- 1School of Chemical Engineering, Northeast Electric Power University, Jilin, China
- 2School of Chemistry, Beihang University, Beijing, China
- 3Pipeline Technology Research Center, China University of Petroleum–Beijing, Beijing, China
One-dimensional (1D) porous NixSy nanostructures have been successfully fabricated by two-step method consisting of solvothermal and subsequent annealing process. The suitable heat treatment temperature and reaction time play crucial roles in the final structure, morphology, as well as performance. The uniform and perfect porous NixSy nanostructures obtained at 310°C exhibit outstanding microwave absorption performances. A minimum reflection loss of −35.6 dB is achieved at 8.5 GHz, and the effective absorption bandwidth almost covers 14.5 GHz with the absorber thickness range of 2.0–5.0 mm. It can be supposed that this porous structure with rough surface which is favor for increasing the microwave multiple reflection and scattering, contributes a high-performance electromagnetic absorption.
Introduction
Microwave absorber with strong capacity in absorption, low proportion in filler loading, thin thickness in coating, and wide bandwidth in absorption frequency, has aroused burgeoning research interest because of their great potential applications both in military and civil fields, including stealth technology, information security, electromagnetic interference shielding, and healthcare (Zhu et al., 2010; Zhao H. et al., 2014). As is well known that many factors such as morphology, geometry and structure, have vital impacts on determining the microwave absorption (MA) properties (He et al., 2013). Conventional microwave absorbers with different morphologies that have been divided into three classes are as follows: (1) one-dimensional nanostructures such as ZnO nanowires (Wang et al., 2014), Bi3S2 nanorods (Luo et al., 2014); (2) two-dimensional materials such as MoS2 nanosheets (Ning et al., 2015), α-Fe2O3 flakes (Lv et al., 2015a); and (3) three-dimensional network structures, including Co20Ni80 hierarchical nanospheres (Liu et al., 2015), Ni chains nets (Liu et al., 2016), Fe3O4@carbon ordered arrays (Yuan et al., 2015) and so on. However, the aforementioned materials usually possess high density, thus leading to severe limitations to their practical applications in some specialized fields. In this regard, materials with characteristics of low density and special void spaces, such as yolk–shell structural microspheres (Liu et al., 2013; Yu et al., 2014; Qiang et al., 2016), foam composites (Zhang Y. et al., 2015; Zhao H. B. et al., 2016), as well as porous nanostructures (Yan et al., 2009; Zhou et al., 2010; Zhu et al., 2011), are highly beneficial to obtaining superior microwave absorption performance.
Considerable attention has been concentrated on the porous structure of MA materials owing to its fascinating characteristics. For example, Liu et al. fabricated porous carbon/Co composites, and the results suggested that the composites with large dielectric loss could achieve a minimum RL of −40 dB at 4.2 GHz with a coating thickness of 5 mm (Liu et al., 2008). Lv et al. synthesized Co/CoO porous 3-D flower nanostructure through annealing process at 400°C and found that the minimal reflection loss was up to −50 dB when the coating thickness was 3.5 mm (Lv et al., 2015b). Similarly, Wang et al. reported the formation of porous flower-like NiO decorated graphene, and the composites with a filler loading of 25 wt% exhibited highly MA performance (−59.6 dB) because of their special porous structures and numerous void spaces (Wang et al., 2017). Benefiting from the porous structure, the above-mentioned materials show superior electromagnetic wave absorption performance, which reveals that these porous materials are effective as MA materials.
Metal sulfides, as semiconductor materials, have been proven to have promising potential as an ideal microwave absorber on account of their typical dielectric loss mechanism (Zhang X. J. et al., 2017). Recent studies have suggested that metal sulfides including MoS2 (Wang et al., 2015), CoS2 (Zhang C. et al., 2017), CdS (Zhang et al., 2014), and CuS (He et al., 2014), as well as various phase of nickel sulfides (Zhou et al., 2010) can effectively absorb electromagnetic waves and attenuate them in the form of thermal energy. However, the microwave-absorbing properties of NixSy with special porous structure have not been reported previously. Based on the above study, we demonstrated the successful design and fabrication of porous one-dimensional NixSy nanomaterial through a facile solvothermal route together with annealing process. The MA properties of resultant composites were investigated in detail for the first time. As expected, the synthesized NixSy nanostructure exhibited excellent microwave absorption property confirming that this material can be used as high-performance microwave absorber.
