- College of Horticulture, Gansu Agricultural University, Lanzhou, China
Both nitric oxide (NO) and calcium ion (Ca2+)/calmodulin (CaM) have been shown to regulate the senescence of cut flowers. However, not much is known about the crosstalk between NO and Ca2+/CaM during the senescence of cut flowers. In this study, cut Oriental × Trumpet hybrid lily “Manissa” were used to investigate the roles and relationship between NO and Ca2+/CaM during postharvest freshness. The results show that the effects of CaCl2 or NO donor SNAP on the vase life, maximum flower diameter and hours until full opening were dose-dependent, with an optimum concentration of 20 mM CaCl2 or 100 μM SNAP. However, Ca2+ chelators EGTA or BAPTA/AM, Ca2+ channel inhibitors LaCl3 or nifedipine and CaM antagonists W-7 or TFP inhibited the promotion of SNAP. SNAP applied alone significantly increased the endogenous Ca2+/CaM contents in cut lily flowers, while EGTA, BAPTA/AM, LaCl3, nifedipine, W-7, and TFP decreased the advancement of SNAP. In addition, the SNAP-induced Ca2+-ATPase activity was more than twice as much as the control, but EGTA, BAPTA/AM, LaCl3, nifedipine, W-7, and TFP also reversed the enhancement. Moreover, EGTA, BAPTA/AM, LaCl3, nifedipine, W-7, and TFP prevented the SNAP-induced upregulation of gene expression of CaM, CBL1, and CBL3, which is associated with calcium signaling pathway. Overall, these results suggest that Ca2+/CaM may function as downstream molecules in NO-regulated senescence of cut flowers.
Introduction
Cut lilies (Lilium spp.), a bulbous plant with large trumpet-shaped and typically fragrant flowers (Liao et al., 2013), are in demand worldwide because of their superior commercial and ornamental value. However, the postharvest life of cut lilies is usually short because of wilting, color changing, abscission, and early leaf yellowing (de Almeida et al., 2017). Senescence is the main reason for the short vase life and poor quality of cut flowers, which involves a general degradation of nucleic acids, proteins, and cell membranes, as well as increased activities of RNase and other hydrolytic enzymes (Shabanian et al., 2018). These structural, biochemical, and molecular changes are also the hallmarks of programmed cell death (PCD). Therefore, ethylene-induced PCD is a critical factor of senescence for ethylene-sensitive flowers (Zhou et al., 2005). Moreover, postharvest life and quality of cut flowers are controlled by a combination of factors including multiple genetic factors, pre-harvest environmental conditions throughout the supply chain, plant maturity and season of planting and harvesting, plant nutritional status, sensitivity to ethylene and oxidative stress, and postharvest temperature fluctuations and water balance (Liao et al., 2013). Therefore, to enhance vase life and maintain quality of cut flowers, convenient, ecological, and economical approaches to decelerate senescence are needed. Furthermore, understanding the mechanism of these fresh preservation methods is of vital importance for exploring new approaches for postharvest freshness.
Previous studies have shown that nitric oxide (NO) may function as an influential plant growth regulator (Asgher et al., 2017). It is evident that NO as a signaling molecule mediates many specific developmental processes, including seed dormancy or germination, de-etiolation, hypocotyl elongation, stomatal movement, pollen tubes growth, flowering, cell wall lignification, xylem differentiation, cellulose biosynthesis, chlorophyll biosynthesis/photosynthesis, gravitropism, cell polarity, maturation, senescence, and root organogenesis (Luis et al., 2015). NO also mediates various plant abiotic responses, such as salinity, water stress, extreme heat and cold, mechanical injury, UV radiation, ozone, heavy metal toxicity, herbicide, nutrient deficiency, and among other risks (Fancy et al., 2016). A recent study found that NO increased water uptake and promoted antioxidant activity and consequently enhanced vase life of cut gerbera flowers (Shabanian et al., 2018). NO in plants is produced by a variety of enzymatic and non-enzymatic mechanisms (Benavides et al., 2016). The enzymatic biosynthesis including NO synthesis (NOS)-like enzymes, nitrite reductase (NR), xanthine oxidase/dehy-drogenase (XDH) and nitric: NO oxidoreductase (Ni-NOR) (Liao et al., 2012a). The arginine and nitrite pathways are most plausible routes in NO generation. The NOS activity has been documented in many plant species, but no cloned NOS enzyme has been identified. NR is the best described enzymatic source of NO in plants which catalyzes nitrite to NO rely on NAD(P)H (Chamizo-Ampudia et al., 2017).
As an essential cytoplasmic second messenger, calcium ion (Ca2+) plays critical roles in plant response to biotic and abiotic stresses, including light, unfavorable temperature, salt and osmotic stress, phytohormones, oxidative stress, wind stimulation, wounding, and anoxia. Ca2+ also plays an important role in plant membrane stability, cell wall stabilization, and cell integrity (Ranty et al., 2016). Moreover, Ca2+ was reported to delay senescence of cut rose flowers by protecting both membrane phospholipids and membrane proteins from degradation, and reducing ethylene production (Torre et al., 1999). In response to various environmental changes, Ca2+ signals must be decoded by several Ca2+ sensors or Ca2+ binding proteins. Calmodulins (CaMs), calcineurin B-like (CBL) proteins, and calcium-dependent protein kinase (CDPK) are three main families of Ca2+ sensors (Boudsocq and Sheen, 2013). CaM, one of the most ubiquitous calcium-modulated proteins, is highly conserved during evolution. CaM transmits Ca2+ signal through interacting target proteins and regulating their activities, and subsequently regulates many critical processes such as immunity, pollen tube growth, cell wall regeneration, cell division, defense against necrotrophic pathogens, and high temperature tolerance (Liao et al., 2013). CDPKs are versatile and evolutionarily conserved Ca2+ sensors/transducers that function in a diverse array of plant process in immune and stress signaling networks (Boudsocq and Sheen, 2013). CBLs also play vital roles in plant responses to diverse abiotic stress (Lu et al., 2017).
Furthermore, there is a crosstalk among NO, Ca2+, and CaM in plant growth and development as well as in response to abiotic stresses (Takata et al., 2011). On the one hand, the synthesis of NO by NOS is strictly Ca2+/CaM dependent. Ca2+ can act as both a promoter and a sensor of NO signaling pathways (Jeandroz et al., 2013). Similarly, Ca2+ signaling can induce endogenous NO accumulation during stomatal closure in Arabidopsis guard cells (Wang et al., 2012). On the other hand, NO regulates the gating/conductance of Ca2+ channels and impact the activity of Ca2+ pumps as well as Ca2+ sensors. Nearly all types of Ca2+ channels appear to be mediated by NO (Clementi and Meldolesi, 1997). The study on marigold indicate that exogenous NO promoted adventitious rooting by increasing endogenous Ca2+ and CaM levels (Liao et al., 2012b). In addition, the involvement of Ca2+/CaM in NO-induced adventitious rooting in cucumber under simulated osmotic stress has also been studied (Niu et al., 2017). Thus, Ca2+ appears to be both upstream and downstream of NO signaling during plant growth and stress responses. Therefore, there is a complex interplay that exists between Ca2+/CaM and NO.
It is clear that both exogenous NO and Ca2+ can enhance vase life and keep quality during postharvest freshness of cut flowers by inhibiting ethylene production and delaying bending, respectively (Liao et al., 2013; Perik et al., 2014). However, not much is known about the crosstalk among NO and Ca2+/CaM on flowers freshness. In this study, cut Oriental × Trumpet hybrid lilium “Manissa” were used to investigate the mechanism by which Ca2+/CaM was involved in NO-enhanced postharvest freshness.
Materials and Methods
Plant Materials and Treatments
Cut Oriental × Trumpet hybrid lilium “Manissa” were purchased from a wholesale cut flowers market in Lanzhou, China. Upon arrival in the laboratory, green bud stage (commercial harvest stage) of lilies were rehydrated for 2 h, then the flowering stems were recut to a uniform length of 45 cm under distilled water to avoid air embolism. The blades of cutters were surface sterilized by rinsing in 95% (v/v) ethanol prior to use. The flowers were selected for uniformity of size, color, and free from any defects. All experiments were performed in the laboratory at temperature of 20 ± 2°C, relative humidity of 60 ± 10%, and light intensity of 12 μmol m-2 s-1 from cool white florescent tubes with a daily light period of 12 h.
Experiment 1: after selecting the flowers, every 5 flowers were randomly put in a 2500 mL glass vase containing 1000 mL distilled water (control 1), 40 mM potassium chloride (KCl, control 2) or various concentrations of 1000 mL of test solutions. Unless otherwise specified, the word “control” refers to control 1 in the full text. Glass vases were surface sterilized with ethanol which was used for cutters as described above. There were two kinds of test solutions which were respectively made by NO donor S-nitro-N-acetyl-penicillamine (SNAP) or calcium dichloride (CaCl2). The doses of SNAP solution were 50, 100, 200, and 400 μM while the concentration grads of CaCl2 solution were 5, 10, 20, 40, and 60 mM. After three replications of each treatment, 100 μM SNAP or 20 mM CaCl2 treatment was used in the following study.
Experiment 2: 10 treatments were applied: (1) Control (distilled water); (2) 100 μM S-nitro-N-acetyl-penicillamine (SNAP); (3) 20 mM calcium dichloride (CaCl2); (4) 120 μM [Ethylene glycol-bis (-aminoethy ether) N, N, N′, N′-tetraacetic acid] (EGTA) + SNAP; (5) 30 μM [1, 2-bis (2-aminophenoxy) ethane–N, N, N′, N′-tetraacetic acid tetrakis (acetoxymethyl ester)] (BAPTA/AM, Tokyo Chemical Industry, Tokyo, Japan) + SNAP; (6) 500 μM lanthanum chloride (LaCl3, Solarbio, Beijing, China) + SNAP; (7) 150 μM nifedipine (Tokyo Chemical Industry, Tokyo, Japan) +SNAP; (8) 80 μM [N-(6-aminohexyl)-5-chloro-1-naphtalene sulfonamide] (W-7, Tokyo Chemical Industry, Tokyo, Japan)+ SNAP; (9) 100 μM trifluoperazine (TFP, Solarbio, Beijing, China) + SNAP; (10) 150 μM nifedipine. Each treatment was conducted in three replications. The concentrations of these chemicals were selected based on the result of a recent experiment conducted in our laboratory (Niu et al., 2017). Fresh test solutions were made every day to replace the vase solutions which were made the day before.
