- 1PowerChina Huadong Engineering Corporation, Hangzhou, China
- 2College of Civil Engineering and Architecture, Xinjiang University, Urumqi, China
The columnar basalt is a widely distributed in Southwestern China with different types. The first type of columnar jointed basalt is distributed below the elevation of 665 m on the left bank and 590 m on the right bank of the arch dam foundation. Micro-fissures are prevalent within the columns, with gently dipping structural surfaces and an intermittent mosaic structure despite poor overall integrity. The structure is easy to relax after unloading, and the acoustic wave of rock mass is obviously reduced. The control of relaxation deformation of columnar joint basalt is a key problem of hydropower exploration. Based on the structural of columnar jointed basalt, this paper analyzed the relaxation characteristics of columnar jointed basalt.
1 Introduction
In recent years, with the rise of the concept of green ecological development in the world, the development and utilization of clean energy has become one of the indispensable means in the world. Hydropower is a globally recognized clean, high-quality and flexible renewable energy power. All countries in the world give priority to hydropower development, and the western developed countries basically completed their hydropower resource development tasks in the 1980 s. Large and medium-sized hydropower projects also have comprehensive utilization functions such as flood control, disaster reduction, water supply, shipping, irrigation, tourism, and breeding, and have become the most important infrastructure for economic and social development in various countries [1–4]. Hydropower development is not only the need to promote the development of new energy, optimize the energy structure, and reduce carbon emissions, but also the need to realize the comprehensive utilization of water resources, disaster prevention and mitigation, and ecological protection. Therefore, the Chinese government attaches great importance to hydropower development and continues to incorporate hydropower development tasks into the national development plan [5, 6].
In hydropower construction, dams are the most common hydropower infrastructure [7]. China’s hydropower station construction is world-renowned [8–14]. The main facility of a hydroelectric power station is the construction of the dam, which is usually located in an unpopulated and difficult terrain. Therefore, dam foundation excavation is an important construction link in water conservancy projects and is the focus of quality control in water conservancy construction. In this paper, the relaxation characteristics of columnar jointed basalt after excavation of the foundation are investigated. At the same time, the treatment measures for the relaxation of columnar jointed basalt are explored.
The Baihetan Hydropower Station’s 289m-high arch dam features a massive structure and complex dam foundation geology. Columnar jointed basalt, characterized by 2–3 m long columns with diameters of 13–25 cm and micro-cracks forming 5 cm-sized fragments, is distributed below 665 m on the left bank and 590 m on the right. This rock mass relaxes easily after excavation, making the study of its relaxation characteristics and treatment measures crucial for the dam foundation’s stability [15].
2 Method
2.1 Structural classification and distribution of columnar jointed basalt
The basalts in the area exhibit diverse lithologies, strong heterogeneity, well-developed structural planes, and significant unloading characteristics. Columnar jointed basalts are irregular, with varying column sizes and lengths, primarily pentagonal or quadrilateral cross-sections, and lack the regular finger structure typically formed by double diffusion and convection. Instead, they result from magma cooling and contraction [16]. Based on geological conditions and dam foundation evaluation requirements, columnar joints are classified into three categories.
(1) The density of columnar joints is relatively high, and most of them are not cut into a complete column. The length of the column is generally 2∼3 m, and the diameter is 13–25 cm. It is about 5 cm.
(2) The columnar joints develop irregularly and are not cut into a complete column. The length of the column is generally between 0.5 and 2.0 m, and the diameter is 25–50 cm. The micro-cracks in it are relatively developed, but they bite each other and are not completely cut. The block size is 10 cm about.
(3) The columnar joints develop irregularly and are not cut into a complete cylinder. The cylinder is thick, with a length ranging from 1.5 to 5 m and a diameter of 0.5–2.5 m. The cutting is incomplete and the chimera is tight.
Among them, the first type of columnar jointed basalt has the characteristics of high wave velocity, high integrity coefficient, small block size, well-developed cracks, small crack spacing, and tightly embedded rock blocks. It is neither a sub-blocky structure nor a fragmented structure. and mosaic structures, but have a unique structure outside the specification standard. Therefore, it is classified into the category of mosaic structure and named as the subcategory of “columnar mosaic structure”. The joint distribution of the first type of columnar joint basalts is shown in Figure 1. P2β33, P2β41 and P2β61 layers of columnar jointed basalts are mainly distributed in the dam foundation, and the specific distribution. The P2β33 sublayer is distributed in the riverbed and the low-elevation dam foundation on both banks, and the columnar joints are the most developed, which is the focus of the dam foundation rock mass research.
