- Department of Chemistry, Wayne State University, Detroit, MI, United States
Considerable research effort has focused on the in vivo use of responsive imaging probes that change imaging properties upon reacting with oxygen because hypoxia is relevant to diagnosing, treating, and monitoring diseases. One promising class of compounds for oxygen-responsive imaging is EuII-containing complexes because the EuII/III redox couple enables imaging with multiple modalities including magnetic resonance and photoacoustic imaging. The use of EuII requires care in handling to avoid unintended oxidation during synthesis and characterization. This review describes recent advances in the field of imaging agents based on discrete EuII-containing complexes with specific focus on the synthesis, characterization, and handling of aqueous EuII-containing complexes.
Introduction
Divalent europium is a paramagnetic ion with photophysical and electrochemical properties that can be modulated with coordination chemistry (Gansow et al., 1977; Yee et al., 1980, 1983; Sabbatini et al., 1982, 1984; Antonio and Soderholm, 1996; Jiang et al., 1998; Shipley et al., 1999; Burai et al., 2000; Soderholm et al., 2002; Botta et al., 2003; Christoffers and Starynowicz, 2008; Gamage et al., 2010; Pan et al., 2011; Garcia and Allen, 2012a,b; Gál et al., 2013; Kelly et al., 2015; Kuda-Wedagedara et al., 2015; Regueiro-Figueroa et al., 2015; Allen, 2016; Ekanger et al., 2016a, 2017; Jin et al., 2016; Vanek et al., 2016; Basal et al., 2017a,b; Burnett et al., 2017; Kawasaki et al., 2017; Corbin et al., 2018; Jenks et al., 2018). Because of these tunable properties, divalent europium complexes have potential use as molecular-imaging probes that sense O2, which is important because O2 imbalances are often correlated with disease (Shweiki et al., 1992; Barnham et al., 2004; Mattson, 2004; Lin and Beal, 2006; Park et al., 2008; Facciabene et al., 2011). One promising technique to investigate oxygenation is contrast-enhanced magnetic resonance imaging (MRI) with EuII-containing complexes. Divalent europium was proposed as a pO2 sensor for MRI (Burai et al., 2000) because divalent europium acts as a T1-shortening contrast agent for MRI until it is oxidized to EuIII, which shows no measurable T1-shortening ability at imaging-relevant concentrations and field strengths (≤6 mM) (Ekanger et al., 2016a; Basal et al., 2017b). Recently, the first example of in vivo imaging using a EuII-containing contrast agent was reported (Ekanger et al., 2015), and other in vivo examples followed (Ekanger et al., 2016b; Funk et al., 2016; Basal et al., 2017a). The recent advancement of EuII-containing complexes as O2 sensors for molecular imaging and the unique experimental challenges of characterizing and handling air-sensitive EuII-containing complexes inspired this review. Unlike GdIII, which is a commonly studied ion for MRI, EuII is air-sensitive and requires careful handling techniques because EuII oxidizes to EuIII when exposed to O2. Many researchers who might be interested in EuII likely have experience with GdIII. However, because of the crucial differences in preparation and handling between EuII and GdIII, this review focuses on the synthesis, handling, and characterization of identity and purity of EuII-containing complexes relevant to molecular imaging (Figure 1). For measurements of molecular-imaging-relevant properties for MRI, readers are referred elsewhere (Caravan et al., 1999; Burai et al., 2000, 2002; Tóth et al., 2001; Botta et al., 2003; Garcia et al., 2011, 2012; Garcia and Allen, 2012b; Ekanger et al., 2014, 2015, 2016a,b; Basal et al., 2017a,b; Lenora et al., 2017; Pierre et al., 2018). Because this review is focused on techniques for handling and characterizing discrete, air-sensitive EuII-containing complexes for molecular imaging, we do not describe other divalent lanthanide complexes, nanoparticles, imaging probes, or nonaqueous EuII-containing complexes. Readers are referred elsewhere for details of those subjects (Evans, 2000; Dorenbos, 2003; Bochkarev, 2004; Bottrill et al., 2006; le Masne de Chermont et al., 2007; Kuda-Wedagedara and Allen, 2014; Pierre et al., 2014; Ekanger and Allen, 2015; Angelovski, 2016; Edelmann, 2016; Wang et al., 2017).
Synthesis of EuII-Containing Complexes
In this section, we describe procedures for the preparation of EuII-containing complexes using the ligands depicted in Figure 2. These procedures are divided into two general strategies (Figure 3): (1) chemical or electrochemical reduction of EuIII-containing complexes or mixtures of EuIII salts and ligands and (2) metalation of ligands with EuII salts. Depending on the route used to generate EuII-containing complexes, different techniques are appropriate to evaluate the identity and purity of the resulting complexes. These characterization techniques and strategies for effectively handling solutions of EuII-containing complexes for analyses are described in the handling section of this article.
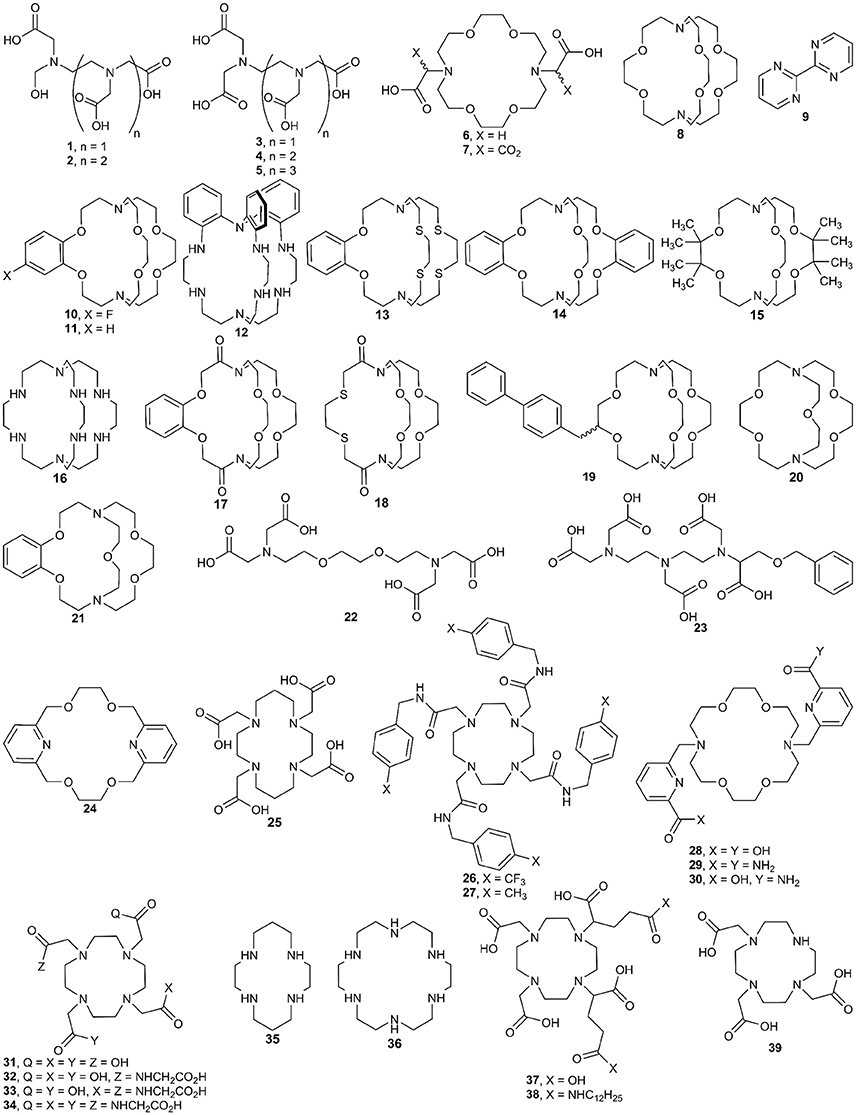
Figure 2. Ligands used with EuII and the techniques described in the synthesis section of this manuscript.
