- 1Department of Physics, Zhejiang Normal University, Jinhua, China
- 2Division of Physics and Applied Physics, School of Physical and Mathematical Sciences, Nanyang Technological University, Singapore, Singapore
Cu+-activated aluminosilicate oxyfluoride glasses were successfully synthesized by melt-quenching method in air by adding Al powder as a reducing agent. Their luminescent properties were studied systematically via optical absorption, excitation, and emission spectra. Upon UV irradiation, a blue-emitting band of Cu+ was observed in glass with relatively low optical basicity. By increasing the optical basicity of glass, obvious redshifts of excitation (290 → 313 nm) and emission (435 → 470 nm) peaks were obtained. Through enhancing Cu+ content, the excitation and emission bands presented further redshifts, which shifted from 313 to 338 nm and 470 to 560 nm, respectively. It is proposed that the absorption band and emitting color of Cu+-doped glass can be tuned by changing the optical basicity of glass and Cu+ concentration. The highest quantum yield can reach 62.5%. More importantly, an investigation of the thermal stability showed that over 85% of the room temperature luminescent intensity is preserved at 200°C. These results imply that Cu+-activated aluminosilicate oxyfluoride glasses may be utilized for UV-converted W-LEDs.
Introduction
Nowadays, ultraviolet (UV)-converted white light-emitting diodes (W-LEDs) have gained significant attention because they have a lower color temperature and a higher color-rendering index compared with those of commercial W-LEDs (Schubert and Kim, 2005; Pimputkar et al., 2009; Shang et al., 2014; Li et al., 2015; Zhou et al., 2015; Lu et al., 2017). One of the most popular ways to realize UV-converted W-LEDs is through the integration of UV LED chips with single-phase white light-emitting phosphors, of which organic resins are always applied as encapsulating materials (Huang et al., 2017; Wei et al., 2018b). However, the encapsulating materials deteriorate easily and turn yellow when the device operates at a higher driving current, giving rise to the shift of luminescence and the degradation of luminous efficiency and long-term reliability.
To avoid this deficiency, an alternative strategy for the combination of UV LEDs with white light luminescent glasses is expected to be developed. Luminescent glasses exhibit simple preparation, low production costs, excellent physical and chemical stability, high transparency, and outstanding optical properties. In particular, they present as free of epoxy resin in encapsulation (Dong et al., 2015; Meza Rocha et al., 2016; Kapoor et al., 2017; Ghosh et al., 2018; Li et al., 2019). Much research about white light-emitting glasses has been performed via doping co-activators including Eu2+/Eu3+/Tb3+ (Fu et al., 2012; Guo et al., 2019), Ce3+/Tb3+/Mn2+ (Ghosh et al., 2017), Ag/Eu3+ (Li et al., 2012), Bi3+/Mn2+ (Liu et al., 2016), Sb3+/Mn2+ (Guo et al., 2012b; Ming et al., 2013).
Cu+, as the other kind of activator, displays broad absorption in the UV region and wide luminescence in the visible region with a d9s → d10 transition in solids; this is very sensitive to host compositions (Xu et al., 2018; Wang et al., 2019). Hence, Cu+-activated glasses have great potential in UV-converted W-LEDs and have been studied extensively (Zhang et al., 2009; Guo et al., 2012a; Wei et al., 2012). Examples include Cu+/Mn2+ (Xu et al., 2018), (Cu+)2/Eu3+ (Guo et al., 2012a), and Cu+/Sm3+ (Wei et al., 2012)-doped glasses. However, the deep UV absorption of Cu+ in many glasses restricts its practical applications. It is still an imperative challenge to shift the absorption band of Cu+ to a longer wavelength to match the UV LED chip.
