- 1Tianjin Key Laboratory of Electronic Materials and Devices, School of Electronics and Information Engineering, Hebei University of Technology, Tianjin, China
- 2Institute of Polymer Materials, Beijing Institute of Machine and Equipment, Beijing, China
Gas sensors were fabricated from Cr2WO6 nanoparticles for NO2 detection. Low dimensional materials Cr2WO6 were prepared by a wet chemistry method followed by hydrothermal treatment. The morphology of the nanoparticles and their sensing properties to NO2 were investigated in both dry and humid conditions. Additionally, the sensing response was also characterized in a non-oxygen condition. It was concluded that the sensor responses in N2 conditions were higher than that in air conditions at 200°C. Moreover, the sensing characteristics were inhibited by water vapor at 200°C. The oxygen adsorption behavior was also investigated to verify the basic sensing mechanism of Cr2WO6 in the absence and presence of NO2 and water vapor separately. Based on the power law response, it was indicated that both NO2 and water vapor have a strong adsorption ability than oxygen ions of Cr2WO6 sensors.
Introduction
National nitrogen oxide (NOx) emissions were 20.6 million metric tons (Mt) in 2015, with annual growth rates of 5.9% since 1949 in China (Richter et al., 2005; Sun et al., 2018). Among them, owing to its toxic effects to animals and plants, NO2 is irritant and corrosive even at ppm level with serious harm to the respiratory tract and causticity particularly in children and elderly (Ling and Leach, 2004; Kida et al., 2009). Thus, determination of NOx emissions is vital to regional and global ozone air pollution, acid deposition, and climate change (Jaegle et al., 2005). There have been great demands for cheap, reliable, and effective methods of real-time monitoring of NO2 level in the environment (Stǎnoiu et al., 2012). To date, gas sensors based on metal oxide semiconductors (MOS) have been extensively investigated and successfully commercialized for NOx detection due to their superior properties, simple structure, and low cost (Afzal et al., 2012). Among them, WO3 based sensors show an excellent property to NO2 in respect of sensitivity (Choi et al., 2004; Hua et al., 2018d). It was reported that WO3 sensors fabricated through a wet process were sensitive to ppb levels of NO2 with a low temperature due to a strong adsorption ability of NO2 onto tungsten atoms compared with the weak adsorption of oxygen (Choi et al., 2004). Recently, gas sensors based on P-type MOS materials have been reported to have a sensitive and selective response to NO2 with a low cross-sensitivity to humidity (Nguyen and El-Safty, 2011; Stǎnoiu et al., 2012). It was reported that the NO2 sensors based on Cr2O3 showed a selectivity relative to CO and low cross-sensitivity to humidity, which was due to the higher surface reactivity toward NO2 than CO through the electrical resistance and work function changes both in dry and humid air (Stǎnoiu et al., 2012). Gas sensors based on NiO nanosheets were fabricated by a hydrothermal method, which showed high sensitivity and selectivity to NO2 (Nguyen and El-Safty, 2011). That may be due to NO2 having higher electron affinity than the pre-adsorbed oxygen (Hoa et al., 2009). In this paper, chromium tungstate (Cr2WO6) was prepared by a wet chemistry method followed by hydrothermal treatment. The sensing properties to NO2 in oxygen and non-oxygen atmosphere were investigated under dry and humid conditions, respectively. It was found that sensors based on P-type Cr2WO6 were very sensitive to NO2 even with a high humid condition. In addition, the sensing mechanism was verified by the oxygen adsorption behavior in different conditions. Eventually, the fundamental sensing mechanism to NO2 was explained.