Vase Life and Maximum Flower Diameter Determination
The vase life of each cut lily flower was determined by the number of days from the time when the flower was placed in the vase solution until flower had no decorative value (wilting, discoloration, or stem bending). The flower diameter was defined as the maximum width of each flower and measured by a vernier caliper. The hours until full flower opening (HFO) was determined by the number of hours from the time when the flower was placed into the vase solution until the flower diameter reached the maximum value. There was an observation every 6 h at the same time of a day.
Determination of Calcium (Ca2+), Calmodulin (CaM), and Ca2+-ATPase Activity
The Ca2+ level in the cut lily leaves were measured by atomic absorption spectral analysis of nitric–perchloric acid digests. Soluble protein content in the extracts for CaM assay was determined according to Bradford (1976) using BSA as standard. The CaM contents were determined by its capacity to activate bovine brain cAMP phosphodiesterase as described by Ye et al. (1990) using maize germ CaM as a standard (Gong et al., 1996). The determination of Ca2+-ATPase activity was performed using the modified one-step lead method of Jian et al. (1999).
Fluorescence Quantitative Real-Time PCR
Total RNA was abstracted from healthy lily leaves with TaKaRa MiniBEST plant RNA extraction kit (Takara Bio Inc., Kusatsu, Shiga, Japan) according to the manufacturer’s instructions. The concentration of total RNA was measured using an ultraviolet spectrophotometer, and the quality of RNA was determined based on its OD260/OD280 ratio. About 2 μL of DNA-free total RNA was used for synthesis cDNA with a PrimeScript RT Master Mix kit (Takara Bio Inc., Kusatsu, Shiga, Japan). Quantitative real-time PCR reactions were performed with a Mastercycler®ep realplex real-time PCR system (Eppendorf, Hamburg, Germany) with SYBR® Premix Ex TaqTM (Takara Bio Inc., Kusatsu, Shiga, Japan). The cDNA was amplified using specific primers (Supplementary Table S1). The amplification conditions were as follows: 95°C pre-degeneration for 30 s and repeat once, followed by 40 cycles of 95°C for 15 s, and then annealing at 60°C for 30 s. The expression levels of corresponding genes were presented as values relative to the corresponding control samples under the indicated conditions, with normalization of data to the geometric average of internal lily actin. Each sample was set three biological replicates.
Statistical Analysis of the Data
All of data presented in the tables and figures were expressed at the mean value ± SE from three independent duplications (five flowering stems per replication). Data collected were subjected to analysis of variance (ANOVA), and statistical divergence among treatments was analyzed through Duncan’s multiple range test (P < 0.05). All statistical analysis was carried out using the statistical package for social science for windows (version13.00; SPSS, Inc., Chicago, IL, United States).
Results
Effects of Various Concentrations of CaCl2 and SNAP on Senescence
There was no difference in the vase life of cut lilies between treatments with distilled water (control 1) and KCl (control 2; Table 1). CaCl2 treatments at the concentration of 10, 20, and 40 mM significantly extended the vase life of cut lily flowers compared to both control 1 and control 2. However, when tested at 5 mM and 60 mM CaCl2, each of the concentration had no significant effects on vase life. The longest vase life obtained by 20 mM CaCl2 treatment, which was 21 and 19% longer than the control 1 and control 2, respectively. As shown in Table 1, exogenous CaCl2 at proper levels (10, 20, and 40 mM) increased the maximum flower diameter of cut lily significantly in comparison to the control. Treatment with 20 mM CaCl2 created the maximum value. Placing cut lily flowers in the 20 mM CaCl2 solution created the maximum HFO, while 5 and 60 mM did not influence HFO (Table 1).
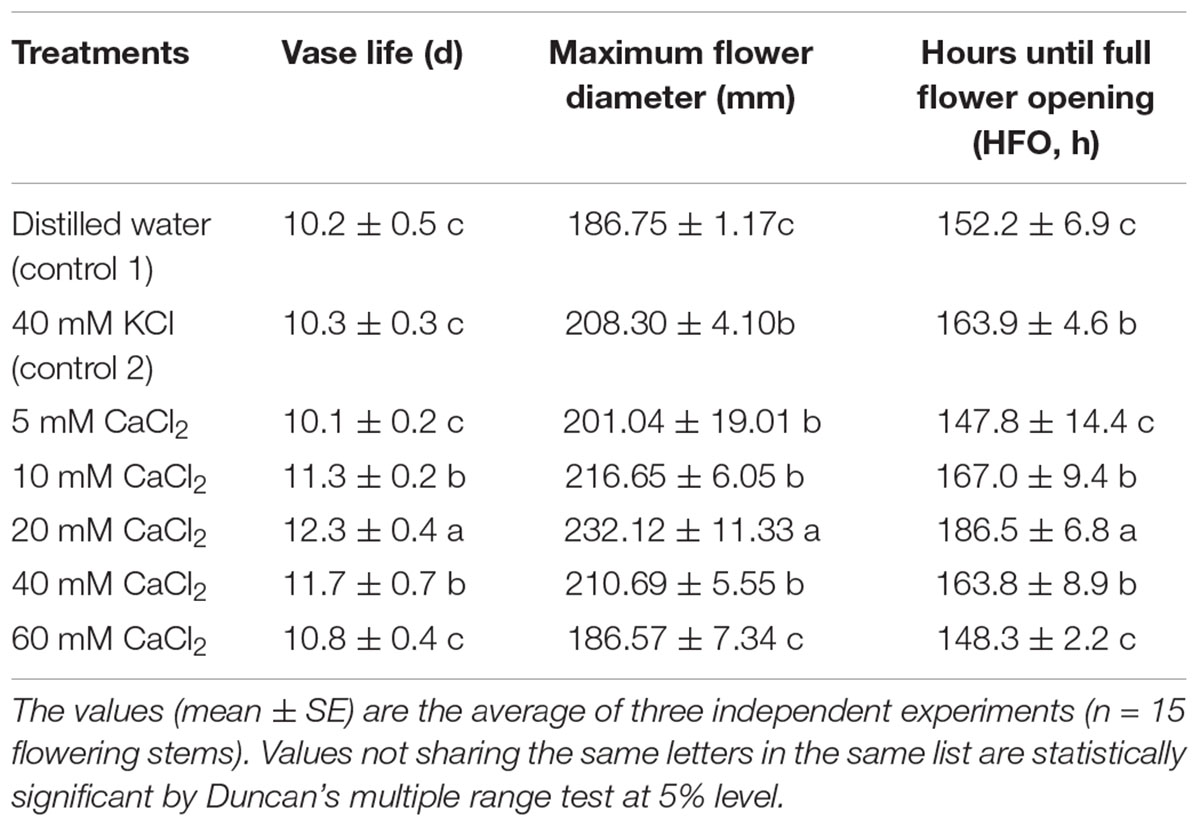
TABLE 1. Effects of various concentrations of CaCl2 on vase life, maximum flower diameter, and hours until full flower opening of cut OT hybrids lily “Manissa.”
As Table 2 shows, the effects of NO donor SNAP on the senescence of cut lily were dose-dependent. The vase life was obviously prolonged by lower dosage of SNAP (50, 100, and 200 μM) while higher dosage of SNAP (400 μM) was phytotoxic, thereby resulting in a shortened vase life. Treatment with 100 μM SNAP produced the longest vase life, which was about 132.29% of the control. Compared to the control, all various concentrations of treatments with SNAP markedly increased the maximum diameter (Table 2). Both treatments with 100 and 200 μM SNAP significantly extended the maximum flower diameter, whereas 100 μM is the best. Treatment with 100 μM SNAP extended HFO, which increased by 44.98% compared with the control. Therefore, 20 mM CaCl2 and 100 μM SNAP might delay senescence by slowing down flower opening, and they were used in further study in this experiment.
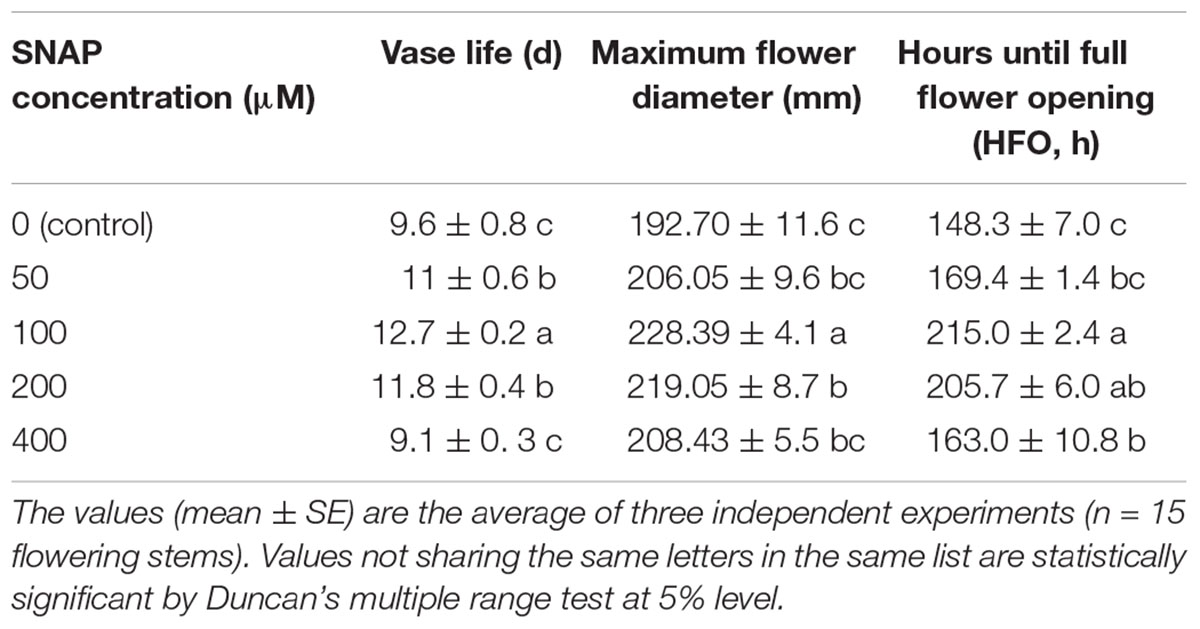
TABLE 2. Effects of various concentrations of a NO donor SNAP on vase life, maximum flower diameter, and hours until full flower opening of cut OT hybrids lily “Manissa.”
