- Universidad Nacional de Educación a Distancia (UNED), Escuela Técnica Superior de Ingenieros Industriales, Departamento de Ingeniería Energética, Madrid, Spain
During the commissioning and start-up phases of IFMIF-DONES, the 40 MeV deuteron beam will be stopped in a conical beam stopper made of copper, giving rise to intense neutron fields. Accurate coupled deuteron-neutron transport simulations are required in order to prevent the potential risks to the workers and equipment involved. However, the experimental data concerning the neutron emission under these circumstances is scarce and limited to a particular range of energies. Different deuteron nuclear data libraries such as JENDL-5 and special files of TENDL-2021 exhibit some differences on the spectrum of the emitted neutrons. The differences are present in the high energy tails, beyond the available experimental data. Calculations of the prompt neutron fields have been performed employing both deuteron data libraries, as well as employing an estimation of the neutron energy-angle distribution derived from experimental data at different irradiation energies. Even though the high energy tails represent a small part of the flux, they have a major impact on the dose rates. The disparity of the results evinces large uncertainties in the IFMIF-DONES beam dump design process. Further experiments are required in order to resolve the differences among nuclear data libraries and reduce the uncertainty.
1 Introduction
In future fusion power plants, the radiation-exposed components will have to withstand severe operational conditions. Safe design, construction, and licensing of future nuclear fusion facilities will demand an understanding of the materials degradation under the neutron bombardment. The International Fusion Materials Irradiation Facility-DEMO Oriented Neutron Source (IFMIF-DONES) is a facility dedicated to the generation of a neutron source capable of producing irradiation conditions like those anticipated for DEMO [1]. To generate this neutron source, IFMIF-DONES consists in a deuteron accelerator where the beam impinges on a liquid lithium target. The nuclear reactions induced by the accelerated deuterons generate a large number of neutrons that in turn interact with the material samples located in test modules [2].
However, during commissioning stages and start up phases, the accelerator will count with a pulsed beam at low duty cycle, a 40 MeV deuteron beam with an intensity of 1.25 mA. DONES will have a dedicated Beam Dump installed on a lateral line of the High Energy Beam Transport Line (HEBT), to allow beam adjustment and beam characterization in these cases. The Beam Dump is made up of a cartridge containing a copper cone (beam stopper), a shroud to provide the path for coolant, and a rear flange to provide mechanical support, as well as radiation shielding [3]. The material choice for the beam stopper was based on the Linear IFMIF Prototype Accelerator (LIPAc) design, where copper was selected based on its neutron production and stresses against a temperature gradient feature [4]. The purpose of the Beam Dump is to stop the particles and dissipate the deposited energy. When the accelerated deuterons hit the beam stopper, they will give rise to a neutron source with an approximate intensity of 2.3 E + 13 n/s, and to a subsequent intense neutron field. Inside the beam transport room, where the Beam Dump is located, the effective dose rates can reach values higher than 100 mSv/h since it is classified as forbidden area. However, the airlocks in the neighboring rooms are classified as controlled areas of free permanence, and must present dose rates lower than 10 μSv/h.
Computational simulations provide assessments during the design of the components and rooms. The nuclear analysis of the IFMIF-DONES beam dump is needed to predict if the dose rate limits are met, and to guide the decision making in case they are not. Hence, the simulations are expected to provide accurate descriptions of the radiation fields. With this aim, the nuclear data used in these simulations need to be as accurate as possible.
The currently available integral experiments regarding the neutron emission during a 40 MeV deuteron irradiation of copper samples provide a scarce source of experimental data which only ranges up to an emitting energy of 27.5 MeV. Different copper nuclear data libraries for incident deuterons such as the JENDL-5 [5] and special files of the TENDL-2021 [6] present a fairly good agreement with the available experimental data. Nonetheless, for emitting energies higher than 25 MeV, these two libraries show some differences. Although the high energy tails in the neutron spectrum represent a small part of the flux, the present work evaluates its impact on the design of the beam dump, so the dose rate limits in controlled areas of free permanence are always respected.
2 Neutron emission during irradiation of natural copper
The design of the IFMIF-DONES beam dump requires an accurate description of the radiation fields. From a radiological point of view, the secondary neutron field produced by the interaction of the deuteron beam with matter is the field of most concern for the dose rates during operation. Thus, an accurate energy-angle distribution, as well as an accurate total neutron production after a deuteron nuclear interaction are required. However, experimental data regarding the neutron production during the irradiation of a copper target by a 40 MeV deuteron beam is not abundant.
