- 1Núcleo de Pesquisas em Limnologia Ictiologia e Aquicultura – Nupélia, Universidade Estadual de Maringá, Maringá, Brazil
- 2Department of Wildlife and Fisheries Sciences, Texas A&M University, College Station, TX, United States
The success of invasive species depends on the overcoming of abiotic and biotic filters. Abiotic variables likely have greater relative importance over invasion at broad spatial scales, while biotic interactions are more important at fine spatial scales. In this study, we tested the hypotheses that (i) the abundance of the invasive Hydrilla verticillata is more correlated with abiotic factors than with competing native species at broad spatial grain; and that (ii) H. verticillata abundance is more correlated with competing native species than with abiotic factors at fine spatial grain. Here, we considered spatial scale as the grain size (i.e., the extent of sampling unit) assuming broad spatial scales as a large area encompassing the entire patches of macrophytes, and fine spatial scales as a small area inside one macrophyte patch. We collected the abundance of hydrilla and the competing native species along with environmental variables in a large subtropical reservoir. To evaluate how the relative importance of the abiotic factors and the competing native species vary between spatial grains we used Bayesian Generalized Linear Models. At broad grain, the abundance of the competing native species, maximum fetch (positive correlation), turbidity and conductivity (negative correlation) were the most important factors to explain the hydrilla abundance. At fine grain, alkalinity, total organic matter of the sediment and the abundance of a competitive native species (all negative correlations) were the most important variables. Our results indicate a greater importance of abiotic factors at broader grains while competitive interactions seem to be important only in the finer spatial grains. Environmental heterogeneity may explain the positive correlation between native and invasive abundances at broad grain, while the negative correlation at fine grain suggests the effect of competition. In synthesis, we show that the abiotic factors that explain the invasion success of a submerged invasive macrophyte are the same in two spatial grains, but the importance of biotic interactions changed with grain. Thus, our data suggest that models that attempt to explain the success of invasive plants, should consider spatial scales.
Introduction
The success of invasive species in their introduced range depends first on the overcoming of dispersion and establishment barriers (Davis, 2009). These processes are determined by assembly rules, which specify which species of a regional pool can potentially establish in a local pool (Keddy, 1992; Moyle and Light, 1996). Thus, multiple mechanisms are involved at different spatial scales, which are related to the invading potential of the species (traits) and to the susceptibility of invasion of native communities and ecosystems (environmental aspects) (Davis, 2009).
In order to colonize and spread successfully in new areas, invasive species have to tolerate and surpass filters as they pass through the different invasion stages. During the dispersion stage, for example, the potential invader must cross the biogeographic barriers, which occurs through human action (Rahel, 2002). Then, at the establishment stage, the physical and chemical aspects act as physiological filters regulating the invasion process (Rahel, 2002). These filters, which include in aquatic ecosystems the underwater radiation (Barko and Smart, 1981), the chemical characteristics of the sediment (Barko and Smart, 1983; Barko et al., 1991; Wang et al., 2017) and water (Vestergaard and Sand-Jensen, 2000a; Lee et al., 2004) and wave disturbances (Doyle, 2001; Strand and Weisner, 2001), are especially important in the colonization phase of many organisms (Theoharides and Dukes, 2007), especially for invasive aquatic plants. Finally, biotic interactions regulate the invasion success by acting as a biotic filter (Rahel, 2002; Theoharides and Dukes, 2007). The main mechanism associated with the biotic filter is the biotic resistance (Elton, 1958), i.e., the resistance that native species provide to the invasive species, mainly through competition (Gurevitch, 2011; Petruzzella et al., 2018) parasitism and other biotic interactions (Levine et al., 2004).
The roles of abiotic and biotic filters above mentioned vary at different spatial scales (Weiher and Keddy, 1995). The interactions between native and exotic species are considered local processes, and therefore tend to be more apparent and important at fine spatial scale (microcosms or plots of few square meters). Biotic interactions at fine spatial scales have been demonstrated both experimentally (Dukes, 2001) and in observational studies (Case, 1990). In contrast, at broad spatial scales which include sufficient heterogeneity in environmental conditions that do not reflect local processes (e.g. species interactions), the response of invaders tends to be more influenced by abiotic factors (Huston, 1999).
In addition, these environmental filters may also be altered by other impacts resulting from human action, which can make ecosystems more susceptible to invasions (Alpert et al., 2000). This scenario has been widely reported for aquatic environments, where dam construction acts as a modifier of environmental filters (Moyle and Light, 1996; Malmqvist and Rundle, 2002; Rahel, 2002; Johnson et al., 2008). For example, reservoirs alter natural conditions over wide areas, acting as stepping stones for species invasion (Moyle and Light, 1996; Havel et al., 2005; Pitelli et al., 2014). Another typical change relates to sediment trapping, what in turn increases the underwater light availability (Malmqvist and Rundle, 2002; Roberto et al., 2009) and improves habitat suitability for submerged macrophytes. In addition to changing these abiotic filters, the construction of dams can also reduce species diversity and increase instability on biotic interactions (Malmqvist and Rundle, 2002; Havel et al., 2005), decreasing the efficiency of biotic resistance. Owning to these environmental changes, reservoirs favor the establishment of invasive species, including submerged macrophytes.
Hydrilla verticillata (L.f.) Royle (Hydrocharitaceae), native to Asia (Zhu et al., 2015) is considered one of the worst aquatic invasive plants worldwide (Cook and Lüönd, 1982; Langeland, 1996; Madeira et al., 2007). It was first recorded outside its native area in 1960 in Florida, United States (Allen, 1976). From then on, H. verticillata expanded its distribution to almost all continents, with the exception of Antarctica (Cook and Lüönd, 1982). In 2005, H. verticillata was first recorded in the Paraná River basin in Brazil (Sousa, 2011). Since then, its distribution has expanded and it reached several reservoirs (Thomaz et al., 2009; Pitelli et al., 2014). The increase in the water transparency promoted by reservoirs in the Paraná River basin and the propagule pressure from the upstream reservoirs facilitated the invasion in several habitats (Thomaz et al., 2009).
Considering the potential ecological, economic and social impacts of H. verticillata and its wide global distribution (Cook and Lüönd, 1982; Langeland, 1996; Sousa, 2011), evaluating the importance of environmental and biotic factors that influence its success becomes a task that interest invasion biologists and environmental managers. In this work, we quantified the relative importance of abiotic variables (abiotic filter) and of a competing native species (biotic filter) on the performance of H. verticillata in a subtropical large reservoir. Considering the importance of spatial scales to determine the role of abiotic and biotic variables on species performance (Duarte and Kalff, 1990), we used two databases, one representing broad spatial grain (entire patches of macrophytes) and another at fine spatial grain (small area inside one macrophyte patch), to address our questions.
We tested the hypotheses that (i) H. verticillata abundance is more correlated with abiotic factors than with competing native species at broad spatial grain; and that (ii) H. verticillata abundance is more correlated with competing native species than with abiotic factors at fine spatial grain. These hypotheses are based on the assumption that abiotic variables tend to be more important for invader establishment in aquatic environments (Moyle and Light, 1996) and that abiotic variables are more important at broad spatial grains while interspecific interactions (biotic filters) are more important at fine spatial grains (Huston, 1999; Fridley et al., 2007). Thus, we expect that the native species Egeria najas Planchon (Hydrocharitaceae), which is a strong and potential H. verticillata competitor, may hamper the invasive species success at the fine grains. We chose this native species because it belongs to the same family of H. verticillata and they are morphologically similar (Sousa, 2011), which may enhance their interactions. Egeria najas is also the most important submerged species in terms of occurrence and abundance at the Itaipu Reservoir (S.M. Thomaz, unpublished), what makes it potentially the most important competitor. In addition, the literature shows that a single competitor plant, as the submerged macrophyte Vallisneria americana Michx., also of the family Hydrocharitaceae, is capable of effectively reduce establishment of hydrilla fragments (Owens et al., 2008).
Materials and Methods
The Itaipu Reservoir (24° 05′- 25° 33′ S, 54° 00′- 54° 37′ W), located between Brazil and Paraguay, was formed by damming the Paraná River in October 1982 (Figure 1). It has an area of 1350 km2, an average depth of 22.5 m, a length of 170 km and an average width of 7 km. It has a residence time of about 40 days, being shorter in the main axis of the reservoir (about 29 days). Its hydrometric level is relatively stable (oscillations of less than 1 m per year). The seven arms evaluated in this study differ in relation to the physical and chemical characteristics (Thomaz et al., 2009), varying from oligotrophic to eutrophic conditions.