Effects of Ca2+ Chelators, Ca2+ Channel Inhibitors, and CaM Antagonists on SNAP-Delayed Senescence
To further analyze whether Ca2+ and CaM is involved in the NO-enhanced postharvest freshness of cut lily flowers, effects of Ca2+ chelators (EGTA and BAPTA/AM), Ca2+ channel inhibitors (LaCl3 and nifedipine), and CaM antagonists (W-7 and TFP) co-treated with SNAP were studied (Figure 1 and Table 3). When treated with SNAP solution alone, the vase life was the longest, which was 26% longer than that of the control. Treatments with SNAP plus Ca2+ chelators or Ca2+ channel inhibitors cut down the advancement of vase life, flower diameter and HFO caused by SNAP to some extent, thus produced significant effects on anti-aging of cut lily flowers. However, when CaM antagonists W-7 or TFP was added to SNAP containing solution, it reversed the SNAP-induced positive impact on cut flowers. In addition, when cut lilies were treated with nifedipine alone, the vase life and HFO was significant suppressed. These results indicate that NO-delayed senescence might relative to Ca2+ and CaM, at least partly.
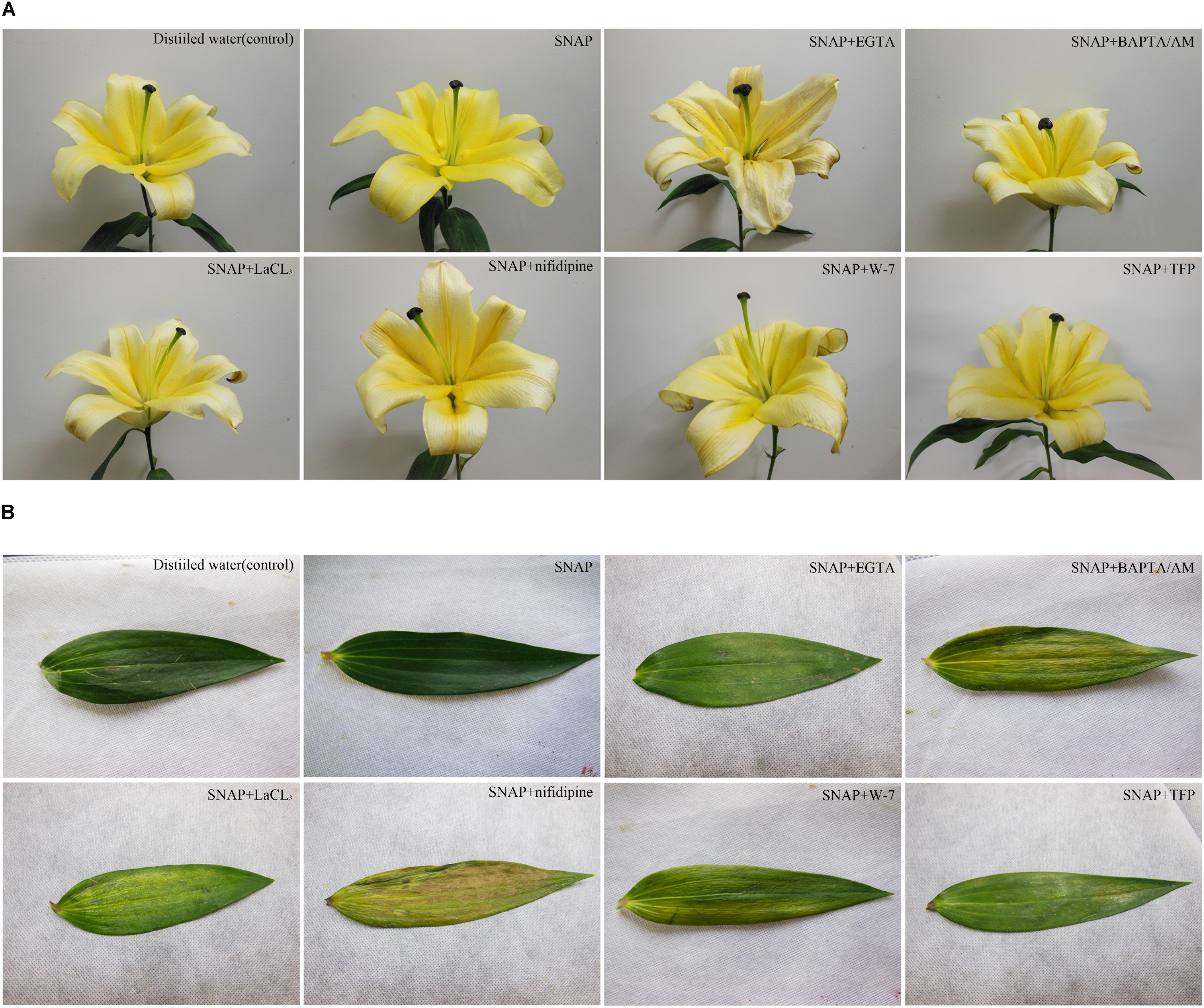
FIGURE 1. Effects of different vase solutions on lilies. Photographs of flowers (A) and leaves (B) were taken after 10 days treatments.
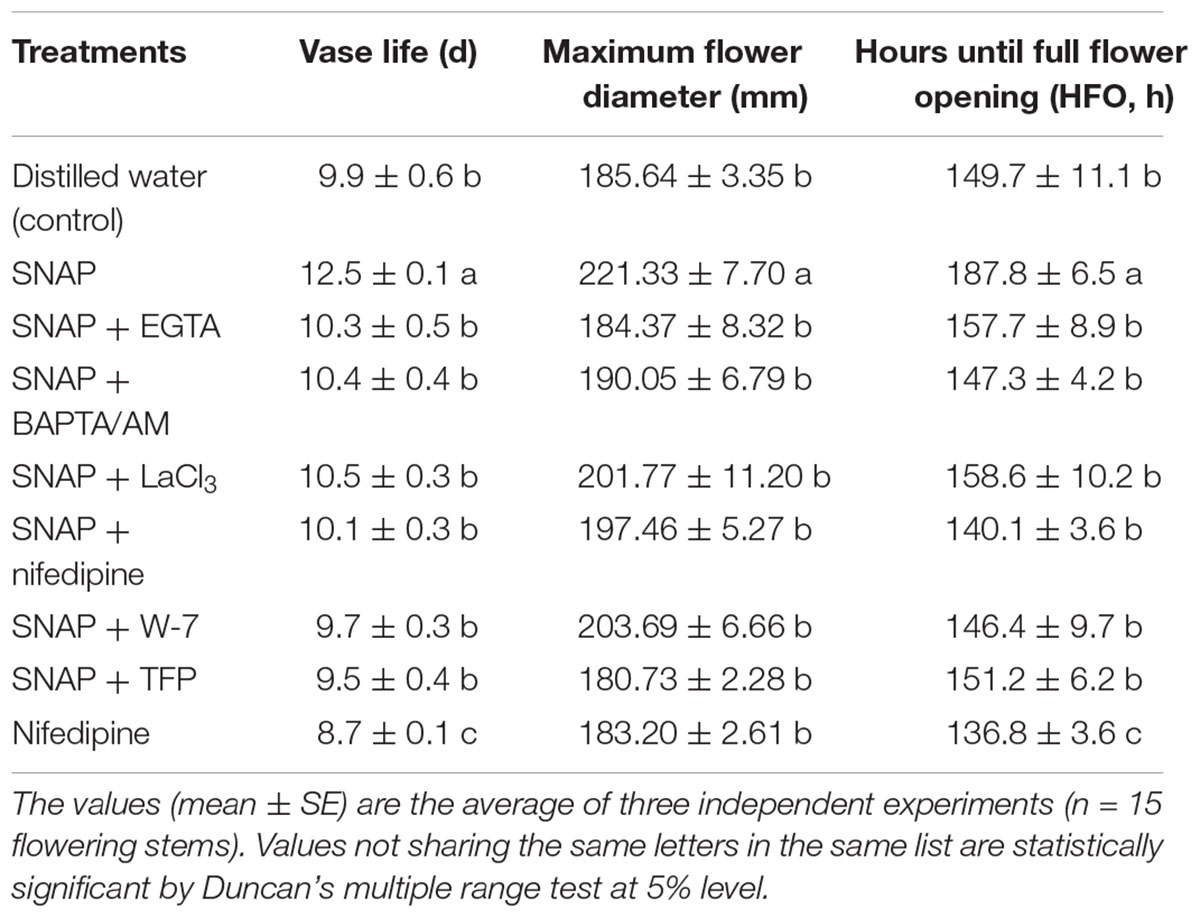
TABLE 3. Effects of Ca2+ chelators, Ca2+ channel inhibitors, and CaM antagonists on SNAP-regulated vase life, maximum flower diameter, and hours until full flower opening of cut OT hybrids lily “Manissa.”