In the work of Schweimer [7], the neutron spectrum in the forward direction was measured and compared with theoretical results for nuclear and Coulomb break-up of deuterons. More precisely, this work provides experimental data concerning the neutrons emitted in the frontal direction (0°) in the energy range comprised between 11.5 and 27.5 MeV. Deuteron breakup reactions cause the emission of neutrons with a narrow energy distribution centered approximately at one-half of the incident deuteron energy. These fragments are also emitted with an angular distribution focused towards forward angles.
To represent the particle emission caused by this type of reactions, Kalbach proposed a phenomenological breakup kinematics [8]. Sauvan developed the methodology to include a breakup parameter in the ENDF format [9], a format developed for the storage and retrieval of evaluated nuclear data to be used for applications of nuclear technology to reconstruct physical quantities such as cross sections and angular distributions. The breakup parameter allows a transport code deciding whether a neutron emission is caused by a breakup reaction and using the appropriate angular distribution. In that work it was proven how, in computational simulations, the forward-peaked neutron emission caused by the deuteron breakup reactions must be considered in order to achieve a good agreement with the experimental data. Special files of TENDL-2021 have been developed following the methodology proposed by Sauvan, even though it is not standard ENDF format yet. Only the TENDL-2021 special files with neutron emission from breakup reactions are considered in the present work.
On the other hand, JENDL-5 also considers the breakup reactions. In this case, the deuteron breakup process is accounted by the continuum-discretized coupled-channels theory and Glauber model. In this case, the double-differential cross-sections for the emitted neutrons does not use a parametrized systematics for the angular distribution, resulting in large data files. Another drawback of this library is that only nine nuclides (6Li, 7Li, 9Be, 12C,13C, 27Al, 63Cu, 65Cu, and 93Nb) are currently available.
Both deuteron libraries have been employed to simulate the Schweimer experiment, obtaining the results presented in Figure 1. MCUNED code [10] has been employed to perform de simulations.
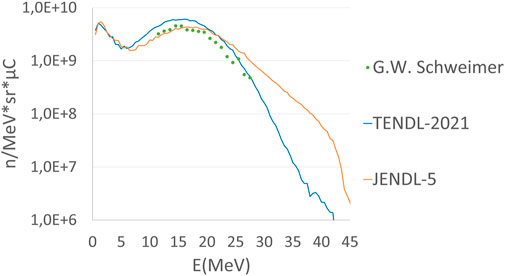
FIGURE 1. Spectrum of the neutrons emitted at 0° during a 40 MeV deuteron irradiation of natural copper.
The total neutron yield at 0° obtained in the simulation employing the TENDL library is 14.5% higher than the obtained employing JENDL. Despite this difference in the total neutron emission at the forward angle, the most relevant differences between the two libraries can be found at emitting energies beyond the experimental data.
On the one hand, the results obtained with the JENDL libraries seem to overestimate the neutron emission at energies higher than 20 MeV, while the TENDL results are tighter. On the other hand, neutron spectrum in the TENDL results presents a quick falloff for energies higher than 20 MeV while the slope in the JENDL results seem to be closer to the trend of the experimental data. The neutrons emitted at energies exciding the experimental data represents only a 1% of the total neutron production in the simulation with TENDL, and a 5% of the total neutron production in the simulation with JENDL. Nonetheless, these small fraction of the neutrons produced during the irradiation of copper could have a great impact on the design of the IFMIF-DONES beam dump due to their high energy.
Since experimental data on the neutron emission produced by 40 MeV deuteron irradiation is scarce, experiments performed at other deuteron energies can be a useful source of information about the neutron yield in the DONES beam dump. In this sense, it is worth highlining the integral experiment presented in the work of Shin et al. [11].
In the work of Shin, a copper foil is irradiated by a 33 MeV deuteron beam. In this case, the experimental data presents a better energy resolution, wider energy range, and the neutron spectrum is measured at different emitting angles. Whilst the experiment of Schweimer comprises an emitting neutron energy range from 11.5 to 27.5 MeV with 17 points, the work of Shin comprises an emitting neutron energy range from 2.45 to 32.45 MeV with 31 points. The experiment has been simulated making use of TENDL and JENDL deuteron libraries, obtaining the results at emitting angles of 0°, 15°, 45°, and 75° presented in Figures 2–5 respectively.
