for their in vitro activities against intramacrophagic L. donovani amastigotes and against the Vero cell line (mammalian kidney epithelial cells), with descriptions of their different activities and selectivity profiles. 2,4,6,7-Tetrasubstitutedquinoline 30 emerged as the most selective of all the studied compounds, some of which had the following order: SI30 = 187.5; SI27 = 33.59; SI31 = 22.26; SI29 = 10.65; SImiltefosine = 6.27; SI28 = 5.54; SI26 = 3.17; and SI5<1.25. Additionally, 30, together with 26, 31, and miltefosine, was studied in an in vivo model of VL (Table 1). The in vivo results, together with some relevant results of 30 pharmacokinetic studies (Gopinath et al., 2014), indicate it could be a drug candidate to be investigated further.
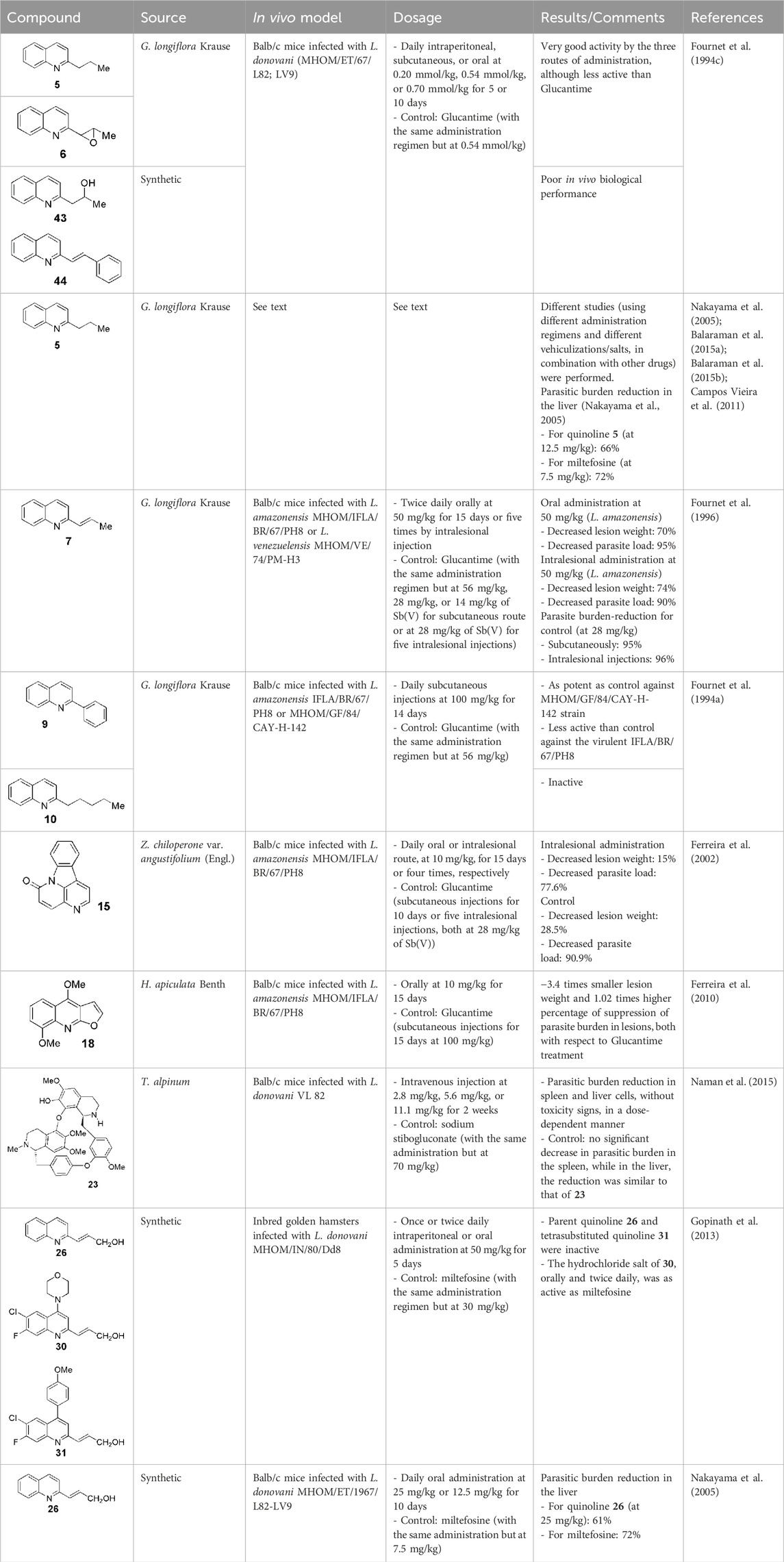
Table 1. Summary of anti-Leishmania studies on animal models of natural quinolines and synthetic inspired by natural ones.