We successfully fabricated Cu+-doped aluminosilicate oxyfluoride glasses via melt-quenching means in air by adding moderate Al powder as a reducing agent. Significant redshifts of Cu+ absorption and emission bands are realized by adjusting the optical basicity of glass and Cu+ concentration. In particular, the highest quantum yield can reach 62.5% and the luminescent intensity at 200°C still maintains over 85% of that at room temperature.
Experimental Details
A series of aluminosilicate oxyfluoride glasses with a nominal molar composition of 55SiO2-20Al2O3-(15-y)CaF2-(10-x)NaF-xNa2O-yCaO-zCu2O-hAl were prepared via a conventional melt-quenching technique. The different samples are labeled and listed in Table 1. Analytical-grade ingredients of SiO2, Al2O3, CaF2, NaF, Na2CO3, CaCO3, Cu2O (A.R., from Sinopharm Chemical Reagent Co., Ltd.,) were applied as raw materials. Meanwhile, moderate Al powder (A.R., from Sinopharm Chemical Reagent Co., Ltd.,) was added as reducing agent. Batches of about 20 g of raw material were ground thoroughly for about 1 h in an agate mortar and then melted in an alumina crucible at 1550°C for 1 h in air atmosphere. After that, the glass melt was immediately poured onto a 300°C preheated copper plate and quickly pressed by another plate. Finally, all the prepared glasses used for the optical measurements were sliced and polished into a thickness of 2 mm.
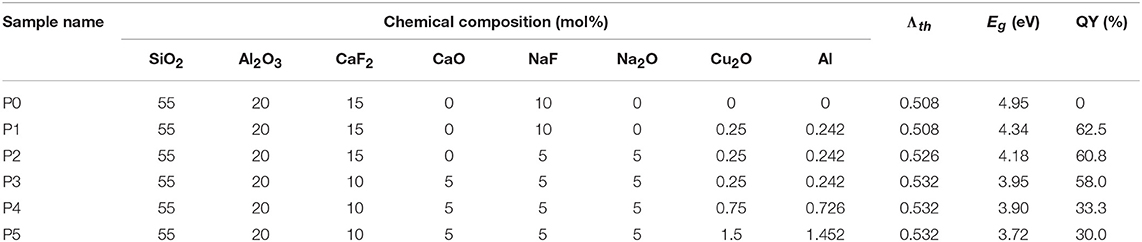
Table 1. Nominal compositions of samples (mol%), calculated optical basicity (Λth), direct optical band gap (Eg), and quantum yield (QY) values.
Optical transmission spectra were recorded using a Hitachi U-3900 UV-Visible spectrophotometer in the range of 200–900 nm. Emission and excitation spectra were performed via an Edinburgh FS5 spectrofluorometer (Edinburgh Instruments, Livingston, UK) equipped with a CW Xe-lamp (150 W). All of the above experiments were conducted at room temperature. In order to figure out the thermal quenching performance of glasses, temperature-dependent emission intensities were achieved using a FS5 spectrofluorometer applied with a TCB1402C temperature controller (China).
Results and Discussion
Figure 1 shows the transmission spectra of P0–P5 glasses. All samples present highly transparent, and the transmittances are over 80%, further verified by the photographs in the inset of Figure 1. Compared with the host P0 sample, redshifts of the absorption edge are observed in Cu+-doped glasses, which can be attributed to the absorption of Cu+, corresponding with the transition from ground d state to excited s state (Zhang et al., 2009). With the growing optical basicity of glass and Cu+ concentration, it is clearly found that the absorption edge shifts toward a longer wavelength. Optical basicity is identified as the average electron donor power of a fluorinated or oxidic compound (Herrmann et al., 2015). For a glass system consisting of fluorinated and oxidic compounds, the optical basicity can be estimated based on Duffy's empirical formula (Sontakke and Annapurna, 2012; Zheng et al., 2014; Guo et al., 2019),
where, qi is the number of fluorine or oxygen atoms in the ith component fluoride or oxide, xi is the component oxide or fluoride mole fraction, and Λi is the optical basicity of oxide or fluoride. Λi is simply 1/γ, where γ signifies the basicity-moderating parameter of the central cation. For the oxide system, values of γ are: Si4+, 2.09; Al3+, 1.64; Na+, 0.87; Ca2+, 1.00, respectively. For the fluoride system, values of γ are achieved through multiplying those for oxide systems by 2.3 (Duffy, 1989, 1996). The calculated Λi are: SiO2, 0.48; Al2O3, 0.60; CaF2, 0.43; NaF, 0.50; Na2O, 1.15; CaO, 1.00. Hence, the estimated optical basicity of samples P1–P3 is 0.508, 0.526 and 0.532 (shown in Table 1), respectively.