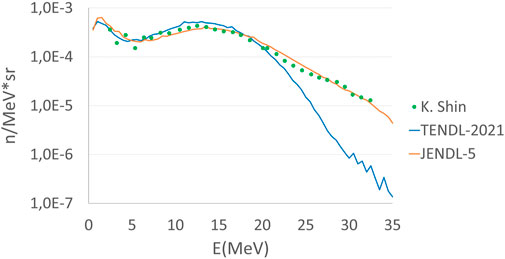
FIGURE 2. Spectrum of the neutrons emitted at 0° during a 33 MeV deuteron irradiation of natural copper.
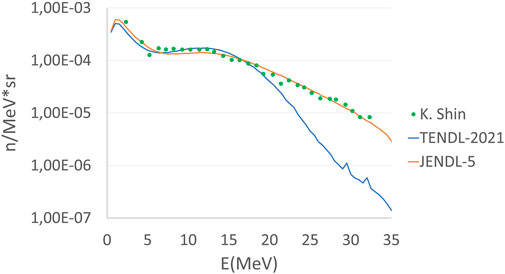
FIGURE 3. Spectrum of the neutrons emitted at 15° during a 33 MeV deuteron irradiation of natural copper.
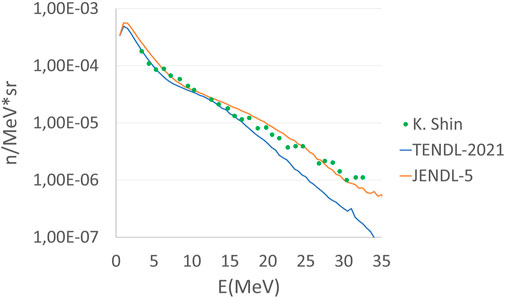
FIGURE 4. Spectrum of the neutrons emitted at 45° during a 33 MeV deuteron irradiation of natural copper.
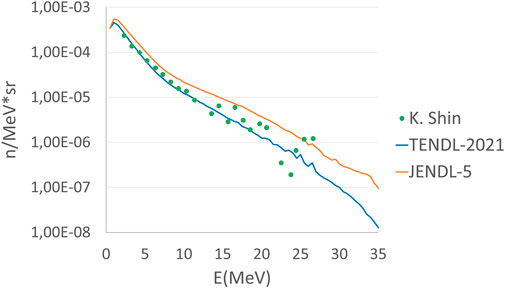
FIGURE 5. Spectrum of the neutrons emitted at 75° during a 33 MeV deuteron irradiation of natural copper.
The information related to the error of the experimental data of the Shin experiment is only provided for some neutron energies at emitting angles of 15°, 45°, and 75°. TENDL yields results within the experimental error for energies lower than 20 MeV. At higher energies, TENDL tends to underestimate the high energy neutron production, especially for the emitting angles close to the direction of the impinging deuteron. JENDL seems to perfectly follow the experimental data and results in neutron emission which are always within the experimental error, except for some atypical cases at 75°. This behavior is surely found with 40 MeV deuteron as well. The work of Shin also provides useful information concerning the neutron emission angular distribution, integrated over the energy. Comparing the simulations against the experimental data, Figure 6, it can be observed how the JENDL library tends to overestimate the total neutron production.
In this case, the TENDL seems to better reproduce the angular distribution and the total neutron production. However, since the spectrum shows a clear underestimation of the high energy tails, especially for the neutrons emitted at directions close to the frontal angles 0° and 15°, see Figures 2, 3, respectively, a new neutron emission double-differential spectra has been developed with the srcUNED-Ac source module [12], to be published in the Proceedings of the SATIF-15. This module allows reproducing secondary radiation sources without the need to transport the primary source particle. Here, the secondary neutron source for the srcUNED-Ac module has been based on TENDL-2021, but the double-differential spectra has been subsequently modified.
This new double-differential spectra includes an extrapolation of the neutron spectrum for energies from 25 MeV which applies only to a conical solid angle of 15° around the beam direction, to mimic the experimental data shown in the Shin experiment. The extrapolation is exponential with the same coefficient as the regression applied to the 33 MeV experimental data. The spectrum has been extended up to 45 MeV to account for neutron production from possible exoenergetic reactions such as the 63Cu(d,n)64Zn or the 65Cu(d,n)66Zn. The results of simulation of the Schweimer experiment with the extrapolated double-differential are presented in Figure 7 in comparison with TENDL and JENDL.
