- 1Center for Instrument Sharing of the University of Pisa (CISUP), University of Pisa, Pisa, Italy
- 2Centro Pisano ricerca e implementazione clinica Flash Radiotheraphy (CPFR), Presidio S. Chiara, Pisa, Italy
- 3U.O. Fisica sanitaria, Azienda Ospedaliero-Universitaria Pisana, Pisa, Italy
- 4Department of Translational Research and New Technologies in Medicine and Surgery, University of Pisa, Pisa, Italy
- 5National Institute of Nuclear Physics (INFN), Section of Catania, Catania, Italy
- 6Department of Radiation Oncology, Azienda Ospedaliero-Universitaria Pisana, University of Pisa, Pisa, Italy
Background: The FLASH effect is a radiobiological phenomena characterized by the reduction of the damage to healthy tissues while maintaining iso-effectiveness on the tumour, obtained by delivering the entire radiation dose in less than 100–200 m and with average dose rate greater than 40–100 Gy/s. Despite the enormous interests of the scientific community, a series of issues must be addressed and overcome to reach its clinical implementation. One of these challenges is related to the dosimetry of ultra-high dose-per-pulse (UHDP) beams, which trigger the effect. The most used dosimeters to date in radiobiological experiments with ultra-high dose rate (UHDR) beams are the radiochromic films. However, a systematic and accurate study of their response by varying dose-per-pulse and dose rate over a wide range has never yet been done.
Purpose: To systematically investigate the response of EBT-XD radiochromic films under UHDR electron beam irradiations by varying different beam parameters independently and over a wide range and using flashDiamond as reference.
Materials and Methods: Thanks to a special research linac, average dose rate (ADR), dose-per-pulse (DP) and instantaneous dose rate (IDR) dependencies on film response have been individually investigated. The reference value of ADR, DP and IDR has been calculated by measuring the dose using a flashDiamond and knowing the temporal beam structure provided by the beam monitoring system of the Linac.
Results: Our results normalized to fD showed an overresponse of the radiochromic films, compared to a conventional irradiation, for ADR values higher than 1000 Gy/s and an IDR higher than 1 MGy/s which could represent a total dose overestimation of about 10%.
Discussion: The gafchromic film EBT-XD have their field of application in UHDP beam dosimetry, considering their excellent spatial resolution; However, particular attention must be paid when using this type of dosimeter for absolute dose measurements at very extreme values of IDR (>1 MGy/s).
1 Introduction
In recent years, the field of radiotherapy has undergone a significant paradigm shift with the emergence of a groundbreaking and promising modality known as FLASH radiotherapy. This innovative approach entails the administration of treatment at ultra high dose-rates (>40 Gy/s), a stark departure from conventional radiotherapy techniques with dose rates of approximately ∼ 1–5 Gy/min. The exploration and investigation of FLASH radiotherapy have garnered substantial attention from research teams worldwide, as evidenced by a growing body of literature spanning various studies [1–4]. However, despite the growing body of evidence supporting the existence and potential clinical significance of the FLASH effect, the underlying principles governing this phenomenon remain to be discovered. To design and execute an experiment focusing on the FLASH effect, meticulous attention must be directed towards establishing robust and rigorous dosimetry protocol. This involves a comprehensive consideration of all the fundamental beam parameters associated with the FLASH effect, such as the average dose-rate (ADR), dose-per-pulse (DP), and instantaneous dose-rate (IDR) [5]. Given the extraordinary irradiation conditions requisite for triggering the FLASH effect, conventional reference active dosimeters have proven inadequate [6]. Although corrective methodologies have been proposed [7, 8] they have fallen short in meeting the exacting dosimetry requirements indispensable for radiobiological investigations. In the last years numerous research groups are actively developing specialized FLASH active dosimeters [9–13] and new methods to correct conventional active dosimeters in UHDP conditions. In this context, passive dosimeters, such as radiochromic films, maintain their field of application, considering also their very good spatial resolution [14].
Radiochromic films, widely recognized for their dose rate independence [15–19], have been widely used both in characterizing FLASH sources [5, 20, 21] and in the dosimetry of radiobiological experiments [22–24]. Despite the inconvenience of a delayed dosimetric readout spanning one to 2 days, radiochromic films have enjoyed decades of application in the realm of radiotherapy. Various well-established protocols exist, and their clinical application has undergone comprehensive scrutiny.
Moreover, the advent of deep learning techniques for image classification could speedup and simplify the employment of large batches of films [25]. Determining the response of radiochromic films to UHDR irradiation is a relatively recent development [17, 19]. Several types of radiochromic films, including EBT3, EBT-XD, and OC1, have undergone testing with a range of UHDR sources, including pulsed electrons [17, 26] of varying energies and protons [19]. The collective evidence from these investigations suggests that radiochromic films do not exhibit a pronounced dependency on ADR, within an uncertainty margin of approximately 4% [17, 19]. However, these works have certain limitations due to the use of devices that do not allow for controlled variation of beam parameters independently of one another. Additionally, the findings have not been validated by alternative measurements using accurate dosimeters that are independent of dose rate. The present study aims to provide a comprehensive analysis of Gafchromic EBT-XD films, exploring their sensitivity to the primary beam parameters under UHDR conditions. We compare their response with measurements made using a prototype detector, which has been extensively tested and its dose rate independence verified through comparisons with DP-independent dosimeters [9].
