- Key Laboratory of Functional Inorganic Material Chemistry, Ministry of Education, School of Chemistry and Materials Science, Heilongjiang University, Harbin, China
Introduction
With the rapid development of industry, the discharge of sulfide gas has been increasing in recent decades, which results in severe air pollution. H2S, as the typical representation of sulfide, is a harmful and toxic acidic gas and widely used in various industries. Even at low concentrations, it can cause hypoxia and seriously threatens the safety of human. When the concentration reaches 1 mg/L (659 ppm) or higher, inflammation or death will occur. According to the U. S. Scientific Advisory Board on Toxic Air Pollutants, the acceptable concentration of H2S in the environment is less than 83 ppb (North Carolina Department of Environment and Natural Resources, 20031). Therefore, it is necessary to fabricate gas sensors which can detect ppb-level H2S in time to reduce the environmental pollution and harm to human.
Zinc stannate (Zn2SnO4) is a typical n-type ternary semiconductor, and has been employed as important multifunctional material in the fields of photocatalytic activity (Das et al., 2017), solar cells (Li et al., 2015), lithium ion batteries (Lim et al., 2016), and so forth. Especially, owing to the excellent gas-sensing performance of ZnO and SnO2 (Sukunta et al., 2017; Zhu and Zeng, 2017; Zhu et al., 2018), the application of Zn2SnO4 in the field of gas sensors has attracted extensive attention (An et al., 2015; Zhao et al., 2016; Yang et al., 2017). Up to now, only one case has concerned on the detection of H2S with Zn2SnO4 hollow octahedron (Ma et al., 2012), which showed the response to H2S with the detection limit being 1 ppm at 260°C. Meanwhile, such reported Zn2SnO4 sensor presents poor selectivity. Apparently, the sensor cannot satisfy the need of practical application for the detection of ppb-level H2S, especially in a complex environment involving other interfering gases due to its poor selectivity and higher working temperature. In view of the fact that gas-sensing performance of materials is highly dependent on their micro-structure and surface state (Yu et al., 2017; Zhang et al., 2018), therefore, it would be a meaningful work to prepare Zn2SnO4 with novel morphology to further decrease the working temperature and improve the selectivity and stability, thus performing the detection of ppb-level H2S.
In this work, Zn2SnO4 lamellar micro-spheres have been synthesized by a facile ethylenediamine-assisted hydrothermal method followed by calcining at 600°C. The diameter of micro-spheres is ~1 μm and they are composed of nanosheets with thickness of ~85 nm. The sensor fabricated from the micro-spheres shows good response and selectivity to H2S at 170°C, and the lowest detection limit is down to 50 ppb. Moreover, it shows good linear relationship in the range of ppb (50–1000 ppb) and ppm (3–50 ppm) level. Meanwhile, the gas-sensing mechanism is also investigated.
Experimental
Preparation of Zn2SnO4 Lamellar Micro-Spheres
All the reagent were analytical grade (AR) and used as received without supplementary purification. Zn(CH3COO)2·2H2O (1.2 mmol) and SnCl4·5H2O (0.6 mmol) were dissolved in the mixture of 20 mL deionized water and 10 mL ethylenediamine. After stirring for 30 min, 7.2 mmol NaOH was added to the above system followed by further stirring for 1 h. Then, the above turbid solution was transferred into a 50 mL Teflon-lined stainless steel autoclave and kept at 200°C for 24 h. After cooling to room temperature, the precipitate was centrifuged and washed with deionized water and ethanol for several times. White products were obtained after drying at 60°C for 12 h, which was then calcined at 600°C for 2 h in air atmosphere to obtain the Zn2SnO4 lamellar micro-spheres.
The characterization, sensor fabrication and measurement were listed in the Supporting Information.
Results and Discussion
The FT-IR spectra of the precursor and calcined product were illustrated in Figure S1a. After calcination, the corresponding vibrations of acetate disappear. The spectrum of calcined product exhibits one peak at 3417 cm−1, corresponding to the stretching vibration of water molecules. The Sn-O and Zn-O vibrations at 572 and 431 cm−1 in this spectrum are slightly enhanced, which indicates that the calcined product has excellent crystallinity. The TG curve of the precursor (Figure S1b) shows that the marked weight loss of 6.27% between 30 and 600°C can be attributed to the loss of water molecules and small amount of acetates. After 600°C, no obvious weight loss occurs with the increase of temperature. Therefore, the precursor is calcined at 600°C for 2 h. The XRD patterns of the precursor and calcined product are also conducted to confirm their identity and phase purity. As illustrated in Figure 1A, all the diffraction peaks in both precursor and calcined product are consistent with standard cubic Zn2SnO4 (JCPDS Card No. 24-1470). In comparison with the precursor, the calcined product presents stronger diffraction peaks and better consistency. It shows that the calcined product has better crystallinity. The surface components of the calcined product characterized by XPS spectrum indicate that the final product involves Zn, Sn and O elements (Figure S2).