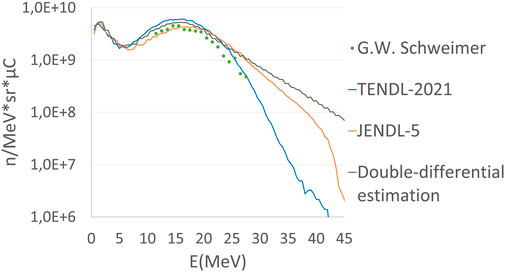
FIGURE 7. Spectrum of the neutrons emitted at 0° during a 40 MeV deuteron irradiation of natural copper.
The spectrum obtained with the new neutron emission estimation seems to result in a slightly higher production of neutrons over 30 MeV than the JENDL. This increase in the high energy neutron emission, which represent less than a 4% of the total neutron emission at 0°, is due to the extrapolation made from the data at 33 MeV, that yields a slope slightly lower than the JENDL.
3 Sensitivity of the neutron dose rates during operation
The goal of the present work is to evaluate the impact of the discrepancies in the nuclear data on the characterization of the radiological conditions in the DONES accelerator system during the commissioning operations. As already anticipated in the introduction, the High Energy Beam Transport Line and Beam Dump System (HEBT and BD) is in charge of guiding the deuteron beam towards the Lithium Target [13]. The HEBT and BD System will include a secondary line with a dedicated Beam Dump where the pulsed beam at low duty cycle will be stopped during the commissioning and start-up phases. The chosen design for the beam dump cartridge and shielding is the one used for IFMIF-LIPAc [4].
This secondary system will be located at the beam transport room, where some of the highest dose rates will be found, as presented in Ref. [14]. This previous nuclear analysis was carried out making use of the TENDL-2015 deuteron data libraries, which do not consider the breakup reactions. It concluded that, with the layout proposed at that time, the dose rates in the controlled areas of free permanence outside the beam transport room met the limitation on 10 μSv/h. The layout of the building has evolved since that analysis and the distance from the rear side of the beam dump to the closest wall has been increased, so the dose rates outside the beam transport room should be expected to be lower than the doses calculated in Ref. [13]. However, simulations with the newly available nuclear data libraries which do consider the breakup reactions will produce a higher amount of neutrons capable of escaping the cartridge and the beam transport room walls.
In the present work, the MCUNED code [10] has been employed to calculate the dose rates in the airlock outside the beam transport room behind the beam dump, with the use of automated variance reduction techniques. The beam has been represented by a 40 MeV deuteron point source located in the center of the last quadrupole of the beam dump transport line, with a source intensity equivalent to 1.25 mA and a conical solid angle centered in the beam line causing the irradiation of the beam stopper. The simulation has been repeated three times, making use of the different deuteron nuclear data sets. Thus, it allows comparing the nuclear responses associated to the neutron fields and determining its sensitivity to the nuclear data. Neutron induced nuclear reactions have been calculated with the FENDL-3.1c/d [15] data library in all cases. The radiation doses are expressed as ambient dose equivalents with ICRP-74 dose flux coefficients. Figure 8 presents the dose rates along the airlock obtained for a wall thickness of 1.25 m.
In this figure it can be observed that for the assumed wall thickness, independently of the nuclear data, the dose rates outside the beam transport room would be higher than 10 μSv/h in all three cases. However, the discrepancies, especially in the forward direction, are so significant that the maximum dose rate values differ by a factor of more than 3.5. Figure 9 shows the energy at the moment of their birth from a deuteron induced reaction of the neutrons that contribute to the dose rate in the airlock.
The distributions presented in Figure 9 should be understood as the product of two functions. The first function is the spectrum of the neutrons at the moment of their emission. The second function is the probability of escaping the concrete walls of the beam transport room depending on the neutron energy at its birth. In the simulation with TENDL, the maximum of the distribution is shifted to a lower energy. The cause of this shift is spectrum of neutrons emitted using the TENDL library, which quickly decays for energies higher than 20 MeV as it was seen in Figure 1. Nonetheless, the neutrons emitted at energies higher than 27.5 MeV (the last available experimental data) represents only a 1% of the total neutron production in the simulation with TENDL, but they are responsible for more than 33% of the outside dose. When the simulation was performed with JENDL and the new double differential estimation, the neutrons emitted at energies higher than 27.5 MeV represent a 5% of the total neutron yield, but they are responsible for around the 75% of the outside dose. These facts clarify how the high energy tail have a great impact on the dose rates outside the beam transport room. It is also made clear how the lack of experimental data with respect to the neutron production at energies higher than 27.5 MeV yields large uncertainties on the calculation.