Reduction of EuIII to Produce EuII-Containing Complexes
In the process of forming EuII-containing complexes, EuIII is often reduced using electrochemical or chemical methods. Transient formation of detectable amounts of EuII-containing complexes can be achieved using cyclic voltammetry, and isolable EuII-containing complexes can be obtained through electrochemical or chemical reduction of either EuIII-containing complexes or mixtures of EuIII with ligands. The most favorable route to form a EuII-containing complex should be determined based upon the ligand and the type of analysis that is needed.
Transient Formation of EuII-Containing Complexes from Cyclic Voltammetry
To obtain information about the reduction and oxidation potentials of EuII-containing complexes, several research groups have formed EuII-containing complexes transiently using cyclic voltammetry. A description of air-free electrochemical techniques used for cyclic voltammetry or bulk electrolysis to form EuII-containing complexes is described in the handling section of this review. When reducing EuIII to form EuII, two routes are commonly taken: the corresponding EuIII-containing complexes are synthesized and purified before electrolysis, such as in the case of Eu-containing complexes of 8, 20, 21, 25, 26, 27, 31–34, and 39 (Gansow et al., 1977; Yee et al., 1980, 1983; Burai et al., 2003; Vanek et al., 2016; Basal et al., 2017a; Burnett et al., 2017). Alternatively, EuIII salts—such as Eu(OTf)3, EuCl3, or Eu(NO3)3–are dissolved in the presence of ligands, enabling the formation of complexes upon electrolysis of EuIII to EuII, such in the case of Eu-containing complexes of 1–8, 10, 11, 13–15, 20–23, 28–30, and 35–38 (Yee et al., 1980, 1983; Sabbatini et al., 1984; Burai et al., 2002, 2003; Botta et al., 2003; Gamage et al., 2010; Gál et al., 2013; Regueiro-Figueroa et al., 2015). In these experiments, cyclic voltammetry peaks that are different than the peaks for or the ligand (if the ligand is redox active in the potentials spanned by the voltammogram) are attributed to the formation of EuII/III-containing complexes (Figure 4).
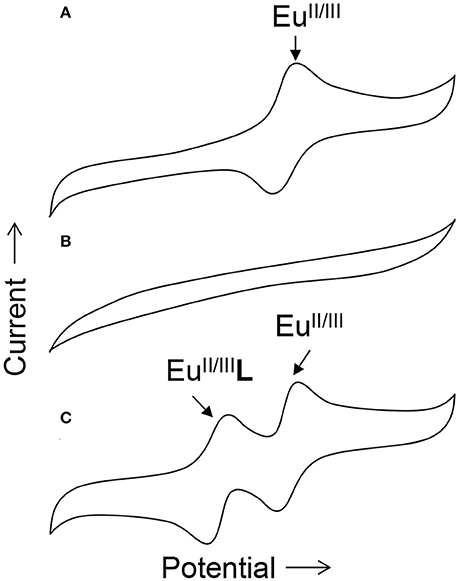
Figure 4. Cartoon cyclic voltammograms of (A) a solution of in water, (B) a solution of ligand L in water, and (C) a solution of both and L in water. The new peak in (C) relative to (A) is indicative of the in-situ formation of the Eu-containing complex EuL.
For the selection of an appropriate route, consideration of ligand structure and solubility is necessary. For example, ligands that readily form complexes with EuII upon reduction from EuIII, like cryptands 8, 20, and 21, produce the same electrochemical profiles whether starting with a mixture of EuIII and ligand or a EuII-containing complex (Yee et al., 1980, 1983). When solubility differences exist between ligands and their corresponding complexes, such as if the ligand is not soluble but the complex is, then one must ensure complexation prior to CV analysis. If the complex is insoluble in aqueous media, then organic solvents can be employed with the caveat that the measured standard electrode potential might not be reflect the potential under aqueous conditions.
Producing EuII-Containing Complexes via Electrochemical Reduction of EuIII
Beyond the transient reduction of EuIII on the surface of electrodes during cyclic voltammetry, EuIII can be reduced on an isolable scale electrochemically using bulk electrolysis in oxygen-free solvent under an atmosphere of inert gas. Reduction via bulk electrolysis involves holding a sufficiently negative potential to reduce EuIII to EuII. The electrochemical potential used to reduce a EuIII-containing complex is often 0.1–0.5 V more negative than the E1/2 of the target complex (Burai et al., 2002, 2003; Botta et al., 2003); however, the reduction potential of the ligand functional groups should be considered before selecting this technique to avoid the possibility of reducing redox-active ligands. Bulk electrolysis was used to obtain EuII-containing complexes of 1–8, 22–25, and 31 (Sabbatini et al., 1982, 1984; Burai et al., 2000, 2002; Christoffers and Starynowicz, 2008). In these studies, solutions containing both EuIII salts and ligands were held at the appropriate potential, typically in a two-compartment glass cell with a fritted glass separator with sparging of inert gas (Bard and Faulkner, 2000). The resulting EuII-containing complexes can be used for further analysis of molecular-imaging-relevant properties, including UV–visible and luminescence spectroscopy, relaxivity measurements, 17O-NMR spectroscopy, and NMRD measurements (Sabbatini et al., 1984; Burai et al., 2000, 2002; Christoffers and Starynowicz, 2008). Bulk electrolysis of a solution of metal and ligand can provide enough material to obtain crystals for X-ray diffraction: for example, bulk electrolysis of EuIII to EuII in the presence of ligand 24 followed by slow evaporation or cooling under inert atmosphere resulted in crystals of EuII 24 (Christoffers and Starynowicz, 2008). Bulk electrolysis to produce isolable EuII-containing complexes is appropriate when the potential needed to reduce a EuIII-containing complex to a EuII-containing complex does not overlap with the redox-activity of the ligand, when the desired EuII salt is unavailable, or when the standard potential or pH of the complex in solution is incompatible with chemical reductants.