Materials and Methods
Preparation of Porous NixSy
Nickel nitrate hexahydrate (Ni(NO3)2·6H2O), elemental sulfur, ethylene glycol (EG), and ethylenediamine (EN) were purchased from Nanjing Chemical Reagent Co. All of the chemical reagents were analytical-grade purity and used without further purification.
Typically, nickel sulfide was synthesized by the reaction of Ni(NO3)2·6H2O, EN and sulfur powder in EG. At first, Ni(NO3)2·6H2O (0.3489 g) was added to EG (135 mL) under strong magnetic stirring to form a light green homogeneous solution. Then the sulfur powder (0.0288 g) was dissolved in EN (15 mL) through ultrasonic treatment. Mix the two solutions together and put it into oil bath, maintained at 120°C for 6 h. After being cooled to room temperature, the resulting solid precursors were centrifuged, washed with alcohol to remove possible remnant, and finally dried in air at 60°C for 24 h. The dried precursors were treated at 310°C for 2 h with a heating rate of 2°C/min under N2 atmosphere to get the final NixSy products.
Preparation of Nickel Sulfide/PVDF Nanocomposites
The polyvinylidene fluoride (PVDF) was first dispersed in N-N dimethylformamide (20 mL) under magnetic stirring for 1 h. Then, the desired amount of nickel sulfide was added into the suspension. After ultrasonication for another 1 h, the mixture was poured onto a glass plate and dried at 80°C for 24 h. The samples for testing were also compacted into a cylindrical compact (Φout = 7.00 mm and Φin = 3.04 mm) by hot pressing at 210°C under 5 MPa (pressed for 15 min, followed by cooling to room temperature under the same pressure).
Instrumental Analyses
The X-ray diffraction (XRD) pattern of the nickel sulfide product was carried out on a Rigaku, Dmax2200 diffractometer equipped with a CuKa radiation source (λ = 1.5416 Å) in the range of 2θ = 10–80°. For the phase analysis. Further microstructural analyses were performed by using a FEI Quanta 250 field emission gun environmental scanning electron microscope (JSM-6700F microscope) at 15 kV. In brief, SEM samples were prepared by diluting the final products with alcohol by ultrasonic treatment and dropping it on the silicon slice. The relative permittivity (ε′, ε″) and permeability (μ′, μ″) values were measured using two-port vector network analyzer (Agilent E5071C) over the frequency of 2–18 GHz at room temperature, coupled with a coaxial wire setup. Finally, the reflection loss (RL, dB) value which presents the ratio of the total reflected microwave power against the incident microwave power can be calculated by using the following formulas (Abbas et al., 2006; Xu et al., 2018).
where Zin is the normalized input characteristic impedance, f is the frequency of microwave, d is the thickness of the absorber. A lower RL value stands for a better MA performance.
Results and Disscussion
An illustration of the synthesis of the NixSy is shown in Figure S1. We first prepared the precursors of NixSy nanorods by a simple solvothermal method in a controlled way as described later. Then the collected dried precursors were transferred into a tube furnace and annealed at 310°C for 2 h under an N2 atmosphere, which eventually led to the generation of the porous NixSy. It is well recognized that reaction parameters such as temperature, pressure, reaction time, type of solvent, and concentration of reagents, have a huge effect on the morphology of the products. In the present reaction system, Ethylene glycol and Ethylenediamine were applied as solvents for the precursors synthesis of NixSy, and the temperature as well as reaction time was tightly regulated so that the precursors at a well-defined state were obtained.
Figure 1 shows the representative SEM images of the precursors prepared at different temperature of 80, 100, 120, 140, 160, and 180°C, respectively. When the reaction is performed under the lower temperature (80 and 100°C), although one-dimensional structures of products can be formed, their thicknesses and lengths are varying greatly. On the contrary, when the temperature reaches 180°C, the 1D nanostructures are nonuniform, and a certain number of spherical impurities emerge. By comparing the morphology of the products under different temperature conditions, the best reaction temperature is determined. The products are well-defined nanorods with diameters of about 50 nm and lengths of several micrometers at the suitable reaction temperature of 120°C (Figure 1c). On the other hand, the stages of the growth process are monitored at 120°C by varying the reaction time from 2 to 10 h (Figure S2). It is interesting to find that reaction time does not change the overall morphology of the precursors. However, the yield of the products is very low within a short period of 2 h, indicating the slow reaction rate in such reaction system. Further increasing the reaction time produce more products, while the diameter and structure of the nanorods remain stable.