Effects of Ca2+ Chelators, Ca2+ Channel Inhibitors, and CaM Antagonists on SNAP-Induced Calcium Level
To investigate the possible roles of Ca2+ concerned with NO-induced effect during the senescence process, the Ca2+ content in cut lily flowers of treatments with SNAP or SNAP in combination with EGTA, BAPTA/AM, LaCl3, nifedipine, W-7, and TFP were tested. Figure 2 shows that when SNAP applied alone, an obviously increase in Ca2+ level was detected, which was 144.45% of the control. When BAPTA/AM, LaCl3 or TFP was added to the SNAP-treated cut flowers, the effects of NO were weakened. However, SNAP treatment with EGTA, nifedipine or W-7 resulted in a significant reduction in Ca2+content. Placing CaM antagonists W-7 into SNAP solution maximally reversed the promotion induced by SNAP, which were 56.86% lower than that of the treatment with SNAP alone.
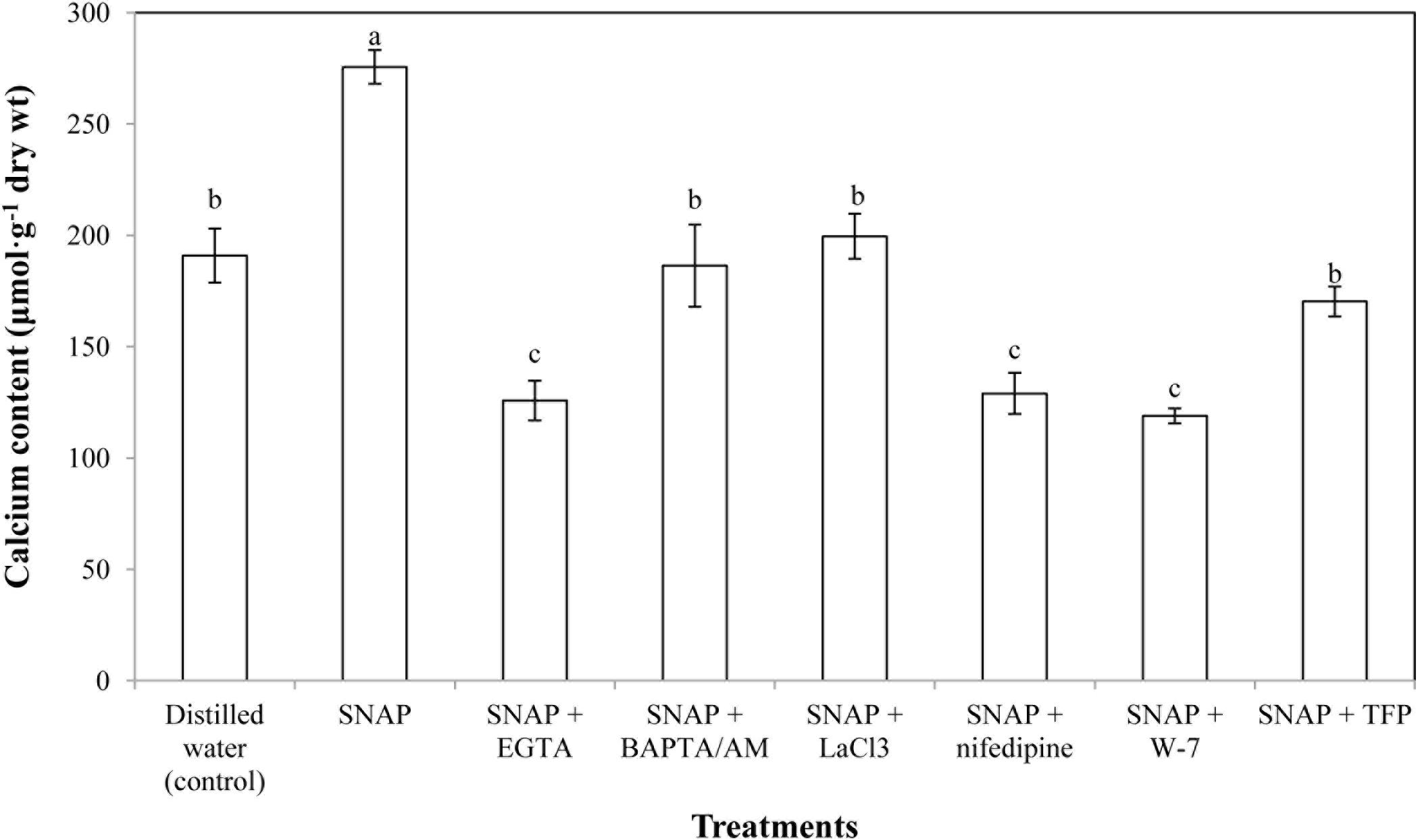
FIGURE 2. Effects of SNAP, Ca2+ channel inhibitors, Ca2+ chelators, and CaM antagonists on calcium content. SNAP, EGTA, BAPTA/AM, LaCl3, nifedipine, W-7, and TFP were used at 100 120, 30, 500, 150, 80, and 100 μM, respectively. Error bars represent standard error and each data in figure represents the mean ± SE of three experiments. Bars not sharing the same letters were significantly different by Duncan’s multiple range test (P < 0.05).
Effects of Ca2+ Chelators, Ca2+ Channel Inhibitors, and CaM Antagonists on SNAP-Induced Calmodulin Level
As shown in Figure 3, the effects of different vase solutions (SNAP was applied alone or together with Ca2+ chelators, Ca2+ channel inhibitors or CaM antagonists) on CaM levels were determined. SNAP treatment gave the maximum CaM content, which was almost twice that of the control. The SNAP-induced increase on CaM content was inhibited by BAPTA/AM, LaCl3, nifedipine, W-7, or TFP, thereby resulted in similar contents to the control. Moreover, the vase solution including both SNAP and Ca2+ chelator EGTA caused a sharp decrease in endogenous CaM content, which was obviously lower than that of the control. These results indicate that SNAP promoted the CaM content of cut lily flowers, while Ca2+ chelators, Ca2+ channel inhibitors or CaM antagonists inhibited the effects.
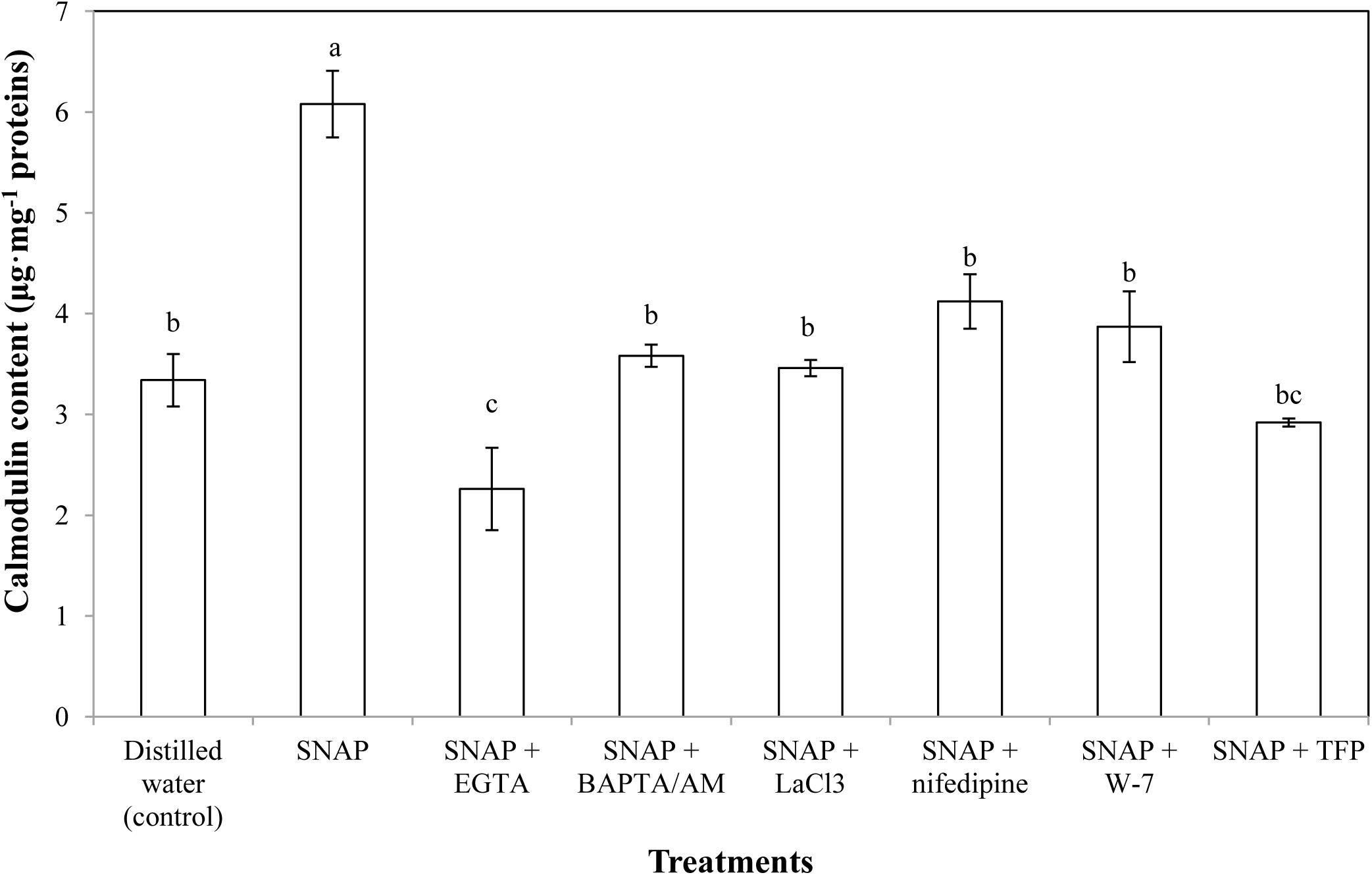
FIGURE 3. Effects of SNAP, Ca2+ channel inhibitors, Ca2+ chelators, and CaM antagonists on calmodulin content. NAP, EGTA, BAPTA/AM, LaCl3, nifedipine, W-7, and TFP were used at 100 120, 30, 500, 150, 80, and 100 μM, respectively. Error bars represent standard error and each data in figure represents the mean ± SE of three experiments. Bars not sharing the same letters were significantly different by Duncan’s multiple range test (P < 0.05).