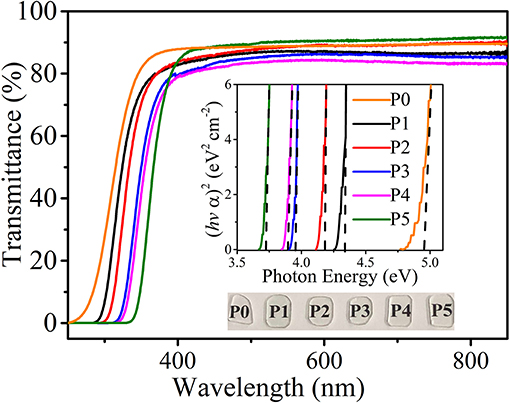
Figure 1. Transmission spectra of samples P0–P5. Insets present the relationship of (hvα)2 vs. photo energy and the photographs of samples P0–P5, respectively.
Usually, the absorption edge is related to the optical band gap of glass. On the basis of the theory reported by Mott and Davis, the direct optical band gap (Eg) is calculated via the formula (Liu et al., 2016),
where, α stands for the absorption coefficient, hv corresponds to the photon energy in eV, and B is a constant. The inset of Figure 1 depicts the plots of (hvα)2 with respect to hv for different samples. Finally, Eg is extracted from the extrapolation of the linear region of (hvα)2 = 0 and the respective values are displayed in Table 1. The values of the optical band gap reduce gradually with an increase in the optical basicity of glass and Cu+ content. In addition, no evident absorption bands from 400 to 850 nm are observed, suggesting that almost no Cu2+ or Cu nanoparticles in the obtained glasses exist (Xu et al., 2018).
Figure 2 portrays the normalized excitation and emission spectra of samples P1–P5 at room temperature. When irradiated by 290 nm UV light, a blue-emitting band centered at 435 nm is observed in P1 glass, resulting from the s → d transition of Cu+ (Guo et al., 2012a). While monitored at 435 nm wavelength, excitation spectra present a broad absorption band peaking at 290 nm, corresponding to the d → s transition of Cu+ (Guo et al., 2012a). Surprisingly, we can observe that the excitation and emission peaks of Cu+ can be shifted by changing the optical basicity of glass. As exhibited in Figures 2A,B, with the growing optical basicity of glass, it is clear that excitation and emission peaks of Cu+ experience redshifts from 290 to 313 nm and 435 to 470 nm, respectively. A growing optical basicity of the glass system signifies an augmenting polarizability at the local active ion sites (Herrmann et al., 2015). According to Dorenbos (2001, 2002), an augmenting polarizability leads to an increasing shift in the excited state of active ions to lower energies, indicative of redshifts of the absorption edge, excitation and emission peaks of the prepared samples.