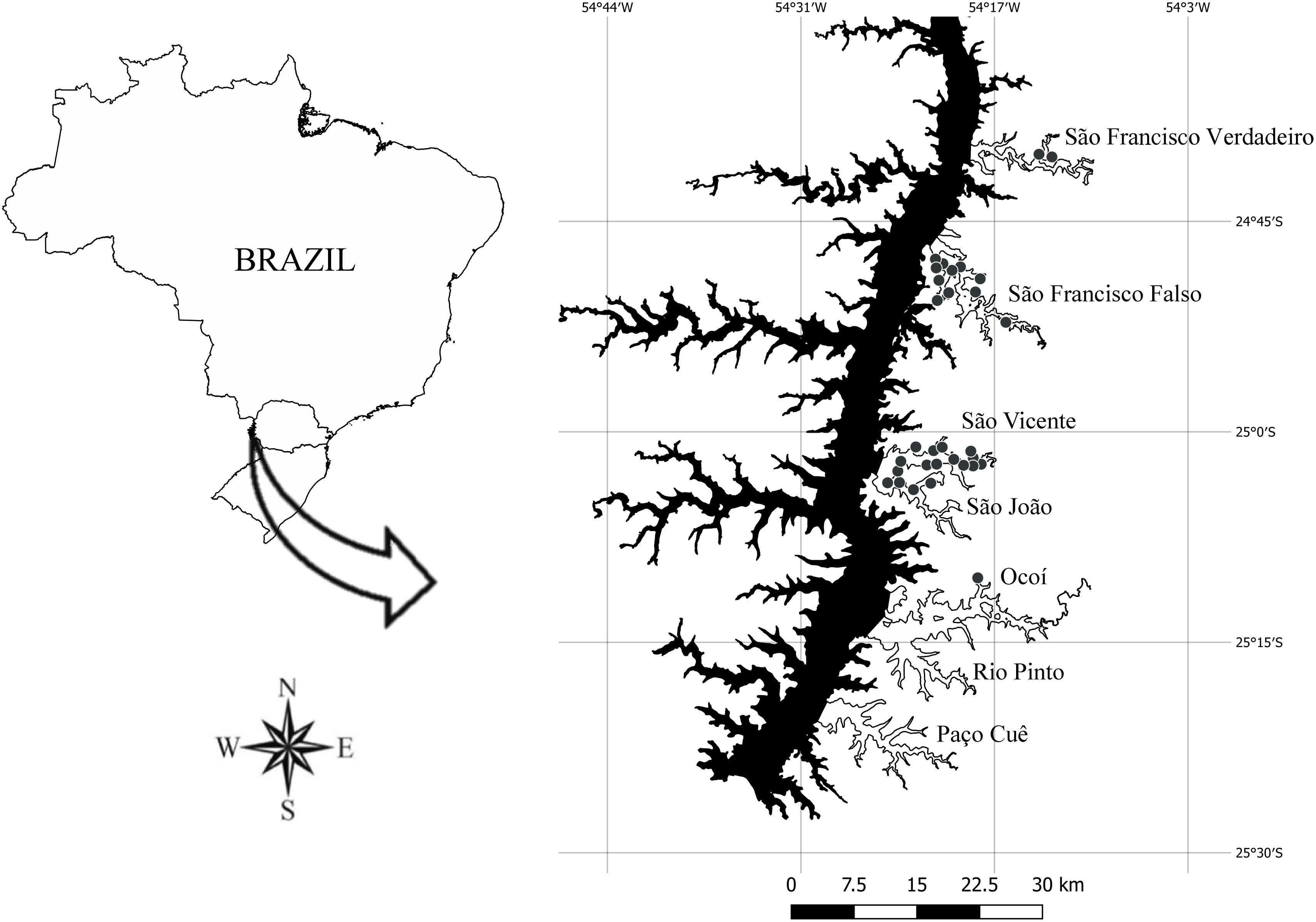
Figure 1. Map of the Itaipu Reservoir, Brazil (Geographic Coordinate System – EPSG: 4326). The sites at broad spatial grain (n = 195) are distributed in the lateral arms: São Francisco Verdadeiro (n = 28), São Francisco Falso (n = 29), São Vicente (n = 27), São João (n = 29), Ocoi (n = 27), Rio Pinto (n = 26) and Paço Cuê (n = 29). The sites at fine grain (n = 31) are represented by grey points on the lateral arms: São Francisco Verdadeiro (n = 2), São Francisco Falso (n = 11), São João (n = 4), São Vicente (n = 13) and Ocoi (n = 1). The sites sampled are located along the Brazilian margin of the reservoir.
The large margin development and the shallow areas in the arms favor the growth of aquatic macrophytes in Itaipu. In addition, damming caused the reduction of water velocity, the loss of periodicity and the amount of upstream flood pulses, and the increase of underwater radiation in the entire reservoir due to sediment deposition (Malmqvist and Rundle, 2002). These factors favored the colonization and growth of submerged aquatic macrophytes. Among the 14 submerged species recorded for the area, the most important in terms of occurrence and abundance are those belonging to the family Hydrocharitaceae. Among them, the most frequent ones are the native E. najas, found in most arms with high biomass and frequency, and the invasive H. verticillata, first recorded in 2007 in Itaipu Reservoir (Thomaz et al., 2009). Other submerged species may occur, but they are historically very rare in the reservoir. According to the monitoring program in Itaipu, E. najas and H. verticillata reached 89.7% of the total sample effort of all the submerged macrophytes (10 species in total) (authors’ personal data – data collected in 2015–2017). Given the low significance of the other submerged macrophytes as possible competitors for H. verticillata and considering their low occurrence in the entire reservoir, we selected only E. najas as an indicator of competition.
In terms of spatial scale, we particularly refer to the grain size, which is related to extent of sampling unit (sensu Wiens, 1989). We defined the grain at the broad scale as a large area encompassing the entire patches of macrophytes or even more than one patch (a transect c.a. 100 m long). At this grain, the macrophyte patches could be either monospecific or with mixed species. On the other hand, we defined the grain at the fine scale as a small area inside one macrophyte patch (a square with 0.5 m side length). Studies investigating biomass variation at small spatial scales have successfully used similar or even smaller sampled areas (Thomaz et al., 2006; Olsen et al., 2019). In addition, we used a fine spatial grain because we wanted to observe the characteristics of the microhabitat of the macrophytes, i.e., in the case of submerged macrophytes, where they are rooted. Considering that these two species are herbaceous small plants, an area of 0.25 m2 is enough to encompass even hundreds of ramets, and then is enough to represent their local abundance. Moreover, such grain extent allowed sampling sites where only a single species occurred and also where both species occurred together (where they potentially compete).
Samplings at Broad Spatial Grain
In April 2017, abundance data of H. verticillata and E. najas and environmental variables were collected at 195 geo-referenced sites (Geographic Coordinate System – EPSG: 4326) (Figure 1). These sites were distributed in seven arms of the Itaipu Reservoir (Figure 1), aiming to cover an extensive area with wide variation of environmental conditions. Among the 195 sampling points, H. verticillata and E. najas co-occurred in 82 points, H. verticillata occurred alone in 8 points and E. najas occurred alone in 70 points; in the others 35 points, none of them occurred, providing information about potential limiting environmental factors.
In order to determine the relative abundance of each species in each site, a sinuous transect were sampled parallel to the shores of the reservoir (about 80 to 100 m in length, over an area of approximately 3000 to 5000 m2). We performed these surveys from a boat at constant low speed. We carried out the samplings up to ca. 4 m depth, which is, in general, the maximum depth of occurrence of the submerged macrophytes in Itaipu (unpublished data). At each transect, we dragged 12 approximately equidistant points with a rake attached to a pipe (4.2 m). In each of these trawls, we measured the abundance rating of each species of macrophyte, which ranged from 0 (absence) to 5 (maximum biomass, with canopies reaching the surface). We estimated the relative abundance for each species of macrophyte per site by adding up all the abundance rating values measured in each transect, to obtain the additive abundance rating. Thus, macrophytes’ additive abundance rating varied potentially from 0 (absence) to 60 (12 points with abundances values of 5) (based on Yin and Kreiling, 2011). This method is highly efficient to represent the relative abundance of submerged macrophytes (Yin and Kreiling, 2011).
At each site, besides the biotic variable (additive abundance rating of E. najas), we measured four additional variables representing the abiotic conditions potentially related to the additive abundance rating of H. verticillata at broad grain (Table 1). In order to obtain the littoral slope, which is an indication of the extent of the potential area colonized by macrophytes, we used Equation 1:
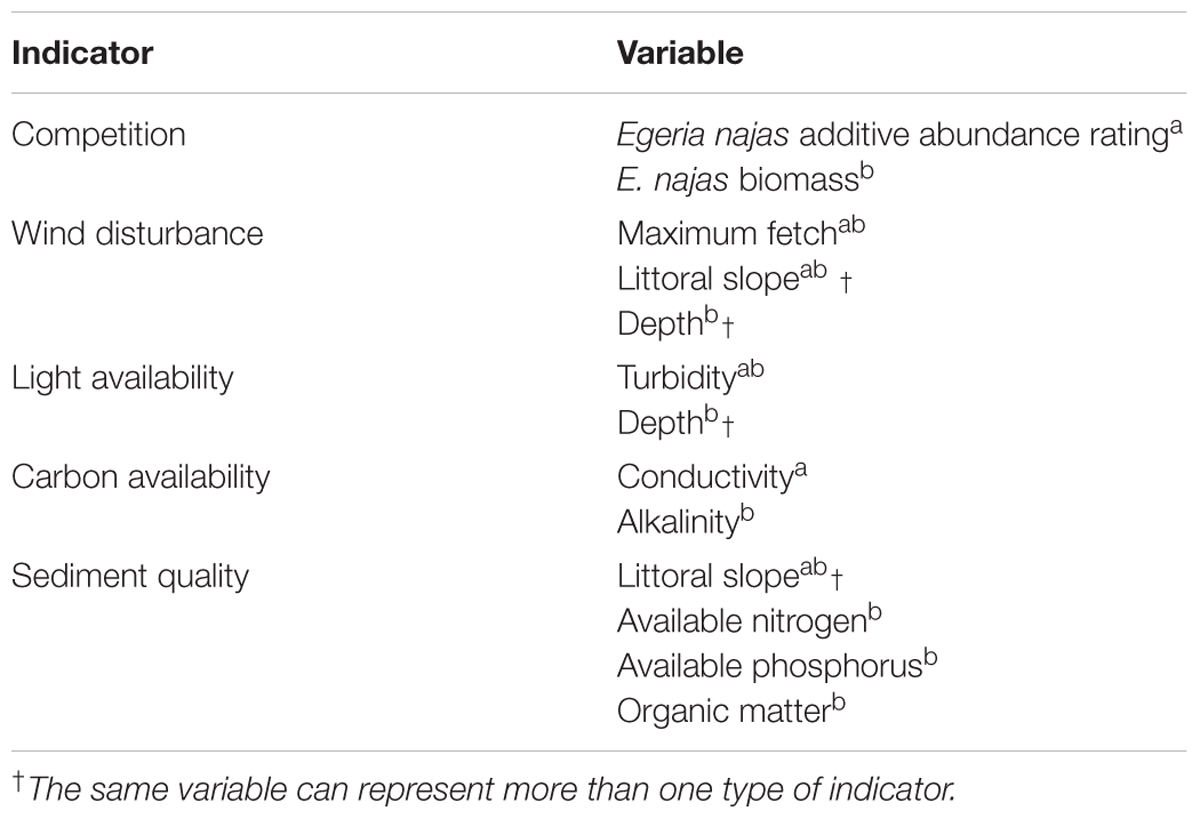
Table 1. Indicators of biotic interaction, disturbances and resource and their respective variables for broad (a) and fine (b) spatial grains.