Experimental
Chromium(III) nitrate nonahydrate (99.95%) and sodium tungstate dehydrate (ACS 99.0–101.0%) were provided by Shanghai Aladdin Biochemical Technology Co., Ltd. Chromium tungstate (Cr2WO6) nanoparticles were synthesized by a hydrothermal assisted process as described in Supplementary Materials A (Zhou et al., 2015). Subsequently, the powders were annealed at 1,000°C in air for 2 h. The samples were characterized using X-ray diffraction (XRD; D8 FOCUS, Bruker, Germany) in Cukα radiation with corresponding wavelengths of 1.54 Å and a filament current and voltage of 15 mA and 40 kV and a field-emission scanning electron microscope (FE-SEM; Nova Nano SEM 450, FEI). The surface morphology of the material was analyzed by transmission electron microscopy (TEM; Tecnai-F20, FEI, USA) with an accelerating voltage of 200 kV. The sample powders were mixed with glycerin to form a homogeneous paste and then was screen-printed on the alumina substrate. In order to obtain good stability, all sensors were aged at 400°C for 24 h. The gas sensing performance was measured by DC resistance with a homemade apparatus equipped with a dynamic gas distribution system as shown schematically in Figure 1. Target gases were supplied by gas cylinders with appropriate concentrations balanced with the carrier gases (air or nitrogen). The humidity and oxygen concentrations were calibrated using a humidity sensor (SHT31-ARP, Sensirion, Switzerland) and oxygen analyzer (SST, England), respectively. Keithley multimeter (Keithley 2000, USA) was used to record all data in real time. The sensor response was defined as S = Ra/Rg, where Ra and Rg were the resistances in the presence of air/N2 and oxidizing gases, respectively.
Results and Discussion
In order to obtain a high purity of Cr2WO6 phase, a high sintering temperature of 1,000°C was used and the crystal structure was characterized by XRD. Figure S3 shows the XRD patterns of prepared Cr2WO6 powders. Obviously, XRD patterns exhibit very narrow peaks indicating a good crystal quality and the patterns could be well-fit with the tetragonal phase (JCPDS 35-0791) indicating a high purity of the prepared Cr2WO6. The morphology of Cr2WO6 powders was characterized by SEM and TEM. Figures 2A,B show SEM images of Cr2WO6 powders, which consisted of huge number of particles in a grain shape with a large size. According to the insert SEM images the grain shape is estimated around 300 nm by counting. TEM images in Figure 2C show that the grain size is consistent with the SEM images. Additionally, HRTEM images in Figure 2D also suggest a good crystalline quality of Cr2WO6 nanoparticles and the lattice spacing of 0.323 nm and 0.248 nm is in good accordance with (110) and (103) planes of tetragonal Cr2WO6 (JCPDS 35-0791) and results of XRD (Zhou et al., 2015).
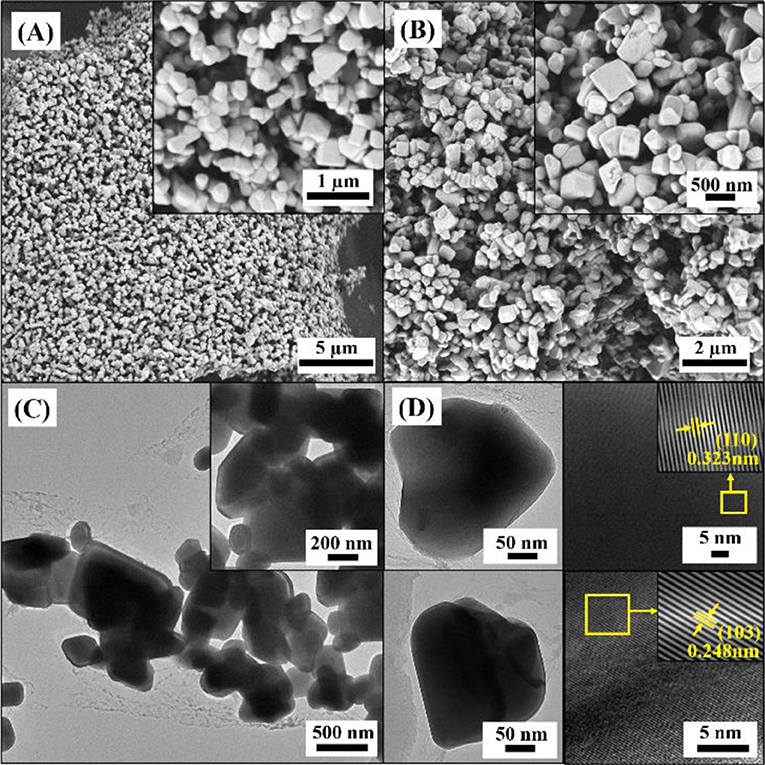
Figure 2. The morphology analysis of powders (A,B) SEM images, (C) TEM images, and (D) HRTEM micrograph with lattice diffraction pattern of nanoparticles.