In the preparation of the quinolone chloroaustralasine A (32, Figure 1C) Jézéquel et al. (2022) generated, as a synthetic intermediate, zanthosimuline (33, Figure 1C) (Wu and Chen, 1993) and other related subproducts with different structural motives, for example, 34 (Figure 1C). The authors synthesized 32 in order to know whether the anti-Leishmania activity previously described in the whole bark extract of Codiaeum peltatum (Billo et al., 2005) could be due to this quinolone. Additionally, from the N-demethylated 33, the authors produced different N-substituted derivatives (for example, 35, Figure 1C). The synthetic products 34 and 35 were active against intramacrophagic amastigotes of L. infantum and especially, unlike the parent compounds, selective toward the parasite, using RAW 264.7 macrophages as the mammal model; SImiltefosine = 8.1; SI34 > 6.8; SI35 > 4.2; SI32 < 1.6; and SI33 < 0.8.
In 2023, Kruth et al. (2023), taking as a structural template the antibiotic isolated from different bacteria (Kunze et al., 1987), aurachin D (36, Figure 1C), described the preparation of a series of derivatives of it to be evaluated as anti-Leishmania agents using whole-cell biotransformations with recombinant E. coli. The E. coli, able to regioselectively incorporate the farnesyl moiety at the 3-position of the heterocycle by expressing aurachin farnesyltransferase AuaA (Kruth et al., 2022), was fed with quinolone precursors with different substitutions at 2-, 5-, 6- and/or 7-positions ((X), Figure 1C). Of the 13 analyzed substrates, only three non-natural ones could not be biotransformed. The generated bio-products were evaluated in vitro against axenic amastigotes of L. donovani, and the selectivity was determined using rat myoblast L6 cells. 4-Quinolones 36 and 38 (Figure 1C) displayed better activities against the parasite than miltefosine, and some of the SIs were: SI36 = 2969.5; SI38 = 203.9; SI39 = 18.9; SI37 = 13.2; SI41 = 5.9; SI42 = 4.7; and SI40 = 4.3.
Naturally isolated quinolines moving from lead to drug stage in the leishmanicidal pipeline
As a way of advancing in the anti-Leishmania quinoline-based drug pipeline, some in vivo efficacy, pharmacokinetic, safety, stability, formulation, and potential mechanisms of action studies have been carried out. These studies will be discussed below.
Fournet et al. (1994) conducted one of the most relevant analyses of the in vivo efficacy of natural quinolines. These authors evaluated 2-substituted quinolines 5 and 6 (Figure 1A) together with two synthetic derivatives, 43 and 44 (Table 1), finding that the natural ones were active against CL and VL (Fournet et al., 1994c) but less effective than the reference drug (Table 1). In another in vivo study (Fournet et al., 1994a), the main products of the alkaloidal extracts of G. longiflora Krause, that is, 9 and 10 (Figure 1A), were evaluated in models of CL. The authors found some interesting activity for 9 (Table 1). Two years later, the authors described the in vivo evaluation of the pure alkaloids 5-7 and 9–11 (Figure 1A) and two total alkaloidal extracts of G. longiflora Krause against a model of CL (Fournet et al., 1996). The results showed that 7, with biological behavior similar to Glucantime (Table 1), is a leading compound to develop into oral anti-Leishmania therapy.