Where: ad = altitude difference and hd = horizontal distance between the altitudes 215-220 m a.s.l. These altitudes correspond to approximate depths where macrophytes colonize the reservoir (data of this work). We obtained the altitudes used to estimate slopes, using satellite imagery.
The maximum fetch represents the maximum open water distance (without crossing the reservoir shore or islands) over which the wind is able to run in a specific direction (Håkansson, 1981; Burrows et al., 2008). Its values were obtained with ArcGIS software (version 9.1), using a reservoir map (Geographic Coordinate System – EPSG: 5880) to measure the maximum distances from the sampling site to the next shore or island. We did not correct the fetch measure for wind velocity, due to the scarcity of wind measurement stations for the large surface area of the Itaipu Reservoir. Even though, we can consider our fetch measure a surrogate of disturbances in macrophyte patches via wave action, as shown previously for the same sampling points by using the same protocol (Thomaz et al., 2003, 2009). We measured the turbidity and the conductivity with a multi-parameter HORIBA sensor. We highlight that the conductivity and alkalinity are highly and positively correlated in the Upper Paraná waters (Roberto et al., 2009) and thus, the former variable can be considered an indicator of inorganic carbon availability, in addition to total ions concentration. Both variables were measured close to macrophyte stands to minimize their effects on local limnological conditions (Carpenter and Lodge, 1986; Duarte and Kalff, 1990).
Samplings at Fine Spatial Grain
In May 2017, we obtained the relative abundance of each species in each site by measuring the biomass of the two submerged macrophyte species and the environmental variables in 31 geo-referenced sites (Geographic Coordinate System – EPSG: 4326) distributed heterogeneously in five arms of the Itaipu Reservoir (Figure 1). We were looking for a biomass gradient among patches, from the absence of plants to well-established patches of both submerged macrophyte species, and also an environmental gradient. Therefore, considering that H. verticillata is widely distributed in the whole reservoir, we rather distributed sampling sites along the environmental gradient than distributing sampling sites homogenously along the reservoir. For this, we haphazardly selected the sampling sites across the arms of the reservoir from a boat, to capture a gradient for all explanatory variables considered. In order to ensured sample independence, we maintained long distances among sampling sites (Figure 1). Among the 31 sampling points, H. verticillata and E. najas co-occurred in 25 points, while H. verticillata occurred alone in one point, E. najas occurred alone in three points, and there were only two points where neither occurred.
In 16 sampling points, we collected the biomass of each species by delimiting an area of 0.25 m2 with a steel box (1.5 m high × 0.5 m × 0.5 m – quadrat of 0.25 m2) and twisting a rake in a 360° turn. After, we collected all the remaining material inside the quadrat with the aid of the rake to remove the plants from the sediment. We carefully washed the plants in tap water to remove excess sediment and attached material and dried them in an oven at 70°C to constant weight. For these quadrats, we obtained the total biomass by adding the biomass of the rake with the biomass remaining in the box. For the other 15 sites, the biomass was obtained only with the rake (without the presence of the box), to facilitate and streamline our samplings. In this case, we predicted the values of biomass per m2 through one simple linear regression between the two biomasses obtained at the previous sampling points (from the rake and from the steel box) for each species. The data were transformed (X0.28, that corresponds to a transformation between cube and fourth root that best linearized data) for both species in order to reach the regression assumptions.
At each site, we collected eight environmental variables potentially related to the biomass of H. verticillata, representing the environmental conditions at fine spatial grain (Table 1). All the variables, except those related to sediment, slope and depth, were measured before sampling the plants, near the border of the macrophyte patches, in order to minimize the effects of the plants on the limnological conditions (Carpenter and Lodge, 1986; Duarte and Kalff, 1990). We measured the slope using Equation 1, with ad as the depth difference between two measures taken and hd as 4.6 m that represents the distance between both ends of the boat where we took the measures. We obtained slope and depth near to the quadrat. We quantified maximum fetch following the same protocol described previously at the broad grain. We quantified the turbidity with a multi-parameter HORIBA sensor. The total alkalinity (a surrogate of inorganic carbon availability) was determined through Gran titration (Carmouze, 1994). We measured both variables close to macrophyte patches by the same reasons presented above (See “Samplings at broad grain”). Sediment was collected inside the patches where plants were collected; we used a Petersen grab and frozen the samples for further analyses of available N (Bremmer, 1965) and available P (Stainton et al., 1977). The percentage of sediment organic matter (OM) was obtained by the difference of sediment weight before and after burning sediment samples in a muffle at 550°C for 4 h (Teixeira et al., 1965).
Data Analysis
In order to assess for independent contribution of environmental variables on the additive abundance rating (at broad grain) and biomass (at fine grain) of the invasive macrophyte H. verticillata, we used Bayesian Generalized Linear Models. For obtaining standardized coefficients, we scaled prior distributions of parameters using zero mean priors divided by two standard deviations. For the broad grain, as the additive abundance rating are discrete measures and showed high over-dispersion of the data, the response variable was modeled following a Negative Binomial distribution; the theta value for describing the shape of the Negative Binomial distribution was obtained by using maximum likelihood techniques. Before analysis, we transformed the explanatory variables E. najas additive abundance rating, turbidity, and maximum fetch into ln (x + 1) to linearize the relations. We used non-informative a priori distributions for estimating parameters, which means that sampling data mostly influenced the resulting a posteriori distribution.
For the fine grain, we adjusted the models using a Gamma distribution that accounts for continuous measurements and positive skewness of residual distributions. We added a small constant (0.01) to the response variables in order to deal with zero values that the Gamma distribution did not handle. Before the analysis, the explanatory variables E. najas biomass, turbidity, maximum fetch and sediment OM were transformed into ln (x + 1), to linearize the relations. Similar to previous analysis, we used non-informative a priori distributions for estimating parameters.
We employed a model selection procedure using the Akaike Information Criterion (AIC) for both spatial grains. The competing explanatory models that followed the conservative selection criterion (ΔAIC < 2) (Burnham and Anderson, 2002) were obtained from all possible subsets of models (all subsets approach). If more than three competing explanatory models were selected, we used Akaike’s weighted average model to infer the effects of the variables. To compare the relative importance between abiotic factors and the competing native species for each spatial grain, we divided the variables contained in the explanatory models selected using AIC into the abiotic group and competing native species abundance. For each of these two groups, we used the sum of the relative weights (wi) of each model containing at least one variable present in the group.
Owning to technical problems, we missed one alkalinity result and, in this case, we applied the missForest non-parametric method to insert missing data, following the protocols of (Stekhoven and Bühlmann, 2012). All analyzes were performed in the R software (R Core Team, 2017), using the MASS, car, arm, missForest, MuMIn and bbmle packages (Venables and Ripley, 2002; Fox and Weisberg, 2011; Stekhoven, 2013; Gelman and Su, 2016; Barton, 2017; Bolker and Development Core Team, 2017).
Results
Environmental Features
In general, sampling at both grains ensured a wide gradient for abundance of the competing native species E. najas (Tables 2, 3). However, this species did not reach maximum additive abundance rating (60) at any sampling point at the broad spatial grain (Table 2). In the two grains, most of the sites had low littoral slopes (< 10%) and the maximum fetch values presented great variations among sites (ca. 0.2 – 19 km), indicating a broad gradient of wind disturbance. The sampling depths, measured only at the fine grain, were also relatively low (most sites < 2m) (Table 3). Most of the sites had high water transparency, based on the observed low values of turbidity (Tables 2, 3). The conductivity and alkalinity indicated low inorganic C availability at most sites (Tables 2, 3). In the sediment samples (collected only at the fine spatial grain), the nitrogen attained lower values than phosphorus and OM covered a relatively broad gradient, although most values remained below 10% OM (Table 3).