2 Materials and Methods
The irradiations, performed using 9 MeV electron beams, were conducted at the ElectronFlash linac of the Centro Pisano for FLASH Radiotherapy (CPFR) [5], which features a unique triode gun and a dual ACCT monitoring system. Given the ability to maintain consistent irradiation setups while independently varying ADR, DP, and IDR (while preserving the same energy spectrum), this study aims to disentangle the distinct dependencies among these variables and reduce the uncertainties observed in previous studies.
2.1 Radiochromic film preparation
We employed Gafchromic EBT-XD radiochromic films (Ashland ECC), which exhibit an optimal response range when exposed to doses ranging from 0.4 cGy to 40 Gy. The films were cut into 1″ x 1″ squares from multiple 10″ x 8″ sheets, and each piece was assigned a unique number in the upper right corner to ensure consistent orientation during subsequent scans and irradiations. Our film batch was identified by the lot number 05262101. The films were stored in a tight black envelope kept in an environment with no radiation exposure. To acquire the film images, we used an Epson 10000XL flatbed scanner in transmission, whose reading panel was masked with a thick black sheet featuring a 1″× 1″aperture in the center. This was done to eliminate lateral light contributions and ensure that the same region of the flat panel captured the data, thereby minimizing scanner inhomogeneity. We configured the acquisition software to operate in positive film acquisition mode, resulting in 48-bit color images in lossless TIFF format with a resolution of 127 dpi. The scanner focus was set to 0.
Each film underwent three consecutive scans shortly before and 24 h after irradiation. While the impact of multiple scans on the film is negligible, this procedure enabled us to assess the error
Where PVbefore and PVafter the pixel values before and after the exposition of the radiochromic film to the radiation, averaged over the three repeated scans.
Once we characterized the beam output with the flashDiamond dosimeter, we generated a calibration curve by exposing the films to a defined range of doses, from 0.5 Gy to 60 Gy, with an ADR of 0.04 Gy/s and DP of 0.04 Gy (e−gun current of ∼4 mA). Films were positioned at the build-up point (13 mm in solid water sheets). The choice of using directly the electron beam rather than a photon beam (from another clinical machine) was made primarly to ensure the same energy spectrum cahracterized with the flashDiamond. This enabled us to establish a functional relationship between the dose and the netOD through data fitting. The relationship can be expressed as:
For this first set of measurements, we used two films that were uniformly irradiated by a 100 mm diameter flat beam (flatness of 4.03% [5]), and each dose measurement was repeated twice.
2.2 Dosimetric acquisition chain
We utilized a PTW flashDiamond (fD, SN:220,439, REF:TW60025) as an additional monitoring system alongside the beam current transformers (BCTs) integrated into the linac [5]. This detector is known for its capability to function effectively under FLASH irradiation conditions without displaying significant saturation effects and has been employed in UHDR linac commissioning procedures in the past [9, 27, 28]. We also tested the fD dependence of the PRF, and its response was totally indipendent of it in the range of interest (1–245 Hz). The fD was connected to a PTW UNIDOS electrometer. To prevent potential issues, an external box provided by PTW was used between the diamond detector’s triaxial cable plug and the electrometer’s signal input. This box contained a RC circuit, which helped to smooth the current pulses from the diamond prototype and prevent excessively high peak currents at the electrometer input [29]. This technique has been demonstrated to not produce any artifacts in the test results [27]. We positioned the EBT-XD films inside a RW3 plastic phantom at a water-equivalent depth corresponding to the R100 value (13 mm for a 4 mA e-gun current) obtained from the PDD (Percentage Dose Depth curve). The positioning depth of the films was kept constant, although the R100 value changes very slightly (by a total of ∼2 mm) when the e-gun current is increased to 100 mA.
The plastic phantom slabs were positioned perpendicular to the beam axis. Using a workbench with adjustable solid water thicknesses, we were able to fix the setup relative to the LINAC and adjust the distance along the beam axis. Centering was achieved by precisely moving the setup closer to or further from the applicator as needed. Additionally, to ensure accurate alignment with the beam center, a laser mounted at the center of the applicator was used to align each component (solid water, GAF film, and fD).
As can be seen in Figure 1, the fD detector was positioned behind the EBT-XD film (with a 1 mm distance of the two points of measurements due to the inherent thickess of the fD(9)), precisely aligned with its center, and enclosed in a 120 mm diameter PMMA cylindrical holder. Using this configuration, we irradiated the Gafchromic films and simultaneously obtained a charge reading from the fD, allowing us to establish an alternative (to the beam monitoring system based on an ACCT signal) correlation between the film response and the beam output. Furthermore, this setup enabled us to account for any output variation from the LINAC.