The sensing properties were characterized with NO2 ranging from 0.2 to 5 ppm balanced with syntheses with an operation temperature of 200–350°C. According to previous reports, chemiresistive type sensors with a low concentration of carriers, i.e., holes in the present case, have a strong transduction ability with high cost of resistance (Bârsan et al., 2010; Hua et al., 2018a). It is worth noting that two identical sensor devices fabricated from the same materials were measured; however, only one sensors' data was presented for simplicity. The other one was used as a reference and not shown. Figure 3A presented the time and temperature dependence of sensor resistance. It was found that sensor resistance significantly increased with reduction in operation temperatures. When the temperature decreased from 250 to 200°C, sensor resistance was almost increased by 3 times. However, when the temperature was lower than 200°C, sensors gave an extremely high resistance reaching to 108 Ω and over the range of measurement (Keithley 2000), which is also very difficult for practical applications. Thus, sensing response was only characterized from 200 to 350°C. One can note that sensor resistance is significantly reduced when exposed to NO2, suggesting a P-type response of Cr2WO6. Moreover, the sensor responses were found to be highly temperature-dependent and increased with reduction in temperatures (Bodneva et al., 2019). When the working temperature increased to 350°C, the responses of 5 ppm NO2 were reduced by 4.5 times.
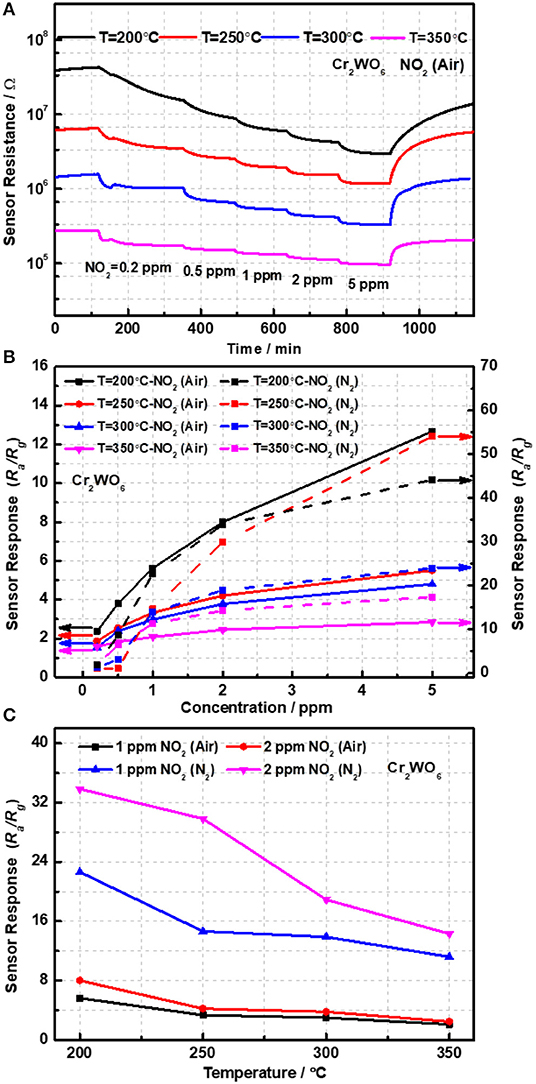
Figure 3. (A) The transient response of sensor devices and (B) the sensor responses as a function of NO2 concentration and (C) temperatures.