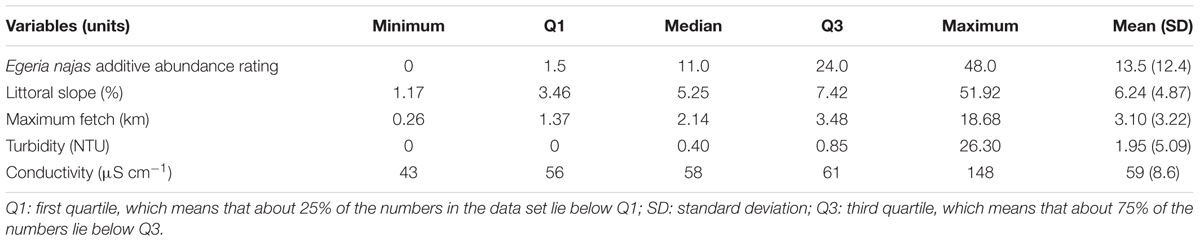
Table 2. Descriptive statistics of the abiotic variables and the competing native species collected in seven arms of the Itaipu Reservoir, at broad spatial grain.
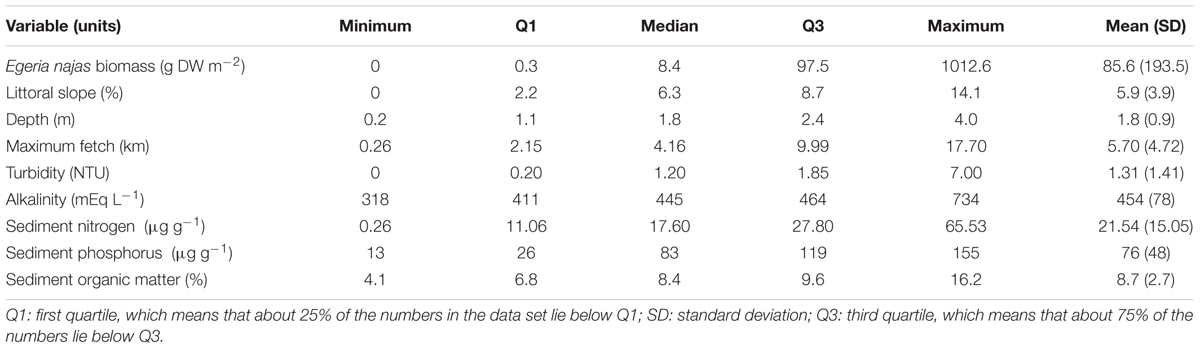
Table 3. Descriptive statistics of the abiotic variables and the competing native species collected in five arms of the Itaipu Reservoir at the fine spatial grain.
Response of H. verticillata at the Broad Spatial Grain
For the broad spatial grain, despite the low explanatory power of the competing models selected using AIC (R21 = 0.176, R22 = 0.179, R23 = 0.179), consistent statistical effects were observed for all the explanatory variables. In general, the group of abiotic variables presented greater relative importance in relation to the native competing species abundance to explain independent portions of the variability of H. verticillata abundance (Figure 2). The maximum fetch, turbidity and conductivity were the most important variables within the abiotic group of variables (Figure 2). Maximum fetch correlated positively with the additive abundance rating of the invasive macrophyte (Figure 3). In contrast, turbidity and conductivity correlated negatively with the additive abundance rating of H. verticillata (Figure 3). Only the second and third models selected the slope and the additive abundance rating of the native competitor species E. najas, respectively (Figure 3).
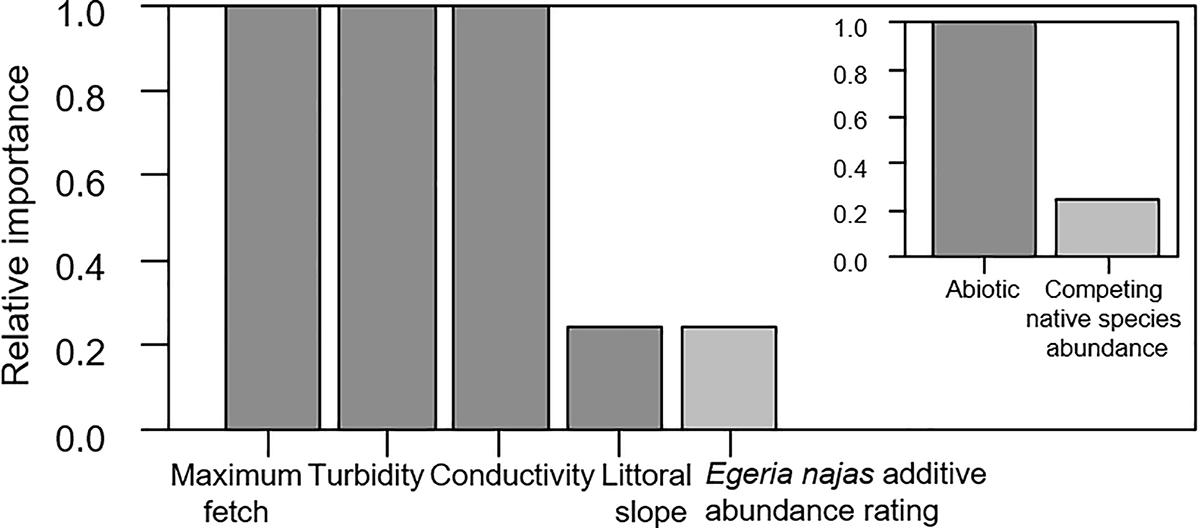
Figure 2. Relative importance of the explanatory variables and of the abiotic and the competing native species Egeria najas, based on the weighting of the explanatory models obtained to explain the variability in the additive abundance rating of Hydrilla verticillata in the Itaipu Reservoir at broad spatial grain (macrophyte patches).
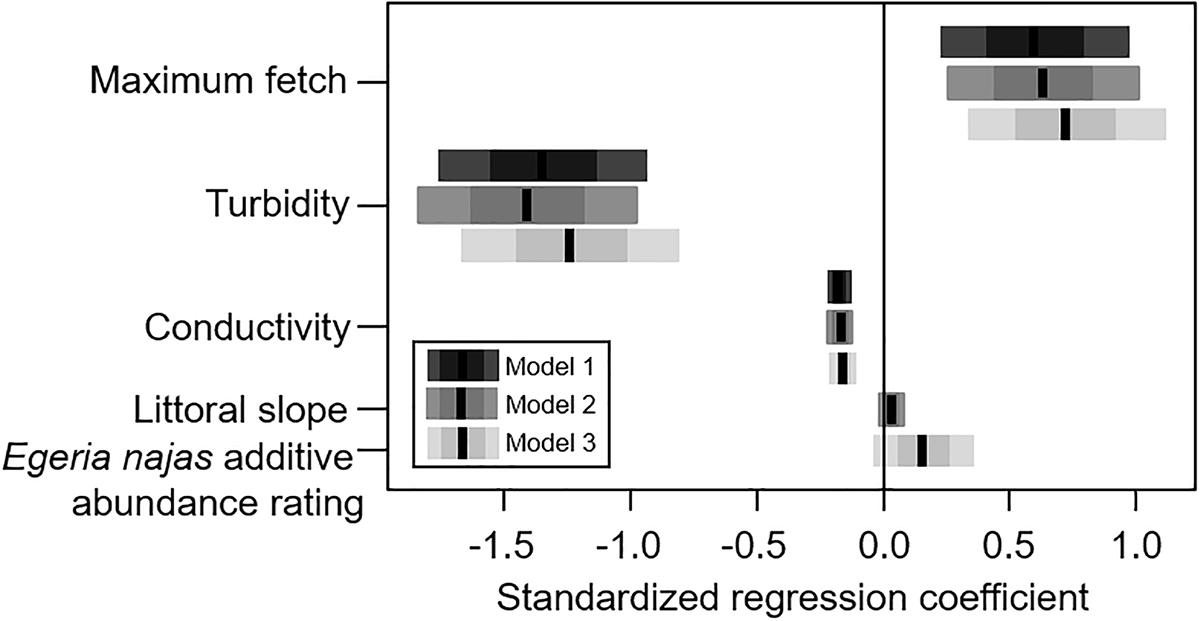
Figure 3. Competitive explanatory models obtained to explain the variability in the additive abundance rating of Hydrilla verticillata in the Itaipu Reservoir, at broad spatial grain (macrophyte patches). The black line, the darkest bars and the lightest bars correspond to the mean, the standard deviation and the confidence interval (95%), respectively, for regression coefficients of each of the three competing models.
Response of H. verticillata at the Fine Spatial Grain
For the fine spatial grain, the weighted model obtained from four selected models had a moderately low explanatory power (R-weighted = 0.38) but presented consistent statistical effects of six of the nine explanatory variables evaluated at this grain. In general, both the group of abiotic variables and the competing native species abundance were equally important to explain independent portions of the abundance variability of the invasive macrophyte H. verticillata at fine grain (Figure 4). Egeria najas biomass, alkalinity and sediment OM were the variables with the highest relative importance (Figure 4) and they correlated negatively with H. verticillata biomass (Figure 5). Alkalinity correlated negatively with H. verticillata biomass, paralleling the relation found for conductivity at the broad grain. For the sediment OM, H. verticillata tended to occur in sites with low percentage of organic matter, which apparently becomes limiting to macrophyte growth at about 10% (inspection in our graphical analysis; data not shown).