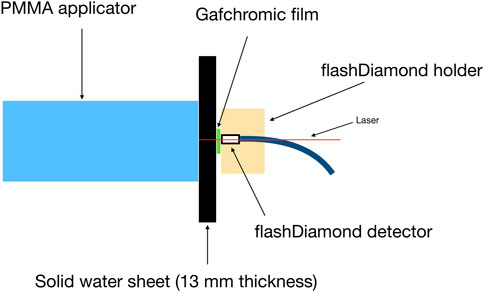
Figure 1. Experimental setup used for irradiations, showing the position of the flashDiamond (fD) relative to the GAF measurement position.
2.3 Irradiation parameters
The investigation of the film response was studied varying the ADR, the DP and the IDR to assess their individual effect. Throughout the irradiations, we maintained the same amount of total dose delivered to the film and small dose variations were corrected correcting the netOD to the flashDiamond charge reading (see Equation 3). Each measurement point was repeated five times with five different films. A summary of all the irradiation conditions is reported in Table 1. We explored the dependence on the ADR using two different DP values (obtained variying the e-gun current): 3.27 Gy and 13.18 Gy. A total number of 12 consecutive pulses were delivered for the lower DP setting while 3 pulses were used for the higher DP. The pulse duration was fixed (4 μs) leading to a constant IDR for each of the two DP settings. The PRF was varied from 1, 80, 160 and 245 Hz and the explored ADR ranged from 3.56 Gy/s to 4845 Gy/s.
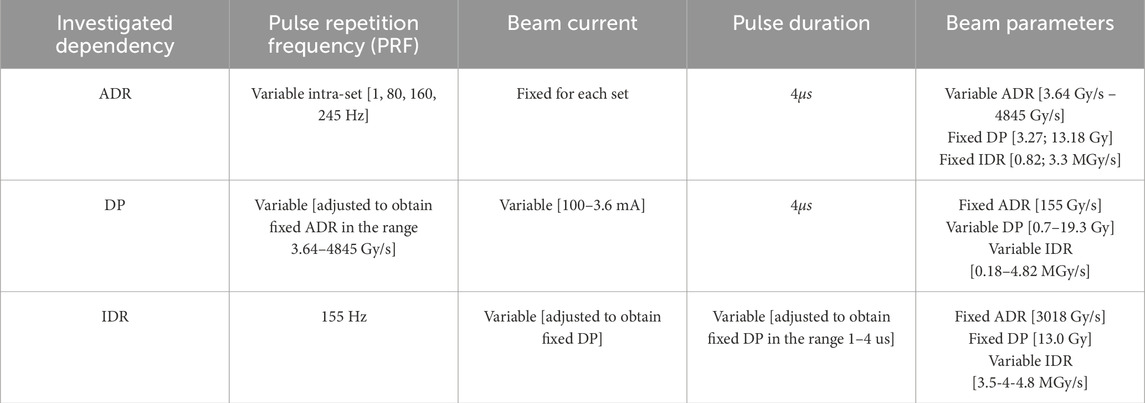
Table 1. Irradiation conditions of the key dosimetric parameters (i.e., Average Dose-Rate (ADR), Dose-per-Pulse (DP) and Instantaneous Dose-Rate (IDR)) along with irradiation parameters (i.e., beam current and pulse duration) explored in this study.
On the other hand, the possible dependence of the film response on the DP was investigated keeping the same total dose delivered and varying the PRF to have the same ADR of 155 Gy/s for all the irradiations, by adjusting the number of pulses. We modified the beam current to obtain seven different values of DP ranging from 0.7 Gy to 19.3 Gy. The IDR varied from 0.18 MGy/s to 4.82 MGy/s.
Finally, to investigate the possible effect of the IDR, we fixed a DP value of 13.0 Gy (obtained by changing three different beam currents and respectively adjusting the pulse duration). The pulse repetition frequency was maintained at a fixed value of 155 Hz, and the number of pulses delivered was set to three. Consequently, we obtained three distinct IDR values: 3.5, 4.0, and 4.8 MGy/s at fixed ADR (i.e., 3018 Gy/s) and DP.
3 Results
We obtained the calibration curve by fitting the measured dose as a function of the netOD using the curve presented in Equation 2. The fitting procedure accounted for uncertainties in both the netOD and the dose. The resulting calibration curve is shown in Figure 2, and the fitting parameters are presented in Table 2.
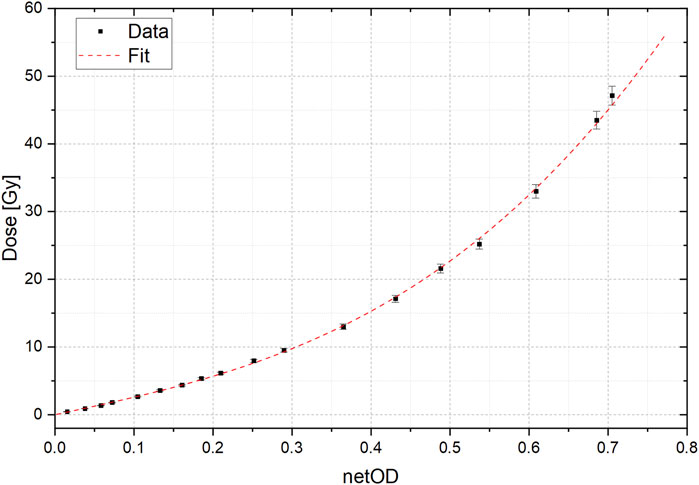
Figure 2. Calibration curve obtained comparing netOD values and the dose obtained using the flashDiamond. Error bars result from the uncertainty propagation of two different measurements for single dose value.