Continuing with the work on the pharmacological activity of bioactive anti-Leishmania secondary metabolites, Fournet and collaborators described in vivo studies and a model of CL using 15 and 16 (Figure 1B) (Ferreira et al., 2002). The best activity was observed for the azaquinoline 15 in an intralesional dose; however, it was discrete compared to Glucantime (Table 1). The furoquinoline 18 was also studied in vivo in the CL model by Fournet´s collaborators in 2010 (Ferreira et al., 2010), who found better activity at lower doses than Glucantime (Table 1).
The in vitro selective 23, identified by Naman et al. (2015) as an anti-Leishmania agent, was tested in vivo in a murine model of VL. This bisisoquinoline displayed good activity in doses lower than Glucantime (Table 1).
In 1998, Iglarz et al. (1998) developed chromatographic methods using HPLC to determine natural-quinoline 5 (Figure 1A) in plasma and liver homogenates of mice. They found a hepatic distribution, where the parasites are localized, with an estimated half-life of 100 min, while in plasma, a second peak at approximately 4 h post-dosage appeared, indicating a possible enterohepatic cycle, although the liver results did not support this hypothesis. In 2005, Nakayama et al. (2005) studied the in vivo efficacy of some natural and synthetic 2-substituted quinolines on the CL, L. amazonensis, and VL, L. infantum and L. donovani, in order to obtain pharmacological data. The alkaloid 5 and synthetic 26 (Figure 1C) reduced the L. donovani parasite burdens in the liver with values similar to miltefosine (Table 1). After that, Campos Vieira et al. (2008) selected three 2-substituted quinolines, that is, 5 (Figure 1A), 26 (Figure 1C), and the O-methyl derivative of 26, for study of their stability and acute oral toxicity. The alkaloid 5, studied in methanol, DMSO, aqueous solution pH 7 (PBS), an aqueous solution of HCl pH 2, and a mixture of carboxymethylcellulose (0.5% in glucose 5%) and Tween 80 (0.5%) (CMC/T80) stored, was the most stable in all the cases, at 4°C or at room temperature with and without light. In the acute oral toxicity studies, administering a single dose of 10 mg/kg, 100 mg/kg, or 1000 mg/kg of quinoline 5 in the CMC/T80 emulsion and analyzing apparent toxicity signs and biochemical and hematological data, the authors found reversible toxicity signs (lethargic and staggering mice) only at 1000 mg/kg, a dose 80 times higher than the therapeutic dose found by Nakayama et al. (2005), without detected signs at the other doses.
The oily character of natural-alkaloid 5, which affects its administration and bioavailability, has led to the study of different formulations to enhance its aqueous solubility. On the one hand, Campos Vieira et al. (2011) transformed 5 in its camphorsulfonic salt, 5-camp, and found that 5-camp retained the therapeutic efficacy of the free-base 5 and had comparable efficacy that miltefosine in the same dosage protocol, orally and daily at 60 μmol/kg of body weight for ten consecutive days.
Balaraman et al. tested two different approaches, in one case as a liposomal formulation and, in the other, as a hydroxypropyl β-cyclodextrin one. The liposomal vehiculization of 5 was active in vitro and in vivo in VL, intravenously administered at 3 mg/kg/day for 5 days (Balaraman et al., 2015a) and, in combination, at 0.75 mg/kg/day, with amphotericin B, at 6 μg/kg/day, for 5 days, and displayed a notable synergistic effect, which is, according the authors, a crucial factor in overcoming drug resistance. The formulation with the cyclodextrin was more than five times as active in vitro against intramacrophagic amastigotes of L. donovani than 5 alone and was also effective against resistant strains without inducing resistance (Balaraman et al., 2015b). In the in vivo studies, the intravenous administration of this formulation of alkaloid 5, at 10 mg/kg/day for 10 days, reduced the parasite load in a comparable manner to miltefosine, without any evidence of toxicity. The pharmacokinetic studies performed by these authors found a rapid plasma concentration decline of 5 with a half-life of 58.7 min, which indicated that this formulation was in vivo effective for its adequate distribution throughout the tissues, facilitating the eradication of parasites in cases of disseminated leishmaniasis.