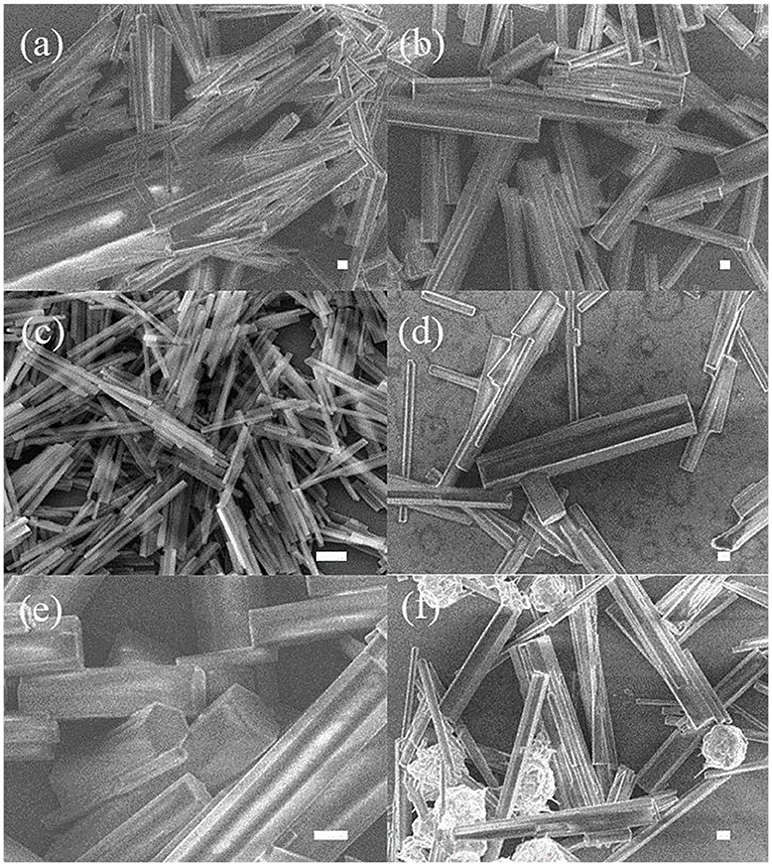
Figure 1. SEM images of precursor of NixSy samples under different reaction temperatures: (a) 80, (b) 100, (c) 120, (d) 140, (e) 160, and (f) 180°C. (scale bar = 1 μm).
To obtain porous 1D nanostructures, the precursors were annealed at different temperatures ranging from 280 to 330°C under the flowing nitrogen gas, and the final products are exhibited in Figure 2. It can be seen that the surfaces are actually becoming porous with annealing temperature, and the as-obtained NixSy retain the rods morphology with appropriate porosity when prepared at 310°C. Further increasing the annealing temperature will led to 1D structural instability and collapse. The energy-dispersive X-ray spectroscopy (EDS) indicates that the obtained product is composed of Ni, S, C, N, and O elements, also demonstrating a very homogeneous elemental distribution (Figure 3). It is worth mentioning that the C, N, and O element signals originate from the incomplete decomposition of organic compositions of precursors during heat treatment. Meanwhile, the XRD results suggest that the as-synthesized products are poorly crystallized, containing NiS2 phase and Ni7S6 phase (Figure S3).
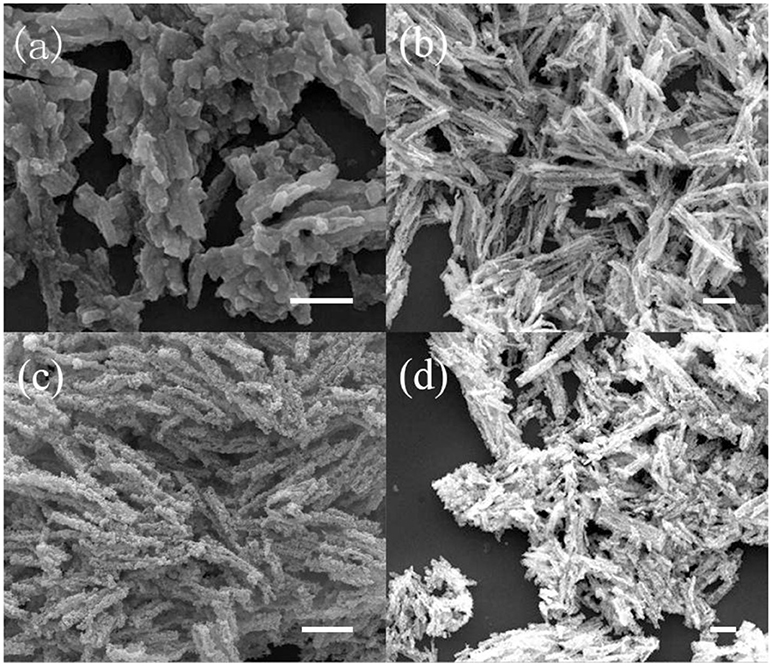
Figure 2. SEM images of NixSy samples under different annealing temperatures: (a) 280, (b) 300, (c) 310, and (d) 330°C. (scale bar = 1 μm).