Chemical Reduction of EuIII-Containing Complexes to Form EuII-Containing Complexes
In addition to bulk electrolysis, chemical reductants are used to generate EuII-containing complexes. Depending on the standard potential of the EuIII-containing complex to be reduced, different reducing agents will be appropriate. For example, the reduction potential of Zn (ZnII + 2e− → Zn0) is −0.960 V vs Ag/AgCl (saturated KCl) (Bard and Faulkner, 2000); therefore, complexes that have standard electrode potentials more positive than −0.960 V vs Ag/AgCl should, thermodynamically, be reduced by Zn0. EuIII-containing complexes were reduced using Zn0 to form EuII 26, EuII 27, and EuII 34 (Ekanger et al., 2016a; Basal et al., 2017a,b). In these studies, the EuIII-containing complexes were dissolved in water in the presence of zinc metal dust, and the pH was adjusted between 4 and 6.5 to expose Zn0, resulting in the reduction of EuIII to EuII. To date, only amalgamated Zn and Zn0 have been used to chemically reduce EuIII-containing complexes to EuII-containing complexes in water (McCoy, 1935; Ekanger et al., 2016a; Basal et al., 2017a,b). However, other chemical reductants, which have been used to reduce other LnIII ions to LnII ions (Teprovich et al., 2008; MacDonald et al., 2013; Fieser et al., 2015), could be used if the low pH required for the use of zinc metal is undesirable or if the standard electrode potential of the Eu-containing complex is more negative than that of Zn0.
Complex Formation by Direct Mixing of EuII Salts and Ligands
Another technique to synthesize EuII-containing complexes is mixing EuII halide salts with ligands. EuII chloride, bromide, and iodide salts are available commercially. When mixing EuII and ligands, often a slight excess of a EuII halide salt (1.1–1.2 equivalents) is mixed with a water-soluble ligand (1 equivalent) in water. Complexes tend to be easier to purify from an excess of EuII relative to an excess of ligand: the addition of phosphate buffer precipitates excess EuII from solution as phosphate salts that can be removed with a small (0.2 micrometer) hydrophilic filter to yield a buffered solution of EuII-containing complex (Garcia and Allen, 2012b). This technique was used to synthesize EuII-containing complexes of 8–11 and 17–19 (Zucchi et al., 2010; Garcia and Allen, 2012b; Garcia et al., 2012; Ekanger et al., 2014, 2015, 2016b, 2017; Lenora et al., 2017).
When a ligand is not water-soluble but the resulting complex is, aqueous solutions of EuII-containing complexes can be prepared by mixing EuII salts with ligands in an organic solvent and then separating the resulting complex from the organic solvent. Purification by precipitation or crystallization results in solids that are soluble in water for imaging. For an example of purification by precipitation, EuII 16 was synthesized in tetrahydrofuran: EuI2 and 16 are soluble in tetrahydrofuran, but EuII 16 is not, enabling isolation of EuII 16 by precipitation (Kuda-Wedagedara et al., 2015). For an example of purification by crystallization, crystals were grown of cryptates EuII 4, EuII 8, EuII 10, EuII 11, EuII 12, and EuII 16 from slow evaporation of a mixture of ligand and EuII halide in acetone, methanol, or methanol/tetrahydrofuran (Burai et al., 2000; Ekanger et al., 2015; Kuda-Wedagedara et al., 2015; Jin et al., 2016; Lenora et al., 2017).
Characterization for Identity and Purity in Aqueous Media
Depending the route chosen to form EuII-containing complexes, different techniques for the characterization of identity and purity of EuII-containing complexes must be used (Figure 5). Assessment of identity of EuII-containing complexes includes evidence for the oxidation state of Eu, coordination environment, and metal-to-ligand ratio. Assessment of the purity of EuII-containing complexes includes the detection of EuII or EuIII, ligand, reactants, or byproducts.
When EuII-containing complexes are generated in situ via cyclic voltammetry, purity with respect to excess can be assessed by observation of a peak for . The minimum detectable concentration of europium by cyclic voltammetry, and hence the boundary of usefulness for this technique, is influenced by multiple experimental parameters including concentration of supporting electrolyte, the identity of the buffer and solvent, and choice of reference electrode (Bard and Faulkner, 2000). With these parameters in mind, minimum detectable concentrations can be determined experimentally (Harris, 2003). Unlike EuII/III, if a ligand is not redox-active, cyclic voltammetry does not provide evidence for the presence of uncomplexed ligand. Therefore, the usefulness of cyclic voltammetry for detection of excess ligand is situationally dependent.
Regarding the identity of the complex that is formed during the course of cyclic voltammetry, formation of a Eu-containing complex can be validated by comparing the standard electrode potential of the new complex with the standard electrode potential of a sample of the Eu-containing complex (Tables 1, 2). For example, EuII-containing complexes of 8, 20, and 21 were synthesized and found to produce the same E1/2 whether starting with a mixture of EuIII and ligand or an already-synthesized EuII-containing complex (Yee et al., 1980, 1983). However, the standard electrode potential is influenced by the same experimental parameters that are listed for consideration of purity using cyclic voltammetry; therefore, care must be taken to note experimental parameters when comparing standard electrode potentials.
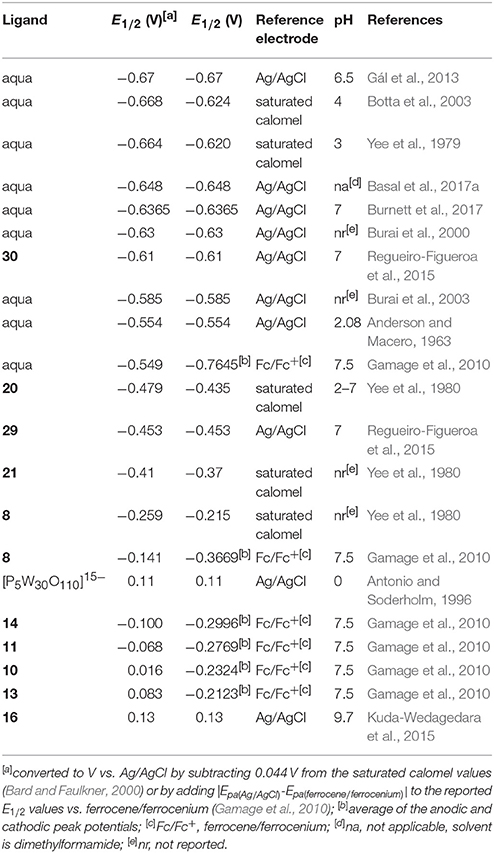
Table 2. Midpoint potentials of EuII/III-containing aqua complexes and complexes more positive than aqua ions.
In the case where EuIII-containing complexes are reduced chemically with zinc, such as 2Eu22Cl3 + Zn0 → 2Eu22Cl2 + ZnCl2, a combination of spectroscopic techniques can be used to provide evidence of the oxidation state and degree of purity. For evidence that ZnII was removed from solution post-reduction, the concentration of ZnII (down to parts-per-billion levels) can be monitored with inductively coupled plasma–mass spectrometry (Ekanger et al., 2016a). For evidence of the reduction of EuIII, a lack of overlap of the excitation bands of EuII- and EuIII-containing species enables monitoring of the presence of EuIII (down to micromolar levels) by luminescence spectroscopy when excitation is performed with a EuIII-specific wavelength (Ekanger et al., 2016a; Basal et al., 2017a). Evidence for the generation of EuII is obtained using electron paramagnetic resonance (EPR) spectroscopy. In its ground state, EuIII has no net magnetic moment (Cullity and Graham, 2009) despite having six unpaired electrons. The magnetic moment (μeff) of lanthanides is calculated using the total angular momentum (J), unlike the magnetic moments of 3dn transition metals that take into account the number of unpaired electrons (Cotton, 2006; Layfield et al., 2015). This difference is due to the quenching of orbital angular momentum by ligands for 3d orbitals but not for the shielded 4f orbitals. Therefore, the EuIII ground state would not be expected to be observed in EPR spectroscopy (Abragam and Bleaney, 1970). However, EuII is paramagnetic and characterized by a signal in EPR spectroscopy with a g factor of ~1.99 (Abragam and Bleaney, 1970; Caravan et al., 1999). Also, EuII-containing complexes can be colored yellow or orange and give rise to broad and relatively intense UV–visible absorptions and emissions that are distinct from the corresponding EuIII-containing species (Burai et al., 2003; Kuda-Wedagedara et al., 2015; Ekanger et al., 2016a). For example, a combination of spectroscopic techniques were used to monitor the formation of EuII-containing complexes Eu22, Eu26, and Eu34 (Ekanger et al., 2016a; Basal et al., 2017a).