Some relevant mode and mechanism of action studies were described for some natural quinolines. Ghosh et al. (1985) demonstrated that amastigotes treated with the alkaloid 1 had (Figure 1A): i) inhibited respiration; ii) inhibited deoxyglucose uptake, indicating altered membrane composition; iii) interfered macromolecular biosynthesis. Additionally, 1 was able to interact with promastigote nuclear DNA in vitro and was found to be DNA-intercalating. Saha et al. demonstrated that 1: i) triggered caspase-independent apoptosis-like death in promastigotes (Saha et al., 2009); ii) killed amastigotes by increasing NO production within the host macrophage (Saha et al., 2011); iii) and generated oxidative burst in infected neutrophils triggering apoptosis and in infected macrophages modulated mitogen-activated protein kinases (MAPKs).
Another pair of very interesting works by Calla-Magariños et al. found that the G. longiflora Krause extract, mainly composed of 2-substituted quinolines 5, 8–11 (Figure 1A) with 9 being the most abundant, showed immunomodulatory activity on the host. The extract interfered with the activation of both mouse and human T cells, evidenced by the in vitro cellular proliferation reduction and interferon-gamma production (Calla-Magariños et al., 2009). Additionally, they found, unlike Glucantime, that the extract’s effect on the interferon-gamma was also seen in vivo with the concomitant IL-12 and tumor necrosis factor alpha suppression in restimulated spleen cells from total Leishmania lysate immunized animals (Calla-Magariños et al., 2013). These results showed that these quinolines would add a relevant advantage in the pharmacological treatment control of the chronic inflammatory reaction characterized in Leishmania infection, in addition to the anti-Leishmania activity.
The mechanisms of action and cellular deaths of natural quinolone 20 (Figure 1B) were studied, together with two synthetic quinolines, by Torres Suarez et al. (2020). The alkaloid 20 increased NO production in human peripheral blood (monocyte)-derived macrophages (MDMs) infected with L. (V.) panamensis promastigotes and produced necrosis-related ultrastructural alterations in intracellular amastigotes. On the other hand, the studied synthetic quinolines displayed different biological phenomena stimulating, in one case, oxidative breakdown in hMDMs and causing ultrastructural alterations in the parasite 4 h posttreatment and, in the other case, failing to induce macrophage modulation and selectively inducing apoptosis of infected hMDMs and alterations in the intracellular parasite ultrastructure.
Concluding remarks
Even though few examples of natural quinolines and structurally related compounds tested against Leishmania have been described, the most promising skeleton for the anti-Leishmania therapies development is the bisbenzyltetrahydroisoquinoline system. Derivatives of this highly fused-cycle and functionalized system were shown to be effective in murine models of both CL and VL. Additionally, 2-substituted quinolines have been widely studied with models of CL and VL, both in vitro and in vivo, and should continue to be studied with different administration regimens or in combination with other agents. These studies could position these quinolines in a relevant place.
Regrettably, the pharmaceutical industry has not kept up with these studies, possibly because the compounds identified in these published findings cannot be patented, which has not allowed these agents to become “bench to bedside” entities.
In addition, unfortunately, biological studies have not yet been oriented to define whether there is a preference on the part of the quinoline derivatives against a certain type of Leishmania parasites that produce CL, MCL, or VL. Similarly, there have been few studies of the mechanism of action of natural quinolines that allow guiding the development of new quinoline-derived anti-Leishmania agents. Research should be directed in these fields.
Author contributions
GY: writing – original draft and writing – review and editing. LH: writing – original draft and writing – review and editing. MR: writing – original draft and writing – review and editing. CV: writing – original draft and writing – review and editing. HC: conceptualization, writing – original draft, and writing – review and editing.
Funding
The author(s) declare that financial support was received for the research and/or publication of this article. This work was supported by the Universidad de la República, Montevideo, Uruguay.
Conflict of interest
The authors declare that the research was conducted in the absence of any commercial or financial relationships that could be construed as a potential conflict of interest.