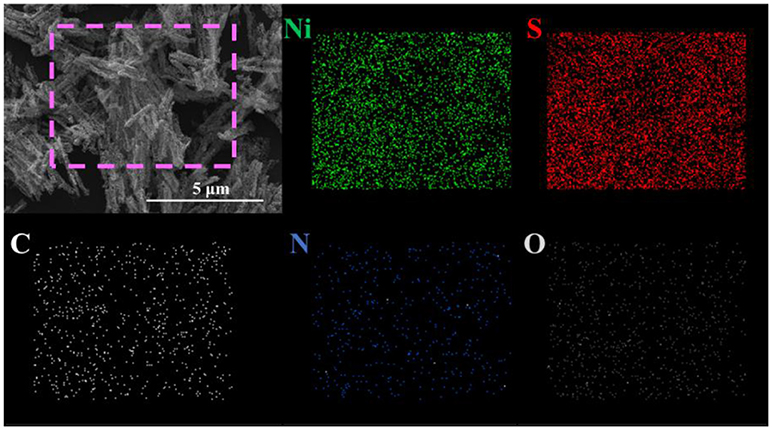
Figure 3. FESEM image of the NixSy nanorods and corresponding elemental mapping images of Ni, S, C, N, and O.
Figures 4A,B show the frequency dependence of the real part (ε′) and imaginary part (ε″) of the complex permittivity for NixSy/PVDF composites with 10, 20, 30, and 40 wt% filler loadings in the frequency range of 2–18 GHz. Generally, the ε′ is known to stand the storage capability of electromagnetic energy, and ε″ associated with various of polarization present the energy dissipation (Zhang X. et al., 2015; Zhao B. et al., 2016). As can be seen in Figure 4, with the increase of NixSy, the ε′ and ε″ values show a similar tendency. For nanohybrids with low concentration of filler (10 and 20 wt%), the values of ε′ and ε″ are approximately equal to some certain constant in the whole frequency range (ε′ = 4, ε″ = 0.5, and ε′ = 6, ε″ = 1). With the NixSy proportion increasing from 20 to 30 wt%, the ε′ increases from 6 to 13 and the ε″ changes from 1 to 4.5 at 2 GHz. However, when the proportion of fillers is increased to 40 wt%, both the ε′ and ε″ dramatically decrease, which is possibly due to the fact that the higher concentration of NixSy in this nanohybrid may result in severe agglomeration. Similar phenomena could be observed in dielectric loss tangent (tan δe = ε″/ε′) which is universally applied to evaluate the dielectric loss capacity of the microwave absorber (Yang et al., 2017), shown in Figure 4C. It can be seen that the dielectric loss tangent increases with the NixSy proportion first, getting a maximum value of 0.35 with 30 wt% NixSy, and then decreases to a value of 0.1.