Detection of NO2 in the non-oxygen atmosphere was highly required and could be applied to some specific scenes, such as the fuel leaks in aerospace systems. The sensor responses to NO2 balanced with N2 were investigated from 200 to 350°C in the absence of oxygen. Owing to its p-type conduction, the baseline resistance in N2 was largely enhanced caused by the increase in electrons released by adsorbed oxygens on the surface. Consequently, the sensor responses were greatly increased to NO2 higher than 1 ppm and much higher than in air atmosphere as shown in Figure 3B. It was shown that the responses in N2 conditions were 4 times as much as that in air conditions at 200°C as shown in Figure 3C. It was well-known that in the presence of oxygen there could be a competitive adsorption between oxygen and NO2, and therefore, that may give a reason for the clear difference of sensing responses in the presence and absence of oxygen (Hua et al., 2018d). What's more, the competitive relation between NO2 and oxygen will be analyzed by the oxygen adsorption behavior in the following. In addition, it was indicated that the sensing responses in N2 atmosphere were highly dependent on the working temperatures similar to that in the air atmosphere. When the temperature decreased from 350 to 200°C, sensor resistance was almost promoted by 2 orders and sensor responses were enhanced by almost 4 times. As shown in Figure S4, it seems that the sensors gave a relatively poor response to NO2 below 1 ppm, almost no response in a dry condition. In other words, the limit of detection (LOD) of NO2 for Cr2WO6 nanoparticles is better than 1 ppm. This is very similar to a typical n-type MOS gas sensor when exposed to reducing gases such H2 and CO (Hua et al., 2018c). A small concentration of gas could be shielded by adsorption of oxygen due to the release of free electrons by the reaction of adsorbed oxygens with reducing gases. Thus, small concentration of reducing gases could not be detected (Hua et al., 2018d). However, in the present case, it is believed that the shielding effect of NO2 could be caused by a competitive adsorption of oxygen and NO2 on the surface of Cr2WO6. When NO2 adsorbed onto the surface and shared the same sites with oxygen adsorption leading to desorption of oxygen, there was no electron charge transfer. As NO2 molecular trap the electrons, which released from desorption of O2, the surface density will not generate a net increase. As a result, there was no resistive response, which was known as a chemical shielding effect for MOS gas sensors (Hua et al., 2018d). Finally, a comparison of MOS sensors to NO2, including various n-type and p-type materials, was given in Table 1 (Ling and Leach, 2004; Hoa et al., 2009; Nguyen and El-Safty, 2011; Vyas et al., 2013; Marichy et al., 2015). In conclusion, the Cr2WO6 sensors have reat sensitivity and lower operation temperatures to NO2 compared with the n-type materials including SnO2/WO3 and ZnO both in air and N2 atmospheres. Furthermore, some typical p-type materials were listed in Table 1, and the Cr2WO6 sensors displayed high sensitivity, which had great potential to be applied to some specific scenes. The stability of sensors have been also investigated in Figure S7 of Supplementary Material E.
Moreover, the sensing properties to NO2 balanced with air in the presence of humidity were also investigated at 200°C and the transient responses were shown in Figure S5 of Supplementary Materials C. It was clear that all sensor resistances were a direct proportion to the relative humidity at 25°C (RH at 25°C) compared with that in dry conditions as revealed in Figure 4. The phenomenon can be explained by water vapor competing for adsorption sites of NO2 on the surface of Cr2WO6 nanoparticles. When the humidity reached 4% RH at 25°C, sensor resistance was promoted by ~2 times and the sensor responses were enhanced in the humid conditions in Figure 4. The sensor response was increased by almost 2 times to 2 ppm NO2 in the presence of 4% RH at 25°C. In the sensing processes, NO2 acted as the acceptor, meanwhile the water vapor was the donor. Moreover, the water vapor gave an inhibition to the oxygen adsorption on the material surface. Interestingly, the sensor responses were reduced when the humidity increased to 14 and 20% RH at 25°C. That may owe to the chemical adsorption of water vapor, which occupies the adsorption sites of NO2. However, the responses were still higher than that in dry conditions. Moreover, the sensing properties balanced with N2 in humid atmosphere were also analyzed by Keithley multimeter; nevertheless, the water vapor gave a promotion to sensor resistance compared with that in dry conditions. Therefore, we cannot evaluate the performance of the sensor devices based on the existing equipment in the laboratory.
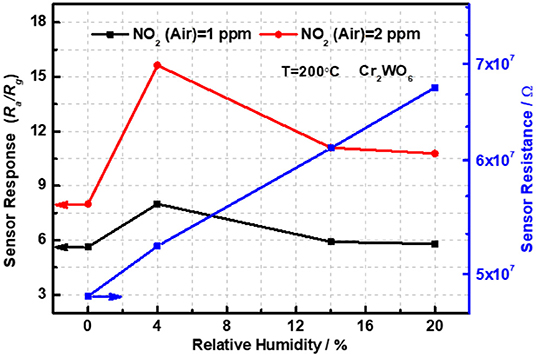
Figure 4. The sensor responses of Cr2WO6 as a function of humidity and NO2 concentration balanced with air or N2 at 200°C.