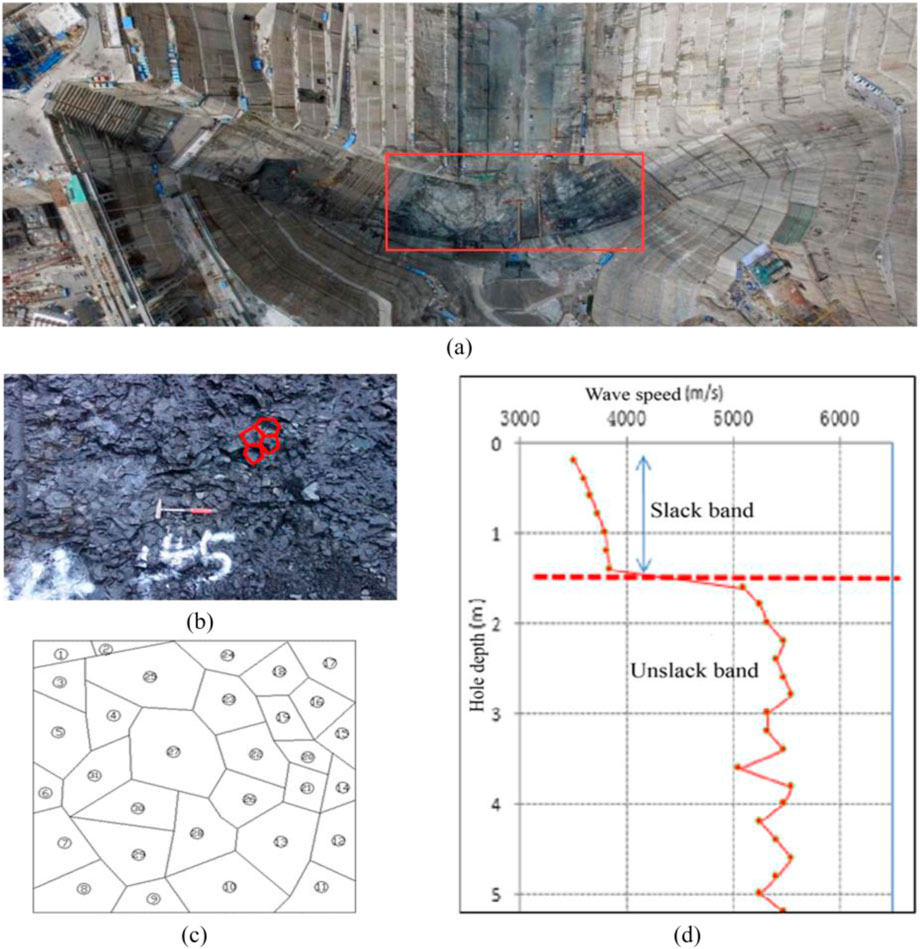
Figure 1. (A) Baihetan hydropower station infrastructure (B) The image of the first type of columnar joint basalt; (C) The geological sketch of the columnar joint basalt; (D) Typical graph of wave speed distribution in relaxed rock mass.
2.2 In-situ experiment test
Two main in situ test, namely, rigid bearing plate method and acoustic borehole testing method are used in this study as shown in Figure 2. Rigid bearing plate test is used to get the deformation modulus, while acoustic borehole test is employed to get the reduction of seismic velocity.