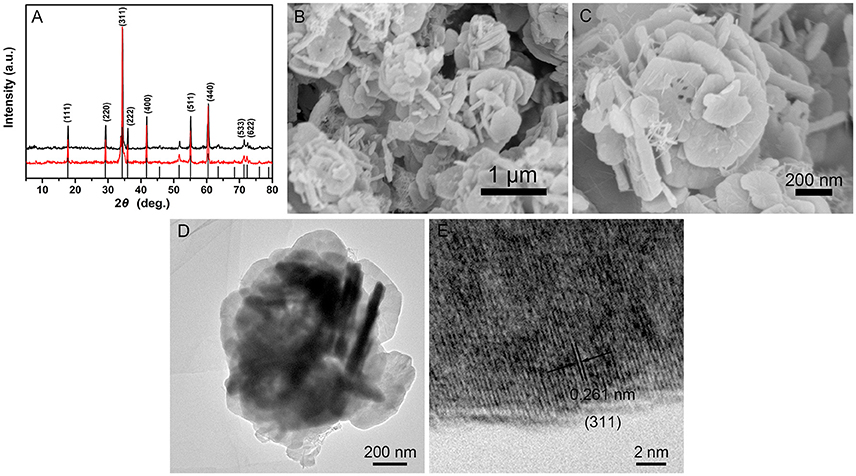
Figure 1. (A) XRD patterns of the precursor and the calcined product. (B,C) SEM images of the calcined product. (D,E) TEM and HRTEM images of the calcined product.
As shown in Figure 1B, the micro-spheres of the precursor with diameter of ~1.5 μm are composed of smooth nanosheets with thickness of ~130 nm. After being calcined at 600°C in air, the calcined products maintain the morphology of precursor, and the diameter of the micro-spheres is slightly decreased from ~1.5 to ~1 μm (Figure 1C and Figure S3). The thickness of the nanosheets is also sharply reduced to ~85 nm. The results shown in Figure S4 reveal that the three elements are distributed very homogeneously in the micro-spheres. Figure 1D shows TEM image for the Zn2SnO4 lamellar micro-sphere which presents a clear contrast between the dark and the pale parts, revealing that the micro-spheres are further assembled by nanosheets. The high resolution TEM (HRTEM) image in Figure 1E exhibits the clear parallel fringes with d-spacing of 0.261 nm for nanosheet, which corresponds to the (311) lattice planes of cubic Zn2SnO4. Meanwhile, some micro-pores appears on the surface of the nanosheets, which implies better gas performance of the lamellar micro-spheres.
Figure 2A is the response of Zn2SnO4 lamellar micro-spheres sensor toward 100 ppm of H2S at different working temperatures. As can be seen, the response of sensor to H2S decreases with the increase of working temperature. This is mainly because the adsorbed H2S gas molecules escaped from the surface of materials easily before the reaction taking place at high operating temperature so as to a poor response as well. In other word, such commonly observed phenomenon in resistive sensors is mainly ascribed to the fact that the desorption plays dominant role at higher temperature which leads to the decrease in sensitivity. It shows that the best working temperature for the Zn2SnO4 sensor is 170°C, which is 90°C lower than that of Zn2SnO4 hollow octahedron (Ma et al., 2012). Thus, the following tests were carried out at 170°C. Figure 2B is the response of the sensor toward 100 ppm of CH4, NH3, CH3COCH3, HCHO, C2H5OH and H2S at 170°C. The responses to the above gases are 1.04, 1.49, 1.08, 1.2, 10.95, 65.13, respectively, which show that the sensor presents the best response to H2S than other gases. The selectivity coefficients (KAB) of H2S to other gases are 62.63, 43.71, 60.31, 54.27, 5.94, respectively, indicating excellent selectivity of the sensor to H2S. Such highly H2S selectivity can be attributed to the reactivities of the reducing test gases. The bond energy of 381 kJ/mol for H-SH in H2S (Liu et al., 2009) is smaller than other inorganic gases and most of organic gases, so that H-SH bond can be easily broken to participate in the reaction with gas sensor during chemical adsorption. Given all that, the sensor has good response and selectivity to H2S at 170°C.