Generative AI statement
The author(s) declare that no Generative AI was used in the creation of this manuscript.
Publisher’s note
All claims expressed in this article are solely those of the authors and do not necessarily represent those of their affiliated organizations, or those of the publisher, the editors and the reviewers. Any product that may be evaluated in this article, or claim that may be made by its manufacturer, is not guaranteed or endorsed by the publisher.
References
Ahsan, M., Gray, A. I., Waterman, P., and Armstrong, J. A. (1994). 4-Quinolinone, 2-quinolinone, 9-acridanone, and furoquinoline alkaloids from the aerial parts of Boronia bowmanii. J. Nat. Prod. 57, 670–672. doi:10.1021/np50107a020
Balaraman, K., Barratt, G., Pomel, S., Cojean, S., Pilcher, B., Kesavan, V., et al. (2015a). “New formulations of 2-n-propylquinoline for the treatment of visceral leishmaniasis,” in Proceedings of the 2nd conference of the European COST action CM1307 “targeted chemotherapy towards diseases caused by endoparasites”, belgrade, Serbia. 26–28 October.
Balaraman, K., Campos Vieira, N., Moussa, F., Vacus, J., Cojean, S., Pomel, S., et al. (2015b). In vitro and in vivo antileishmanial properties of a 2-n-propylquinoline hydroxypropyl beta-cyclodextrin formulation and pharmacokinetics via intravenous route. Biomed. Pharmacother. 76, 127–133. doi:10.1016/j.biopha.2015.10.028
Billo, M., Fournet, A., Cabalion, P., Waikedre, J., Bories, C., Loiseau, P., et al. (2005). Screening of New Caledonian and Vanuatu medicinal plants for antiprotozoal activity. J. Etnopharmacol. 96, 569–575. doi:10.1016/j.jep.2004.10.006
Calla-Magariños, J., Giménez, A., Troye-Blomberg, M., and Fernández, C. (2009). An alkaloid extract of Evanta, traditionally used as anti-Leishmania agent in Bolivia, inhibits cellular proliferation and interferon-gamma production in polyclonally activated cells. Scand. J. Immunol. 69, 251–258. doi:10.1111/j.1365-3083.2008.02219.x
Calla-Magariños, J., Quispe, T., Giménez, A., Freysdottir, J., Troye-Blomberg, M., and Fernández, C. (2013). Quinolinic alkaloids from Galipea longiflora Krause suppress production of proinflammatory cytokines in vitro and control inflammation in vivo upon Leishmania infection in mice. Scand. J. Immunol. 77, 30–38. doi:10.1111/sji.12010
Campos Vieira, N., Herrenknecht, C., Vacus, J., Fournet, A., Bories, C., Figadère, B., et al. (2008). Selection of the most promising 2-substituted quinoline as antileishmanial candidate for clinical trials. Biomed. Pharmacother. 62, 684–689. doi:10.1016/j.biopha.2008.09.002
Campos-Vieira, N., Vacus, J., Fournet, A., Baudouin, R., Bories, C., Séon-Méniel, B., et al. (2011). Antileishmanial activity of a formulation of 2-n-propylquinoline by oral route in mice model. Parasite 18, 333–336. doi:10.1051/parasite/2011184333
Cavé, A., Leboeuf, M., Hocquemiller, R., Bouquet, A., and Fournet, A. (1979). Alcaloïdes du Limaciopsis loangensis. Planta Med. 35, 31–41. doi:10.1055/s-0028-1097180
Cebrián-Torrejón, G., Kablan, L., Ferreira, M. E., Rodríguez de la Cruz, D., Doménech-Carbó, A., Vera de Bilbao, N., et al. (2015). Harvesting canthinones: identification of the optimal seasonal point of harvest of Zanthoxylum chiloperone leaves as a source of 5-methoxycanthin-6-one. Nat. Prod. Res. 29, 2054–2058. doi:10.1080/14786419.2015.1022774
Coy Barrera, C. A., Coy Barrera, E. D., Granados Falla, D. S., Delgado Murcia, G., and Cuca Suarez, L. E. (2011). seco-Limonoids and quinoline alkaloids from Raputia heptaphylla and their antileishmanial activity. Chem. Pharm. Bull. 59, 855–859. doi:10.1248/cpb.59.855
DasGupta, B. M., and Dikshit, B. B. (1929). Berberine in the treatment of oriental sore. Indian Med. Gaz. 64, 67–70.