In the case where the formation of a complex was achieved by mixing EuCl2 with ligands, evidence of 1:1 complex formation in solution was determined by measuring the change in relaxivity as a function of EuII-to-ligand ratio, a technique known as proton relaxation enhancement (Lauffer, 1987; Lenora et al., 2017). Another solution-phase technique to monitor complex formation is a Job plot where both ligand and metal ratios are varied, and a unique property of the complex, such as a complex-specific emission, is monitored (Renny et al., 2013; Kuda-Wedagedara et al., 2015). The choice of spectral feature to monitor in a Job plot is complex-dependent. Typically, EuII-based emission is largely quenched in aqueous media due to the abundance of OH oscillators (Jiang et al., 1998); therefore, luminescence spectroscopy is not suitable to characterize the formation of every EuII-containing complex. Other features to monitor as a function of metal-to-ligand ratio include complex-specific absorbance peaks, relaxivity, or cyclic voltammetry peaks.
In the case where single crystals of a EuII-containing complex are obtained, X-ray diffraction combined with elemental analysis provides information regarding identity and purity. X-ray diffraction provides information about the oxidation state and coordination environment of the EuII ion in the solid state, including bond distances and number and identity of counter ions (Zucchi et al., 2010; Ekanger et al., 2015; Kuda-Wedagedara et al., 2015; Jin et al., 2016; Basal et al., 2017a; Lenora et al., 2017). Elemental analysis provides information about elemental composition as an indication of purity. However, it is important to note that for molecular-imaging applications, solution-phase characterization is often more important than solid-phase analysis of solids because solid-state properties do not necessarily reflect solution-phase behavior or coordination environment. For example, EuII-containing complexes Eu10, Eu11, and Eu16, crystallize with one chloride counteranion bound to EuII. In the case of Eu16, solution-phase characterization suggests that the counteranion remains coordinated to EuII in solution (Kuda-Wedagedara et al., 2015); however, for Eu10 and Eu11, molar-conductivity data suggests that counteranions dissociate in solution (Ekanger et al., 2015; Lenora et al., 2017).
Handling EuII-Containing Samples to Prevent Oxidation
Preventing EuII-containing complexes from oxidizing over the course of analyses is critical for the collection of accurate data: EuIII and EuII have different properties, and misinterpretation of experimental results can occur if care is not taken to prevent unintentional oxidation of EuII. Rigorous techniques must be used in the synthesis and handling of EuII-containing complexes. This section describes apparatuses and techniques that were successfully used to study EuII-containing complexes (Figures 6, 7).
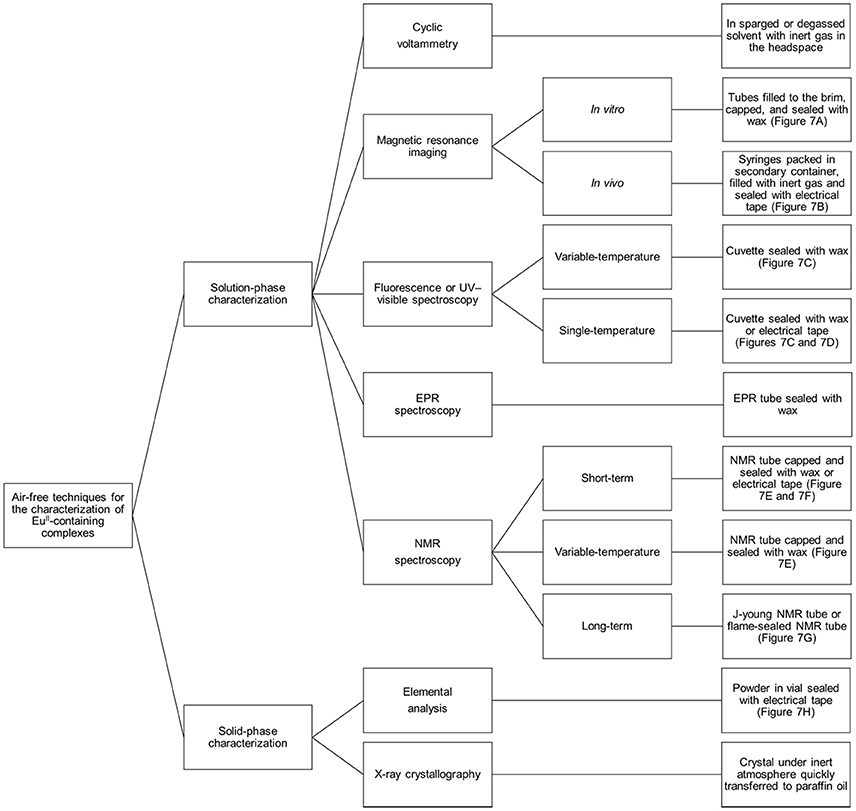
Figure 6. Diagram describing techniques that can be used to analyze EuII-containing complexes with air-free conditions.
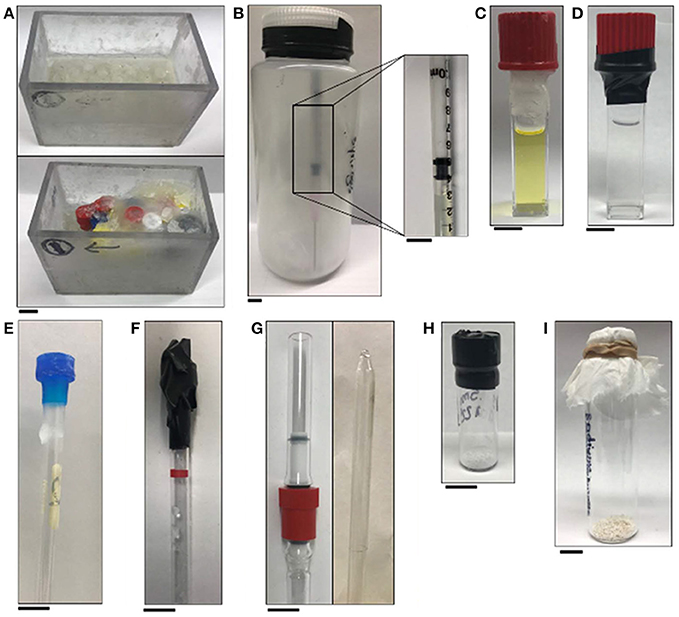
Figure 7. Pictures of apparatuses included in the handling section of this manuscript: (A) empty tube holder (top) and tube holder with tubes that are covered in wax (bottom); (B) jar sealed with electrical tape that contains glass wool and syringes of EuII-containing complexes packed under an inert atmosphere and (inset) a plastic 1 mL syringe with a rubber tip on the plunger that contains a solution of a EuII-containing complex; (C) wax-sealed cuvette; (D) electrical-tape-sealed cuvette; (E) wax-sealed NMR tube; (F) electrical-tape-sealed NMR tube; (G) J-young NMR tube (left) and flame-sealed NMR tube (right); (H) electrical-tape-sealed vial containing a solid sample; and (I) glass vial that contains solid sample covered with a tissue that is secured with a rubber band. All scale bars represent 1 cm.