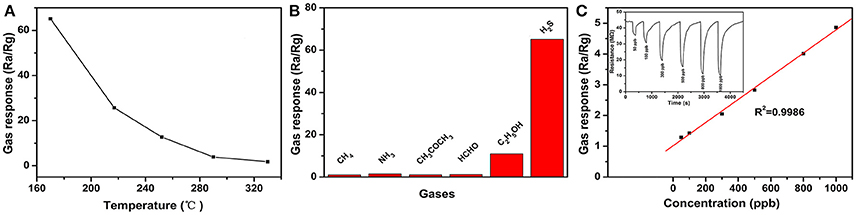
Figure 2. (A) The response of Zn2SnO4 lamellar micro-spheres sensor toward 100 ppm of H2S at different working temperatures. (B) The response of Zn2SnO4 hierarchical microspheres sensor toward 100 ppm of different testing gases at 170°C. (C) The relationship between the responses of Zn2SnO4 lamellar micro-spheres sensor and ppb-level concentrations of H2S (Inset: the response-recovery characteristics of the sensor to different concentrations of H2S at 170°C).
The relationship between the responses of the sensor and ppb-level concentrations of H2S and the response-recovery characteristics of the sensor to different concentrations of H2S at 170°C are shown in Figure 2C. The gas response of the sensor increases in a good linear relationship from 50 to 1000 ppb (R2 = 0.9986), indicating that the detection limit of the sensor is 50 ppb (response of 1.29) to H2S. In contrast to the reported Zn2SnO4 octahedron sensor (Ma et al., 2012), the present sensor displays lower working temperature and ppb-level detection limit. Even compared with other preferable ternary metal oxides, such as La2NiO4 particles (500°C, 20 ppb, Hao et al., 2018), K2W4O13 nano-wires (300°C, 0.3 ppm, Supothina et al., 2014), Fe2(MoO4)3 nano-particles and micro-spheres (300°C, 1 ppm, Liang et al., 2016), the present material still shows better sensing performance toward H2S. To the best of our knowledge, the detection limit of Zn2SnO4 lamellar micro-spheres sensor is only slightly higher than that of flower-like Bi2MoO6 (Cui et al., 2017) which has the lowest detection limit of 0.1 ppb to H2S. The response of the sensor in the range from 3 to 50 ppm also increases in a good linear relationship (R2 = 0.9927) (Figure S5). The responses of the sensor to 10 ppm H2S during 5 consecutive tests at 170°C is shown in Figure S6a. It shows that the sensor keeps its initial response amplitude after 5 cycles. The sensor measurement also maintains initial response to 10 ppm H2S with the standard error of 4.9% after 60 days (Figure S6b). These results indicate that this sensor has a satisfactory reproducibility and stability. Meanwhile, the influence of relative humidity on the sensor was considered at 170°C (Figure S7). It can be seen from Figure S7 that the sensor response has a small change from 1.2 to 1.4 in different humidity environment, suggesting that the influence of humidity on the sensor can be neglected at such temperature. Therefore, the present Zn2SnO4 lamellar micro-spheres sensor could be utilized as promising material for detecting ppb-level H2S.
It is considered that the gas sensing property of semiconductor oxides is related to the surface adsorption oxygen, therefore, the chemical state changes of each element in the Zn2SnO4 sensor were analyzed before and after the sensor contacting with H2S at 170°C. The results indicate that there is no change in the spectra of the Zn 2p and Sn 3d (Figure S8). As shown in Figures S9a,b, the O 1s spectra of Zn2SnO4 could be deconvoluted into three peaks at 531.9/531.8, 530.8/530.7, 529.4/529.3, corresponding to hydroxyl oxygen, surface adsorbed oxygen and lattice oxygen, respectively. The percentage of surface adsorbed oxygen drops from 30.04 to 27.01% after the sensor contacting with H2S. The obvious decreasing of the adsorbed oxygen indicates that H2S participates in redox reaction with the surface adsorbed oxygen. When the Zn2SnO4 sensor is exposed to H2S at 170°C, the S 2p XPS spectrum displays three peaks at 168.9, 163.2 and 161.5 eV that correspond to SO2 and sulfide (S 2p3/2 and S 2p1/2) (Figure S9c), respectively. The appearance of the three peaks indicates that H2S is oxidized to SO2. Combined with previous reports (Yu et al., 2015), the redox reaction engenders between H2S and the surface adsorbed oxygen, and generates the product of SO2.