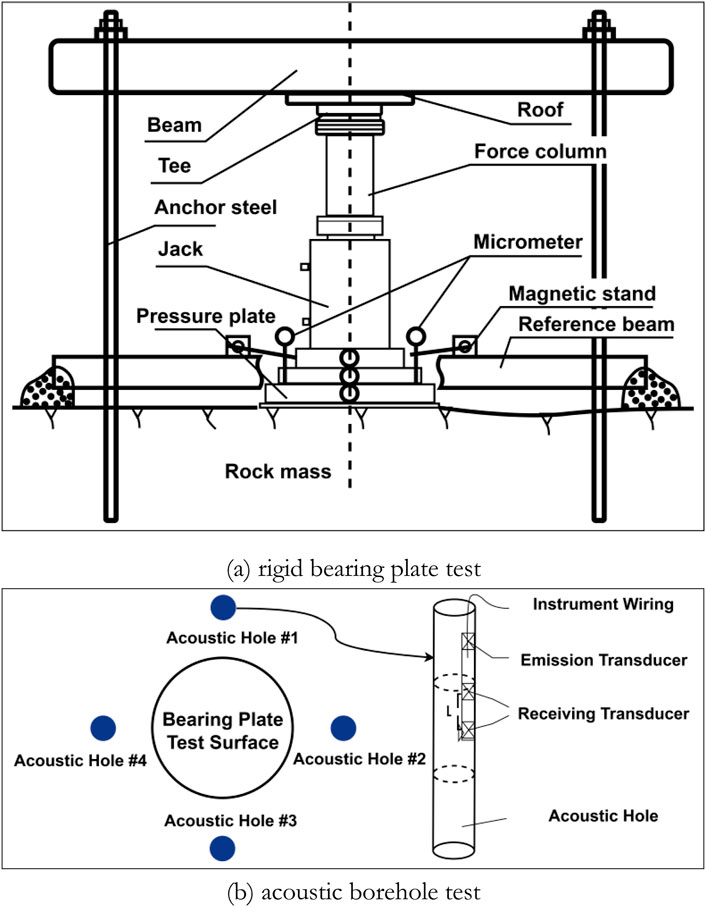
Figure 2. Experiment test used in this study. (A) Rigid bearing plate test. (B) acoustic borehole test.
2.2.1 Rigid bearing plate method
2.2.1.1 Experiment procedure
In the rigid pressure plate test, a Φ504.6 mm, 60 mm thick pressure plate with a surface area of 2000 cm2 is used. A 3200 kN jack and a 60 MPa high-pressure rubber hose connect to a manual or electric oil pump for loading. Four mechanical micrometers are symmetrically fixed to measuring rods embedded in concrete as reference points. Pressurization follows a step-by-step approach with five pressure levels: 2.0 MPa, 4.0 MPa, 6.0 MPa, 8.0 MPa, and 10.0 MPa, applied in single steps, with a maximum pressure of 10.0 MPa. The test adheres to geological standards and specifications.
To measure rock deformation, four measurement points are symmetrically arranged around the pressure plate, each equipped with a micrometer. Loading begins only after ensuring instrument stability, confirmed by consistent readings across three consecutive checks. Upon loading, an initial reading is taken immediately, followed by readings every 10 min. The criterion for deformation stability is that the ratio of the difference between two consecutive readings of the 4 μm to the difference between the initial reading at the current pressure level and the final reading at the previous level must remain within 5% per hour. After the loading process, pressure is gradually released in steps. When the pressure reaches zero, an immediate reading is recorded, followed by additional readings every 10 min until the experiment concludes.
2.2.1.2 Deformation modulus computation
The total deformation and elastic deformation of the rock mass are determined by averaging the measurements from the 4 μm. The deformation modulus and elastic modulus of the rock mass are subsequently calculated using the following formulas:
Where, the parameters include the Poisson’s ratio of the rock mass (μ), unit pressure(P), diameter of the pressure plate(D), and the measured deformation of the rock mass(W). When total deformation values are substituted into the formulas, the resulting modulus(E) represents the deformation modulus (E0). Conversely, when elastic deformation values are substituted, the calculated modulus represents the elastic modulus.
2.2.2 Acoustic borehole testing method
2.2.2.1 Basic theory
A Yanhai RS-ST01C non-metallic acoustic detector was used for single-hole acoustic wave tests with a single-transmit, dual-receive configuration. The probe transmits acoustic waves, received by two transducers, and the propagation velocity is calculated from the time difference and known transducer spacing. This velocity provides insights into rock mass properties, failure surfaces, and structural integrity. Measurements are taken every 20 cm from top to bottom, with the test conducted before the rock deformation test.