Cyclic voltammetry or bulk electrolysis performed either inside or outside of a glovebox should use solvents that have been degassed (under reduced pressure, for example, on a Schlenck line) or well-sparged (≥5 min of vigorous bubbling with an inert gas for volumes of ~3 mL in a capped vessel that contains a vent needle). To ensure that there is no detectable dissolved oxygen in solution, cyclic voltammetry of degassed solvents should not show peaks for O2 (Green, 2018). If outside of a glovebox, the inert-gas source should be retracted to the head-space of the vessel once sparging is complete prior to cyclic voltammetry or bulk electrolysis. Inside the glovebox, there is no need for an inert gas line in the headspace because the atmosphere is O2-free. If outside of a glovebox, transfer of solutions of EuII from bulk electrolysis for crystal growth or other analyses must be performed using air-free techniques (Shriver and Drezdzon, 1986).
For other routes to synthesize and handle EuII-containing complexes in aqueous media, air-free handling is achieved using Shlenck techniques, a wet (water allowed but no molecular oxygen) glovebox, or a combination of both. During the synthesis of EuII-containing complexes in a glovebox, the glovebox atmosphere must not be contaminated with oxygen. Either a commercial oxygen sensor or chemical indicator can be used to monitor the atmosphere. One suitable chemical indicator for this purpose is dicyclopentadienyltitanium(IV) dichloride (TiIVCp2Cl2) (Burgmayer, 1998). When the titanium metallocene is dissolved in acetonitrile in the presence of zinc metal, a deep blue solution is obtained. An aliquot of this solution is filtered through celite or a hydrophilic filter and diluted with acetonitrile to yield a diffuse, blue solution caused by the presence of TiIIICp2(NCCH3)2. If the solution remains blue upon evaporation, TiIIICp2(NCCH3)2 is unoxidized, indicating that the atmosphere is good (<5 ppm O2) (Shriver and Drezdzon, 1986). A color change to green is caused by formation of a dimeric species or some oxidation of TiIII to TiIV. A color change to yellow is indicative of near-complete oxidation to TiIVCp2(NCCH3)2. Either green or yellow suggests a bad atmosphere with respect to O2, and steps to address the quality of the atmosphere should be taken prior to working with EuII. If the indicator persists as green or yellow after refreshing the glovebox atmosphere by purging the glovebox with inert gas, then the oxygen-removing catalyst should be replaced or regenerated. Ideally, the atmosphere of a wet glovebox should be checked at least daily, and the atmosphere should be purged with inert gas before and after each use. All liquids to be used in a wet glovebox should be rigorously degassed before transport into the glovebox. Solids to be brought into a glovebox can be placed in an open vial and brought into the antechamber if the solids do not sublime at the temperature and pressure of the glovebox antechamber. To prevent loss of solid from bumping or the vial accidentally tipping, the top of the vial can be covered with tissue that is secured with a rubber band (Figure 7I). If solids sublime at the temperature and pressure of the antechamber, then the solids should be placed under an inert atmosphere in a sealed container prior to being brought into the glovebox.
Solution-phase characterization of EuII-containing samples, including NMR spectroscopy, MRI, and fluorescence or UV–visible absorbance spectroscopy, requires that samples be sealed to prevent oxygen contamination that would interfere with the integrity of the results. For NMR spectroscopy, J-young NMR tubes with Teflon seals or flame-sealed NMR tubes are appropriate for long term studies (Figure 7G). Alternatively, NMR tubes capped with a plastic cap and sealed with paraffin wax or electrical tape suffice for studies that last a few hours (Figures 7E,F and Video S1). For samples that must be shipped, samples can be loaded into NMR tubes that are subsequently flame-sealed (Lenora et al., 2017). For MRI, tubes (for example, glass vials that have a 400 μL capacity) can be filled to the brim with solution (to avoid bubbles), capped, dipped in wax, and loaded into an apparatus that is then covered in paraffin wax (Figure 7A) (Garcia and Allen, 2012b; Garcia et al., 2012; Basal et al., 2017a,b). For in-vivo injections, syringes with rubber-tip plungers can be loaded with sample, packaged in a bottle that is under an atmosphere of N2 or Ar, and sealed with electrical tape (Figure 7B; Basal et al., 2017a). Packed this way, the integrity of samples is sufficient for shipping with glass wool included in the bottle to minimize vibrations during shipping. For samples in cuvettes (for example, samples for emission or absorbance spectroscopy that must be removed from the glovebox), quartz cuvettes with Teflon caps can be sealed with paraffin wax (Figure 7C) or electrical tape (Figure 7D) (Kuda-Wedagedara et al., 2015; Ekanger et al., 2016a; Jin et al., 2016; Basal et al., 2017a). For samples that will undergo temperature changes (heating or cooling), we have observed that paraffin wax is more reliable than electrical tape.
To ensure that a technique or apparatus successfully seals EuII from air over the course of an experiment, the relaxivity, UV–visible absorption, or luminescence spectra of the EuII-containing complex can be measured immediately after sample preparation and after the analyses are complete, if the analyses are nondestructive. Another way to assess the air-free environment and handling of a EuII-containing sample is to measure a spectral feature of the EuII-containing complex as a function of time at different concentrations of europium. Time-dependent measurements can reveal the presence of oxidizing impurities (Burai et al., 2002). For example, the oxidation half-life (t1/2), which is the time at which half of the complex has oxidized, was measured for Eu6 by monitoring the intensity of a complex-specific UV–visible absorbance peak as a function of time (Burai et al., 2002). For a 5 mM solution of Eu6, the t1/2 was found to be 10 days. However, t1/2 increased as a function of concentration of europium (>1 month for Eu6 at 100 mM), suggesting that the t1/2 reported at 5 mM was influenced by the presence of oxidizing impurities such as O2. Oxygen can be avoided using the techniques described in this section.
If a EuII-containing sample cannot be monitored to check the effectiveness of an air-free technique, then another way to assess if an apparatus is sealed from air is to monitor the color change of a solution of TiIIICp2Cl2 sealed in parallel to the sample to be measured (Burgmayer, 1998). The use of TiIIICp2Cl2 as an indicator provides information regarding the technique used to seal the solution from air. However, a limitation of this method is that it does not provide direct information about the EuII-containing complex being analyzed.
Conclusions and Outlook
The unique and tuneable properties of EuII make EuII-containing complexes promising molecular imaging agents. The synthesis, characterization, and handling of EuII require care with respect to the use of air-free techniques and characterization of oxidation states because EuII and EuIII have different molecular imaging properties that confound results if the ions are inadvertently comingled within a sample. We expect that the techniques described in this review will guide the future synthesis, characterization, and handling of EuII-containing complexes for molecular imaging.