Based on the aforementioned results, the gas sensing mechanism is speculated as follows: the resistance changes of the gas sensor are observed before and after contacting with H2S. When the Zn2SnO4 sensor is exposed in air, oxygen molecules which is adsorbed on the surface of the sensor (Equation 1) captures electrons in conduction band of Zn2SnO4 to form of O− at 170°C (Equation 2), and engender electron depletion layer. Then, after the sensor contacting with the H2S, the H2S is oxidized by the O− on the surface of sensor and releases electrons back into the conduction band (Equations 3, 4), resulting in an increase in surface electrons and conductivity, and a decrease in resistance.
Conclusion
In conclusion, a facile ethylenediamine-assisted hydrothermal method followed by calcining at 600°C led to the formation of Zn2SnO4 lamellar micro-spheres which comprise of nanosheets with thickness of ~85 nm. Such sensor exhibits excellent selectivity, sensitivity, humidity resistance and stability to H2S at working temperature 170°C. The responses of the sensor, increased with the increasing concentrations in the range of 50–1000 ppb and 3–50 ppm exhibit good relationships with the detection limit of 50 ppb. These results indicate that the Zn2SnO4 lamellar micro-spheres could be utilized as promising sensor material for detecting ppb-level H2S.
Author Contributions
T-TX performed the experiments and analyzed the data with the help from Y-MX and X-FZ. Z-PD wrote the manuscript with input from all authors. L-HH and SG conceived the study. All authors read and approved the manuscript.
Conflict of Interest Statement
The authors declare that the research was conducted in the absence of any commercial or financial relationships that could be construed as a potential conflict of interest.
Acknowledgments
This work is financial supported by Interational Science & Technology Cooperation Program of China (2016YFE0115100), the Project of Natural Science Foundation of Heilongjiang Province (No. B2015007), the Scientific and Technological Innovation Talents of Harbin (2016RAQXJ005), and the Young Innovation Talents of college in Heilongjiang Province (UNPYSCT-2016074). We thank the Key Laboratory of Functional Inorganic Material Chemistry, Ministry of Education, Heilongjiang University for supporting this study.
Supplementary Material
The Supplementary Material for this article can be found online at: https://www.frontiersin.org/articles/10.3389/fchem.2018.00165/full#supplementary-material
Footnotes
1. ^http://daq.state.nc.us/toxics/studies/H2S/ (accessed 2003).
References
An, D., Wang, Q., Tong, X., Zhou, Q., Li, Z., Zou, Y., et al. (2015). Synthesis of Zn2SnO4 via a co-precipitation method and its gas-sensing property toward ethanol. Sens. Actuat. B 213, 155–163. doi: 10.1016/j.snb.2015.02.042
Cui, X. L., Xu, Y. M., Zhang, X. F., Cheng, X. L., Gao, S., Zhao, H., et al. (2017). In-situ deposited flower-like Bi2MoO6 microspheres thin film based sensors for highly selective detection of ppb-level H2S at low temperature. Sens. Actuat. B 247, 681–690. doi: 10.1016/j.snb.2017.03.100
Das, P. P., Roy, A., Tathavadekar, M., and Devi, P. S. (2017). Photovoltaic and photocatalytic performance of electrospun Zn2SnO4 hollow fibers. Appl. Catal. B 203, 692–703. doi: 10.1016/j.apcatb.2016.10.035
Hao, X. D., Ma, C., Yang, X., Liu, T., Wang, B., Liu, F. M., et al. (2018). YSZ-based mixed potential H2S sensor using La2NiO4 sensing electrode. Sens. Actuat. B 255, 3033–3039. doi: 10.1016/j.snb.2017.09.127
Li, Z. D., Zhou, Y., Mao, W. T., and Zou, Z. G. (2015). Nanowire-based hierarchical tin oxide/zinc stannate hollow microspheres: enhanced solar energy utilization efficiency for dye-sensitized solar cells and photocatalytic degradation of dyes. J. Power Sources 274, 575–581. doi: 10.1016/j.jpowsour.2014.10.129