To determine the acoustic velocity of the rock mass along the borehole wall, the time difference of the acoustic waves traveling from various measurement points to the two receiving transducers is extracted from the original acoustic wave record. The acoustic velocity of the rock mass is then calculated by dividing the distance between the transducers by this time difference, as expressed in the following formula:
Where, t1, t2 represent the first arrival times of the same excitation signal at each of the two receiving transducers, respectively. The time difference between these arrivals, denoted as Δt is measured in microseconds (μs). The spacing between the two receiving transducers, L is fixed at 0.2 m (m). The speed of sound in the rock mass, represented as vp, is then calculated in meters per second (m/s) using this time difference and the known distance between transducers.
2.2.2.2 Evaluation of the relaxation effect
Assuming the original wave velocity of the rock mass (v0) is known, the wave velocity (vw) is then measured again after the surface of the rock mass is excavated, resulting in a reduced wave velocity. To quantify the change between these two wave velocity values, a wave velocity change coefficient (η)can be defined.
According to the Technical Specifications for Construction of Rock Foundation Excavation Engineering of Hydraulic Structures, it is stipulated that when the wave velocity change coefficient (η) of the excavated rock mass is less than or equal to 10%, excavation is considered to have no impact on rock mass quality. Conversely, when η exceeds 15%, the rock mass quality is regarded as being significantly affected by the excavation. If η falls between these two values, excavation is considered to have only a minor impact on the quality of the rock mass.
3 Results
3.1 Physical and mechanical parameters of columnar jointed basalt
There is anisotropy in the columnar joint basalt structure, and there is a certain difference in the deformation modulus perpendicular to the direction parallel to the columnar joints [17–19]. In order to study the deformation modulus of columnar joint basalt in different directions, Baihetan Project conducted a series of on-site in situ tests, which can better reflect the natural characteristics of the rock mass. The in situ test mainly adopts the rigid bearing plate test, and the load direction is divided into horizontal direction and vertical direction [20, 21].
In the in situ pressure plate test at Baihetan, the deformation modulus of III1 rock mass ranges from 9–11 GPa horizontally and 7–9 GPa vertically, while for III2 rock mass, it ranges from 7–9 GPa horizontally and 5–7 GPa vertically. Vertical deformation modulus is significantly lower than horizontal, with vertical-to-horizontal ratios of 0.8 for III1 and 0.75 for III2, indicating pronounced anisotropy.
3.2 Structural and mechanical properties of columnar basalt after relaxation
There must be relaxation after excavation of columnar jointed basalt. The relaxed rock mass needs to be used as the foundation of high arch dams. It is necessary to study the characteristics of columnar jointed basalt after relaxation.
The Maxwell model can be used to describe the properties of rocks that exhibit instantaneous deformation, steady creep, and relaxation. Next, the relaxation phenomenon of rocks is explained based on the physical concept of the model. The Maxwell model is a viscoelastic body composed of a spring and a damper connected in series.
(1) Constitutive equation
Due to:
Therefore:
(2) Creep equation
Under a constant load σ (σ = σ0), since dσ/dt = 0, the constitutive equation simplifies to:
Solving this differential equation gives:
Where C is the integration constant. Using the initial conditions to solve for C, when t = 0,
From the equation, it can be seen that the model exhibits instantaneous strain and that the strain gradually increases with time. This model represents steady-state creep.
(3) Relaxation equation
To maintain a constant strain ε,
Solving this equation yields:
Where C is the integration constant. Using the initial condition, at t = 0, σ = σ0 (σ0 is the instantaneous stress), we find C = -lnσ0. Substituting C into the equation gives:
Thus,
From this equation, it can be observed that as t increases, σ gradually decreases. In other words, when the strain is constant, the stress decreases over time, a mechanical phenomenon known as relaxation.
From the physical concept of the model, relaxation can be understood as follows: at t = 0, the viscous element cannot deform instantaneously, so only the elastic element undergoes deformation. However, as time progresses, the viscous element gradually deforms under the action of the spring. As the damper elongates, the spring gradually contracts, leading to a reduction in stress within the spring. This is the essence of relaxation.
Long-term observations were conducted on columnar joint basalt to analyze its structural and mechanical properties after relaxation. Following the excavation of a simulated test hole in March 2011, geological points were observed again in June 2015, with comparison photos recorded. Observations from March to July 2015 at the dam foundation revealed no significant changes in rock mass structure, though surface rock blocks became more prone to detachment. After 4 years and 3 months, the relaxed layer at the dam base site stabilized, with slight surface weathering and discoloration but no significant opening of columnar joints or microfractures, maintaining a columnar mosaic structure.