The sensitivity of the film at a given dose level was determined by performing a Taylor expansion of the function and then inverting it. For small differences in dose, we can obtain a corresponding difference in optical density using the Equation 4:
Considering that the dose delivered to the film for the netOD dependence study is about 40 Gy, a variation of 2% in dose would lead to a variation of 0.8% of the netOD.
The acquired data and the corresponding fit are presented in Figure 2.
To evaluate the influence of each irradiation parameter to the film response, we first investigated the dependence on ADR. The results of fixed total delivered dose at several ADR values and two different DP settings are shown in Figure 3. In particular, the corrected netOD (which represents the read netOD, corrected to the dose measured with the flashDiamond) as a function of the ADR for DP values of 3.27 Gy and 13.18 Gy is shown. For ADR less than about 1000 Gy/s, the response in terms of netOD (normalized to the fD charge) remained relatively constant for both DP settings, with data points fluctuating randomly. However, as ADR increased, we observed a slight (approximately 2.3%) overresponse normlized against fD, with data points consistently above the average values obtained for ADR lower than 1000 Gy/s. Given the observed slight dependence on the ADR, we conducted subsequent measurements by fixing it and investigate any other possible dependences.
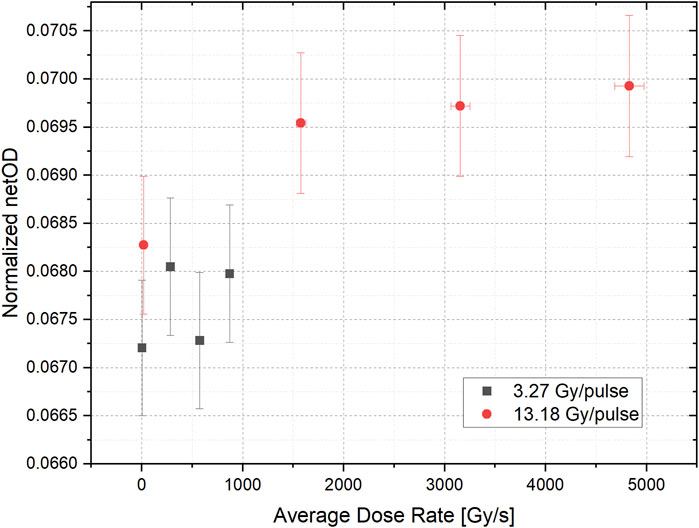
Figure 3. Normalized netOD to fD charge for two different dose-per-pulse values (3.27 Gy in black and 13.18 Gy in red) in function of the average dose-rate (ADR). Error bars result from the error proagation of netOD and dose obtained from fD.
Figure 4 illustrates the normalized response (against fD) of the films to varying DP for an ADR of 155 Gy/s. It is evident from the figure that the normalized netOD increases in a significative way, around 4.5%, starting monotonically from a DP of 5 Gy onward.
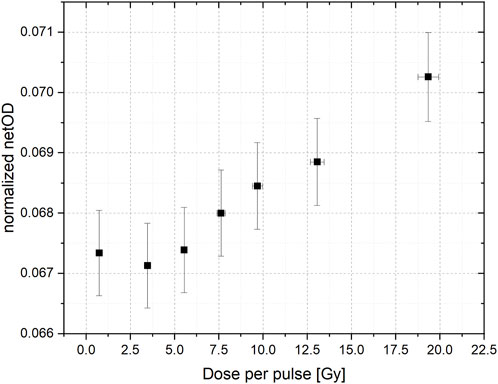
Figure 4. Normalized netOD to fD charge in function of the dose-per-pulse. Error bars result from the proagation. Error bars result from the error proagation of netOD and dose obtained from fD.
The data acquired at a constant DP of 13.0 Gy obtained by varying the IDR are presented in Figure 5, alongside the data obtained by converting the variable DP shown in Figure 4 into the corresponding IDR. It can be observed that there is a good agreement between the two datasets, and the difference between GAF reponse againts fD can be seen even when only the IDR is higher than 2 MGy/s. Moreover, the magnitude of this difference (compared to fD) is consistent with the findings from previous data analysis.
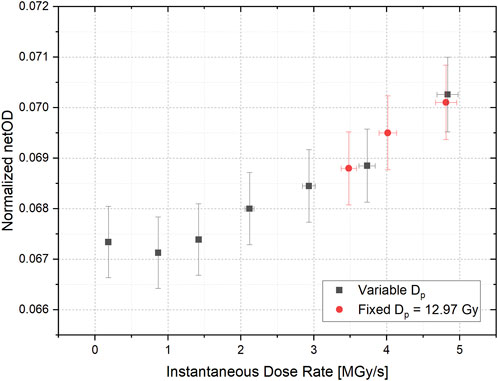
Figure 5. Normalized netOD to fD charge in function of the instantaneous dose-rate for a fixed dose-per-pulse (12.97 Gy in red) and variable dose-per-pulse (in black). Error bars result from the error proagation of netOD and dose obtained from fD.