da Silva, E. C., Dias Rayol, C., Medeiros, P. L., Bressan Queiroz Figueiredo, R. C., Piuvezan, M. R., Brabosa-Filho, J. M., et al. (2012). Antileishmanial activity of warifteine: a bisbenzylisoquinoline alkaloid isolated from Cissampelos sympodialis Eichl. (Menispermaceae). Sci.World J. 2012, 1–5. Article ID 516408. doi:10.1100/2012/516408
DNDi (2024). Drug for neglected diseases initiative. Available online at: https://dndi.org/(accessed on December 02, 2024).
Fakhfakh, M. A., Fournet, A., Prina, E., Mouscadet, J. F., Franck, X., Hocquemiller, R., et al. (2003). Synthesis and biological evaluation of substituted quinolines: potential treatment of protozoal and retroviral co-infections. Bioorg. Med. Chem. 11, 5013–5023. doi:10.1016/j.bmc.2003.09.007
Ferreira, M. E., Rojas de Arias, A., Torres de Ortiz, S., Inchausti, A., Nakayama, H., Thouvenel, C., et al. (2002). Leishmanicidal activity of two canthin-6-one alkaloids, two major constituents of Zanthoxylum chiloperone var. angustifolium. J. Ethnopharmacol. 80, 199–202. doi:10.1016/s0378-8741(02)00025-9
Ferreira, M. E., Rojas de Arias, A., Yaluff, G., Vera de Bilbao, N., Nakayama, H., Torres, S., et al. (2010). Antileishmanial activity of furoquinolines and coumarins from Helietta apiculata. Phytomedicine 17, 375–378. doi:10.1016/j.phymed.2009.09.009
Fournet, A., Angelo, A. A., Muñoz, V., Hocquemiller, R., and Cavé, A. (1993a). Effect of some bisbenzylquinoline alkaloids on American Leishmania sp. in BALB/c mice. Phytother. Res. 7, 281–284. doi:10.1002/ptr.2650070404
Fournet, A., Angelo, A. A., Muñoz, V., Hocquemiller, R., Roblot, F., Cavé, A., et al. (1994a). Antiprotozoal activity of quinoline alkaloids isolated from Galipea longiflora, a Bolivian plant used as a treatment for cutaneous leishmaniasis. Phytother. Res. 8, 174–178. doi:10.1002/ptr.2650080312
Fournet, A., Barrios, A. A., and Muñoz, V. (1994b). Leishmanicidal and trypanocidal activities of bolivian medicinal plants. J. Ethnopharmacol. 41, 19–37. doi:10.1016/0378-8741(94)90054-x
Fournet, A., Barrios, A. A., Muñoz, V., Hocquemiller, R., Cave, A., and Bruneton, J. (1993b). 2-Substituted quinoline alkaloids as potential antileishmanial drugs. Antimicrob. Agents Chemother. 37, 859–863. doi:10.1128/aac.37.4.859
Fournet, A., Ferreira, M. E., Rojas de Arias, A., Guy, I., Guinaudeau, H., and Heinzen, H. (2007). Phytochemical and antiprotozoal activity of Ocotea lancifolia. Fitoterapia 78, 382–384. doi:10.1016/j.fitote.2007.03.003
Fournet, A., Ferreira, M. E., Rojas de Arias, A., Torres de Ortiz, S., Fuentes, S., Nakayama, H., et al. (1996). In vivo efficacy of oral and intralesional administration of 2-substituted quinolines in experimental treatment of new world cutaneous leishmaniasis caused by Leishmania amazonensis. Antimicrob. Agents Chemother. 40, 2447–2451. doi:10.1128/aac.40.11.2447
Fournet, A., Gantier, J. C., Gautheret, A., Leysalles, L., Munos, M. H., Maryrargue, J., et al. (1994c). The activity of 2-substituted quinoline alkaloids in BALB/c mice infected with Leishmania donovani. J. Antimicrob. Chemother. 33, 537–544. doi:10.1093/jac/33.3.537