To assess the mechanical properties of the relaxed layer of columnar joint basalt at the Baihetan dam foundation (650–660 m elevation), 11 rigid bearing plate tests were conducted. Results show the deformation modulus of the relaxed layer is 4–6 GPa, slightly lower than the 5–7 GPa of the unrelaxed rock mass. While the deformation modulus has deteriorated, the rock mass structure remains intact, and the mechanical properties of the relaxed and unrelaxed rock masses are largely comparable.
3.3 Determination of relaxation property in columnar jointed basalt
Due to the dense joints of columnar joint basalt, the relaxation effect is more obvious after excavation and unloading, the columnar joints of the surface rock mass open, the transverse micro-cracks gradually appear, and the sound wave velocity of the rock mass decreases, especially in the surface layer where the ground stress is high.
As shown in Table 1, for the First Type of Columnar Jointed Basalt, the proportion of rocks with a wave velocity variation coefficient η>15% is 43.3%, 10%<η ≤ 15% is 13.3%, and η<10% is 43.3%. Therefore, excavation has a relatively large impact on the quality of the First Type of Columnar Jointed Basalt. The cryptocrystalline basalt has all wave velocity variation coefficients η below 15%, indicating that excavation has only a slight impact on the quality of cryptocrystalline basalt. For breccia lava, the proportion of rocks with a wave velocity variation coefficient η ≤ 10% is 33.3%, 10%<η ≤ 15% is 11.1%, and η>15% is 55.6%, Thus, excavation has a greater impact on the quality of breccia lava, with more than half of the rocks affected by excavation.
4 Discussion
The first type of columnar jointed basalt, characterized by dense joints and developed transverse micro-cracks, easily relaxes after excavation and unloading. Thus, its relaxation characteristics are a key focus in the foundation treatment of the Baihetan Dam. Extensive acoustic detection tests have been conducted in exploration tunnels, simulation test tunnels, diversion tunnels, and dam foundation test areas to systematically study these characteristics.
Comparing the factors affecting the relaxation depth of columnar joint basalt such as size effect, time effect and in situ stress, the specific effects are as follows.
4.1 Size effect
Comparing the excavation size and the average relaxation layer thickness, the thickness of the columnar joint basalt relaxation layer basically increases with the increase of the excavation size. When the excavation size is larger, the influence on the relaxation layer thickness decreases [22].Unloading relaxation exhibits a certain size effect, specifically manifested as follows: for smaller sizes, the relaxation depth increases with the size, while for larger sizes, the relaxation depth no longer increases. Fault zones have a certain impact on rock mass relaxation, particularly at the leading edge of the fault zone, where localized shear deformation occurs.
4.2 Time effect
Statistics show that the stable time of the relaxed layer is roughly between 25 days and 46 days, and the stable time tends to prolong with the expansion of the excavation surface. The change process of the rock mass excavation relaxation depth measured by the acoustic inspection hole in the dam foundation excavation test area is shown in Figure 3 below. The relaxation depth gradually increases with time, and the relaxation depth reaches a stability about 40 days after excavation, with less variation later on [23].
4.3 In-situ stress effect
Comparing the test results of the observation section of 3# and 4# diversion tunnels, the relaxation depth of 4# diversion tunnel with higher in situ stress is 5.2–7.7 m, which is significantly higher than that of 3# diversion tunnel at 2.5–3.0 m. The higher the in situ stress, the greater the unloading rebound deformation, and the greater the stress relaxation [24].
5 Conclusion and prospect
The relaxation and deformation control of columnar jointed basalt foundations is crucial for super-high hydropower projects. This study investigates the structural and relaxation characteristics of columnar jointed basalt through various tests and proposes comprehensive control measures, including thick-layer protection, grouting consolidation, deep anchorage, and precise blasting. These measures effectively limit the relaxation depth to levels comparable to conventional rock masses, meeting the requirements for super-high hydropower stations.
This paper combines extensive monitoring data with experimental research to explore the deformation and strength characteristics of unloaded columnar jointed basalt, deepening the understanding of its mechanical properties. However, further research is needed to address shortcomings, including detailed studies of intact columnar jointed basalt alongside the relaxation layer. Integrating findings from other research units at the dam site will provide a more comprehensive understanding of columnar jointed basalt.