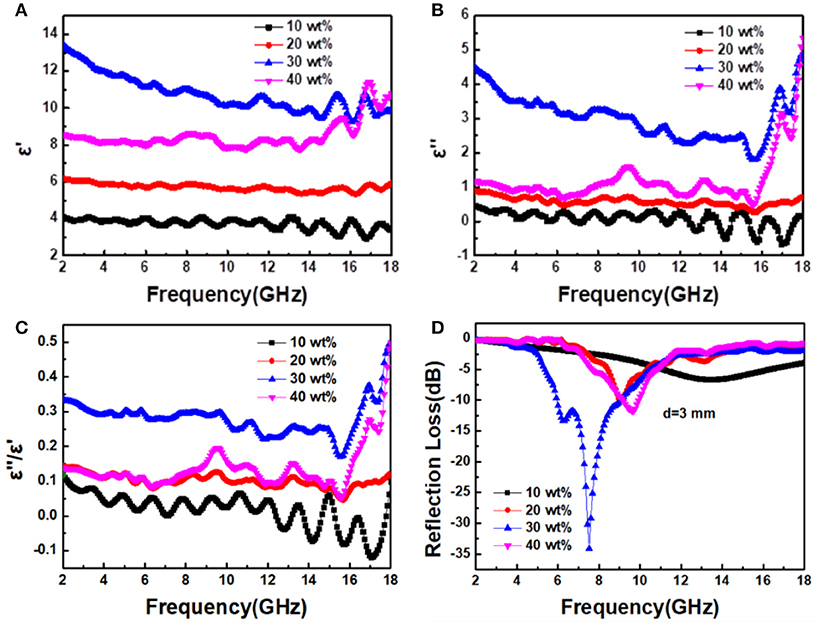
Figure 4. Frequency dependence of (A) real part and (B) imaginary part of permittivity, (C) dielectric loss tangent, and (D) reflection loss under a 3 mm thickness for NixSy composites with different filler loadings.
On the basis of the above analysis, it can be deduced that the nanohybrids with 30 wt% NixSy possess the best microwave absorption properties which is highly consistent with the test results, shown in Figure 4D. The minimum RL reaches −34 dB at 7.5 GHz with a thickness of 3 mm, indicating that 99.9% of incident electromagnetic wave is attenuated. Since NixSy is a typical semiconductive material, various polarization, and related relaxation resulting in a strong dielectric loss are the dominant mechanism for microwave attenuation (Zhao et al., 2015). The dielectric loss of NixSy mainly originate from the defect dipole polarization, the interfacial polarization, and the electronic relaxation loss. First, the defect dipoles are generated by the charge unbalance around the sulfur vacancies in the NixSy lattice, while the interfacial polarizations come from the existence of plentiful interfaces between porous fillers and polymer matrix. Moreover, the porous structures of fillers with rough surfaces further induce the multiple reflection and scattering, resulting in more longer propagation path and greater energy loss (Figure 5). Second, the carbonation of precursors benefits electrical conduction, while the internal doping with nitrogen favors electron transport thus further improving electrical conduction. Furthermore, the calcination also induces a structural disorder and defects into NixSy that frequently lead to an enhanced electrical activity. This calcination process results in significant electronic relaxation polarization, which would enhance the dielectric loss obviously.
Figures 6A–D shows three-dimensional presentations of calculated reflection loss for the NixSy/PVDF with different filler loadings. Clearly, the composite with 30 wt% NixSy has the best performance, and the RL values under different thickness are shown in Figure 6E. The minimal reflection loss of −35.6 dB is obtained at 8.5 GHz with a coating thickness of 2.7 mm and the effective bandwidth is about 3 GHz. Furthermore, the RLs exceeding −10 dB in the frequency range of 3.68–18 GHz are obtained for a variation in absorber thicknesses of 2.0–5.0 mm, demonstrating that this kind of materials has great potential for use as a microwave absorber. Meanwhile, there is an interesting phenomenon that with the increasing thickness of absorber the RL peaks shift to the lower-region frequency. This phenomenon is consistent with a so-called quarter-wavelength (λ/4) matching model (Deng and Han, 2007; Wang et al., 2011, 2013) which plays another significant role in electromagnetic attenuation. The model can be expressed as , where |μr| and |εr| are the moduli of μr and εr, respectively. Besides, when the thickness of absorber satisfies above equation, the curves corresponding to wavelengths of λ/4 (crescent shape) can be observed (seen in Figure 6C).