Fournet, A., Muñoz, V., Manjon, A. M., Angelo, A., Hocquemiller, R., Cortes, D., et al. (1988). Activité antiparasitaire d´alcaloides bisbenzylisoquinoléiques. I. Activité in vitro sur des promastigotes de trois sourches de Leishmania. J. Ethnopharmacol. 24, 327–335. doi:10.1016/0378-8741(88)90162-6
Ghosh, A. K., Bhattacharyya, F. K., and Ghosh, D. K. (1985). Leishmania donovani: amastigote inhibition and mode of actior of berberine. Exp. Parasitol. 60, 404–413. doi:10.1016/0014-4894(85)90047-5
Gopinath, V. S., Pinjari, J., Dere, R. T., Verma, A., Vishwakarma, P., Shivahare, R., et al. (2013). Design, synthesis and biological evaluation of 2-substituted quinolines as potential antileishmanial agents. Eur. J. Med. Chem. 69, 527–536. doi:10.1016/j.ejmech.2013.08.028
Gopinath, V. S., Rao, M., Shivahare, R., Vishwakarma, P., Ghose, S., Pradhan, A., et al. (2014). Design, synthesis, ADME characterization and antileishmanial evaluation of novel substituted quinoline analogs. Bioorg. Med. Chem. Lett. 24, 2046–2052. doi:10.1016/j.bmcl.2014.03.065
Iglarz, M., Baune, B., Gantier, J. C., Hocquemillerc, R., and Farinotti, R. (1998). Determination of 2-n-propylquinoline in mouse plasma and liver by high-performance liquid chromatography. J. Chromatogr. B 714, 335–340. doi:10.1016/s0378-4347(98)00219-9
Jézéquel, G., Cardoso, L. N. d.F., Olivon, F., Dennemont, I., Apel, C., Litaudon, M., et al. (2022). Synthesis and anti-Leishmanial properties of quinolones derived from zanthosimuline. Molecules 27, 7892. doi:10.3390/molecules27227892
Karamchandani, P. V. (1927). An analysis of 337 cases of oriental sore treated by various methods. Indian Med. Gaz. 62, 558.
Kruth, S., Schibajew, L., and Nett, M. (2022). Biocatalytic production of the antibiotic aurachin D in Escherichia coli. Amb. Express 12, 138. doi:10.1186/s13568-022-01478-8
Kruth, S., Zimmermann, C. J. M., Kuhr, K., Hiller, W., Lütz, S., Pietruszka, J., et al. (2023). Generation of aurachin derivatives by whole-cell biotransformation and evaluation of their antiprotozoal properties. Molecules 28, 1066. doi:10.3390/molecules28031066
Kunze, B., Höfle, G., and Reichenbach, H. (1987). The aurachins, new quinoline antibiotics from myxobacteria: production, physico-chemical and biological properties. J. Antibiot. 40, 258–265. doi:10.7164/antibiotics.40.258
Mollataghi, A., Coudiere, E., Hadi, A. H. A., Mukhtar, M. R., Awang, K., Litaudon, M., et al. (2012). Anti-acetylcholinesterase, anti-α-glucosidase, anti-leishmanial and anti-fungal activities of chemical constituents of Beilschmiedia species. Fitoterapia 83, 298–302. doi:10.1016/j.fitote.2011.11.009
Monzote, L. (2009). Current treatment of leishmaniasis: a review. Open Antimicrob. Agents J. 1, 9–19. doi:10.2174/1876518100901010009
Nakayama, H., Loiseau, P. M., Bories, C., Torres de Ortiz, S., Schinini, A., Serna, E., et al. (2005). Efficacy of orally administered 2-substituted quinolines in experimental murine cutaneous and visceral leishmaniases. Antimicrob. Agents Chemother. 49, 4950–4956. doi:10.1128/aac.49.12.4950-4956.2005
Naman, C. B., Gupta, G., Varikuti, S., Chai, H., Doskotch, R. W., Satoskar, A. R., et al. (2015). Northalrugosidine is a bisbenzyltetrahydroisoquinoline alkaloid from Thalictrum alpinum with in vivo antileishmanial activity. J. Nat. Prod. 78, 552–556. doi:10.1021/np501028u