Effects of Ca2+ Chelators, Ca2+ Channel Inhibitors, and CaM Antagonists on SNAP-Induced Ca2+-ATPase Activity
There was a significant increase in the Ca2+-ATPase activity twice more than the control when SNAP applied alone (Figure 4). Placing EGTA, LaCl3, or TFP into SNAP solution partly reversed the promotion of SNAP. SNAP treatment with BAPTA/AM, nifedipine, or W-7 also caused a decrease in Ca2+-ATPase activity. The minimum Ca2+-ATPase activity were obtained by the SNAP plus Ca2+ channel inhibitor nifedipine, suggesting that nifedipine maximally prevented the NO-induced positive effects in the senescence process of cut lilies.
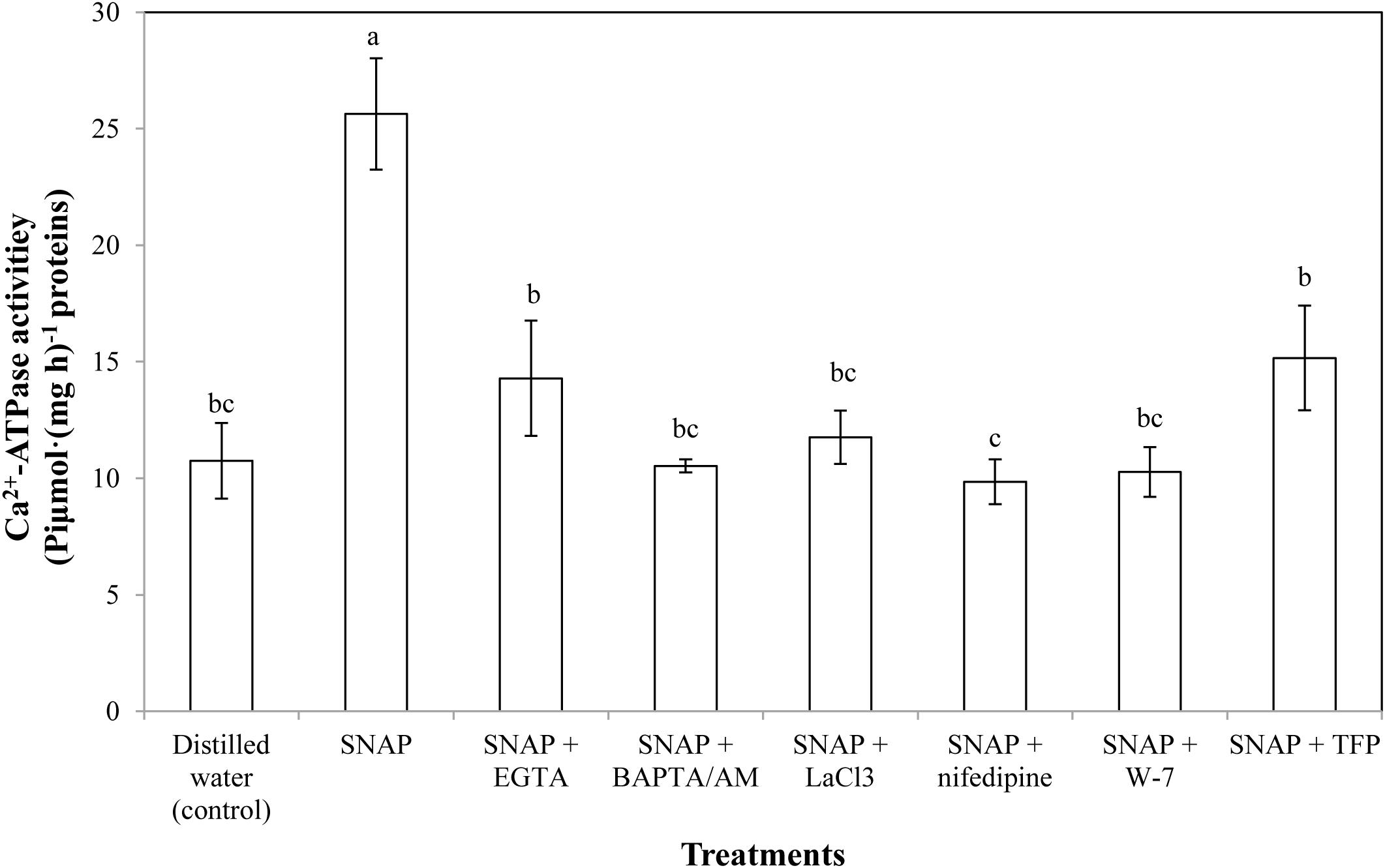
FIGURE 4. Effects of SNAP, Ca2+ channel inhibitors, Ca2+ chelators, and CaM antagonists on Ca2+-ATPase activity. NAP, EGTA, BAPTA/AM, LaCl3, nifedipine, W-7, and TFP were used at 100 120, 30, 500, 150, 80, and 100 μM, respectively. Error bars represent standard error and each data in figure represents the mean ± SE of three experiments. Bars not sharing the same letters were significantly different by Duncan’s multiple range test (P < 0.05).
Effects of Ca2+ Chelators, Ca2+ Channel Inhibitors, and CaM Antagonists on SNAP-Induced the Expression of Ca2+ Signaling-Related Genes
The gene expression of CaM was significantly increased by SNAP, resulted in a maximum value which was almost double the control; while the co-treatment with SNAP and EGTA, BAPTA/AM, LaCL3, nifedipine,W-7, or TFP obviously reduced the positive effects of SNAP (Figure 5A). There was no difference in CaM expression between the control and SNAP plus EGTA, BAPTA/AM, LaCL3, or TFP. However, SNAP treatment in combination with nifedipine or W-7 caused a significant decrease compared to the control. Placing SNAP into the vase solution also upregulated CBL1 expression significantly, whereas there was a great inhibition when SNAP applied together with EGTA, BAPTA/AM, LaCL3, nifedipine, W-7, or TFP (Figure 5B). Especially when EGTA, BAPTA/AM, LaCL3, W-7, or TFP was applied to SNAP-treated cut lily flowers, the expression of CBL1 was lower than that of the control. Similarly, EGTA, BAPTA/AM, LaCL3, nifedipine, W-7, or TFP obviously reduced the SNAP-induced increase on CBL3 expression (Figure 5C).
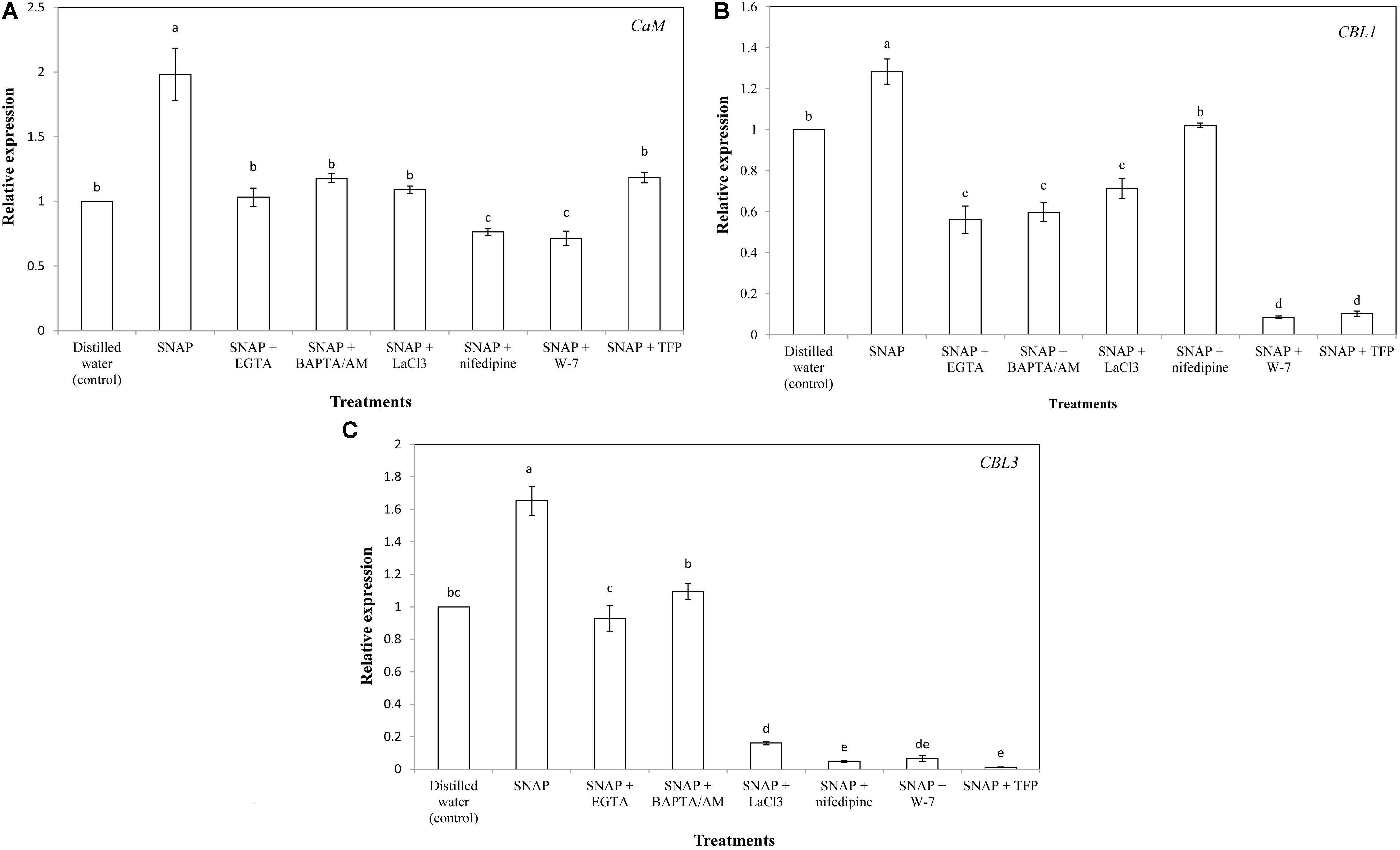
FIGURE 5. Effects of SNAP, Ca2+ channel inhibitors, Ca2+ chelators, and CaM antagonists on the expression levels of (A) CaM, (B) CBL1, and (C) CBL3. NAP, EGTA, BAPTA/AM, LaCl3, nifedipine, W-7, and TFP were used at 100 120, 30, 500, 150, 80, and 100 μM, respectively. Error bars represent standard error and each data in figure represents the mean ± SE of three experiments. Bars not sharing the same letters were significantly different by Duncan’s multiple range test (P < 0.05).