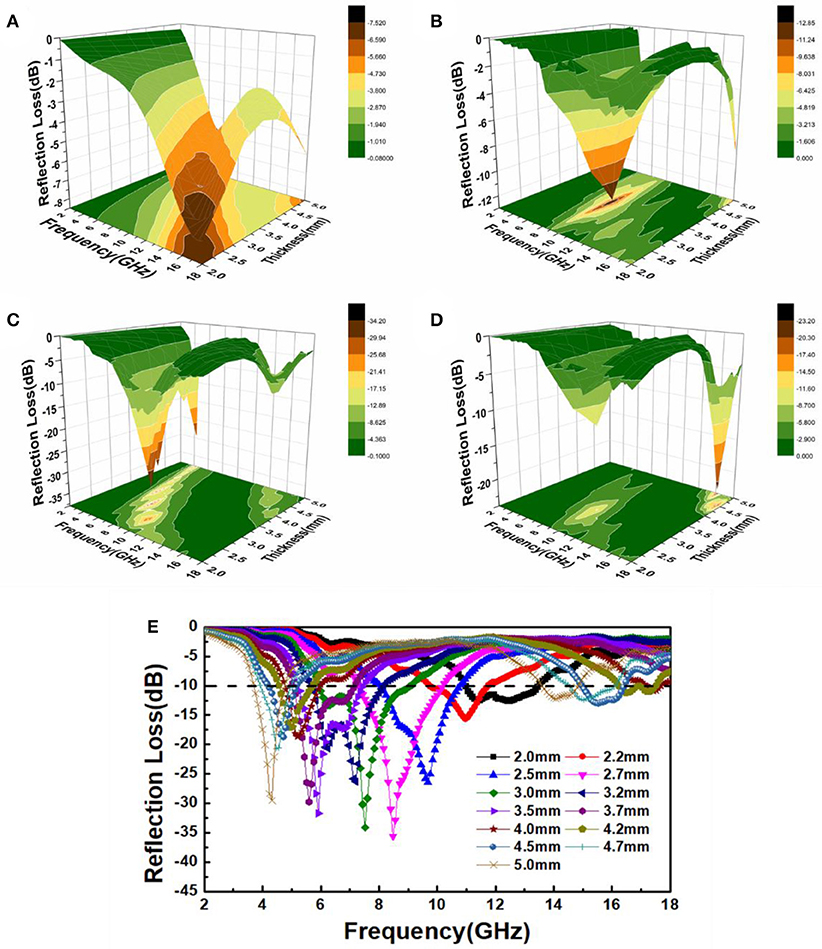
Figure 6. Three-dimensional presentations of the reflection loss for the NixSy composites with different filler loadings: (A) 10, (B) 20, (C) 30, and (D) 40 wt%. (E) RL curves for 30 wt% NixSy composites with different thickness.
Conclusions
In summary, we have successfully demonstrated an approach for the large-scale production of 1D porous NixSy nanostructures via solvothermal synthesis together with an annealing process. Through controlling the reaction temperature and time, products with uniform morphology are obtained. The results reveal that the interesting porous structure of NixSy might benefit the access of incident microwave and offer more active sites for multiple reflections and scattering, and thereby improve microwave absorbing performance. The minimum RL of −35.6 dB is achieved with a thickness of 2.7 mm at −35.6 dB GHz. The absorption bandwidth with RLs below −10 dB is up to 14.5 GHz when considering thicknesses of 2.0–5.0 mm. Combining the synergistic effect with intrinsic chemical properties and special structures, the NixSy nanorods are promising for utilization as MA materials in various fields, such as aeroplanes and spacecraft.
Author Contributions
ML designed experiments, carried out experiments, analyzed experimental results, and wrote the manuscript. QW, WX, H-YZ, and XD helped experimental result. X-HG gave guidance on the revision of the experimental plan and manuscript. G-SW and S-HD funded experimental topics.
Conflict of Interest Statement
The authors declare that the research was conducted in the absence of any commercial or financial relationships that could be construed as a potential conflict of interest.
Acknowledgments
This project was supported by the National Nature Science Foundation of China (No. 51472012), and the Fundamental Research Funds for the Central Universities.