Saha, P., Bhattacharjee, S., Sarkar, A., Manna, A., Majumder, S., and Chatterjee, M. (2011). Berberine chloride mediates its anti-leishmanial activity via differential regulation of the mitogen activated protein kinase pathway in macrophages. PLoS ONE 6, e18467. doi:10.1371/journal.pone.0018467
Saha, P., Sen, R., Hariharan, C., Kumar, D., Das, P., and Chatterjee, M. (2009). Berberine chloride causes a caspase-independent, apoptotic-like death in Leishmania donovani promastigotes. Free Radic. Res. 43, 1101–1110. doi:10.1080/10715760903186124
Staerk, D., Lemmich, E., Christensen, J., Kharazmi, A., Olsen, C. E., and Jaroszewski, J. W. (2000). Leishmanicidal, antiplasmodial and cytotoxic activity of indole alkaloids from Corynanthe pachyceras. Planta Med. 66, 531–536. doi:10.1055/s-2000-8661
Steverding, D. (2017). The history of leishmaniasis. Parasit. Vectors 10, 82. doi:10.1186/s13071-017-2028-5
Torres Suarez, E., Granados-Falla, D. S., Robledo, S. M., Murillo, J., Upegui, Y., and Delgado, G. (2020). Antileishmanial activity of synthetic analogs of the naturally occurring quinolone alkaloid N-methyl-8-methoxyflindersin. PLoS ONE 15, e0243392. doi:10.1371/journal.pone.0243392
Vennerstrom, J. L., Lovelace, J. K., Waits, V. B., Hanson, W. L., and Klayman, D. L. (1990). Berberine derivatives as antileishmanial drugs. Antimicrob. Agents Chemother. 1990, 918–921. doi:10.1128/aac.34.5.918
Wei, J. S. (1965). A study of the constituents of the roots of Rauwolfia yunnanensis Tsiang. I. Isolation of ajmalicine and reserpine. Yao Xue Xue Bao 12, 429–434.
Wenning, B., Price, H., Nuwangi, H., Reda, K. T., Walters, B., Ehsanullah, R., et al. (2022). Exploring the cultural effects of gender on perceptions of cutaneous leishmaniasis: a systematic literature review. Glob. Health Res. Policy 7, 34. doi:10.1186/s41256-022-00266-y
WHO (2024). World health organization: leishmaniasis. Available online at: https://www.who.int/news-room/fact-sheets/detail/leishmaniasis (accessed on December 17, 2024).
Keywords: 2-substituted quinoline, furoquinoline, polyhydroquinoline, bisbenzylisoquinoline, quinolinone
Citation: Yaluff G, Herrera L, Rolón MS, Vega C and Cerecetto H (2025) The quinoline framework and related scaffolds in natural products with anti-Leishmania properties. Front. Chem. 13:1571067. doi: 10.3389/fchem.2025.1571067
Received: 04 February 2025; Accepted: 27 March 2025;
Published: 25 April 2025.
Edited by:
Gildardo Rivera, National Polytechnic Institute (IPN), MexicoReviewed by:
Silvia Pérez Silanes, University of Navarra, SpainCopyright © 2025 Yaluff, Herrera, Rolón, Vega and Cerecetto. This is an open-access article distributed under the terms of the Creative Commons Attribution License (CC BY). The use, distribution or reproduction in other forums is permitted, provided the original author(s) and the copyright owner(s) are credited and that the original publication in this journal is cited, in accordance with accepted academic practice. No use, distribution or reproduction is permitted which does not comply with these terms.
*Correspondence: Hugo Cerecetto, aGNlcmVjZXR0b0BjaW4uZWR1LnV5