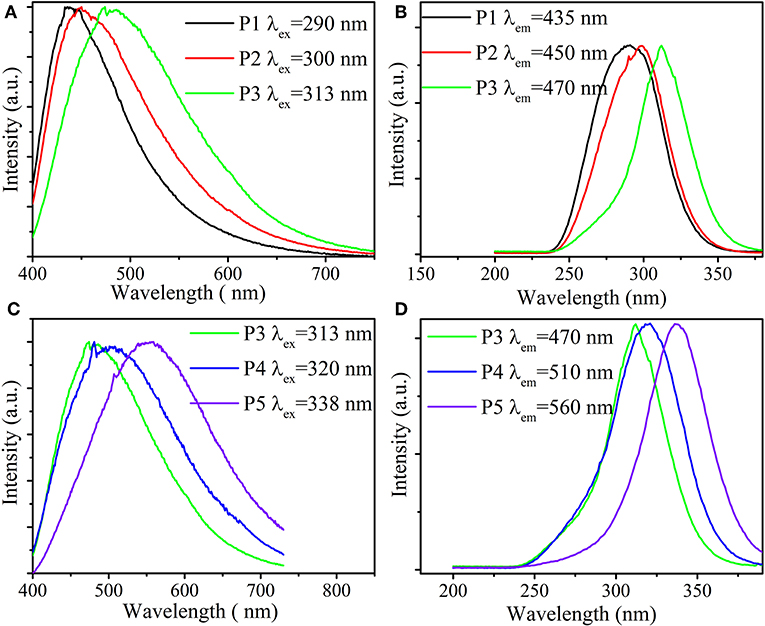
Figure 2. Emission (A) and excitation (B) spectra of samples P1–P3 as increasing optical basicity of glass. Emission (C) and excitation (D) spectra of samples P3–P5 with growing Cu+ content.
On the other hand, the influence of the Cu+ content on the excitation and emission peaks was also investigated. As displayed in Figures 2C,D, the excitation and emission peaks, from 313 to 338 nm and from 470 to 560 nm, respectively, shift to longer wavelengths as Cu+ concentration increases. Such phenomena may be explained as follows (Barrie et al., 1989; Guo et al., 2012a): with growing Cu+ concentration, the distance between Cu+ ions is shortened and the overlap of the s excited state of Cu+ is enhanced, bringing the s excited state closer to the d ground state. Hence, increasing Cu+ content can also induce the redshifts of excitation and emission peaks.
The CIE color coordinates in Figure 3 first depict the evident variation of the emission color from blue to green with increasing optical basicity of glass and then from green to yellow with increasing Cu+ content. These results suggest that the absorption band and emitting color of Cu+-doped glass can be modified by varying the optical basicity of glass and Cu+ content.
The quantum yield (QY) of samples P1–P5 was measured and the values can be estimated via the following formula (Guo et al., 2019),
where, LS stands for the emission spectra of the obtained glasses, and ER and ES represent the spectra of absorption light with and without the obtained glasses in integrating spheres. The estimated QY of Cu+-doped aluminosilicate oxyfluoride glasses are listed in Table 1. The highest QY can reach 62.5%.
The thermal stability of luminescent material is of great importance due to its practical applications. Thus, the temperature-dependent emission behaviors of samples P1–P5 when irradiated at 338 nm light were investigated. As delineated in Figure 4A, the luminescent intensity of sample P5 is almost unchanged at first when temperature augments from room temperature to 140°C, over which the emission intensity gradually decreases due to thermal quenching (because the optimum excitation wavelength of P5 is largest compared with other samples, the temperature-dependent emission spectra of P5 are presented in the figure). Figure 4B plots the integrated emission intensities with respect to the temperature of Cu+-doped glasses. It shows that luminescent intensities at 200°C of all the Cu+-doped samples still maintain over 85% of those measured at room temperature (40°C), which are superior to those of most recently reported luminescent materials (Li et al., 2015; Zhou et al., 2015; Guo et al., 2019). Generally, the thermal stability of luminescent material can be assessed by the activation energy (ΔE), where higher ΔE signifies better thermal stability (Qiao et al., 2018). ΔE can be calculated by using the Arrhenius equation (Li et al., 2015; Liu et al., 2016; Wei et al., 2018a),
where, I0 and I(T) stand for the integrated luminescent intensities at initial and differently operated temperatures, respectively. C represents a constant and k corresponds to the Boltzmann constant (8.625 × 10−5 eV/K). Figure 4C illustrates the relationship of In[(I0/I(T))-1] vs. 1/kT for sample P5. The slope of the best fit line is −0.52463, which means that ΔE is approximately 0.52 eV. The activation energy of the as-prepared glass is higher than that of most popular luminescent materials including nitrides (Wang et al., 2014), silicates (Lv et al., 2014; Wei et al., 2018a), etc. Such results imply that Cu+-doped aluminosilicate oxyfluoride glasses have a promising utilization in solid-state lighting and other areas.