Supplementary Material
The Supplementary Material for this article can be found online at: https://www.frontiersin.org/articles/10.3389/fchem.2018.00405/full#supplementary-material
References
Abbas, S. M., Chandra, M., Verma, A., Chatterjee, R., and Goel, T. C. (2006). Complex permittivity and microwave absorption properties of a composite dielectric absorber. Compos. A Appl. Sci. Manufact. 37, 2148–2154. doi: 10.1016/j.compositesa.2005.11.006
Deng, L. J., and Han, M. G. (2007). Microwave absorbing performances of multiwalled carbon nanotube composites with negative permeability. Appl. Phys. Lett. 91:023119. doi: 10.1063/1.2755875
He, S., Lu, C., Wang, G. S., Wang, J. W., Guo, H. Y., and Guo, L. (2014). Synthesis and growth mechanism of white-fungus-like nickel sulfide microspheres and their application in polymer composites with enhanced microwave-absorption properties. ChemPlusChem 79, 569–576. doi: 10.1002/cplu.201300435
He, S., Wang, G. S., Lu, C., Liu, J., Wen, B., Liu, H., et al. (2013). Enhanced wave absorption of nanocomposites based on the synthesized complex symmetrical CuS nanostructure and poly(vinylidene fluoride). J. Mater. Chem. A 1, 4685–4692. doi: 10.1039/c3ta00072a
Liu, J., Cao, M. S., Luo, Q., Shi, H. L., Wang, W. Z., and Yuan, J. (2016). Electromagnetic property and tunable microwave absorption of 3D nets from nickel chains at elevated temperature, ACS Appl. Mater. Interfaces 8, 22615–22622. doi: 10.1021/acsami.6b05480
Liu, J., Xu, J., Che, R., Chen, H., Liu, M., and Liu, Z. (2013). Hierarchical Fe3O4@TiO2 yolk-shell microspheres with enhanced microwave-absorption properties. Chem. A Eur. J. 19, 6746–6752. doi: 10.1002/chem.201203557
Liu, Q., Xu, X., Xia, W., Che, R., Chen, C., Cao, Q., et al. (2015). Dependency of magnetic microwave absorption on surface architecture of Co20Ni80 hierarchical structures studied by electron holography. Nanoscale 7, 1736–1743. doi: 10.1039/c4nr05547k
Liu, Q. L., Zhang, D., and Fan, T. X. (2008). Electromagnetic wave absorption properties of porous carbon/Co nanocomposites. Appl. Phys. Lett. 93:013110. doi: 10.1063/1.2957035
Luo, X., Wang, G. S., Guo, H. Y., Zhang, X. J., Cao, W. Q., Wei, Y. Z., et al. (2014). Enhanced wave-absorption properties of nanocomposites based on the synthesized Bi2S3 nanorods and polyvinylidene fluoride. ChemPlusChem 79, 1089–1095. doi: 10.1002/cplu.201402050
Lv, H., Liang, X., Cheng, Y., Zhang, H., Tang, D., Zhang, B., et al. (2015a). Coin-like α-Fe2O3@CoFe2O4 core–shell composites with excellent electromagnetic absorption performance. ACS Appl. Mater. Interfaces 7, 4744–4750. doi: 10.1021/am508438s
Lv, H., Liang, X., Ji, G., Zhang, H., and Du, Y. (2015b). Porous three-dimensional flower-like Co/CoO and its excellent electromagnetic absorption properties. ACS Appl. Mater. Interfaces 7, 9776–9783. doi: 10.1021/acsami.5b01654
Ning, M. Q., Lu, M. M., Li, J. B., Chen, Z., Dou, Y. K., Wang, C. Z., et al. (2015). Two-dimensional nanosheets of MoS2: a promising material with high dielectric properties and microwave absorption performance. Nanoscale 7:15734. doi: 10.1039/c5nr04670j
Qiang, R., Du, Y., Wang, Y., Wang, N., Tian, C., Ma, J., et al. (2016). Rational design of yolk-shell C@C microspheres for the effective enhancement in microwave absorption. Carbon 98, 599–606. doi: 10.1016/j.carbon.2015.11.054
Wang, B., Wei, J., Yang, Y., Wang, T., and Li, F. (2011). Investigation on peak frequency of the microwave absorption for carbonyl iron/epoxy resin composite. J. Magnet. Magnetic Mater. 323, 1101–1103. doi: 10.1016/j.jmmm.2010.12.028
Wang, G. S., Wu, Y. Y., Zhang, X. J., Li, Y., Guo, L., and Cao, M. S. (2014). Controllable synthesis of uniform ZnO nanorods and their enhanced dielectric and absorption properties. J. Mater. Chem. A 2, 8644–8651. doi: 10.1039/c4ta00485j