4 Determination of uncertainties
Uncertainties must be determined and appropriately propagated for the following measurements:
- ADR: depends on the dose measured with flashDiamond and total irradiation time.
- DP: depends on the dose measured with flashDiamond.
- IDR: depends on the dose measured with flashDiamond and pulse duration measurement.
- OD: depends on all factors involved in processing radiochromic films.
Regarding dose measurement with the flashDiamond, literature indicates an uncertainty of approximately 3% with this detector [9]. The total irradiation time is related to the PRF, the number of pulses (np) and the pulse duration (tp). Thus, ADR is given by:
The uncertainty on ADR, if the pulse duration tp ≪ 1/PRF, is mainly due to the uncertainty in dose measurement. Nonetheless, if tp is not negligible with respect to 1/PRF, or in the case of IDR measurements, tp is measured using on-line measurements of IDR with flashDiamond and an oscilloscope (Rigol DS1074Z Plus). In this case, the uncertainty Δtp on tp corresponds to the scale on the oscilloscope and can be set so that Δtp ≪ tp, making it negligible compared to other sources of uncertainty.
Finally, we estimated OD uncertainty following the recommendations of AAPM TG 235 [30] using an estimation of about 5% for dose measurement with radiochromic films, a value consistent with previous literature [31, 32].
5 Discussion
The aim of this study was to assess the response of EBT-XD gafchromic film by independently varying the ADR, the DP and the IDR, by using the fD as reference. We found that, for a total delivered dose of about 40 Gy, there was a significant difference (∼2.3%) in the netOD (normalized against the fD reading) response between low (3.56 Gy/s) and high (4845 Gy/s) ADR values. The results also show a dependence of the film response on the IDR, which was investigated up to 4.82 MGy/s. A maximum netOD overresponse (compared to the fD) between 2.5% and 6.5% was found for the extreme IDR of 4.82 MGy/s when compared with IDR of 2 MGy/s. If future studies validate the use of fD as a reference, our finding implies a not negligible corresponding maximum dose overestimation of 7% ± 3.3% for a dose of 40 Gy obtained applying the dose calibration performed at low ADR and IDR conditions. The obtained data suggest that there are two contributing factors to the netOD overestimation. The first factor is linked to the ADR, which was evaluated for two different DP. Though not statistically significant, we observed an increasing trend from an ADR exceeding 1500 Gy/s up to approximately 5,000 Gy/s. The second factor is dependent on the IDR where the effect begins to manifest starting from around 1.5 MGy/s. The study of the IDR dependence was executed at an ADR of 3018 Gy/s, hence higher than the value found with the overresponse linked to the ADR. Given the irradiation conditions the two dependencies can be deemed independent.
The overall uncertainties in the irradiations were minimized thanks to the ElectronFlash linac that enabled us to maintain the same experimental setup and energy spectrum. We also verified, through a calibration curve executed with conventional irradiation parameters, that the selected dose value was within the optimal range of the film’s response, excluding phenomena linked to non-standard working conditions. This is consistent with the manufacturer specification and several other studies [33]. Any minor dose variations from the nominal one caused by delivery fluctuations were measured using as monitoring device the same dosimeter used for the reference dosimetry, the flashDiamond, and the resulting netOD was corrected accordingly.
Previous studies have already examined the response of radiochromic films to various beam parameters and concluded that gafchromic films do not exhibit any differences in their response for irradiation conditions. However, in Jaccard et al., the films were irradiated with uneven dose values and compared trough the calibration curve [17]. This approach could mask low entity dependency (below 5%) due to the increased uncertainty associated with the conversion of netOD values to dose values and the inherent uncertainty associated with selecting the appropriate irradiation condition for the calibration curve. As a result, we conducted our analysis directly on the netOD values to avoid these shortcomings.
More extreme irradiation condition (up to 109 Gy/s) were tested in Karsch et al. [15], but again, the comparison was made through the calibration curve (obtained at completely different setup from the one of the study). Meanwhile, a more recent study by Guan et al. [16] asserted that no dose rate dependence was found, but their study was limited to a maximum dose rate of 150 Gy/s. More recently, a study from Villoing et al. [19], started to observe an overresponse of the EBT-XD film to higher ADR and IDR (7500 Gy/s), but they were unable to test the response of the films to individual beam parameters. Our study is subject to certain limitations that warrant consideration.
The study would have been conceptually more precise by reconstructing complete dose-optical density curves across varying beam parameters (ADR, DP, IDP). However, this approach would be overly labor-intensive and practically unfeasible due to the totally different dosimetric steps across different DPs. Instead, we decided to fix the dose level for the irradiations, which allowed us to vary the parameters we wanted to investigate in an extreme manner. We then demonstrated that, for dose variations within 2% (the maximum variation recorded in our data), the resulting change in netOD is negligible and we applied a correction for minor dose variations. While not optimal, since the calibration curve parameters can shift with beam parameters, this correction is certainly more accurate than a linear approximation or no correction at all.