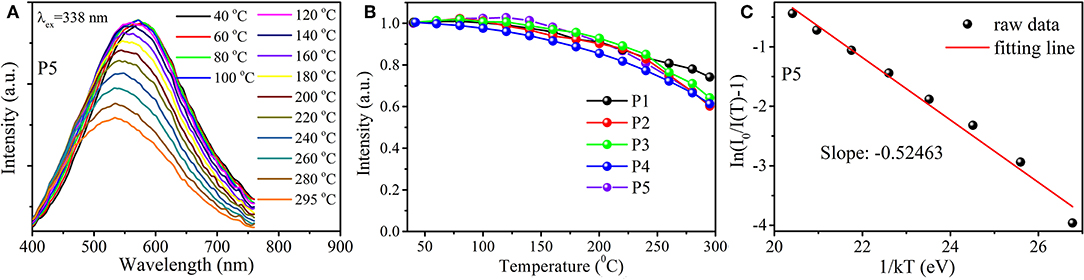
Figure 4. (A) Emission spectra of sample P5 at temperature from 40 to 295°C under irradiation at 338 nm; (B) Integrated emission intensities of samples P1–P5 with various temperatures; (C) Relationship of In[I0/I(T)-1] with respect to 1/kT for sample P5.
Conclusion
Cu+-doped aluminosilicate oxyfluoride glasses with high transparency (over 80%) were successfully prepared in air using Al powder as a reducing agent. Broad UV absorption and blue-emitting bands in Cu+-doped glass with relatively low optical basicity were obtained. By promoting the optical basicity of glass, excitation and emission peaks can be modified to longer wavelengths, which are from 290 to 313 nm and 435 to 470 nm, respectively. Besides, by growing Cu+ concentration, further redshifts of excitation (313 → 338 nm) and emission (470 → 560 nm) peaks are realized. Such work paves pathways for tuning the absorption band and emitting color of Cu+-doped glass, of which the highest quantum yield can reach 62.5%. More importantly, at 200°C, the as-prepared Cu+-doped glasses still retain over 85% luminescent intensities at 40°C. This value is higher than that of most recently reported luminescent materials. All the situations suggest that Cu+-doped aluminosilicate oxyfluoride glasses are potential candidates for application in solid-state lighting and other areas.
Data Availability Statement
All datasets generated for this study are included in the manuscript/supplementary files.
Author Contributions
YS investigated the luminescent properties of samples and wrote the article. RW and HG provided the experimental ideas and revised the article. JG prepared the samples. XT and FH measured the spectra and recorded the experimental data.
Funding
This work was financially supported by the National Natural Science Foundation of China (Grant Nos. 51802285, 11804303), the Natural Science Foundation of Zhejiang Province (Grant No. LQ18A040004), and the Open Fund of the State Key Laboratory of Luminescent Materials and Devices (South China University of Technology).
Conflict of Interest
The authors declare that the research was conducted in the absence of any commercial or financial relationships that could be construed as a potential conflict of interest.