Liang, J. J., Zhuo, M., Guo, D., Chen, Z., Ren, W. J., Zhang, M., et al. (2016). Green and rapid synthesis of 3D Fe2(MoO4)3 by microwave irradiation to detect H2S gas. Mater Lett. 168, 171–175. doi: 10.1016/j.matlet.2016.01.048
Lim, Y. R., Jung, C. S., Im, H. S., Park, K., Park, J., Cho, W. I., et al. (2016). Zn2GeO4 and Zn2SnO4 nanowires for high-capacity lithium- and sodium-ion batteries. J. Mater Chem. A 4, 10691–10699. doi: 10.1039/C6TA02829B
Liu, Z., Fan, T., Zhang, D., Gong, X., and Xu, J. (2009). Hierarchically porous ZnO with high sensitivity and selectivity to H2S derived from biotemplates. Sens. Actuat. B 136, 499–509. doi: 10.1016/j.snb.2008.10.043
Ma, G. X., Zou, R. J., Jiang, L., Zhang, Z. Y., Xue, Y. F., Yu, L., et al. (2012). Phase-controlled synthesis and gas-sensing properties of zinc stannate (ZnSnO3 and Zn2SnO4) faceted solid and hollow microcrystals. Cryst. Eng. Comm. 14, 2172–2179. doi: 10.1039/c2ce06272k
Sukunta, J., Wisitsoraat, A., Tuantranont, A., Phanichphant, S., and Liewhiran, C. (2017). Highly-sensitive H2S sensors based on flame-made V-substituted SnO2 sensing films. Sens. Actuat. B 242, 1095–1107. doi: 10.1016/j.snb.2016.09.140
Supothina, S., Suwan, M., and Wisitsoraat, A. (2014). Hydrothermal synthesis of K2W4O13 nanowire with high H2S gas sensitivity. Microelectron. Eng. 126, 88–92. doi: 10.1016/j.mee.2014.06.015
Yang, H. M., Ma, S. Y., Jiao, H. Y., Chen, Q., Lu, Y., Jin, W. X., et al. (2017). Synthesis of Zn2SnO4 hollow spheres by a template route for high-performance acetone gas sensor. Sens. Actuat. B 245, 493–506. doi: 10.1016/j.snb.2017.01.205
Yu, T. T., Cheng, X. L., Zhang, X. F., Sui, L. L., Xu, Y. M., Gao, S., et al. (2015). Highly sensitive H2S detection sensors at low temperature based on hierarchically structured NiO porous nanowall arrays. J. Mater Chem. A 3, 11991–11999. doi: 10.1039/C5TA00811E
Yu, Y., Xia, Y., Zeng, W., and Liu, R. (2017). Synthesis of multiple networked NiO nanostructures for enhanced gas sensing performance. Mater Lett. 206, 80–83. doi: 10.1016/j.matlet.2017.06.119
Zhang, Y., Zeng, W., Ye, H., and Li, Y. (2018). Enhanced carbon monoxide sensing properties of TiO2 with exposed {001} facet: a combined first-principle and experimental study. Appl. Surf. Sci. 442, 507–516. doi: 10.1016/j.apsusc.2018.02.036
Zhao, Q., Ju, D., Song, X., Deng, X., Ding, M., Xua, X., et al. (2016). Polyhedral Zn2SnO4: synthesis, enhanced gas sensing and photocatalytic performance. Sens. Actuat. B 229, 627–634. doi: 10.1016/j.snb.2016.01.129
Zhu, L., Li, Y., and Zeng, W. (2018). Hydrothermal synthesis of hierarchical flower-like ZnO nanostructure and its enhanced ethanol gas-sensing properties. Appl. Surf. Sci. 427, 281–287. doi: 10.1016/j.apsusc.2017.08.229
Keywords: Zn2SnO4, H2S gas, ppb-level, lamellar micro-spheres, sensitivity
Citation: Xu T-T, Xu Y-M, Zhang X-F, Deng Z-P, Huo L-H and Gao S (2018) Enhanced H2S Gas-Sensing Performance of Zn2SnO4 Lamellar Micro-Spheres. Front. Chem. 6:165. doi: 10.3389/fchem.2018.00165
Received: 12 March 2018; Accepted: 23 April 2018;
Published: 14 May 2018.
Edited by:
Wen Zeng, Chongqing University, ChinaCopyright © 2018 Xu, Xu, Zhang, Deng, Huo and Gao. This is an open-access article distributed under the terms of the Creative Commons Attribution License (CC BY). The use, distribution or reproduction in other forums is permitted, provided the original author(s) and the copyright owner are credited and that the original publication in this journal is cited, in accordance with accepted academic practice. No use, distribution or reproduction is permitted which does not comply with these terms.
*Correspondence: Zhao-Peng Deng, ZGVuZ3poYW9wZW5nQGhsanUuZWR1LmNu
Shan Gao, c2hhbmdhbzY3QHlhaG9vLmNvbQ==