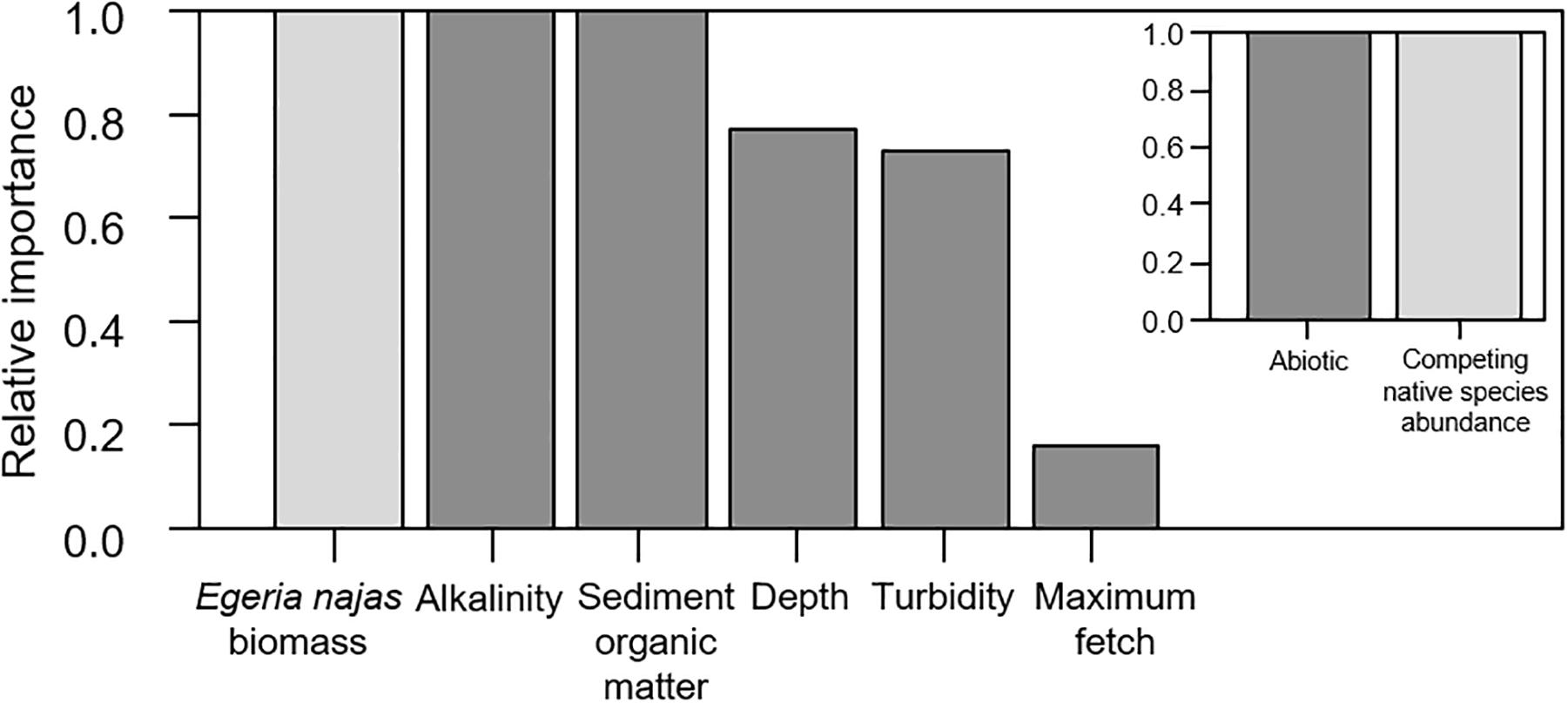
Figure 4. Relative importance of the explanatory variables and of the abiotic and competing native species Egeria najas, based on the weighting of the explanatory models obtained to explain the variability in the biomass of Hydrilla verticillata in the Itaipu reservoir, at fine spatial grain (quadrat).
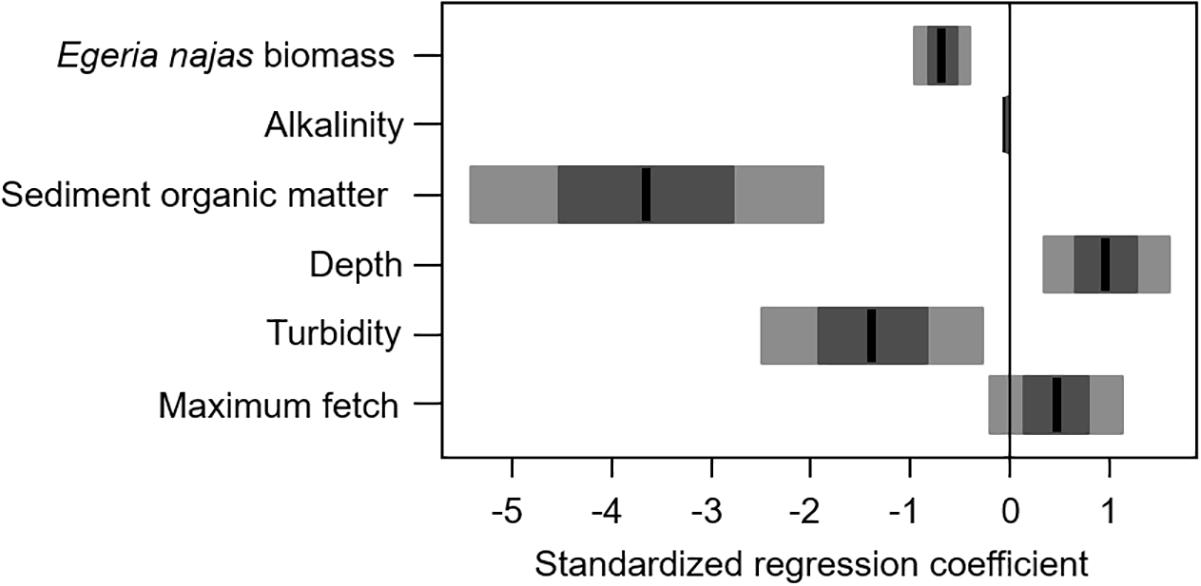
Figure 5. Weighted model for the explanatory models obtained to explain the variability in the biomass of Hydrilla verticillata in the Itaipu Reservoir, at fine spatial grain (quadrat). The black line, the darkest bars and the lightest bars correspond to the mean, standard deviation and confidence interval (95%), respectively.
The depth, turbidity, and maximum fetch were less important to explain H. verticillata biomass, although they also contributed to explaining independent portions of the invasive biomass variability at the fine spatial grain (Figure 4). The depth correlated positively with the invasive biomass (Figure 5). Both turbidity and maximum fetch paralleled the relationships found for the broad spatial grain, being negatively (the former) and positively (the latter) related with H. verticillata biomass (Figures 3, 5). However, at the fine spatial grain, both variables had less relative importance to explain H. verticillata biomass than they had at the broad spatial grain to explain H. verticillata additive abundance rating (Figures 2, 4).
Discussion
Our main findings show that at broad spatial grain (i.e., transects), abiotic variables are important to explain the invasive H. verticillata abundance, providing support for our first hypothesis. In contrast, at fine spatial grain (quadrats), our findings demonstrate that both the abiotic variables and the native competing species abundance are equally important, which rejects our second hypothesis. These results provide additional support to the idea that environmental factors are more relevant to explain invasive success at broad grain and, at a fine grain, competition may also play an important role (invasion paradox; Fridley et al., 2007).
Response of H. verticillata at the Broad Spatial Grain
Our study is consistent with others that have suggested the greater importance of abiotic factors in relation to biotic factors at broad spatial scales for the success of invasive species (e.g., Stohlgren et al., 2006; Thomaz et al., 2009). Although the definition of scale extension is somewhat subjective (in our study measured as the grain size, sensu Wiens, 1989), broad scales can be understood as those that include environmental heterogeneity high enough so that no single species is able to inhabit the entire spatial grain (Fridley et al., 2007). Indeed, in the wide grain chosen in our work (ca. 3000 to 5000 m2), both the invasive H. verticillata and the native E. najas did not occur in all the sampling points, and they showed a great variation of abundance where they occurred. It shows the importance of the abiotic factors regulating the presence and the abundance of these species in the study area. In additon, broad scales can be understood as those where many individuals can inhabit but with little direct interaction (e.g., competition) among individuals within their neighborhoods (Fridley et al., 2007). Thus, the strength of local competitive interactions decreases while the variation of environmental conditions increases with the scale (Fridley et al., 2007). Therefore, extrinsic factors, rather than biotic interactions, tend to determine the structure of communities at broad scales (Davies et al., 2005), which is consistent with our results.
In this study, H. verticillata abundance positively correlated with maximum fetch and negatively correlated with turbidity and conductivity at broad spatial grain. Although the presence and abundance of most macrophyte species tend to be negatively related to fetch, a surrogate of wind disturbance (Doyle, 2001; Thomaz et al., 2003; Azza et al., 2007), some submerged species might be positively affected by waves, when the disturbance is mild (e.g., Doyle, 2001). The positive relationship we found for the abundance of the invasive H. verticillata and fetch are in line with previous studies performed in the same study area (Thomaz et al., 2009). The successful performance of H. verticillata in sites more exposed to wind is likely related to the presence of well-developed root systems and resistance organs (tubers and turions) that allow a rapid regeneration after disturbances (Langeland, 1996; Bianchini et al., 2010; Sousa, 2011). In addition, wind disturbances can break plant stems and generate propagules, enhancing the propagule pressure. Due to the rapid regeneration of macrophyte fragments (Kuntz et al., 2014; Bickel, 2017), H. verticillata is able to rapidly colonize new sites, benefiting from dispersing capabilities enhanced by waves in places with long fetch values.