References
Barrie, J. D., Dunn, B., Hollingsworth, G., and Zink, J. I. (1989). Optical spectroscopy of copper(I)-doped Na+-β” alumina. J. Phys. Chem. 93, 3958–3963. doi: 10.1021/j100347a018
Dong, G., Wang, H., Chen, G., Pan, Q., and Qiu, J. (2015). Quantum dot-doped glasses and fibers: fabrication and optical properties. Front. Mater. 2:13. doi: 10.3389/fmats.2015.00013
Dorenbos, P. (2001). 5d-level energies of Ce3+ and the crystalline environment. III. Oxides containing ionic complexes. Phys. Rev. B 64:125117. doi: 10.1103/PhysRevB.64.125117
Dorenbos, P. (2002). Relating the energy of the [Xe]5d1 configuration of Ce3+ in inorgnic compounds with anion polarizability and cation electronegativity. Phys. Rev. B 65, 2351101–2351106. doi: 10.1103/PhysRevB.65.235110
Duffy, J. A. (1989). A common optical basicity scale for oxide and fluoride glasses. J. Non-Cryst. Solids. 109, 35–39. doi: 10.1016/0022-3093(89)90438-9
Duffy, J. A. (1996). Redox equilibria in glass. J. Non-Cryst. Solids 196, 45–50. doi: 10.1016/0022-3093(95)00560-9
Fu, H., Qiao, X., Cui, S., Luo, Q., Qian, J., Fan, X., et al. (2012). Tunable white light emission from glass-ceramics containing Eu2+, Tb3+, Eu3+ co-doped SrLaF5 nanocrystals. Mater. Lett. 71, 15–17. doi: 10.1016/j.matlet.2011.12.004
Ghosh, D., Biswas, K., Balaji, S., and Annapurna, K. (2017). Tunable white light generation from Ce3+-Tb3+-Mn2+ doped metaphosphate glass for LED and solar cell applications. J. Lumin. 183, 143–149. doi: 10.1016/j.jlumin.2016.11.025
Ghosh, D., Biswas, K., Balaji, S., and Annapurna, K. (2018). Realization of warm white light from Ce-Eu-Tb doped zinc fluoroboro silicate glass for lighting applications. J. Alloys Compd. 747, 242–249. doi: 10.1016/j.jallcom.2018.02.326
Guo, H., Teng, L., and Wei, R. F. (2019). Tunable white light emission and energy transfer of Eu2+-Tb3+-Eu3+ tri-activated glasses synthesized in air. J. Am. Ceram. Soc. 102, 6777–6786. doi: 10.1111/jace.16532
Guo, H., Wei, R., and Liu, X. (2012a). Tunable white luminescence and energy transfer in (Cu+)2, Eu3+ codoped sodium silicate glasses. Opt. Lett. 37, 1670–1672. doi: 10.1364/OL.37.001670
Guo, H., Wei, R., Wei, Y., Liu, X., Gao, J., and Ma, C. (2012b). Sb3+/Mn2+ co-doped tunable white emitting borosilicate glasses for LEDs. Opt. Lett. 37, 4275–4277. doi: 10.1364/OL.37.004275
Herrmann, A., Othman, H. A., Assadi, A. A., Tiegel, M., Kuhn, S., and Ruessel, C. (2015). Spectroscopic properties of cerium-doped aluminosilicate glasses. Opt. Mater. Express 5, 720–732. doi: 10.1364/OME.5.000720
Huang, A., Yang, Z., Yu, C., Chai, Z., Qiu, J., and Song, Z. (2017). Tunable and white light emission of a single-phased Ba2Y(BO3)2Cl:Bi3+,Eu3+ phosphor by energy transfer for ultraviolet converted white LEDs. J. Phys. Chem. C 121, 5267–5276. doi: 10.1021/acs.jpcc.7b00019
Kapoor, S., Wondraczek, L., and Smedskjaer, M. M. (2017). Pressure-induced densification of oxide glasses at the glass transition. Front. Mater. 4:1. doi: 10.3389/fmats.2017.00001