Author Contributions
LB and MA: Contributed to the manuscript and approved the final version.
Conflict of Interest Statement
The authors declare that the research was conducted in the absence of any commercial or financial relationships that could be construed as a potential conflict of interest.
Acknowledgments
MA gratefully acknowledges support from the National Institutes of Health (R01EB013663), and LB was supported by a Rumble fellowship from Wayne State University.
Supplementary Material
The Supplementary Material for this article can be found online at: https://www.frontiersin.org/articles/10.3389/fchem.2018.00065/full#supplementary-material
Video S1. Video demonstration of the sealing of an NMR sample with paraffin wax.
References
Abragam, A., and Bleaney, B. (1970). Electron Paramagnetic Resonance of Transition Ions. Belfast: Oxford University Press.
Allen, M. J. (2016). Aqueous lanthanide chemistry in asymmetric catalysis and magnetic resonance imaging. Synlett 27, 1310–1317. doi: 10.1055/s-0035-1561363
Anderson, L. B., and Macero, D. J. (1963). The formal reduction potential of the europium(III)–europium(II) system. J. Phys. Chem. 67, 1942–1942. doi: 10.1021/j100803a520
Angelovski, G. (2016). What we can really do with bioresponsive MRI contrast agents. Angew. Chem. Int. Ed. 55, 7038–7046. doi: 10.1002/anie.201510956
Antonio, M. R., and Soderholm, L. (1996). Redox behavior of europium in the Preyssler heteropolyanion [EuP5W30O110]12−. J. Clust. Sci. 7, 585–591. doi: 10.1007/BF01165803
Bard, A. J., and Faulkner, L. R. (2000). Electrochemical Methods: Fundamentals and Applications, 2nd Edn. New York, NY: John Wiley & Sons, Inc.
Barnham, K. J., Masters, C. L., and Bush, A. I. (2004). Neurodegenerative diseases and oxidative stress. Nat. Rev. Drug Discov. 3, 205–214. doi: 10.1038/nrd1330
Basal, L. A., Bailey, M. D., Romero, J., Ali, M. M., Kurenbekova, L., Yustein, J., et al. (2017a). Fluorinated EuII-based multimodal contrast agent for temperature- and redox-responsive magnetic resonance imaging. Chem. Sci. 8, 8356–8350. doi: 10.1039/C7SC03142D
Basal, L. A., Yan, Y., Shen, Y., Haacke, E. M., Mehrmohammadi, M., and Allen, M. J. (2017b). Oxidation-responsive, EuII/III-based, multimodal contrast agent for magnetic resonance and photoacoustic imaging. ACS Omega 2, 800–805. doi: 10.1021/acsomega.6b00514
Bochkarev, M. N. (2004). Molecular compounds of “new” divalent lanthanides. Coord. Chem. Rev. 248, 835–851. doi: 10.1016/j.ccr.2004.04.004
Botta, M., Ravera, M., Barge, A., Bottaro, M., and Osella, D. (2003). Relationship between ligand structure and electrochemical and relaxometric properties of acyclic poly(aminocarboxylate) complexes of Eu(II). Dalton Trans. 1628–1633. doi: 10.1039/b211533f
Bottrill, M., Kwok, L., and Long, N. J. (2006). Lanthanides in magnetic resonance imaging. Chem. Soc. Rev. 35, 557–571. doi: 10.1039/b516376p
Burai, L., Scopelliti, R., and Tóth, É. (2002). EuII-cryptate with optimal water exchange and electronic relaxation: a synthon for potential pO2 responsive macromolecular MRI contrast agents. Chem. Commun. 2366–2367. doi: 10.1039/B206709A
Burai, L., Tóth, É., Moreau, G., Sour, A., Scopelliti, R., and Merbach, A. E. (2003). Novel macrocyclic EuII complexes: fast water exchange related to an extreme M—Owater distance. Chem Eur. J. 9, 1394–1404. doi: 10.1002/chem.200390159
Burai, L., Tóth, E., Seibig, S., Scopelliti, R., and Merbach, A. E. (2000). Solution and solid-state characterization of EuII chelates: a possible route towards redox responsive MRI contrast agents. Chemistry 6, 3761–3770. doi: 10.1002/1521-3765(20001016)6:20<3761::AID-CHEM3761>3.0.CO;2-6
Burgmayer, S. J. N. (1998). Use of a titanium metallocene as a colorimetric indicator for learning inert atmosphere techniques. J. Chem. Ed. 75, 460–460. doi: 10.1021/ed075p460
Burnett, M. E., Adebesin, B., Funk, A. M., Kovacs, Z., Sherry, A. D., Ekanger, L. A., et al. (2017). Electrochemical investigation of Eu3+/2+ redox couple in complexes with variable numbers of glycinamide and acetate pendant arms. Eur. J. Inorg. Chem. 2017, 5001–5005. doi: 10.1002/ejic.201701070
Caravan, P., Tóth, É., Rockenbauer, A., and Merbach, A. E. (1999). Nuclear and electronic relaxation : an extremely labile aqua ion. J. Am. Chem. Soc. 121, 10403–10409. doi: 10.1021/ja992264v
Christoffers, J., and Starynowicz, P. (2008). A europium(II) complex with bis-pyridino-18-crown-6. Polydron 27, 2688–2692. doi: 10.1016/j.poly.2008.05.028
Corbin, B. A., Hovey, J. L., Thapa, B., Schlegel, H. B., and Allen, M. J. (2018). Luminescence differences between two complexes of divalent europium. J. Organomet. Chem. 857, 88–93. doi: 10.1016/j.jorganchem.2017.09.007
Cullity, B. D., and Graham, C. D. (2009). Introduction to Magnetic Materials, 2nd Edn. Hoboken, NJ: John Wiley & Sons, Inc.