Our decision to focus exclusively on a single type of radiochromic film (EBT-XD) was predicated on its perceived suitability due to its more appropriate dose range response for FLASH irradiation, spanning up to 40 Gy. In contrast, the optimal dose range of EBT-3 (0.2–10 Gy) could potentially prove restrictive in certain extreme FLASH settings necessitating DP values exceeding the 10 Gy threshold. Consequently, opting for EBT-XD enabled us to operate within the optimal range delineated for the film’s efficacy (0.4–40 Gy) and conseguently have a superior response compared to the EBT3 film.
In our investigation, a uniform dose of approximately 40 Gy was administered across all films. This was motivated by our goal to create the most extreme irradiation conditions achievable within the operational limits of the linac, while independently manipulating the beam parameters. While existing studies suggest that observable effects may not emerge until doses reach 30 Gy [19], our decision to deliver higher doses was also informed by the potential insights that could be gained at higher values.
Further steps will be made to extend the results of this study to more extreme irradiation conditions and different beam energies. In conclusion, we advise caution when employing these films for absolute dosimetry within FLASH conditions—both in the context of FLASH source commissioning and radiobiological experiments.
Data availability statement
The raw data supporting the conclusions of this article will be made available by the authors, without undue reservation.
Author contributions
DD: Conceptualization, Data curation, Formal Analysis, Investigation, Methodology, Project administration, Resources, Software, Supervision, Validation, Visualization, Writing – original draft. LM: Conceptualization, Data curation, Formal Analysis, Investigation, Methodology, Project administration, Resources, Software, Supervision, Validation, Visualization, Writing – original draft, Writing – review and editing. AC: Data curation, Investigation, Methodology, Software, Validation, Visualization, Writing – original draft. MC: Data curation, Investigation, Methodology, Software, Validation, Visualization, Writing – original draft. TF: Methodology, Validation, Visualization, Writing – review and editing. GG: Methodology, Validation, Visualization, Writing – review and editing. NG: Methodology, Validation, Visualization, Writing – review and editing. AG: Visualization, Writing – review and editing, Methodology, Validation. GM: Data curation, Investigation, Methodology, Software, Validation, Visualization, Writing – review and editing. FP: Funding acquisition, Methodology, Validation, Visualization, Writing – original draft. JP: Data curation, Investigation, Methodology, Software, Validation, Visualization, Writing – original draft, Writing – review and editing. FR: Data curation, Investigation, Methodology, Software, Validation, Visualization, Writing – original draft, Writing – review and editing. FD: Conceptualization, Data curation, Formal Analysis, Funding acquisition, Investigation, Methodology, Project administration, Resources, Software, Supervision, Validation, Visualization, Writing – original draft, Writing – review and editing.
Funding
The author(s) declare that financial support was received for the research and/or publication of this article. This work was funded by European Union - Next Generation EU, Mission 4 Component 2 Inv. 1.5, ECS00000017 “Ecosistema dell’innovazione” Tuscany Health Ecosystem (THE), Spoke 1 “Advanced Radiotherapies and Diagnostics in Oncology”—CUP I53C22000780001.
Acknowledgments
We thank Fondazione Pisa for funding CPFR with the grant “prog. n. 134/2021”.
We also thank Minibeam Radiotheraphy (MIRO) INFN project for scientific collaboration.
Conflict of interest
The authors declare that the research was conducted in the absence of any commercial or financial relationships that could be construed as a potential conflict of interest.
The author(s) declared that they were an editorial board member of Frontiers, at the time of submission. This had no impact on the peer review process and the final decision.
Publisher’s note
All claims expressed in this article are solely those of the authors and do not necessarily represent those of their affiliated organizations, or those of the publisher, the editors and the reviewers. Any product that may be evaluated in this article, or claim that may be made by its manufacturer, is not guaranteed or endorsed by the publisher.