The discrepancies on the nuclear data have a great impact on the design of the beam dump, since the fact that calculating a dose rated higher than 10 μSv/h in a controlled area of free permanence motivates the increasing of the wall thickness. Consequently, the increase of the wall thickness needed to respect the dose rate limits have been calculated depending on the nuclear data. In Figure 10 the evolution of the dose rate as it penetrates the wall of the beam transport room is presented.
With the slope of the curves in Figure 10, it can be calculated that the wall of the beam transport room should be increased by 6.5 cm corresponding to the results from the TENDL library, 28.9 cm based on the results from the JENDL library, and 25 cm according to the results obtained with estimated double-differential spectra. But beside this difference, the influence of the high energy tails can be seen in the slope of the curves in the figure. Since the estimation developed in this work estimates a higher neutron production at energies over 27.5 MeV, this curve has the lowest slope. Even though the dose rate outside the beam transport room calculated with the JENDL is the highest due to its larger total neutron production, if the wall were thick enough, an intersection point between the two curves would be found. Small differences in regions of the energy-angle phase space with a lack of experimental data have a clear influence in the design process.
4 Conclusion
This work reviewed the radiological conditions of the DONES AS during the commissioning stage.
Previous nuclear analysis employed the nuclear data available at the time, which presented a lack from accurate representation of the angular distribution of neutron emission. Currently, two deuteron libraries exhibit good agreement with the experimental data concerning the neutron emission during the irradiation of copper targets by a 40 MeV deuteron beam. However, some differnces can be found in spectrum at emitting energies beyond the range covered by the experimental data. An estimation of the neutron energy-angle distribution derived from experimental data at different irradiation energies has been developed.
Even though, it represents a small part of the flux, the differences are so significant, that the computed dose rates outside the beam transport room differs by a factor of more than 3.5. This difference may also have a direct impact on the residual doses around this part of the beam dump and surrounding area since neutron escapes determine the residual dose field from activation by neutrons. This disparity of results, and the scarcity of experimental data to test with, indicates a large uncertainty in the response functions associated to the neutron flux. Further experiments are required in order to reduce the uncertainty.
Data availability statement
The original contributions presented in the study are included in the article/Supplementary Material, further inquiries can be directed to the corresponding author.
Author contributions
VL and FO contributed to conception and design of the study. FO developed the nuclear data estimation. VL performed the simulations. PS and VL contributed to the analysis of the results. VL wrote the first draft of the manuscript. All authors contributed to the article review and approved the submitted version.
Funding
This work has been carried out partially within the framework of the EUROfusion Consortium, funded by the European Union via the Euratom Research and Training Programme (Grant Agreement No 101052200—EUROfusion). Views and opinions expressed are however those of the author(s) only and do not necessarily reflect those of the European Union or the European Commission. Neither the European Union nor the European Commission can be held responsible for them. This work has been funded by Spanish Ministery of Science and Innovation under project reference PID2019-106775RB-I00.
Acknowledgments
Authors acknowledge the support of the Escuela Técnica Superior de Ingenieros Industriales (UNED) of Spain, project 2023-ETSII-UNED-03.
Conflict of interest
The authors declare that the research was conducted in the absence of any commercial or financial relationships that could be construed as a potential conflict of interest.
Publisher’s note
All claims expressed in this article are solely those of the authors and do not necessarily represent those of their affiliated organizations, or those of the publisher, the editors and the reviewers. Any product that may be evaluated in this article, or claim that may be made by its manufacturer, is not guaranteed or endorsed by the publisher.