Dorenbos, P. (2003). f→ d transition energies of divalent lanthanides in inorganic compounds. J. Phys. Condens. Matter 15, 575–594. doi: 10.1088/0953-8984/15/3/322
Edelmann, F. T. (2016). Lanthanides and actinides: annual survey of their organometallic chemistry covering the year 2015. Coord. Chem. Rev. 318, 29–130. doi: 10.1016/j.ccr.2016.04.001
Ekanger, L. A., Ali, M. M., and Allen, M. J. (2014). Oxidation-responsive Eu2+/3+-liposomal contrast agent for dual-mode magnetic resonance imaging. Chem. Commun. 50, 14835–14838. doi: 10.1039/C4CC07027E
Ekanger, L. A., and Allen, M. J. (2015). Overcoming the concentration-dependence of responsive probes for magnetic resonance imaging. Metallomics 7, 405–421. doi: 10.1039/C4MT00289J
Ekanger, L. A., Basal, L. A., and Allen, M. J. (2017). The role of coordination environment and pH in tuning the oxidation rate of europium(II). Chem. Eur. J. 23, 1145–1150. doi: 10.1002/chem.201604842
Ekanger, L. A., Mills, D. R., Ali, M. M., Polin, L. A., Shen, Y., Haacke, E. M., et al. (2016a). Spectroscopic characterization of the 3+ and 2+ oxidation states of europium in a macrocyclic tetraglycinate complex. Inorg. Chem. 55, 9981–9988. doi: 10.1021/acs.inorgchem.6b00629
Ekanger, L. A., Polin, L. A., Shen, Y., Haacke, E. M., and Allen, M. J. (2016b). Evaluation of EuII-based positive contrast enhancement after intravenous, intraperitoneal, and subcutaneous injections. Contrast Media Mol. Imaging 11, 299–303. doi: 10.1002/cmmi.1692
Ekanger, L. A., Polin, L. A., Shen, Y., Haacke, E. M., Martin, P. D., and Allen, M. J. (2015). A EuII-containing cryptate as a redox sensor in magnetic resonance imaging of living tissue. Angew. Chem. Int. Ed. 54, 14398–14401. doi: 10.1002/anie.201507227
Evans, W. J. (2000). Perspectives in reductive lanthanide chemistry. Coord. Chem. Rev. 206–207, 263–283. doi: 10.1016/S0010-8545(00)00267-8
Facciabene, A., Peng, X., Hagemann, I. S., Balint, K., Barchetti, A., Wang, L.-P., et al. (2011). Tumour hypoxia promotes tolerance and angiogenesis via CCL28 and Treg cells. Nature 475, 226–230. doi: 10.1038/nature10169
Fieser, M. E., MacDonald, M. R., Krull, B. T., Bates, J. E., Ziller, J. W., Furche, F., et al. (2015). Structural, spectroscopic, and theoretical comparison of traditional vs recently discovered Ln2+ Ions in the [K(2.2.2-cryptand)][(C5H4SiMe3)3Ln] complexes: the variable nature of Dy2+ and J. Am. Chem. Soc. 137, 369–382. doi: 10.1021/ja510831n
Funk, A. M., Clavijo Jordan, V., Sherry, A. D., Ratnakar, S. J., and Kovacs, Z. (2016). Oxidative conversion of a europium(II)-based T1 agent into a europium(III)-based paraCEST agent that can be detected in vivo by magnetic resonance imaging. Angew. Chem. Int. Ed. 55, 5024–5027. doi: 10.1002/anie.201511649
Gál, M., Kielar, F., Sokolová, R., Ramešová, Š., and Kolivoška, V. (2013). Electrochemical study of EuIII/EuII redox properties of complexes with potential MRI ligands. Eur. J. Inorg. Chem. 2013, 3217–3223. doi: 10.1002/ejic.201300252
Gamage, N. D., Mei, Y., Garcia, J., and Allen, M. J. (2010). Oxidatively stable, aqueous europium(II) complexes through steric and electronic manipulation of cryptand coordination chemistry. Angew. Chem. Int. Ed. 49, 8923–8925. doi: 10.1002/anie.201002789
Gansow, O. A., Kausar, A. R., Triplett, K. M., Weaver, M. J., and Yee., E. L. (1977). Synthesis and chemical properties of lanthanide cryptates. J. Am. Chem. Soc. 99, 7087–7089. doi: 10.1021/ja00463a065
Garcia, J., and Allen, M. J. (2012a). Developments in the coordination chemistry of europium(II). Eur. J. Inorg. Chem. 2012, 4550–4563. doi: 10.1002/ejic.201200159
Garcia, J., and Allen, M. J. (2012b). Interaction of biphenyl-functionalized Eu2+-containing cryptate with albumin: implications to contrast agents in magnetic resonance imaging. Inorg. Chim. Acta 393, 324–327. doi: 10.1016/j.ica.2012.07.006
Garcia, J., Kuda-Wedagedara, A. N. W., and Allen, M. J. (2012). Physical properties of Eu2+-containing cryptates as contrast agents for ultrahigh-field magnetic resonance imaging. Eur. J. Inorg. Chem. 2012, 2135–2140. doi: 10.1002/ejic.201101166
Garcia, J., Neelavalli, J., Haacke, E. M., and Allen, M. J. (2011). EuII-containing cryptates as contrast agents for ultra-high field strength magnetic resonance imaging. Chem. Commun. 47, 12858–12860. doi: 10.1039/c1cc15219j
Green, K. (2018). Cyclic Voltammetry (CV). Cambridge, MA: JoVE Science Education Database. Analytical Chemistry. JoVE.
Harris, D. C. (2003). Quantitative Chemical Analysis, 6th Edn. New York, NY: W. H. Freeman and Company.
Jenks, T. C., Bailey, M. D., Hovey, J. L., Fernando, S., Basnayake, G., Cross, M. E., et al. (2018). First use of a divalent lanthanide for visible-light-promoted photoredox catalysis. Chem. Sci. 9, 1273–1278. doi: 10.1039/C7SC02479G
Jiang, J., Higashiyama, N., Machiida, K., and Adachi, G., (1998). The luminescent properties of divalent europium complexes of crown ethers and cryptands. Coord. Chem. Rev. 170, 1–29. doi: 10.1016/S0010-8545(98)00070-8
Jin, G X., Bailey, M. D., and Allen, M. J. (2016). Unique Eu(II) coordination environments with a janus cryptand. Inorg. Chem. 55, 9085–9090. doi: 10.1021/acs.inorgchem.6b01659
Kawasaki, K., Sugiyama, R., Tsuji, T., Iwasa, T., Tsunoyama, H., Mizuhata, Y., et al. (2017). A designer ligand field for blue-green luminescence of organoeuropium(II) sandwich complexes with cyclononatetraenyl ligands. Chem. Commun. 53, 6557–6560. doi: 10.1039/C7CC03045B
Kelly, R. P., Bell, T. D. M., Cox, R. P., Daniels, D. P., Deacon, G. B., Jaroscik, F., et al. (2015). Divalent tetra- and penta-phenylcyclopentadienyl europium and samarium sandwich and half-sandwich complexes: synthesis, characterization, and remarkable luminescence properties. Organometallics 34, 5624–5636. doi: 10.1021/acs.organomet.5b00842
Kuda-Wedagedara, A. N., and Allen, M. J. (2014). Enhancing magnetic resonance imaging with contrast agents for ultra-high field strengths. Analyst 139, 4401–4410. doi: 10.1039/C4AN00990H
Kuda-Wedagedara, A. N., Wang, C., Martin, P. D., and Allen, M. J. (2015). Aqueous Eu(II)-containing complex with bright yellow luminescence. J. Am. Chem. Soc. 137, 4960–4963. doi: 10.1021/jacs.5b02506
Lauffer, R. B. (1987). Paramagnetic metal complexes as water proton relaxation agents for NMR imaging: theory and design. Chem. Rev. 87, 901–927. doi: 10.1021/cr00081a003
Layfield, R. A., and Murugesu, M., (eds.). (2015). Lanthanides and Actinides in Molecular Magnetism. Weinheim: Wiley-VCH Verlag GmbH & Co.