According to our previous reports, oxygen adsorption, and reaction play a basic role in the sensing process with the formation of , O−, or O2− on the surface of metal oxides (Hua et al., 2018a,b,c). The oxygen adsorption behavior could be analyzed by the relationship between sensor resistance (Rg) and partial pressure of oxygen (PO2) in different atmospheres, i.e., power-law response. Figure 5 shows the linear plot of Rg on the PO2 ranging from 0.1 to 0.7 atm (1 atm = 100% in volume) in a double logarithm-scale at an operation temperature of 200°C. It was obvious that the Rg decreased with increasing PO2, indicating the presence of oxygen adsorption on the surface of Cr2WO6 nanoparticles. Adsorption of oxygen on the surface traps electrons and releases holes resulting in a reduction in sensor resistance. Moreover, Figure 5A presents the power-law response to oxygen, and the fitting slope (n) was −0.15 at 200°C. However, for p-type materials, the receptor and transducer functions have not been built yet. Thus, the fitting power-law exponent could not be well-clarified. However, it was quite clear that oxygen adsorption served as the receptor function for Cr2WO6 without difference with typical n-type WO3 or p-type Cr2O3 (Hua et al., 2018a,b,c). As mentioned before, water vapor forms chemical adsorption on surface leading to a block on oxygen adsorption as shown in Figure 5A. With humidity increasing from 0 to 10% RH at 25°C, Rg was promoted at all PO2 and the absolute value of n was decreased, suggesting a strong electronic interaction of oxygen with the Cr2WO6 surface. This may be caused by the competitive adsorption between oxygen and water molecules (Hua et al., 2018c). With the presence of NO2, the power-law response to oxygen was also investigated to clarify the role of oxygen on the sensing process of NO2 (Hua et al., 2018d). When exposed to NO2 of 1 ppm, the sensor resistance greatly decreased. According to Figure 5B, the power-law response to oxygen is not linear, suggesting that the adsorption of oxygen is influenced by NO2 adsorption. More specifically, the electronic interaction of oxygen with the Cr2WO6 surface was significantly inhibited by NO2, i.e., shielded by NO2. This could be due to a competitive adsorption of NO2, which may share the same adsorption sites, in case of typical MOS, are metal atoms and oxygen vacancies. When exposed to humidity in the presence of NO2 (1 ppm balanced with N2), sensor resistance was almost independent of PO2, and the power-law exponent was merely 0.06, which is much smaller than that in a dry condition. The corresponding transient responses of power law response were shown in Figure S6 of Supplementary Material D. In addition, it was noted that the absolute value of the power-law exponent, n became much smaller compared with that in the absence of NO2. In other words, humidity not only promotes the sensing response but also changes the basic mechanism of p-type Cr2WO6 to NO2 according to the power-law response. It is possible that in the presence of humidity oxygen adsorption may take place with a competitive adsorption with water, which forms a weak adsorption on the surface of Cr2WO6. This causes a weak adsorption.
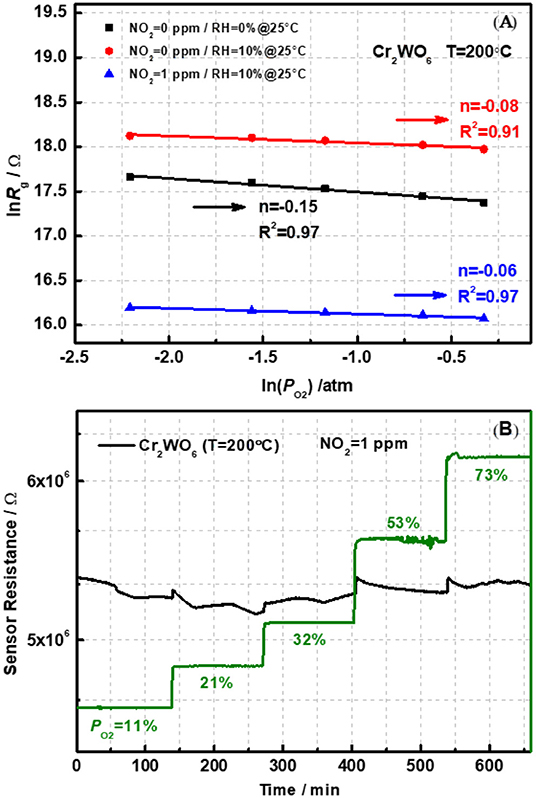
Figure 5. (A) The power-law response to oxygen for pristine Cr2WO6 in the absence and presence of water vapor and (B) a transient response to O2 in the presence of 1 ppm NO2 at 200°C.