Light availability has been considered one of the main predictors of submerged macrophyte abundances (Duarte et al., 1986), including H. verticillata (Sousa et al., 2009), which explains the negative response of H. verticillata to increasing turbidity. We also found a negative relation between abundance of H. verticillata and conductivity, which can be likely explained by the effect of ion absorption (including inorganic carbon) by submerged macrophytes. In this respect, it is worth mentioning that macrophytes belonging to the elodeids group (including H. verticillata) are able to use HCO3- as a carbon source (Madsen and Sand-Jensen, 1991; Vestergaard and Sand-Jensen, 2000b) and may be capable of reducing this ion from the water column. In experiments, for example, the high photosynthetic rates of H. verticillata can reduce inorganic C to near zero (Van et al., 1976; S.M. Thomaz, unpublished data from Itaipu Reservoir). The effects of submerged macrophytes on carbon concentration might be enhanced where inorganic carbon is less available, like in Itaipu (alkalinities values usually < 500 m Eq L-1; see Table 3). Thus, even that we conducted the sampling near the macrophyte patches, the water mixture probably kept the electrical conductivity low near macrophytes, which likely explains the negative relation between H. verticillata abundance and conductivity observed here.
Although others have reported a negative correlation between the littoral slope and macrophyte biomass (Duarte and Kalff, 1986) and macrophyte diversity (Thomaz et al., 2003), we found a positive relationship between littoral slope and H. verticillata abundance. It is important to highlight that only one model selected the slope, resulting in a weaker and less consistent relation with the abundance of H. verticillata, and so, interpretations should be made with caution. The conflicting result we found here might be associated with several non-mutually exclusive potential causes. For example, the abundance of H. verticillata is likely to be smaller in sites with gentle slopes, which are more sensitive to water level fluctuations, which also negatively affects submerged macrophytes through desiccation (Beklioglu et al., 2006; Carmignani and Roy, 2017).
Response of H. verticillata at the Fine Spatial Grain
The effects of environmental heterogeneity and dispersion on invasive success are minimized at fine spatial scales where individuals interact directly (Fridley et al., 2007). Our study pointed an increase of the importance of the native E. najas competing species to explain H. verticillata abundance at the fine spatial grain. However, different from our expectations, our results also suggested an important role of abiotic factors at the fine grain. This finding is, in some degree, congruent with other studies (e.g., June-Wells et al., 2016). Species competitiveness may change according to environmental variations (Moyle and Light, 1996), highlighting the importance of both factors at fine spatial scales, as we found here.
In this study, the H. verticillata abundance correlated negatively with alkalinity and sediment OM at the fine spatial grain. The negative correlation between the invasive macrophyte abundance and alkalinity paralleled the one found between the invasive abundance and conductivity at broad spatial grain, which is not surprising. For the negative relationship found between H. verticillata and sediment OM, this outcome may reflect accumulation of phytotoxic compounds from aerobic decomposition that is commonly observed in organic sediments (Barko and Smart, 1983; Wu et al., 2009). In fact, similar patterns were previously observed in other ecosystems in the Upper Paraná River (Sousa et al., 2009).
Depth and maximum fetch correlated positively with H. verticillata abundance at the fine spatial grain, although to a lesser relative importance than found at the broad spatial grain. This species shows a particular ability to colonize deep areas (maximum 4 m in this study, 4.0-7.3 m - (Thomaz et al., 2009), where the native species cannot survive (< 2 m for E. najas; Thomaz et al., 2003). Colonizing different zones of the aquatic environment allows this invader macrophyte to avoid the high biotic resistance imposed by the higher density of native species at lower depths (Thomaz et al., 2003, 2009), reducing competition between them. In addition, the high abundance of other macrophytes in shallower areas increases the concentration of sediment OM (Godshalk and Wetzel, 1978), which should also indirectly explain why H. verticillata was favored in deeper areas. In relation to maximum fetch, the same rationale employed to explain the findings at broad spatial scale may apply at fine spatial scale. In addition, moderate wave action increases water flow and reduces the boundary layer around the leaves, enhancing CO2 uptake (Madsen et al., 2001), and reducing periphyton growth (Istvánovics et al., 2008). Both processes increase the photosynthetic rates of submerged macrophytes and probably explain why H. verticillata attains higher abundances where fetch is longer.
The negative correlation between turbidity and H. verticillata abundance was also similar to results we found at the broad spatial grain. However, the relative importance of this variable was smaller at the fine than at the broad spatial grain. The greater variability of turbidity between the different arms obtained at the broad spatial grain can explain this finding (Tables 2, 3). Thus, light availability may have been more limiting, and therefore its explanatory importance was greater at the broad spatial grain.
Spatial Grain Dependency of the Importance of Native Competitor on the Invasive Performance
The abundance of the competing native species E. najas correlated positively with the invasive H. verticillata abundance at broad spatial grain, but the abundance of both species correlated negatively at fine spatial grain. Opposing correlations between indicators of the success of invasive and native species (usually expressed by the diversity of both groups) at different spatial scales have been reported by others (Herben et al., 2004; Davies et al., 2005; Fridley et al., 2007). As the spatial scale (or grain) increases, the variation of resources among local habitats (abiotic heterogeneity), rather than the average of these resources, enhances the native and exotic cumulative diversity, favoring the co-occurrence among them (Davies et al., 2005; in this specific study, the spatial scale varied from a 1 m2 quadrat to a 23 716 m2 block in a grassland). Then, high co-occurrences between the invasive and the native species would exist at large spatial scales in case of a great spatial heterogeneity (Fridley et al., 2007), which is observed in the arms of the Itaipu Reservoir (Thomaz et al., 2003). A great environmental heterogeneity allows more species (including invasive ones) to co-occur, because there is a great variability of available resources and abiotic conditions to be explored (Davis et al., 2000; Tilman, 2004). Thus, at broad spatial scales, one expects to find positive relations between the performance of invasive species and native ones, as observed for the two species evaluated in this study.
The negative native-invasive relationship we found at fine spatial grain should be more cautiously interpreted, since the abundance of E. najas presented less influence on abundance of H. verticillata. Even though, this result may open some questions about the mechanisms involved in the interaction between both species. We can suggest that this relationship may be related to factors associated with the niche theory, considering that both species are phylogenetically close and functionally similar (Sousa, 2011). We observed that when both plants were neighbors, the abundance of H. verticillata usually decreased with rising E. najas abundance. It suggests competition between species may occur, or they differ in terms of environmental optima due to the different physiological tolerances (Fridley et al., 2007). Both observational studies (Sousa et al., 2009) and experimental studies (Thomaz et al., 2012; Silveira et al., 2016) have been describing an indication of inter-specific competition between H. verticillata and E. najas and differences between their environmental optima. However, stronger conclusions require more studies investigating mechanisms driving this relationship.
Concluding Remarks
In summary, we found that similar abiotic factors (mainly the fetch and underwater light) might play an important role regulating the success of an invasive submerged macrophyte at both large and fine spatial grains. Particularly at fine grains, other abiotic factors related to sediment fertility and stress, like organic matter, become important determinants of the invasive success. Moreover, in addition to mechanisms driven by abiotic constraints, competing interactions may relate to invasion inhibition only at these fine spatial grains. It is important to note, however, that the explanatory power of the selected models was not strong, and thus the generalizations should be considered with caution. It is worth noting also that the result obtained here are limited to restricted spatial scales in a large reservoir and, so, extrapolation to different spatial scales and other ecosystems (e.g., streams or rivers) deserves additional investigation. Despite of limitations, our major finding suggests that models that attempt to explain the success of invasive plants, should consider spatial scales. Our findings may guide future investigations addressing mechanisms that are more specific regulating invasion success by H. verticillata at different spatial scales.
Author Contributions
MP, MD-F, and ST conceived the ideas and the sampling design. MP and MD-F conducted the field samples. MP and EC conducted the statistical analysis and their interpretations. MP and ST lead the manuscript writing. All authors contributed crucially to the final version of the manuscript and agree with it.
Funding
The Coordination for the Improvement of Higher Education Personnel (CAPES), an organ of the Brazilian Government, provided MSc scholarship for MP. The Brazilian Council of Research (CNPq) provided the MD-F’s Ph.D. scholarship. The Fundação Araucária, an organization of the Parana state government (Brazil), provided the EC’s Ph.D. scholarship. ST is Productivity Researcher from the CNPq and acknowledges this agency for constant funding. The authors are grateful to Itaipu Binacional, which also funded partially this work.
Conflict of Interest Statement
The authors declare that the research was conducted in the absence of any commercial or financial relationships that could be construed as a potential conflict of interest.
The handling Editor is currently organizing a Research Topic with one of the authors ST, and confirms the absence of any other collaboration.
Acknowledgments
We thank Lucas Assumpção Lolis (Aquatic Macrophytes Laboratory) for helping with field samplings. We also acknowledge Raquel Freitas Duarte (Itaipu Binacional) for providing data about slopes at broad spatial grain.
References
Allen, G. E. (1976). “Investigations and current status of insect enemies as biological control agents of aquatic weeds,” in Aquatic Weeds in S.E. Asia, eds C. K. Varshney and J. RzSska (The Hague: Junk), 299–306.