References
1. Bourhis J, Sozzi WJ, Jorge PG, Gaide O, Bailat C, Duclos F, et al. Treatment of a first patient with FLASH-radiotherapy. Radiother Oncol (2019) 139:18–22. doi:10.1016/j.radonc.2019.06.019
2. Montay-Gruel P, Petersson K, Jaccard M, Boivin G, Germond JF, Petit B, et al. Irradiation in a flash: unique sparing of memory in mice after whole brain irradiation with dose rates above 100 Gy/s. Radiother Oncol (2017) 124(3):365–9. doi:10.1016/j.radonc.2017.05.003
3. Favaudon V, Caplier L, Monceau V, Pouzoulet F, Sayarath M, Fouillade C, et al. Ultrahigh dose-rate FLASH irradiation increases the differential response between normal and tumor tissue in mice. Sci Transl Med (2014) 6:245ra93. doi:10.1126/scitranslmed.3008973
4. Durante M, BräUer-Krisch E, Hill M. Faster and safer? FLASH ultra-high dose rate in radiotherapy. Br J Radiol (2018) 91:1082. doi:10.1259/bjr.20170628
5. Di Martino F, Del Sarto D, Bass G, Capaccioli S, Celentano M, Coves D, et al. Architecture, flexibility and performance of a special electron linac dedicated to Flash radiotherapy research: electronFlash with a triode gun of the centro pisano flash radiotherapy (CPFR). Front Phys (2023) 11:1268310. doi:10.3389/fphy.2023.1268310
6. Di Martino F, Barca P, Barone S, Bortoli E, Borgheresi R, De Stefano S, et al. FLASH radiotherapy with electrons: issues related to the production, monitoring, and dosimetric characterization of the beam. Front Phys (2020) 8:570697. doi:10.3389/fphy.2020.570697
7. Di Martino F, Del Sarto D, Barone S, Giuseppina Bisogni M, Capaccioli S, Galante F, et al. A new calculation method for the free electron fraction of an ionization chamber in the ultra-high-dose-per-pulse regimen. Physica Med (2022) 103:175–80. doi:10.1016/j.ejmp.2022.11.001
8. Petersson K, Jaccard M, Germond JF, Buchillier T, Bochud F, Bourhis J, et al. High dose-per-pulse electron beam dosimetry - a model to correct for the ion recombination in the Advanced Markus ionization chamber. Med Phys (2017) 44(3):1157–67. doi:10.1002/mp.12111
9. Marinelli M, di Martino F, Del Sarto D, Pensavalle JH, Felici G, Giunti L, et al. A diamond detector based dosimetric system for instantaneous dose rate measurements in FLASH electron beams. Phys Med Biol (2023) 68(17):175011. doi:10.1088/1361-6560/acead0
10. Di Martino F, Del Sarto D, Giuseppina Bisogni M, Capaccioli S, Galante F, Gasperini A, et al. A new solution for UHDP and UHDR (Flash) measurements: theory and conceptual design of ALLS chamber. Physica Med (2022) 102:9–18. doi:10.1016/j.ejmp.2022.08.010
11. Gómez F, Gonzalez-Castaño DM, Fernández NG, Pardo-Montero J, Schüller A, Gasparini A, et al. Development of an ultra-thin parallel plate ionization chamber for dosimetry in FLASH radiotherapy. Med Phys (2022) 49(7):4705–14. doi:10.1002/mp.15668
12. Romano F, Milluzzo G, Di Martino F, D’Oca MC, Felici G, Galante F, et al. First characterization of novel silicon carbide detectors with ultra-high dose rate electron beams for FLASH radiotherapy. Appl Sci (2023) 13(5):2986. doi:10.3390/app13052986
13. Medina E, Ferro A, Abujami M, Camperi A, Centis Vignali M, Data E, et al. First experimental validation of silicon-based sensors for monitoring ultra-high dose rate electron beams. Front Phys (2024) 12:1258832. doi:10.3389/fphy.2024.1258832
14. Pensavalle JH, Romano F, Celentano M, Sarto DD, Felici G, Franciosini G, et al. Realization and dosimetric characterization of a mini-beam/flash electron beam. Front Phys (2023) 16(11):1269495. doi:10.3389/fphy.2023.1269495
15. Karsch L, Beyreuther E, Burris-Mog T, Kraft S, Richter C, Zeil K, et al. Dose rate dependence for different dosimeters and detectors: TLD, OSL, EBT films, and diamond detectors. Med Phys (2012) 39(5):2447–55. doi:10.1118/1.3700400
16. Guan F, Wang X, Yang M, Draeger E, Han D, Iga K, et al. Dosimetric response of Gafchromic™ EBT-XD film to therapeutic protons. Precision Radiat Oncol (2023) 7(1):15–26. doi:10.1002/pro6.1187
17. Jaccard M, Petersson K, Buchillier T, Germond J, Durán MT, Vozenin M, et al. High dose-per-pulse electron beam dosimetry: usability and dose-rate independence of EBT3 Gafchromic films. Med Phys (2017) 44(2):725–35. doi:10.1002/mp.12066
18. Togno M, Nesteruk K, Schäfer R, Psoroulas S, Meer D, Grossmann M, et al. Ultra-high dose rate dosimetry for pre-clinical experiments with mm-small proton fields. Physica Med (2022) 104:101–11. doi:10.1016/j.ejmp.2022.10.019
19. Villoing D, Koumeir C, Bongrand A, Guertin A, Haddad F, Métivier V. Technical note: proton beam dosimetry at ultra-high dose rates (FLASH): evaluation of GAFchromicTM (EBT3, EBT-XD) and OrthoChromic (OC-1) film performances. Med Phys (2022) 49:2732–45. doi:10.1002/mp.15526