Li, J. J., Chen, J. D., Wei, R. F., and Guo, H. (2012). Combined white luminescence from Eu3+, ML-Ag particles and Ag+ in Ag-Eu3+ co-doped H3BO3-BaF2 glasses. J. Am. Ceram. Soc. 95, 1208–1211. doi: 10.1111/j.1551-2916.2012.05112.x
Li, K., Fan, J., Shang, M., Lian, H., and Lin, J. (2015). Sr2Y8(SiO4)6O2:Bi3+/Eu3+: a single-component white-emitting phosphor via energy transfer for UV w-LEDs. J. Mater. Chem. C 3, 9989–9998. doi: 10.1039/C5TC01993A
Li, X., Guo, H., Liu, X., Wei, R., Hu, F., and Zhou, S. (2019). A new parameter for characterizing Eu3+ distribution in mixed-valence Eu-doped cubic LaF3-based transparent glass-ceramics. J. Am. Ceram. Soc. 102, 6640–6648. doi: 10.1111/jace.16542
Liu, X. Y., Guo, H., Liu, Y., Ye, S., Peng, M. Y., and Zhang, Q. Y. (2016). Thermal quenching and energy transfer in novel Bi3+/Mn2+ co-doped white-emitting borosilicate glasses for UV LEDs. J. Mater. Chem. C 4, 2506–2512. doi: 10.1039/C6TC00370B
Lu, X., Liu, H., Yang, X., Tian, Y., Gao, X., Han, L., et al. (2017). A single-phase white-emitting La10(SiO4)6O3: Eu2+/Eu3+ phosphor for near-UV LED-based application. Ceram. Inter. 43, 11686–11691. doi: 10.1016/j.ceramint.2017.05.358
Lv, W., Jia, Y., Zhao, Q., Jiao, M., Shao, B., Lu, W., et al. (2014). Crystal structure and luminescence properties of Ca8Mg3Al2Si7O28:Eu2+ for WLEDs. Adv. Opt. Mater. 2, 183–188. doi: 10.1002/adom.201300448
Meza Rocha, A. N., Lozada Morales, R., Speghini, A., Bettinelli, M., and Caldino, U. (2016). White light generation in Tb3+/Eu3+/Dy3+ triply-doped Zn(PO3)2 glass. Opt. Mater. 51, 128–132. doi: 10.1016/j.optmat.2015.11.032
Ming, C., Song, F., Ren, X., and An, L. (2013). High efficient white-light in Sb3+/Mn2+ co-doped P2O5-Li2O-Al2O3 glass. J. Alloys Compd. 580, 465–467. doi: 10.1016/j.jallcom.2013.06.145
Pimputkar, S., Speck, J. S., DenBaars, S. P., and Nakamura, S. (2009). Prospects for LED lighting. Nat. Photonics. 3, 179–181. doi: 10.1038/nphoton.2009.32
Qiao, J., Ning, L., Molokeev, M. S., Chuang, Y. C., Liu, Q., and Xia, Z. (2018). Eu2+ site preferences in the mixed cation K2BaCa(PO4)2 and thermally stable luminescence. J. Am. Chem. Soc. 140, 9730–9736. doi: 10.1021/jacs.8b06021
Schubert, E. F., and Kim, J. K. (2005). Solid-state light sources getting smart. Science 308, 1274–1278. doi: 10.1126/science.1108712
Shang, M., Li, C., and Lin, J. (2014). How to produce white light in a single-phase host? Chem. Soc. Rev. 43, 1372–1386. doi: 10.1039/C3CS60314H
Sontakke, A. D., and Annapurna, K. (2012). Study on Tb3+ containing high silica and low silica calcium aluminate glasses: Impact of optical basicity. Spectrochim. Acta A Mol. Biomol. Spectrosc. 94, 180–185. doi: 10.1016/j.saa.2012.03.052
Wang, X., Seto, T., Zhao, Z., Li, Y., Wu, Q., Li, H., et al. (2014). Preparation of Sr1−xCaxYSi4N7:Eu2+ solid solutions and their luminescence properties. J. Mater. Chem. C 2, 4476–4481. doi: 10.1039/C4TC00190G