References
1. Ibarra A, Arbeiter F, Bernardi D, Cappelli M, García A, Heidinger R, et al. The IFMIF-DONES project: Preliminary engineering design. Nucl Fus (2018) 58:105002. doi:10.1088/1741-4326/aad91f
2. Ibarra A, Arbeiter F, Bernardi D, Krolas W, Cappelli M, Fischer U, et al. The European approach to the fusion-like neutron source: The IFMIF-DONES project. Nucl Fus (2019) 59:065002. doi:10.1088/1741-4326/ab0d57
3. Sánchez-Herranz D, Ortego P, Nomen O, Brañas B, Ogando F, Sauvan P, et al. Design and configurations for the shielding of the beam dump of IFMIF DONES. Fusion Eng Des (2020) 153:111475. doi:10.1016/j.fusengdes.2020.111475
4. Brañas B, Arranz F, Nomen O, Iglesias D, Ogando F, Parro M, et al. The LIPAc beam dump. Fus Eng (2018) 127:127–38. doi:10.1016/j.fusengdes.2017.12.018
5. Iwamoto O, Iwamoto N, Shibata K, Ichihara A, Kunieda S, Minato F, et al. Status of JENDL. EPJ Web of Conferences (2020) 239:09002–6. doi:10.1051/epjconf/202023909002
6. Koning AJ, Rochman D, Sublet J, Dzysiuk N, Fleming M, van der Marck S. Tendl: Complete nuclear data library for innovative nuclear science and technology. Nucl Data Sheets (2019) 155:1–55. doi:10.1016/j.nds.2019.01.002
7. Schweimer GW. Fast neutron production with 54 MeV deuterons. Nucl Phys A (1967) 100:537–44. doi:10.1016/0375-9474(67)90122-4
8. Kalbach C. Phenomenological model for light-projectile breakup. Rev C (2017) 95:014606. doi:10.1103/physrevc.95.014606
9. Sauvan P, Koning A, Ogando F, Sanz J. Implementation of a new energy angular distribution of particles emitted by deuteron induced nuclear reaction in transport simulations. EPJ Web of Conferences (2017) 146:02010. doi:10.1051/epjconf/201714602010
10. Sauvan P, Sanz J, Ogando F. New capabilities for Monte Carlo simulation of deuteron transport and secondary products generation. Nucl Instr Methods Phys Res A (2010) 614:323–30. doi:10.1016/j.nima.2009.12.084
11. Shin K, Hibi K, Fujii M, Uwamino Y, Nakamura T. Neutron and photon production from thick targets bombarded by 30-MeV p, 33-MeV d, 65-MeV 3He, and 65-MeV α ions: Experiment and comparison with cascade Monte Carlo calculations. Phys Rev C (1984) 29:1307–16. doi:10.1103/physrevc.29.1307
12. Ogando F, Sauvan P, Lopez V. Advanced Monte-Carlo method for prompt and residual radiation calculations in light-ion accelerators. United States: Michigan State University (2022).
13. Nomen O, Sanchez-Herranz D, Oliver C, Podadera I, Varela R, Ogando F, et al. Preliminary design of the HEBT of IFMIF DONES. Fusion Eng Des (2020) 153:111515. doi:10.1016/j.fusengdes.2020.111515
14. Fischer U, Bienkowska B, Drozdowicz K, Frisoni M, Mota F, Ogando F, et al. Neutronics of the IFMIF-DONES irradiation facility. Fus Eng Des (2019) 146:1276–81. doi:10.1016/j.fusengdes.2019.02.057
15. Forrest RA (2012). FENDL-3 library – summary documentation. Available at: https://www-nds.iaea.org/fendl/index.html.
Keywords: IFMIF-DONES, beam dump, nuclear data, breakup, neutronics
Citation: Lopez V, Ogando F and Sauvan P (2023) Sensitivity to nuclear data of the design of the IFMIF-DONES beam dump. Front. Phys. 11:1144057. doi: 10.3389/fphy.2023.1144057
Received: 13 January 2023; Accepted: 16 May 2023;
Published: 05 June 2023.
Edited by:
Ivan Alexander Kodeli, United Kingdom Atomic Energy Authority, United KingdomReviewed by:
Hatun Korkut, Sinop University, TürkiyeEugenia Naselli, Laboratori Nazionali del Sud (INFN), Italy
Copyright © 2023 Lopez, Ogando and Sauvan. This is an open-access article distributed under the terms of the Creative Commons Attribution License (CC BY). The use, distribution or reproduction in other forums is permitted, provided the original author(s) and the copyright owner(s) are credited and that the original publication in this journal is cited, in accordance with accepted academic practice. No use, distribution or reproduction is permitted which does not comply with these terms.
*Correspondence: Victor Lopez, dmxvcGV6QGluZC51bmVkLmVz