Conclusion
A new sensing material Cr2WO6 was prepared and its sensing properties to NO2 were investigated in both dry and humid conditions. The basic sensing mechanism was also analyzed by surface oxygen adsorption behavior in different atmospheres. Here are the conclusions:
➢ Sensors based on Cr2WO6 nanoparticles showed great response to NO2 based on air atmosphere down to 0.2 ppm. The sensor responses in N2 conditions were higher than in air conditions over 1 ppm of NO2.
➢ Sensor resistances were raised up with the relative humidity increasing. However, sensor responses to all gases were enhanced by water vapor over the range of 0–20% RH at 25°C.
➢ The oxygen adsorption played a vital basic role in the sensing process. However, the oxygen adsorption behavior was inhibited by the presence of water vapor and NO2.
In conclusion, sensing material Cr2WO6 shows a great response to detect NO2 in dry and humid conditions.
Data Availability Statement
All datasets generated for this study are included in the article/Supplementary Material.
Author Contributions
MY and CT performed the experiments and analyzed the data with the help from YW, YL, and ZH. YW and ZH conceived and modified the manuscript based on experimental data.
Funding
This study was supported by the National Natural Science Foundation of China (Grant Nos. 51977059 and 51777056). The characterization results were supported by Beijing Zhongkebaice Technology Service Co., Ltd, and we acknowledge critical and quantity of testing work supported by Beijing Zhongkebaice Technology Service Co., Ltd.
Conflict of Interest
YL was employed by the Beijing Institute of Mechanical Equipment that is a government-owned non-profit organization.
The remaining authors declare that the research was conducted in the absence of any commercial or financial relationships that could be construed as a potential conflict of interest.
Supplementary Material
The Supplementary Material for this article can be found online at: https://www.frontiersin.org/article/10.3389/fchem.2019.00907/full#supplementary-material
References
Afzal, A., Cioffi, N., Sabbatini, L., and Torsi, L. (2012). NOx sensors based on semiconducting metal oxide nanostructures: Progress and perspectives. Sens. Actuators B Chem. 171, 25–42. doi: 10.1016/j.snb.2012.05.026
Bârsan, N., Simion, C., Heine, T., Pokhrel, S., and Weimar, U. (2010). Modeling of sensing and transduction for p-type semiconducting metal oxide based gas sensors. J. Electroceram. 25, 11–19. doi: 10.1007/s10832-009-9583-x
Bodneva, V., Ilegbusi, O., Kozhushner, M., Kurmangaleev, K., Posvyanskii, V., and Trakhtenberg, L. (2019). Modeling of sensor properties for reducing gases and charge distribution in nanostructured oxides: a comparison of theory with experimental data. Sens. Actuators B Chem. 287, 218–224. doi: 10.1016/j.snb.2019.02.034
Choi, Y., Sakai, G., Shimanoe, K., and Yamazoe, N. (2004). Wet process-based fabrication of WO3 thin film for NO2 detection. Sens. Actuators B Chem. 101, 107–111. doi: 10.1016/j.snb.2004.02.031
Hoa, N., Quy, N., and Kim, D. (2009). Nanowire structured SnOx-SWNT composites: high performance sensor for NOx detection. Sens. Actuators B Chem. 142, 253–259. doi: 10.1016/j.snb.2009.07.053
Hua, Z., Li, Y., Zeng, Y., and Wu, Y. (2018a). A theoretical investigation of the power-law response of metal oxide semiconductor gas sensors I: Schottky barrier control. Sens. Actuators B Chem. 255, 1911–1919. doi: 10.1016/j.snb.2017.08.206