Data availability statement
The original contributions presented in the study are included in the article/supplementary material, further inquiries can be directed to the corresponding authors.
Author contributions
JG: Conceptualization, Software, Writing–original draft, Writing–review and editing. JW: Formal Analysis, Writing–original draft, Writing–review and editing. MH: Methodology, Writing–original draft, Writing–review and editing. SY: Visualization, Writing–original draft, Writing–review and editing. SL: Data curation, Supervision, Writing–original draft, Writing–review and editing.
Funding
The author(s) declare that financial support was received for the research, authorship, and/or publication of this article. This study is supported by the Fund for Less Developed Regions of the National Natural Science Foundation of China (Grant No. 52068066), the Fund for Less Developed Regions of the National Natural Science Foundation of China (Grant No. 52368052), and the National Science Fund for Distinguished Young Scholars of Xinjiang Uygur Autonomous Region (Grant No. 2022D01E32).
Conflict of interest
Authors JG, JW, and MH were employed by PowerChina Huadong Engineering Corporation.
The remaining authors declare that the research was conducted in the absence of any commercial or financial relationships that could be construed as a potential conflict of interest.
Generative AI statement
The author(s) declare that no Generative AI was used in the creation of this manuscript.
Publisher’s note
All claims expressed in this article are solely those of the authors and do not necessarily represent those of their affiliated organizations, or those of the publisher, the editors and the reviewers. Any product that may be evaluated in this article, or claim that may be made by its manufacturer, is not guaranteed or endorsed by the publisher.
References
1. Li X-z., Chen Z-j., Fan X-c., Cheng Z. Hydropower development situation and prospects in China. Renew Sustainable Energy Rev (2018) 82:232–9. doi:10.1016/j.rser.2017.08.090
2. Bartle A. Hydropower potential and development activities. Energy policy (2002) 30(14):1231–9. doi:10.1016/s0301-4215(02)00084-8
3. Moran EF, Lopez MC, Moore N, Müller N, Hyndman DW. Sustainable hydropower in the 21st century. Proc Natl Acad Sci (2018) 115(47):11891–8. doi:10.1073/pnas.1809426115
4. Egré D, Milewski JC. The diversity of hydropower projects. Energy policy (2002) 30(14):1225–30. doi:10.1016/s0301-4215(02)00083-6
5. Chang X, Liu X, Zhou W. Hydropower in China at present and its further development. Energy (2010) 35(11):4400–6. doi:10.1016/j.energy.2009.06.051
6. Sun X, Wang X, Liu L, Fu R. Development and present situation of hydropower in China. Water Policy (2019) 21(3):565–81. doi:10.2166/wp.2019.206
7. Zarfl C, Lumsdon AE, Berlekamp J, Tydecks L, Tockner K. A global boom in hydropower dam construction. Aquat Sci (2015) 77:161–70. doi:10.1007/s00027-014-0377-0
8. Tang H, Wasowski J, Juang CH. Geohazards in the three gorges reservoir area, China–lessons learned from decades of research. Eng Geology (2019) 261:105267. doi:10.1016/j.enggeo.2019.105267
9. Sun G, Yang Y, Jiang W, Zheng H. Effects of an increase in reservoir drawdown rate on bank slope stability: a case study at the Three Gorges Reservoir, China. Eng Geology (2017) 221:61–9. doi:10.1016/j.enggeo.2017.02.018
10. Niu C, Wang Q, Chen J, Zhang W, Xu L, Wang K. Hazard assessment of debris flows in the reservoir region of wudongde hydropower station in China. Sustainability (2015) 7(11):15099–118. doi:10.3390/su71115099
11. Li B, Xu N, Dai F, Gu G, Ke W. Microseismic monitoring and stability analysis for the large-scale underground caverns at the Wudongde hydropower station. Bull Eng Geology Environ (2020) 79:3559–73. doi:10.1007/s10064-020-01777-z
12. Zhang Z, Xu W, Wang W, Wang R. Triaxial creep tests of rock from the compressive zone of dam foundation in Xiangjiaba Hydropower Station. Int J Rock Mech Mining Sci (2012) 50:133–9. doi:10.1016/j.ijrmms.2012.01.003
13. Zhang S-l., Zhu Z-h., Qi S-c., Hu Y, Du Q, Zhou J. Deformation process and mechanism analyses for a planar sliding in the Mayanpo massive bedding rock slope at the Xiangjiaba Hydropower Station. Landslides (2018) 15:2061–73. doi:10.1007/s10346-018-1041-x
14. Zhao Y, Wang X, Zhang X, Jia Z, Zeng X, Zhang H. Rock borehole shear tests in dam foundation of Xiangjiaba hydropower station. J Rock Mech Geotechnical Eng (2012) 4(4):360–6. doi:10.3724/sp.j.1235.2012.00360
15. Shi A, Tang M, Zhou Q. Research of deformation characteristics of columnar jointed basalt at Baihetan hydropower station on Jinsha river. Chin J Rock Mech Eng (2008) 27(10):2079–86.