le Masne de Chermont Q., Chanéac, C., Seguin, J., Pellé, F., Maîtrejean, S., Jolivet, J. P., et al. (2007). Nanoprobes with near-infrared persistent luminescence for in vivo imaging. Proc. Natl. Acad. Sci. U.S.A. 104, 9266–9271. doi: 10.1073/pnas.0702427104
Lenora, C. U., Carniato, F., Shen, Y., Latif, Z., Haacke, E. M., Martin, P. D., et al. (2017). Structural features of EuII-containing cryptates that influence relaxivity. Chem. Eur. J. 23, 15404–15414. doi: 10.1002/chem.201702158
Lin, M. T., and Beal, M. F. (2006). Mitochondrial dysfunction and oxidative stress in neurodegenerative diseases. Nature 443, 787–795. doi: 10.1038/nature05292
MacDonald, M. R., Bates, J. E., Ziller, J. W., Furche, F., and Evans, W. J. (2013). Completing the series of +2 ions for the lanthanide elements: synthesis of molecular complexes of Pr2+, Gd2+, Tb2+, and Lu2+. J. Am. Chem. Soc. 135, 9857–9868. doi: 10.1021/ja403753j
Mattson, M. P. (2004). Pathways towards and away from Alzheimer's disease. Nature 430, 631–639. doi: 10.1038/nature02621
McCoy, H. N. (1935). The separation of europium from other rare earths. J. Am. Chem. Soc. 57:1756. doi: 10.1021/ja01312a506
Pan, C. L., Pan, Y. S., Wang, J., and Song, J. F. (2011). A heterometallic sandwich complex of europium(II) for luminescent studies. Dalton Trans. 40, 6361–6363. doi: 10.1039/c1dt10635j
Park, L., Zhou, P., Pitstick, R., Capone, C., Anrather, J., Norris, E. H., et al. (2008). Nox2-Derived radicals contribute to neurovascular and behavioral dysfunction in mice overexpressing the amyloid precursor protein. Proc. Natl. Acad. Sci. U.S.A. 105, 1347–1352. doi: 10.1073/pnas.0711568105
Pierre, V. C., and Allen, M. J., (eds.). (2018). Contrast Agents for MRI: Experimental Methods. Cambridge: Royal Society of Chemistry.
Pierre, V. C., Allen, M. J., and Caravan, P. (2014). Contrast agents for MRI: 30+ years and where are we going? J. Biol. Inorg. Chem. 19, 127–131. doi: 10.1007/s00775-013-1074-5
Regueiro-Figueroa, M., Barriada, J. L., Pallier, A., Esteban-Gómez, D., Blas, A., Rodríguez-Blas, T., et al. (2015). Stabilizing divalent europium in aqueous solution using size-discrimination and electrostatic effects. Inorg. Chem. 54, 4940–4952. doi: 10.1021/acs.inorgchem.5b00548
Renny, J. S., Tomasevich, L. L., Tallmadge, E. H., and Collum, D. B. (2013). Method of continuous variations: applications of job plots to the study of molecular associations in organometallic chemistry. Angew. Chem. Int. Ed. 52, 11998–12013. doi: 10.1002/anie.201304157
Sabbatini, N., Ciano, M., Dellonte, S., Bonazzi, A., and Balzani, V. (1982). Absorption and emission properties of a europium(II) cryptate in aqueous solution. Chem. Phys. Lett. 90, 265–268. doi: 10.1016/0009-2614(82)83236-3
Sabbatini, N., Ciano, M., Dellonte, S., Bonazzi, A., Bolletta, F., and Balzani, V. (1984). Photophysical properties of europium(II) cryptates. J. Phys. Chem. 88, 1534–1537. doi: 10.1021/j150652a018
Shipley, C. P., Capecchi, S., Salata, O. V., Etchells, M., Dobson, P. J., and Christou, V. (1999). Orange electroluminescence from a divalent europium complex. Adv. Mater. 11, 533–536. doi: 10.1002/(SICI)1521-4095(199905)11:73.0.CO;2-U
Shriver, D. F., and Drezdzon, M. A. (1986). The Manipulation of Air-Sensitive Compounds. New York, NY: John Wiley & Sons, Inc.
Shweiki, D., Itin, A., Soffer, D., and Keshet, E. (1992). Vascular endothelial growth factor induced by hypoxia may mediate hypoxia-initiated angiogenesis. Nature 359, 843–845. doi: 10.1038/359843a0
Soderholm, L., Antonio, M. R., Skanthakumar, S., and Williams, C. W. (2002). Correlated electrons in the Eu-exchanged Preyssler anion [EuP5W30O110]n−. J. Am. Chem. Soc. 124, 7290–7291. doi: 10.1021/ja025821d
Teprovich, J. A. Jr., Antharjanam, P. K. S., Prasad, E., Pesciotta, E. N., and Flowers, R. A. II. (2008). Generation of SmII reductants using high intensity ultrasound. Eur. J. Inorg. Chem. 2018, 5015–5019. doi: 10.1002/ejic.200800876
Tóth, É., Burai, L., and Merbach, A. E. (2001). Similarities and differences between the isoelectronic GdIII and EuII complexes with regard to MRI contrast agent applications. Coord. Chem. Rev. 216–217, 363–382. doi: 10.1016/S0010-8545(01)00312-5
Vanek, J., Smrčka, F., Lubal, P., Trískov,á, I., and Trnkov,á, L. (2016). Dual carbonate sensor based on Eu(III) complex of DO3A ligand. Monatsh. Chem. 147, 925–934. doi: 10.1007/s00706-016-1722-x
Wang, J., Ma, Q., Wang, Y., Shen, H., and Yuan, Q. (2017). Recent progress in biomedical applications of persistent luminescence nanoparticles. Nanoscale 9, 6204–6218. doi: 10.1039/C7NR01488K
Yee, E. L., Cave, R. J., Guyer, K. L., Tyma, P. D., and Weaver, M. J. (1979). A survey of ligand effects upon the reaction entropies of some transition metal redox couples. J. Am. Chem. Soc. 101, 1131–1137. doi: 10.1021/ja00499a013
Yee, E. L., Gansow, O. A., and Weaver, M. J. (1980). Electrochemical studies of europium and ytterbium cryptate formation in aqueous solution. Effects of varying the metal oxidation state upon cryptate thermodynamics and kinetics. J. Am. Chem. Soc. 102, 2278–2285. doi: 10.1021/ja00527a024
Yee, E. L., Hupp, J. T., and Weaver, M. J. (1983). The nonadiabicity question for Europium(III/II): outer-sphere reactivities of Europium (III/II) cryptates. Inorg. Chem. 22, 3465–3470. doi: 10.1021/ic00165a020
Keywords: chelating ligands, contrast agents, coordination chemistry, divalent europium, lanthanides, molecular imaging probes
Citation: Basal LA and Allen MJ (2018) Synthesis, Characterization, and Handling of EuII-Containing Complexes for Molecular Imaging Applications. Front. Chem. 6:65. doi: 10.3389/fchem.2018.00065
Received: 16 January 2018; Accepted: 28 February 2018;
Published: 19 March 2018.
Edited by:
Zsolt Baranyai, Bracco Imaging S.p.a, ItalyReviewed by:
Mark Woods, Portland State University, United StatesJean-Claude Georges Bunzli, École Polytechnique Fédérale de Lausanne, Switzerland
Copyright © 2018 Basal and Allen. This is an open-access article distributed under the terms of the Creative Commons Attribution License (CC BY). The use, distribution or reproduction in other forums is permitted, provided the original author(s) and the copyright owner are credited and that the original publication in this journal is cited, in accordance with accepted academic practice. No use, distribution or reproduction is permitted which does not comply with these terms.
*Correspondence: Matthew J. Allen, bWFsbGVuQGNoZW0ud2F5bmUuZWR1