Discussion
Our results show that exogenous NO and Ca2+ at proper dose could help against cut lily flowers senescence, while high concentration of NO and Ca2+ were invalid. This conforms to our previous study, which demonstrated that the effects of NO on vase life and maximum flower diameter of cut roses were dose-dependent (Liao et al., 2013). In addition, the effects of NO on dormancy release in bulbs of oriental lily also depend on NO concentration (Niu et al., 2015). Thus, the dual role of NO as an effective antioxidant or potent oxidant is dependent on NO concentrations (Beligni and Lamattina, 1999). Exogenous NO at a relatively low dose could increase vase life of various kinds of cut flowers, such as roses (Liao et al., 2013), tuberose (Beni et al., 2013), gladiolus (Dwivedi et al., 2016), gerberas (Shabanian et al., 2018), sunflowers, lisianthus (Nazirimoghaddam et al., 2014), lilies, snapdragons, delphiniums, tulips, irises, chrysanthemums (Badiyan et al., 2004), pineapple lilies (Salachna and Byczyńska, 2017), and carnations (Naing et al., 2017). It also appears that Ca2+ plays a critical role during postharvest freshness. Exogenous Ca2+ is effective for quality preservation and disease resistance of apples (Zhao and Wang, 2015). Pre-harvest treatment with nano-calcium showed promoting effect on pepper fruits quality after plucking (Amini et al., 2016). The author also showed that Ca2+ improved total soluble solids, ascorbic acid, weight loss, electrolyte leakage, lipid peroxidation, and protein content. We used two controls (treatment with distilled water or KCl) to eliminate the influence of chloride ion and further identify the promoting effects of Ca2+ on postharvest life of cut lily flowers. The results suggests that Ca2+ enhanced vase life because KCl had no significant effects on postharvest freshness in cut lily flowers compared with the control, while CaCl2 significantly increased vase life, flower diameter and HFO. Our results also supported by the observation of authors who indicated that Ca2+ could significantly increase vase life of cut gerbera flowers by delaying stem bending (Perik et al., 2014). However, the optimum concentration of CaCl2 for longer vase life in the study of Perik et al. (2014) and our experiment are not the same. The most likely explanation for this observation is that different plant species show different sensitivities to Ca2+. Additionally, dissimilar concentration grads used in the two experiments may be another explanation.
The possibility that NO and Ca2+, as plant signaling regulators, may work together in response to abiotic stresses has been reported (Silveira et al., 2017). In the present study, when Ca2+ chelators, Ca2+ channel inhibitors, and CaM antagonists were applied, the promoting effects of NO on vase life was blocked. Meanwhile, the Ca2+ channel inhibitor nifedipine itself played negative roles in fresh-keeping by inhibiting endogenous Ca2+. According to our previous study, the Ca2+ chelators (EGTA, BAPTA/AM) and CaM antagonists (W-7, TFP) applied alone might inhibit adventitious rooting of marigold, while NO or Ca2+ promoted this process (Liao et al., 2012b). However, van Doorn et al. (2013) found that neither CaM antagonists W-7 nor TFP had an effect on visible senescence of cut iris flowers. Thus, these chemicals might have different roles in different physiological processes or in different plant species. There is, therefore, a definite need for studying the roles of these inhibitors or antagonists in plant growth and development. It should be noted that we did not investigate the effects of Ca2+ chelators and CaM antagonists on senescence alone in this study, and this should be carefully treated. Our results further show that Ca2+/CaM might be involved in the NO-regulated postharvest freshness process of cut lily flowers. The earlier reports about other plants, including cucumber (Niu et al., 2017), fava bean (Zhao et al., 2013), grape (Vandelle et al., 2006), tobacco (Lamotte et al., 2006), Arabidopsis (Abdul-Awal et al., 2016), tall fescue (Xu et al., 2016), tomato (Siddiqui et al., 2017), wheat (Tian et al., 2015), rice (Chen and Kao, 2012), and marigold (Liao et al., 2012b), are in conformity with our results, suggesting there are intricate interplays between NO and Ca2+.
Cytosolic free Ca2+ concentration ([Ca2+]cyt) variations have been reported in response to a large set of stimuli (Liao et al., 2012b). NO plays a role in elevating free [Ca2+]cyt by the mobilization of intracellular pools of Ca2+, which appears to act through cyclic guanosine monophosphate (cGMP) and cyclic adenosine diphosphoribose (cADPR; Tian et al., 2015). In the present study, about 144.45% of increase in the accumulation of Ca2+ was observed in cut lilies treated with NO, while Ca2+ chelators, Ca2+ channel inhibitors, and CaM antagonists significantly reduced Ca2+ content in NO-treated flowers. The results indicate that Ca2+ was involved in the NO-related postharvest freshness, and the positive effect of NO might depend on increased Ca2+ content (Figure 6), at least partly. Recent studies from our laboratory have shown that intracellular Ca2+ amount was increased by NO in cucumber hypocotyls during the development of adventitious roots under osmotic stress (Niu et al., 2017). These results are in full agreement with the findings of Siddiqui et al. (2017), who found that exogenous NO and Ca2+ applied individually as well as in combination significantly enhanced of Ca2+ content under both heat stress and non-stress conditions. Interestingly, exogenous NO also could promote the selectivity of uptake and transport of Ca2+, the authors also found that Ca2+ could counteract the toxic effect of exogenous NO on root. Therefore, combined application of NO and Ca2+ could compensate for each other’s disadvantages to obtain a synergic effect in alleviating the adverse effects of salt stress (Tian et al., 2015).
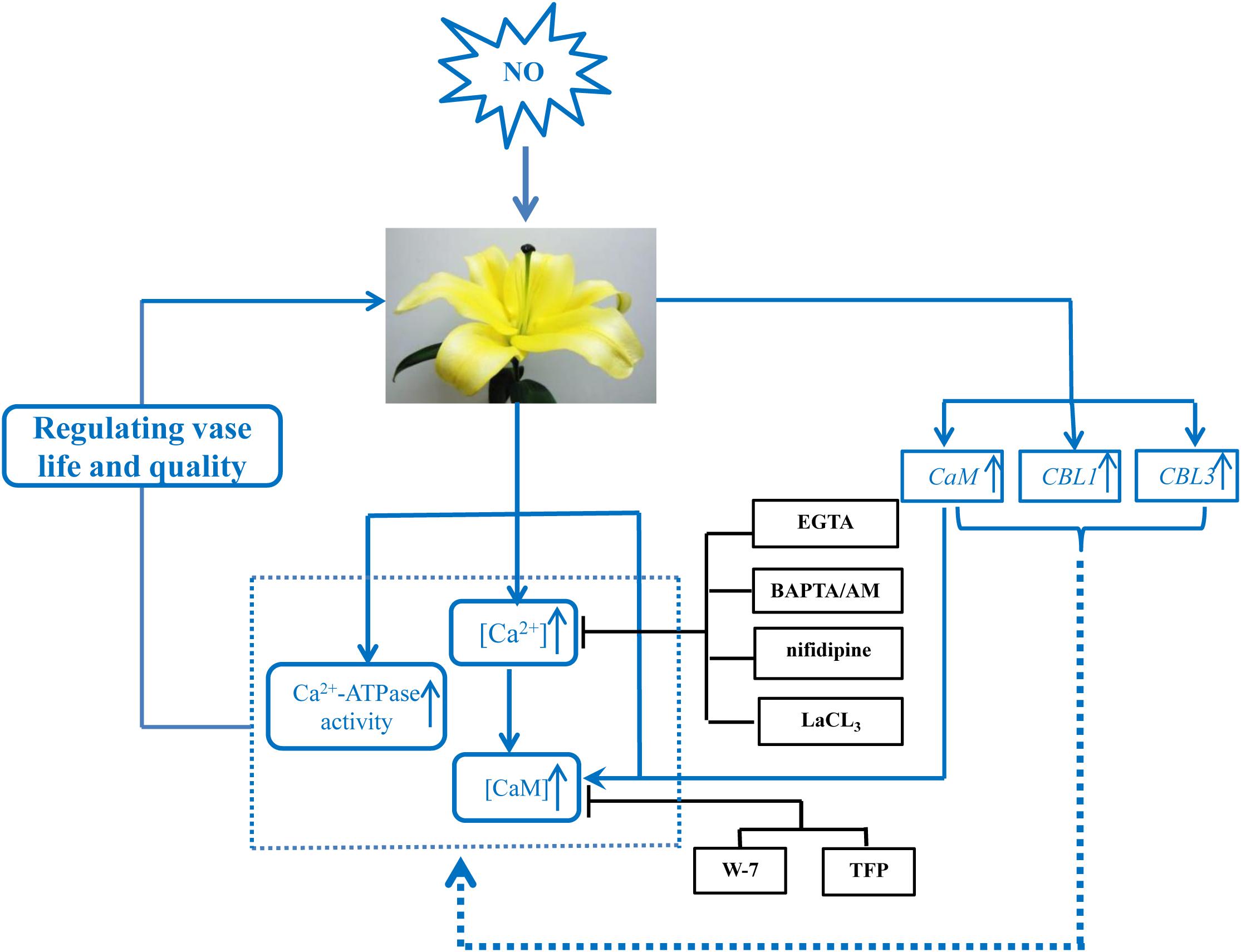
FIGURE 6. Schematic model of the signaling networks involving NO and Ca2+/CaM during postharvest freshness of cut lily flowers. NO induces the upregulation of Ca2+ signaling-regulated genes as well as the content of Ca2+/CaM and Ca2+-ATPase activity, as a consequence contributes to the increase of vase life. ↑, increase; ⊢, inhibition. All the inhibitors assayed in this study are black boxed.