16. Ni S, He S, Chen Y. The failure modes, failure mechanisms and countermeasures of columnar jointed basalt rock mass. Chin J Rock Mech Eng (2016) 35(S1):3064–75. doi:10.13722/j.cnki.jrme.2015.0006
17. Di SJ, Zheng WT, Zhang JK. Back analysis on mechanical deformation parameters of columnar jointed rock mass [C]. Proc Appl Mech Mater (2011). doi:10.4028/www.scientific.net/AMM.55-57.1947
18. Kelsall PC, Case JB, Chabannes CR. Evaluation of excavation-induced changes in rock permeability. Int J Rock Mech Mining Sci and Geomechanics Abstr (1984) 21(3):123–35. doi:10.1016/0148-9062(84)91530-4
19. Pusch R, Stanfors R. The zone of disturbance around blasted tunnels at depth. Int J Rock Mech Mining Sci (1992) 29(5):447–56. doi:10.1016/0148-9062(92)92629-q
20. Fan Q, Wang Z, Xu J, Zhou M, Jiang Q, Li G. Study on deformation and control measures of columnar jointed basalt for baihetan super-high arch dam foundation. Rock Mech And Rock Eng (2018) 51(8):2569–95. doi:10.1007/s00603-017-1378-9
21. Carlson SR, Young RP. Acoustic emission and ultrasonic velocity study of excavation-induced micro-crack damage at the Underground Research Laboratory. Int J Rock Mech Mining Sci Geomechanics Abstr (1993) 30(7):901–7. doi:10.1016/0148-9062(93)90042-c
22. Wentang Z, Weiya X, Yu N. Scale effect and anisotropy of deformation modulus of closely jointed basaltic mass. J Eng Geology (2010) 18(4):559–65.
23. Pei S, Feng X, Zhang J. Time-dependent relaxation characteristics of columnar jointed basalts in high-slope dam foundation during excavation. Rock Soil Mech (2018) 39(10):3743–54. doi:10.16285/j.rsm.2018.0153
Keywords: hydropower exploration, columnar joint basalt, intermittent mosaic structure, relaxation characteristics, treatment measures
Citation: Gu J, Wang J, He M, Yang S and Li S (2025) Research on relaxation characteristics of columnar jointed basalts of deep foundation in hydropower station. Front. Phys. 13:1522240. doi: 10.3389/fphy.2025.1522240
Received: 04 November 2024; Accepted: 06 February 2025;
Published: 26 February 2025.
Edited by:
Sanjay Shrawan Nimbalkar, University of Technology Sydney, AustraliaReviewed by:
Zeeshan Asghar, Prince Sultan University, Saudi ArabiaGan Feng, Sichuan University, China
Yifei Gong, Hohai University, China
Copyright © 2025 Gu, Wang, He, Yang and Li. This is an open-access article distributed under the terms of the Creative Commons Attribution License (CC BY). The use, distribution or reproduction in other forums is permitted, provided the original author(s) and the copyright owner(s) are credited and that the original publication in this journal is cited, in accordance with accepted academic practice. No use, distribution or reproduction is permitted which does not comply with these terms.
*Correspondence: Shu Yang, bWF0cml4d2hvQHN0dS54anUuZWR1LmNu; Shuaikang Li, MTc3OTk3MDMyNjNAMTYzLmNvbQ==