20. Oh K, Gallagher KJ, Hyun M, Schott D, Wisnoskie S, Lei Y, et al. Initial experience with an electron FLASH research extension (FLEX) for the Clinac system. J Appl Clin Med Phys (2024) 25(2):14159. doi:10.1002/acm2.14159
21. Felici G, Barca P, Barone S, Bortoli E, Borgheresi R, De Stefano S, et al. Transforming an IORT linac into a FLASH research machine: procedure and dosimetric characterization. Front Phys (2020) 8:569691. doi:10.3389/fphy.2020.00374
22. Byrne KE, Poirier Y, Xu J, Gerry A, Foley MJ, Lauren Jackson I, et al. Technical note: a small animal irradiation platform for investigating the dependence of the FLASH effect on electron beam parameters. Med Phys (2024) 51:1421–32. doi:10.1002/mp.16909
23. Patriarca A, Fouillade C, Auger M, Martin F, Pouzoulet F, Nauraye C, et al. Experimental set-up for FLASH proton irradiation of small animals using a clinical system. Int J Radiat Oncol Biol Phys (2018) 102:619–26. doi:10.1016/j.ijrobp.2018.06.403
24. Schüler E, Trovati S, King G, Lartey F, Rafat M, Villegas M, et al. Experimental platform for ultra-high dose rate FLASH irradiation of small animals using a clinical linear accelerator. Int J Radiat Oncol Biol Phys (2017) 97:195–203. doi:10.1016/j.ijrobp.2016.09.018
25. Imran I, Younus M, Walayat K, Kakar MU, Ma J. Automated multi-class classification of skin lesions through deep convolutional neural network with dermoscopic images. Comput Med Imaging graphics (2021) 88:101843. doi:10.1016/j.compmedimag.2020.101843
26. Jorge PG, Jaccard M, Petersson K, Gondré M, Durán MT, Desorgher L, et al. Dosimetric and preparation procedures for irradiating biological models with pulsed electron beam at ultra-high dose-rate. Radiother Oncol (2019) 139:34–9. doi:10.1016/j.radonc.2019.05.004
27. Marinelli M, Felici G, Galante F, Gasparini A, Giuliano L, Heinrich S, et al. Design, realization, and characterization of a novel diamond detector prototype for FLASH radiotherapy dosimetry. Med Phys (2022) 49:1902–10. doi:10.1002/mp.15473
28. Verona RG, Felici G, Galante F, Gasparini A, Kranzer R, Mariani G, et al. Application of a novel diamond detector for commissioning of FLASH radiotherapy electron beams. Med Phys (2022) 49:5513–22. doi:10.1002/mp.15782
29. Kranzer R, Schüller A, Bourgouin A, Hackel T, Poppinga D, Lapp M, et al. Response of diamond detectors in ultra-high dose-per-pulse electron beams for dosimetry at FLASH radiotherapy. Phys Med & Biol (2022) 67(7):075002. doi:10.1088/1361-6560/ac594e
30. Niroomand-Rad A, Chiu-Tsao ST, Grams MP, Lewis DF, Soares CG, Van Battum LJ, et al. Report of AAPM Task Group 235 radiochromic film dosimetry: an update to TG-55. Med Phys (2020) 47:5986–6025. doi:10.1002/mp.14497
31. Sorriaux J, Kacperek A, Rossomme S, Lee JA, Bertrand D, Vynckier S, et al. Evaluation of Gafchromic® EBT3 films characteristics in therapy photon, electron and proton beams. Phys Med (2013) 29:599–606. doi:10.1016/j.ejmp.2012.10.001
32. Akdeniz Y. Comparative analysis of dosimetric uncertainty using GafchromicTM EBT4 and EBT3 films in radiochromic film dosimetry. Radiat Phys Chem (2024) 20:111723. doi:10.1016/j.radphyschem.2024.111723
Keywords: FLASH radiotherapy, dosimetry, gafchromic films, dose-rate dependence, UHDR regime
Citation: Del Sarto D, Masturzo L, Cavalieri A, Celentano M, Fuentes T, Gadducci G, Giannini N, Gonnelli A, Milluzzo G, Paiar F, Pensavalle JH, Romano F and Di Martino F (2025) A systematic investigation on the response of EBT-XD gafchromic films to varying dose-per-pulse, average dose-rate and instantaneous dose-rate in electron flash beams. Front. Phys. 13:1474416. doi: 10.3389/fphy.2025.1474416
Received: 01 August 2024; Accepted: 18 April 2025;
Published: 29 April 2025.
Edited by:
Paola Ballesteros Zebadua, Manuel Velasco Suárez National Institute of Neurology and Neurosurgery, MexicoReviewed by:
Imran Iqbal, Yale University, United StatesVeljko Grilj, Centre Hospitalier Universitaire Vaudois (CHUV), Switzerland
Copyright © 2025 Del Sarto, Masturzo, Cavalieri, Celentano, Fuentes, Gadducci, Giannini, Gonnelli, Milluzzo, Paiar, Pensavalle, Romano and Di Martino. This is an open-access article distributed under the terms of the Creative Commons Attribution License (CC BY). The use, distribution or reproduction in other forums is permitted, provided the original author(s) and the copyright owner(s) are credited and that the original publication in this journal is cited, in accordance with accepted academic practice. No use, distribution or reproduction is permitted which does not comply with these terms.
*Correspondence: L. Masturzo, bHVpZ2kubm90ZS5tYXN0dXJ6b0BnbWFpbC5jb20=