Alpert, P., Bone, E., and Holzapfel, C. (2000). Invasiveness, invasibility and the role of environmental stress in the spread of non-native plants. Perspect. Plant Ecol. Evol. Syst. 3, 52–66. doi: 10.1078/1433-8319-00004
Azza, N., van de Koppel, J., Denny, P., and Kansiime, F. (2007). Shoreline vegetation distribution in relation to wave exposure and bay characteristics in a tropical great lake, Lake Victoria. J. Trop. Ecol. 23, 353–360. doi: 10.1017/S0266467407004117
Barko, J. W., Gunnison, D., and Carpenter, S. R. (1991). Sediment interactions with submersed macrophyte growth and community dynamics. Aquat. Bot. 41, 41–65. doi: 10.1016/0304-3770(91)90038-7
Barko, J. W., and Smart, R. M. (1981). Sediment-based nutrition of submersed macrophytes. Aquat. Bot. 10, 339–352. doi: 10.1016/0304-3770(81)90032-2
Barko, J. W., and Smart, R. M. (1983). Effects of organic matter additions to sediment on the growth of aquatic plants. J. Ecol. 71, 161–175. doi: 10.2307/2259969
Barton, K. (2017). MuMIn: Multi-Model Inference. R Package Version 1.40.0. Available at: https://CRAN.R-project.org/package=MuMIn
Beklioglu, M., Altinayar, G., and Tan, C. O. (2006). Water level control over submerged macrophyte development in five shallow lakes of Mediterranean Turkey. Arch. Hydrobiol. 166, 535–556. doi: 10.1127/0003-9136/2006/0166-0535
Bianchini, I. Jr., Cunha-Santino, M. B., Milan, J. A. M., Rodrigues, C. J., and Dias, J. H. P. (2010). Growth of Hydrilla verticillata (L.f.) royle under controled conditions. Hydrobiologia 644, 301–312. doi: 10.1007/s10750-010-0191-1
Bickel, T. O. (2017). Processes and factors that affect regeneration and establishment of the invasive aquatic plant Cabomba caroliniana. Hydrobiologia 788, 157–168. doi: 10.1007/s10750-016-2995-0
Bolker, B. R., and Development Core Team (2017). bbmle: Tools for General Maximum Likelihood Estimation. R Package Version 1.0.20. Available at: https://CRAN.R-project.org/package=bbmle
Bremmer, J. M. (1965). “Inorganic forms of nitrogen,” in Methods of Soil Analysis, ed. C. A. Block (Madison, WI: American society of Agronomy), 1179–1237.
Burnham, K. P., and Anderson, D. R. (2002). Model Selection and Multimodel Inference: A Practical Information-Theoretic Approach, 2nd Edn. New York, NY: Springer-Verlag. doi: 10.1016/j.ecolmodel.2003.11.004
Burrows, M. T., Harvey, R., and Robb, L. (2008). Wave exposure indices from digital coastlines and the prediction of rocky shore community structure. Mar. Ecol. Prog. Ser. 353, 1–12. doi: 10.3354/meps07284
Carmignani, J. R., and Roy, A. H. (2017). Ecological impacts of winter water level drawdowns on lake littoral zones: a review. Aquat. Sci. 803–824. doi: 10.1007/s00027-017-0549-9
Carmouze, J. P. (1994). O Metabolismo dos Ecossistemas Aquáticos. Fundamentos Teóricos, Métodos de Estudo e Análises Químicas. São Paulo: Edgard Blucher.
Carpenter, S. R., and Lodge, D. M. (1986). Effects of submersed macrophytes on ecosystem processes. Aquat. Bot. 26, 341–370. doi: 10.1016/0304-3770(86)90031-8
Case, T. J. (1990). Invasion resistance arises in strongy interacting species-rich model competition communities. Proc. Natl. Acad. Sci. U.S.A. 87, 9610–9614. doi: 10.1073/pnas.87.24.9610
Cook, C. D. K., and Lüönd, R. (1982). A revision of the genus Hydrilla (Hydrocharitaceae). Aquat. Bot. 13, 485–504. doi: 10.1016/0304-3770(82)90074-2
Davies, K. F., Chesson, P., Harrison, S., Inouye, B. D., Melbourne, B. A., and Rice, K. J. (2005). Spatial heterogeneity explains the scale dependence of the native-exotic diversity relationship. Ecology 86, 1602–1610. doi: 10.1890/04-1196
Davis, M. A. (2009). Invasion Biology. Oxford: Oxford University Press. doi: 10.1017/CBO9781107415324.004
Davis, M. A., Grime, J. P., and Thompson, K. (2000). Fluctuating resources in plant comunities: a general theory of invasibility. J. Ecol. 88, 528–534. doi: 10.1046/j.1365-2745.2000.00473.x
Doyle, R. D. (2001). Effects of waves on the early growth of Vallisneria americana. Freshw. Biol. 46, 389–397. doi: 10.1046/j.1365-2427.2001.00668.x
Duarte, C. M., and Kalff, J. (1986). Littoral slope as a predictor of the maximum biomass of submerged macrophyte communities. Limnol. Oceanogr. 31, 1072–1080. doi: 10.4319/lo.1986.31.5.1072
Duarte, C. M., and Kalff, J. (1990). Patterns in the submerged macrophyte biomass of lakes and the importance of the scale of analysis in the interpretation. Can. J. Fish. Aquat. Sci. 47, 357–363. doi: 10.1139/f90-037
Duarte, C. M., Kalff, J., and Peters, R. H. (1986). Patterns in biomass and cover of aquatic macrophytes in lakes. Can. J. Fish. Aquat. Sci. 43, 1900–1908. doi: 10.1139/f86-235
Dukes, J. S. (2001). Biodiversity and invasibility in grassland microcosms. Oecologia 126, 563–568. doi: 10.1007/s004420000549
Elton, C. S. (1958). The Ecology of Invasions by Animals and Plants. London: Methuen. doi: 10.1007/978-1-4899-7214-9
Fox, J., and Weisberg, S. (2011). An R Companion to Applied Regression, Second Edition. Thousand Oaks, CA: Sage. Avalilable at: http://socserv.socsci.mcmaster.ca/jfox/Books/Companion.
Fridley, J. D., Stachowicz, J. J., Naeem, S., Sax, D. F., Seabloom, E. W., Smith, M. D., et al. (2007). The invasion paradox: reconciling pattern and process in species invasions. Ecology 88, 3–17. doi: 10.1890/0012-9658(2007)88[3:TIPRPA]2.0.CO;2
Gelman, A., and Su, Y. S. (2016). arm: Data Analysis Using Regression and Multilevel/Hierarchical Models. R Package Version 1.9-3. Cambridge: Cambridge University Press. Available at: https://CRAN.R-project.org/package=arm
Godshalk, G. L., and Wetzel, R. G. (1978). Decomposition of aquatic angiosperms. II. Particulate components. Aquat. Bot. 5, 301–327. doi: 10.1016/0304-3770(78)90074-8
Gurevitch, J. (2011). “Plant competition,” in Encyclopedia of Biological Invasions2, eds D. Simberloff and M. Rejmánek (Los Angeles, CA: University of California Press), 122–125.
Håkansson, L. (1981). A Manual of Lake Morphometry. Berlin: Springer-Verlag. doi: 10.1007/978-3-642-81563-8
Havel, J. E., Lee, C. E., and Zanden, M. J. V. (2005). Do reservoirs facilitate invasions into landscapes? Bioscience 55, 518–525. doi: 10.1641/0006-3568(2005)055[0518:DRFIIL]2.0.CO;2
Herben, T., Mandák, B., Bímová, K., and Münzbergová, Z. (2004). Invasibility and species richness of a community: a neutral model and a survey of published data. Ecology 85, 3223–3233. doi: 10.1890/03-0648
Huston, M. A. (1999). Local process and regional patterns: appropiate scales for understanding variation in the diversity of plants and animals. Oikos 86, 393–401. doi: 10.2307/3546645
Istvánovics, V., Honti, M., Kovács,Á, and Osztoics, A. (2008). Distribution of submerged macrophytes along environmental gradients in large, shallow Lake Balaton (Hungary). Aquat. Bot. 88, 317–330. doi: 10.1016/j.aquabot.2007.12.008
Johnson, P. T. J., Olden, J. D., and Zanden, M. J. V. (2008). Dam invaders: impoundments facilitate biological invasions into freshwaters. Front. Ecol. Environ. 6:357–363. doi: 10.1890/070156
June-Wells, M., Gallagher, F., Hart, B., Malik, V., and Bugbee, G. (2016). The relative influences of fine and landscape scale factors on the structure of lentic plant assemblages. Lake Reserv. Manage. 32, 116–131. doi: 10.1080/10402381.2015.1136012
Keddy, P. A. (1992). Assembly and response rules: two goals for predictive community ecology. J. Veg. Sci. 3, 157–164. doi: 10.2307/3235676
Kuntz, K., Heidbu, P., and Hussner, A. (2014). Effects of water nutrients on regeneration capacity of submerged aquatic plant fragments. Ann. Limnol. – Int. J. Lim. 50, 155–162. doi: 10.1051/limn/2014008
Langeland, K. A. (1996). Hydrilla verticillata (L.F.) royle (Hydrocharitaceae), “the perfect aquatic weed.” Bot. Soc. 61, 293–304.