To further investigate the relationships of NO and Ca2+, the CaM level was tested during senescence process of cut lily flowers. When NO applied exogenously, CaM content was almost double of the control, indicating that CaM was involved in the NO-related freshness preservation (Figure 6). This result was further confirmed by the use of Ca2+ chelators, Ca2+ channel inhibitors, and CaM antagonists, which resulted in a significant decrease in CaM content. This result is in conformity with our previous study which shows that the NO-induced CaM level was inhabited by Ca2+ chelators BAPTA or CaM antagonist W-7 (Liao et al., 2012b). Therefore, Ca2+/CaM may function as downstream signaling molecules of NO in the postharvest freshness of cut lily flowers. The evidence has shown that Ca2+/CaM might be both upstream and downstream of NO signaling cascades in both animals and plants (Weiner et al., 1994; Ali et al., 2007). Thus, different targets and/or circuitry for NO signaling must exist. Moreover, an active Ca2+ transport system, such as the Ca2+ pump (Ca2+-ATPase) on cellular membranes, is critical for maintaining Ca2+ homeostasis (Jian et al., 1999). Plant Ca2+-ATPase was also involved in biological process, such as hormonal regulation, pathogens response, and mineral nutrition/toxicity (Bonza and De Michelis, 2011). In the present study, Ca2+-ATPase activity was significantly increased when NO was applied, suggesting that Ca2+-ATPase is beneficial for NO-induced freshness preservation of cut flowers (Figure 6). A previous study shows that CaM3 expression was induced by heat and CaCl2 and correlated positively with thermotolerance of lily (Cao et al., 2013). Our results showed exogenous NO increased the expression of CaM during cut lily postharvest freshness process, while Ca2+ chelators (EGTA, BAPTA/AM), Ca2+ channel inhibitors (LaCl3, nifedipine) and CaM antagonists (W-7, TFP) inhibited the NO-induced CaM gene expression. Interestingly, neither membrane-impermeable Ca2+ chelators EGTA nor membrane-permeable Ca2+ chelators BAPTA/AM inhibited CBL3 expression as much as CBL1 expression. Probably because CBL1 and CBL3 have different sensitivity to these two Ca2+ chelators and this possibility should be further investigated. Similarly, the CBL1 and CBL3 gene expression was also upregulated by NO, and the NO-enhanced gene expression was suppressed by these chelators, antagonists, or inhibitors (Figure 6). Notably, CaM antagonists W-7 and TFP also largely inhibited CBL1 and CBL3 expression. These results further verified the study of Anil and Rao (2000), who reported that the compounds TFP and W-7 were not specific for inhibiting CDPKs. TFP and W-7 also been reported to affect the activity of other Ca2+-binding proteins such as CaM and CBLs (Anil and Rao, 2000). Thus, these results suggested that NO might help cut lily flowers against senescence by inducing the Ca2+ signaling-regulated gene expression of CaM, CBL1, and CBL3.
Conclusion
Altogether, the results of our study have shown that exogenous application of NO and Ca2+ could prolong vase life of cut flowers. NO might promote postharvest freshness by increasing Ca2+/CaM content, and regulating gene expression of Ca2+ signaling-regulated proteins. Our data also revealed that Ca2+/CaM might act downstream molecules of NO signaling pathway. However, the interaction between NO and Ca2+/CaM seems to be very complex. Therefore, more efforts are needed to demonstrate the detailed mechanism during senescence processes of cut flowers.
Author Contributions
WL designed the experiment. JZ, HF, JH, and DH preformed the experiment. JZ, HF, JH, and BW performed the data analysis. JZ wrote the manuscript. WL revised the manuscript. All the authors read and approved the final manuscript.
Funding
This research was supported by the National Natural Science Foundation of China (Grant Nos. 31860568, 31560563, and 31160398), the Research Fund of Higher Education of Gansu, China (Grant No. 2018C-14), the Post-Doctoral Foundation of China (Grant Nos. 20100470887 and 2012T50828), the Natural Science Foundation of Gansu Province, China (Grant Nos. 1606RJZA073, 1606RJZA077, and 1606RJYA252), Scientific Research Foundation for the Yong Graduate Supervisor of Gansu Agricultural University in Lanzhou, China (GAU-QNDS-201709), and Feitian and Fuxi Excellent Talents in Gansu Agricultural University.
Conflict of Interest Statement
The authors declare that the research was conducted in the absence of any commercial or financial relationships that could be construed as a potential conflict of interest.
Acknowledgments
We would like to thank Miss Carol and her husband Tich for their help in revising the manuscript.
Supplementary Material
The Supplementary Material for this article can be found online at: https://www.frontiersin.org/articles/10.3389/fpls.2018.01284/full#supplementary-material
References
Abdul-Awal, S. M., Hotta, C. T., Dodd, A. N., Davey, M. P., Smith, A. G., and Webb, A. A. (2016). NO-mediated [Ca2+]cyt increases depend on ADP-ribosyl cyclase activity in Arabidopsis. Plant Physiol. 171, 623–631. doi: 10.1104/pp.15.01965
Ali, R., Ma, W., Lemtiri-Chlieh, F., Tsaltas, D., Leng, Q., von Bodman, S., et al. (2007). Death do not have no mercy and neither does calcium: Arabidopsis cyclic nucleotide gated channel2 and innate immunity. Plant Cell 19,1081–1095. doi: 10.1105/tpc.106.045096
Amini, F., Bayat, L., and Hosseinkhani, S. (2016). Influence of preharvest nano calcium applications on postharvest of sweet pepper (Capsicum annum). Nusantara Biosci. 8, 215–220. doi: 10.13057/nusbiosci/n080213
Anil, V. S., and Rao, K. S. (2000). Calcium-mediated signaling during sandalwood somatic embryogenesis. role for exogenous calcium as second messenger. Plant Physiol. 123, 1301–1312. doi: 10.1104/pp.123.4.1301
Asgher, M., Per, T. S., Masood, A., Fatma, M., Freschi, L., Corpas, F. J., et al. (2017). Nitric oxide signaling and its crosstalk with other plant growth regulators in plant responses to abiotic stress. Environ. Sci. Pollut. Res. 24, 2273–2285. doi: 10.1007/s11356-016-7947-8
Badiyan, D., Wills, R. B. H., and Bowyer, M. C. (2004). Use of a nitric oxide donor compound to extend the vase life of cut flowers. Hort. Sci. 39, 1371–1372.
Beligni, M. V., and Lamattina, L. (1999). Nitric oxide counteracts cyto-toxic processes mediated by reactive oxygen species in plant tissues. Planta 208, 337–344. doi: 10.1007/s004250050567
Benavides, M. P., Gallego, S. M., Artuso, F. R., Checovich, M., and Galatro, A. (2016). The complexity of nitric oxide generation and function in plants. Biol. Cell. 40, 1–5.
Beni, M. A., Hatamzadeh, A., Nikbakht, A., Ghasemnezhad, M., and Zarchini, M. (2013). Improving physiological quality of cut tuberose (Polianthes tuberosa cv. Single) flowers by continues treatment with humic acid and nano-silver particles. J. Ornam. Plants 3, 133–141.
Bonza, M. C., and De Michelis, M. I. (2011). The plant Ca2+-ATPase repertoire: biochemical features and physiological functions. Plant Biol. 13, 421–430. doi: 10.1111/j.1438-8677.2010.00405.x
Boudsocq, M., and Sheen, J. (2013). CDPKs in immune and stress signaling. Trends Plant Sci. 18, 30–40. doi: 10.1016/j.tplants.2012.08.008
Bradford, M. M. (1976). A rapid and sensitive method for the quantification of microgram quantities of protein utilising the principle of protein-dye binding. Anal. Biochem. 72, 248–254. doi: 10.1016/0003-2697(76)90527-3
Cao, X., Yi, J., Wu, Z., Luo, X., Zhong, X., Wu, J., et al. (2013). Involvement of Ca2+ and CaM3 in regulation of thermotolerance in lily (Lilium longiflorum). Plant Mol. Biol. Rep. 31, 1293–1304. doi: 10.1007/s11105-013-0587-y
Chamizo-Ampudia, A., Sanz-Luque, E., Llamas, A., Galvan, A., and Fernandez, E. (2017). Nitrate reductase regulates plant nitric oxide homeostasis. Trends Plant Sci. 22, 163–174. doi: 10.1016/j.tplants.2016.12.001
Chen, Y. H., and Kao, C. H. (2012). Calcium is involved in nitric oxide- and auxin-induced lateral root formation in rice. Protoplasma 249, 187–195. doi: 10.1007/s00709-011-0277-2
Clementi, E., and Meldolesi, J. (1997). The cross-talk between nitric oxide and Ca2+: a story with a complex past and a promising future. Trends Pharmacol. Sci. 18, 4, 266–269. doi: 10.1016/j.plaphy.2017.04.011
de Almeida, D. B., Barbosa, J. G., Saraiva Grossi, J. A., Finge, F. L., and Heidemann, J. C. (2017). Influence of vernalization and bulb size on the production of lily cut flowers and lily bulbs. Semin. Cienc. Agrar. 38(4 Suppl. 1), 2399–2408. doi: 10.5433/1679-0359.2017v38n4Supl1p2399
Dwivedi, S. K., Arora, A., Singh, V. P., Sairam, R., and Bhattacharya, R. C. (2016). Effect of sodium nitroprusside on differential activity of antioxidants and expression of SAGs in relation to vase life of gladiolus cut flowers. Sci. Hortic. 210, 158–165. doi: 10.1016/j.scienta.2016.07.024
Fancy, N. N., Bahlmann, A. K., and Loake, G. J. (2016). Nitric oxide function in plant abiotic stress. Plant Cell Environ. 40, 462–472. doi: 10.1111/pce.12707
Gong, M., Du, C. K., and Xu, W. Z. (1996). Involvement of calcium and calmodulin in the regulation of drought resistance in Zea mays seedlings. Acta Botanica Boreali Occidentalia Sinica 16, 214–220.