Wang, Y., Di Sarcina, I., Cemmi, A., Baccaro, S., and Chen, G. (2019). Enhanced, shortened and tunable emission in Eu3+ doped borosilicate glasses by Cu+ co-doping. Opt. Mater. 87, 80–83. doi: 10.1016/j.optmat.2018.05.056
Wei, R., Ma, C., Wei, Y., Gao, J., and Guo, H. (2012). Tunable white luminescence and energy transfer in novel Cu+, Sm3+ co-doped borosilicate glasses for W-LEDs. Opt. Express 20, 29743–29750. doi: 10.1364/OE.20.029743
Wei, R., Wang, L., Hu, F., Li, X., Peng, X., Shi, Y., et al. (2018a). Tunable emission and energy transfer in single-phased Ba9Lu2Si6O24:Bi3+, Eu3+ for UV W-LEDs. J. Lumin. 197, 291–296. doi: 10.1016/j.jlumin.2018.01.033
Wei, R., Zheng, Z., Shi, Y., Peng, X., Wang, H., Tian, X., et al. (2018b). Tunable emission with excellent thermal stability in single-phased SrY2O4:Bi3+,Eu3+ phosphors for UV-LEDs. J. Alloys Compd. 767, 403–408. doi: 10.1016/j.jallcom.2018.07.101
Xu, D. K., Shi, Y. F., Peng, X. S., Wei, R. F., Hu, F. F., and Guo, H. (2018). Tunable broad photoluminescence in Cu+/Mn2+ co-doped oxyfluoride glasses sintered in air atmosphere. J. Lumin. 202, 186–191. doi: 10.1016/j.jlumin.2018.05.050
Zhang, Q., Chen, G., Dong, G., Zhang, G., Liu, X., Qiu, J., et al. (2009). The reduction of Cu2+ to Cu+ and optical properties of Cu+ ions in Cu-doped and Cu/Al-codoped high silica glasses sintered in an air atmosphere. Chem. Phys. Lett. 482, 228–233. doi: 10.1016/j.cplett.2009.09.105
Zheng, L. H., Sun, X. Y., Mao, R. H., Chen, H. H., Zhang, Z. J., and Zhao, J. T. (2014). Luminescence properties of Ce3+-doped lithium borophosphate glasses and their correlations with the optical basicity. J. Non-Cryst. Solids 403, 1–4. doi: 10.1016/j.jnoncrysol.2014.06.010
Keywords: Cu+, glass, tunable luminescence, optical basicity, quantum yield, thermal stability
Citation: Shi Y, Wei R, Guo J, Tian X, Hu F and Guo H (2019) Influence of Optical Basicity on Cu+ Luminescence in Aluminosilicate Oxyfluoride Glasses. Front. Mater. 6:246. doi: 10.3389/fmats.2019.00246
Received: 19 July 2019; Accepted: 18 September 2019;
Published: 10 October 2019.
Edited by:
Jianrong Qiu, Zhejiang University, ChinaReviewed by:
Olga Sergeevna Dymshits, Vavilov State Optical Institute, RussiaStefan Karlsson, RISE Research Institutes of Sweden, Sweden
Copyright © 2019 Shi, Wei, Guo, Tian, Hu and Guo. This is an open-access article distributed under the terms of the Creative Commons Attribution License (CC BY). The use, distribution or reproduction in other forums is permitted, provided the original author(s) and the copyright owner(s) are credited and that the original publication in this journal is cited, in accordance with accepted academic practice. No use, distribution or reproduction is permitted which does not comply with these terms.
*Correspondence: Rongfei Wei, cmZ3ZWlAempudS5lZHUuY24=; Hai Guo, Z2hoQHpqbnUuY24=