Lee, D. B., Lee, K. B., Kim, C. H., Kim, J. G., and Na, S. Y. (2004). Environmental assessment of water, sediment and plants in the Mankyeong River, ROK. Environ. Geochem. Health 26, 135–145. doi: 10.1023/B:EGAH.0000039576.01300.4a
Levine, J. M., Adler, P. B., and Yelenik, S. G. (2004). A meta-analysis of biotic resistance to exotic plant invasions. Ecol. Lett. 7, 975–989. doi: 10.1111/j.1461-0248.2004.00657.x
Madeira, P. T., Coetzee, J. A., Center, T. D., White, E. E., and Tipping, P. W. (2007). The origin of Hydrilla verticillata recently discovered at a South African dam. Aquat. Bot. 87, 176–180. doi: 10.1016/j.aquabot.2007.04.008
Madsen, J. D., Chambers, P. A., James, W. F., Koch, E. W., and Westlake, D. F. (2001). The interaction between water movement, sediment dynamics and submersed macrophytes. Hydrobiologia 444, 71–84. doi: 10.1023/A:1017520800568
Madsen, T. V., and Sand-Jensen, K. (1991). Photosynthetic carbon assimilation in aquatic macrophytes. Aquat. Bot. 41, 5–40. doi: 10.1016/0304-3770(91)90037-6
Malmqvist, B., and Rundle, S. (2002). Threats to the running water ecosystems of the world. Environ. Conserv. 29, 134–153. doi: 10.1017/S0376892902000097
Moyle, P. B., and Light, T. (1996). Biological invasions of fresh water: empirical rules and assembly theory. Biol. Conserv. 78, 149–161. doi: 10.1016/0006-3207(96)00024-9
Olsen, Y. S., Mattio, L., Perez, A. Z., Babcock, R. C., Thompson, D., Haywood, M. D. E., et al. (2019). Drivers of species richness and abundance of marine macrophytes on shallow tropical reefs of north-western Australia. J. Biogeogr. 46, 170–184. doi: 10.1111/jbi.13470
Owens, C. S., Smart, R. M., and Dick, G. O. (2008). Resistance of vallisneria to invasion from hydrilla fragments. J. Aquat. Plant Manag. 46, 113–116.
Petruzzella, A., Manschot, J., van Leeuwen, C. H. A., Grutters, B. M. C., and Bakker, E. S. (2018). Mechanisms of invasion resistance of aquatic plant communities. Front. Plant Sci. 9:134. doi: 10.3389/fpls.2018.00134
Pitelli, R. L. C. M., Pitelli, R. A., Rodrigues, C. J., and Dias, J. H. P. (2014). Aquatic plant community in porto primavera reservoir. Planta Daninha 32, 467–473. doi: 10.1590/S0100-83582014000300001
Rahel, F. J. (2002). Homogenization of freshwater faunas. Annu. Rev. Ecol. Syst. 33, 291–315. doi: 10.1146/annurev.ecolysis.33.010802.150429
Roberto, M. C., Santana, N. N., and Thomaz, S. M. (2009). Limnology in the Upper Paraná River floodplain: large-scale spatial and temporal patterns, and the influence of reservoirs. Braz. J. Biol. 69, 717–725. doi: 10.1590/S1519-69842009000300025
Silveira, M. J., Harthman, V. C., Michelan, T. S., and Antônio, L. (2016). Anatomical development of roots of native and non-native submerged aquatic macrophytes in different sediment types. Aquat. Bot. 133, 24–27. doi: 10.1016/j.aquabot.2016.05.006
Sousa, W. T. Z. (2011). Hydrilla verticillata (Hydrocharitaceae), a recent invader threatening Brazil’s freshwater environments: a review of the extent of the problem. Hydrobiologia 669, 1–20. doi: 10.1007/s10750-011-0696-2
Sousa, W. T. Z., Thomaz, S. M., Murphy, K. J., Silveira, M. J., and Mormul, R. P. (2009). Environmental predictors of the occurrence of exotic Hydrilla verticillata (L.f.) Royle and native Egeria najas Planch. in a sub-tropical river floodplain: the Upper River Paraná, Brazil. Hydrobiologia 632, 65–78. doi: 10.1007/s10750-009-9828-3
Stainton, M., Capel, M., and Armstrong, E. (1977). The Chemical Analysis of Freshwater. Shepherdstown, WV: Freshwater Institute
Stekhoven, D. J. (2013). missForest: Nonparametric Missing Value Imputation Using Random Forest. R Package Version 1.4. Available at: https://github.com/stekhoven/missForest
Stekhoven, D. J., and Bühlmann, P. (2012). Missforest-non-parametric missing value imputation for mixed-type data. Bioinformatics 28, 112–118. doi: 10.1093/bioinformatics/btr597
Stohlgren, T. J., Jarnevich, C., Chong, G. W., and Evangelista, P. H. (2006). Scale and plant invasions: a theory of biotic acceptance. Preslia 78, 405–426.
Strand, J. A., and Weisner, S. E. B. (2001). Morphological plastic responses to water depth and wave exposure in an aquatic plant (Myriophyllum spicatum). J. Ecol. 89, 166–175. doi: 10.1046/j.1365-2745.2001.00530.x
Teixeira, C., Tundisi, J. G., and Kutner, M. B. (1965). Plankton studies in a mangrove. II: the standing- stock and some ecological factors. Bol. Inst. Oceanogr. 24, 23–41. doi: 10.1590/S0373-55241965000100002
Theoharides, K. A., and Dukes, J. S. (2007). Plant invasion across space and time: factors affecting nonindigenous species success during four stage of invasion. New Phytol. 176, 256–273. doi: 10.1111/j.1469-8137.2007.02207.x/pdf
Thomaz, S. M., Agostinho, A. A., Gomes, L. C., Silveira, M. J., Rejmánek, M., Aslan, C. E., et al. (2012). Using space-for-time substitution and time sequence approaches in invasion ecology. Freshw. Biol. 57, 2401–2410. doi: 10.1111/fwb.12005
Thomaz, S. M., Carvalho, P., Mormul, R. P., Ferreira, F. A., Silveira, M. J., and Michelan, T. S. (2009). Temporal trends and effects of diversity on occurrence of exotic macrophytes in a large reservoir. Acta Oecol. 35, 614–620. doi: 10.1016/j.actao.2009.05.008
Thomaz, S. M., Pagioro, T. A., Bini, L. M., and Murphy, K. J. (2006). Effect of reservoir drawdown on biomass of three species of aquatic macrophytes in a large sub-tropical reservoir (Itaipu, Brazil). Hydrobiologia 570, 53–59. doi: 10.1007/s10750-006-0161-9
Thomaz, S. M., Souza, D. C., and Bini, L. M. (2003). Species richness and beta diversity of aquatic macrophytes in a large subtropical reservoir (Itaipu Reservoir, Brazil): the influence of limnology and morphometry. Hydrobiologia 505, 119–128. doi: 10.1023/B:HYDR.0000007300.78143.e1
Tilman, D. (2004). Niche tradeoffs, neutrality, and community structure: a stochastic theory of resource competition, invasion, and community assembly. Proc. Natl. Acad. Sci. U.S.A. 101, 10854–10861. doi: 10.1073/pnas.0403458101
Van, T. K., Haller, W. T., and Bowes, G. (1976). Comparison of the photosynthetic characteristics of three submersed aquatic plants. Plant Physiol. 58, 761–768. doi: 10.1104/pp.58.6.761
Venables, W. N., and Ripley, B. D. (2002). Modern Applied Statistics With S. New York, NY: Springer.
Vestergaard, O., and Sand-Jensen, K. (2000a). Alkalinity and trophic state regulate aquatic plant distribution in Danish lakes. Aquat. Bot. 67, 85–107. doi: 10.1016/S0304-3770(00)00086-3
Vestergaard, O., and Sand-Jensen, K. (2000b). Aquatic macrophyte richness in Danish lakes in relation to alkalinity, transparency, and lake area. Can. J. Fish. Aquat. Sci. 57, 2022–2031. doi: 10.1139/f00-156
Wang, T., Jiangtao, H., Gao, Y., Yu, D., and Liu, C. (2017). Disturbance, trait similarities, and trait advantages facilitate the invasion success of Alternanthera philoxeroides (Mart.). Griseb. Soil Air Water 45:1600378. doi: 10.1002/clen.201600378
Weiher, E., and Keddy, P. A. (1995). Assembly rules, null models, and trait dispersion: new questions from old patterns. Oikos 74, 159–164. doi: 10.2307/3545686
Wiens, J. A. (1989). Spatial scaling in ecology spatial scaling in ecology1. Sour. Funct. Ecol. 3, 385–397. doi: 10.2307/2389612
Wu, J., Cheng, S., Liang, W., and Wu, Z. (2009). Effects of organic-rich sediment and below-ground sulfide exposure on submerged macrophyte, Hydrilla verticillata. Bull. Environ. Contam. Toxicol. 83, 497–501. doi: 10.1007/s00128-009-9800-y
Yin, Y., and Kreiling, R. M. (2011). The evaluation of a rake method to quantify submersed vegetation in the Upper Mississippi River. Hydrobiologia 675, 187–195. doi: 10.1007/s10750-011-0817-y
Keywords: invasive species, competition, modeling statistics, standing water, fresh water
Citation: Pulzatto MM, Cunha ER, Dainez-Filho MS and Thomaz SM (2019) Association Between the Success of an Invasive Macrophyte, Environmental Variables and Abundance of a Competing Native Macrophyte. Front. Plant Sci. 10:514. doi: 10.3389/fpls.2019.00514
Received: 30 July 2018; Accepted: 03 April 2019;
Published: 25 April 2019.
Edited by:
Te Cao, Institute of Hydrobiology (CAS), ChinaReviewed by:
Manuel J. Macía, Autonomous University of Madrid, SpainFan Liu, Wuhan Botanical Garden (CAS), China
Copyright © 2019 Pulzatto, Cunha, Dainez-Filho and Thomaz. This is an open-access article distributed under the terms of the Creative Commons Attribution License (CC BY). The use, distribution or reproduction in other forums is permitted, provided the original author(s) and the copyright owner(s) are credited and that the original publication in this journal is cited, in accordance with accepted academic practice. No use, distribution or reproduction is permitted which does not comply with these terms.
*Correspondence: Sidinei Magela Thomaz, smthomaz@nupelia.uem.br