Hua, Z., Qiu, Z., Li, Y., Zeng, Y., Wu, Y., Tian, X., et al. (2018b). A theoretical investigation of the power-law response of metal oxide semiconductor gas sensors II: Size and shape effects. Sens. Actuators B Chem. 255, 3541–3549. doi: 10.1016/j.snb.2017.09.189
Hua, Z., Tian, C., Huang, D., Yuan, W., Zhang, C., Tian, X., et al. (2018c). Power-law response of metal oxide semiconductor gas sensors to oxygen in presence of reducing gases. Sens. Actuators B Chem. 267, 510–518. doi: 10.1016/j.snb.2018.04.002
Hua, Z., Tian, C., Qiu, Z., Li, Y., Tian, X., Wang, M., et al. (2018d). An investigation on NO2 sensing mechanism and shielding behavior of WO3 nanosheets. Sens. Actuators B Chem. 259, 250–257. doi: 10.1016/j.snb.2017.12.016
Jaegle, L., Steinberger, L., Martin,. R., and Chance, K. (2005). Global partitioning of NOx sources using satellite observations: relative roles of fossil fuel combustion, biomass burning and soil emissions. Faraday Discuss. 130, 407–423. doi: 10.1039/b502128f
Kida, T., Nishiyama, A., Yuasa, M., Shimanoe, K., and Yamazoe, N. (2009). Highly sensitive NO2 sensors using lamellar-structured WO3 particles prepared by an acidification method. Sens. Actuators B Chem. 135, 568–574. doi: 10.1016/j.snb.2008.09.056
Ling, Z., and Leach, C. (2004). The effect of relative humidity on the NO2 sensitivity of a SnO2/WO3 heterojunction gas sensor. Sens. Actuators B Chem. 102, 102–106. doi: 10.1016/j.snb.2004.02.017
Marichy, C., Donato, N., Latino, M., Georg Willinger, M., Tessonnier, J.-P., Neri, G., et al. (2015). Gas sensing properties and p-type response of ALD TiO2 coated carbon nanotubes. Nanotechnology 26:024004. doi: 10.1088/0957-4484/26/2/024004
Nguyen, D., and El-Safty, S. (2011). Synthesis of mesoporous NiO nanosheets for the detection of toxic NO2 gas. Chem. Eur. J. 17, 12896–12901. doi: 10.1002/chem.201101122
Richter, A., Burrows, J., Nüß, H., Granier, C., and Niemeier, U. (2005). Increase in tropospheric nitrogen dioxide over China observed from space. Nature 437, 129–132. doi: 10.1038/nature04092
Stǎnoiu, A., Simion, C., Diamandescu, L., Tǎrǎbǎşanu-Mihǎilǎ, D., and Feder, M. (2012). NO2 sensing properties of Cr2O3 highlighted by work function investigations. Thin Solid Films 522, 395–400. doi: 10.1016/j.tsf.2012.09.003
Sun, W., Shao, M., Granier, C., Liu, Y., Ye, C., and Zheng, J. (2018). Long-term trends of anthropogenic SO2, NOx, CO, and NMVOCs emissions in China. Earths Future 6, 1112–1133. doi: 10.1029/2018EF000822
Vyas, R., Sharma, S., Gupta, P., Prasad, A. K., Dhara, S., Tyagi, A. K., et al. (2013). Nitrogen dioxide induced conductivity switching in ZnO thin film. J. Alloys Comp. 571, 6–11. doi: 10.1016/j.jallcom.2013.03.217
Keywords: nitrogen dioxide, Cr2WO6, low dimensional material, gas sensors, humidity
Citation: Wu Y, Yan M, Tian C, Liu Y and Hua Z (2020) NO2 Sensing Properties of Cr2WO6 Gas Sensor in Air and N2 Atmospheres. Front. Chem. 7:907. doi: 10.3389/fchem.2019.00907
Received: 04 November 2019; Accepted: 16 December 2019;
Published: 23 January 2020.
Edited by:
Mingshui Yao, Kyoto University, JapanReviewed by:
Le-Xi Zhang, Tianjin University of Technology, ChinaLiwei Wang, Guangxi University, China
Copyright © 2020 Wu, Yan, Tian, Liu and Hua. This is an open-access article distributed under the terms of the Creative Commons Attribution License (CC BY). The use, distribution or reproduction in other forums is permitted, provided the original author(s) and the copyright owner(s) are credited and that the original publication in this journal is cited, in accordance with accepted academic practice. No use, distribution or reproduction is permitted which does not comply with these terms.
*Correspondence: Yi Wu, d3V5aUBoZWJ1dC5lZHUuY24=