Jeandroz, S., Lamotte, O., Astier, J., Rasul, S., Trapet, P., Besson-Bard, A., et al. (2013). There’s more to the picture than meets the eye: nitric oxide crosstalk with Ca2+ signaling. Plant Physiol. 163, 459–470. doi: 10.1104/pp.113.220624
Jian, L. C., Li, J. H., Chen, W. P., Li, P. H., and Ahlstrand, G. G. (1999). Cytochemical localization of calcium and Ca2+-ATPase activity in plant cells under chilling stress: a comparative study between the chilling-sensitive maize and the chilling-insensitive winter wheat. Plant Cell Physiol. 40, 1061–1071. doi: 10.1093/oxfordjournals.pcp.a029488
Lamotte, O., Courtois, C., Dobrowolska, G., Besson, A., Pugin, A., and Wendehenne, D. (2006). Mechanisms of nitric-oxide-induced increase of free cytosolic Ca2+ concentration in Nicotiana plumbaginifolia cells. Free Radic. Biol. Med. 40, 1369–1376. doi: 10.1016/j.freeradbiomed.2005.12.006
Liao, W. B., Huang, G. B., Yu, J. H., and Zhang, M. L. (2012a). Nitric oxide and hydrogen peroxide alleviate drought stress in marigold explants and promote its adventitious root development. Plant Physiol. Biochem. 58, 6–15. doi: 10.1016/j.plaphy.2012a.06.012
Liao, W. B., Zhang, M. L., Huang, G. B., and Yu, J. H. (2012b). Ca2+ and CaM are involved in NO- and H2O2-induced adventitious root development in marigold. J. Plant Growth Regul. 31, 253–264. doi: 10.1007/s00344-011-9235-7
Liao, W. B., Zhang, M. L., and Yu, J. H. (2013). Role of ntric oxide in delaying senescence of cut rose flowers and its interaction with ethylene. Sci. Hortic. 155, 30–38. doi: 10.1016/j.scienta.2013.03.005
Lu, T., Zhang, G., Sun, L., Wang, J., and Hao, F. (2017). Genome-wide identification of CBL family and expression analysis of CBLs in response to potassium deficiency in cotton. PeerJ 5:e3653. doi: 10.7717/peerj.3653
Luis, S., Pablo, A., Isabel, M., Inmaculada, S. V., Tamara, L.,María, F. M., et al. (2015). Nitric oxide (NO) and phytohormones crosstalk during early plant development. J. Exp. Bot. 66, 2857–2868. doi: 10.1093/jxb/erv21
Naing, A. H., Lee, K., Arun, M., Lim, K. B., and Kim, C. K. (2017). Characterization of the role of sodium nitroprusside (SNP) involved in long vase life of different carnation cultivars. BMC Plant Biol. 17:149. doi: 10.1186/s12870-017-1097-0
Nazirimoghaddam, N., Hashemabadi, D., and Kaviani, B. (2014). Improvement of vase life of cut rose, sunflower and lisianthus with sodium nitroprusside. Euro. J. Exp. Biol. 4, 162–165.
Niu, L., Li, B., Liao, W., Zhu, Y., Wang, M., Jin, X., et al. (2015). Effect of nitric oxide on dormancy release in bulbs of Oriental lily (Lilium orientalis)‘Siberia’. J. Hort. Sci. Biotech. 90, 594–598. doi: 10.1080/14620316.2015.11668720
Niu, L., Yu, J., Liao, W., Yu, J., Zhang, M., and Dawuda, M. M. (2017). Calcium and calmodulin are involved in nitric oxide-induced adventitious rooting of cucumber under simulated osmotic stress. Front. Plant Sci. 8:1864. doi: 10.3389/fpls.2017.01684
Perik, R. R., Razé, D., Ferrante, A., and van Doorn, W. G. (2014). Stem bending in cut Gerbera Perik jamesonii flowers: effects of a pulse treatment with sucrose and calcium ions. Postharvest Biol. Technol. 98, 7–13. doi: 10.1016/j.postharvbio.2014.06.008
Ranty, B., Aldon, D., Cotelle, V., Galaud, J. P., Thuleau, P., and Mazars, C. (2016).Calcium sensors as key hubs in plant responses to biotic and abiotic stresses. Front. Plant Sci. 7:327. doi: 10.3389/fpls.2016.00327
Salachna, P., and Byczyńska, A. (2017). Effect of nitric oxide and production location on vase life of cut Eucomis ‘sparkling burgundy’ flowers. World Sci. News 83, 229–234.
Shabanian, S., Esfahani, M. N., Karamian, R., and Tran, L. S. P. (2018). Physiological and biochemical modifications by postharvest treatment with sodium nitroprusside extend vase life of cut flowers of two gerbera cultivars. Postharvest Biol. Technol. 137, 1–8. doi: 10.1016/j.postharvbio.2017.11.009
Siddiqui, M., Alamri, S. A., Mutahhar, Y. Y., Al-Khaishany, M. A., Al-Qutami, H. M., and Nasir Khan, M. A. (2017). Nitric oxide and calcium induced physiobiochemical changes in tomato (Solanum Lycopersicum) plant under heat stress. Fresen. Environ. Bull. 26, 1663–1672
Silveira, N. M., Hancock, J. T., Frungillo, L., Siasou, E., Marcos, F. C., Salgado, I., et al. (2017). Evidence towards the involvement of nitric oxide in drought tolerance of sugarcane. Plant Physiol. Biochem. 115, 354–359.
Takata, T., Kimura, J., Tsuchiya, Y., Naito, Y., and Watanabe, Y. (2011). Calcium/calmodulin-dependent protein kinases as potential targets of nitric oxide. Nitric Oxide 25, 142–152. doi: 10.1016/j.niox.2011.01.004
Tian, X., He, M., Wang, Z., Zhang, J., Song, Y., He, Z., et al. (2015). Application of nitric oxide and calcium nitrate enhances tolerance of wheat seedlings to salt stress. Plant Growth Regul. 77, 343–356. doi: 10.1007/s10725-015-0069-3
Torre, S., Borochov, A., and Halevy, A. H. (1999). Calcium regulation of senescence in rose petals. Physiol. Plant. 107, 214–219. doi: 10.1034/j.1399-3054.1999.100209.x
van Doorn, W. G., Çelikel, F. G., Pak, C., and Harkema, H. (2013). Delay of Iris flower senescence by cytokinins and jasmonates. Physiol. Plant. 148, 105–120. doi: 10.1111/j.1399-3054.2012.01690.x
Vandelle, E., Poinssot, B., Wendehenne, D., Bentéjac, M., and Pugin, A. (2006). Integrated signaling network involving calcium nitric oxide, and active oxygen species but not mitogen-activated protein kinases in bcpg1-elicited grapevine defenses. Mol. Plant Microbe. In. 19, 429–440. doi: 10.1094/MPMI-19-0429
Wang, W. H., Yi, X. Q., Han, A. D., Liu, T. W., Chen, J., Wu, F. H., et al. (2012). Calcium-sensing receptor regulates stomatal closure through hydrogen peroxide and nitric oxide in response to extracellular calcium in Arabidopsis. J. Exp. Bot. 63, 177–190. doi: 10.1093/jxb/err259
Weiner, C. P., Lizasoain, I., Baylis, S. A., Knowles, R. G., Charles, I. G., and Moncada, S. (1994). Induction of calcium-dependent nitric oxide synthases by sexhor mones Proc. Natl. Acad. Sci. U.S.A. 91, 5212–5216.
Xu, Y. F., Chu, X. T., Fu, J. J., Yang, L. Y., and Hu, T. M. (2016). Crosstalk of nitric oxide with calcium induced tolerance of tall fescue leaves to high irradiance. Biol. Plant. 60, 376–384. doi: 10.1007/s10535-016-0597-3
Ye, Z. H., Guo, J. F., and Sun, D. Y. (1990). Quantitative assay of plant calmodulin by phosphodiesterase. Plant Physiol. Commun. 26, 54–58.
Zhao, X., Li, Y. Y., Xiao, H. L., Xu, C. S., and Zhang, X. (2013). Nitric oxide blocks blue light-induced k+ influx by elevating the cytosolic Ca2+ concentration in Vicia faba L. guard cells. J. Integr. Plant Biol. 55, 527–536. doi: 10.1111/jipb.12038
Zhao, Y., and Wang, C. (2015). Effect of calcium chloride in combination with salicylic acid on post-harvest freshness of apples. Food Sci. Biotechnol. 24, 1139–1146. doi: 10.1007/s10068-015-0145-5
Keywords: postharvest freshness, calcium ion/calmodulin, Ca2+-ATPase, gene expression, calcium signaling
Citation: Zhang J, Fang H, Huo J, Huang D, Wang B and Liao W (2018) Involvement of Calcium and Calmodulin in Nitric Oxide-Regulated Senescence of Cut Lily Flowers. Front. Plant Sci. 9:1284. doi: 10.3389/fpls.2018.01284
Received: 08 June 2018; Accepted: 16 August 2018;
Published: 03 September 2018.
Edited by:
Antonio Ferrante, Università degli Studi di Milano, ItalyReviewed by:
Uulke Van Meeteren, Wageningen University and Research, NetherlandsJulian C. Verdonk, Wageningen University and Research, Netherlands
Copyright © 2018 Zhang, Fang, Huo, Huang, Wang and Liao. This is an open-access article distributed under the terms of the Creative Commons Attribution License (CC BY). The use, distribution or reproduction in other forums is permitted, provided the original author(s) and the copyright owner(s) are credited and that the original publication in this journal is cited, in accordance with accepted academic practice. No use, distribution or reproduction is permitted which does not comply with these terms.
*Correspondence: Weibiao Liao, liaowb@gsau.edu.cn