Wang, L., Xing, H., Gao, S., Ji, X., and Shen, Z. (2017). Porous flower-like NiO@graphene composites with superior microwave absorption properties. J. Mater. Chem. C 5, 2005–2014. doi: 10.1039/c6tc05179k
Wang, Y., Chen, D., Yin, X., Xu, P., Wu, F., and He, M. (2015). Hybrid of MoS2 and reduced graphene oxide: a lightweight and broadband electromagnetic wave absorber. ACS Appl. Mater. Interfaces 7, 26226–26234. doi: 10.1021/acsami.5b08410
Wang, Y., Wang, L., and Wu, H. (2013). Enhanced microwave absorption properties of α-Fe2O3-Filled ordered mesoporous carbon nanorods. Materials 6, 1520–1529. doi: 10.3390/ma6041520
Xu, W., Pan, Y. F., Wei, W., and Wang, G. S. (2018). Nanocomposites of oriented nickel chains with tunable magnetic properties for high-performance broadband microwave absorption. ACS Appl. Nano Mater. 1, 1116–1123. doi: 10.1021/acsanm.7b00293
Yan, D., Cheng, S., Zhuo, R. F., Chen, J. T., Feng, J. J., Feng, H. T., et al. (2009). Nanoparticles and 3D sponge-like porous networks of manganese oxides and their microwave absorption properties. Nanotechnology 20:105706. doi: 10.1088/0957-4484/20/10/105706
Yang, R., Wang, B., Xiang, J., Mu, C., Zhang, C., Wen, F., et al. (2017). Fabrication of NiCo2-anchored graphene nanosheets by liquid-phase exfoliation for excellent microwave absorbers. ACS Appl. Mater. Interfaces 9, 12673–12679. doi: 10.1021/acsami.6b16144
Yu, M., Liang, C., Liu, M., Liu, X., Yuan, K., Cao, H., et al. (2014). Yolk–shell Fe3O4@ZrO2 prepared by a tunable polymer surfactant assisted sol-gel method for high temperature stable microwave absorption. J. Mater. Chem. C 2, 7275–7283. doi: 10.1039/c4tcQ1285b
Yuan, K., Che, R., Cao, Q., Sun, Z., Yue, Q., and Deng, Y. (2015). Designed fabrication and characterization of three-dimensionally ordered arrays of core–shell magnetic mesoporous carbon microspheres. ACS Appl. Mater. Interfaces 7, 5312–5319. doi: 10.1021/am508683p
Zhang, C., Wang, B., Xiang, J., Su, C., Mu, C., Wen, F., et al. (2017). Microwave absorption properties of CoS2 nanocrystals embedded into reduced graphene oxide. ACS Appl. Mater. Interfaces 9, 28868–28875. doi: 10.1021/acsami.7b06982
Zhang, D. D., Zhao, D. L., Zhang, J. M., and Bai, L. Z. (2014). Microwave absorbing property and complex permittivity and permeability of graphene-CdS nanocomposite. J. Alloys Compounds 589, 378–383. doi: 10.1016/j.jallcom.2013.11.195
Zhang, X., Ji, G., Liu, W., Quan, B., Liang, X., Shang, C., et al. (2015). Thermal conversion of an Fe3O4@metal-organic framework: a new method for an efficient Fe-Co/nanoporous carbon microwave absorbing material. Nanoscale 7, 12932–12942. doi: 10.1039/c5nr03176a
Zhang, X. J., Wang, S. W., Wang, G. S., Li, Z., Guo, A. P., Zhu, J. Q., et al. (2017). Facile synthesis of NiS2@MoS2 core–shell nanospheres for effective enhancement in microwave absorption. RSC Adv. 7, 22454–22460. doi: 10.1039/c7ra03260a
Zhang, Y., Huang, Y., Zhang, T., Chang, H., Xiao, P., Chen, H., et al. (2015). Broadband and tunable high-performance microwave absorption of an ultralight and highly compressible graphene foam. Adv. Mater. 27, 2049–2053. doi: 10.1002/adma.201405788
Zhao, B., Guo, X., Zhao, W., Deng, J., Shao, G., Fan, B., et al. (2016). Yolk–Shell Ni@SnO2 composites with a designable interspace to improve the electromagnetic wave absorption properties. ACS Appl. Mater. Interfaces 8, 28917–28925. doi: 10.1021/acsami.6b10886
Zhao, B., Shao, G., Fan, B., Zhao, W., Xie, Y., and Zhang, R. (2015). Facile preparation and enhanced microwave absorption properties of core–shell composite spheres composited of Ni cores and TiO2 shells. Phys. Chem. Chem. Phys. 17, 8802–8810. doi: 10.1039/c4cp05632a
Zhao, H., Li, Z., Zhang, N., Du, Y., Li, S., Shao, L., et al. (2014). γ-irradiation induced one-step synthesis of electromagnetic functionalized reduced graphene oxide-Ni nanocomposites. RSC Adv. 4, 30467–30470. doi: 10.1039/c4ra05477f
Zhao, H. B., Fu, Z. B., Chen, H. B., Zhong, M. L., and Wang, C. Y. (2016). Excellent electromagnetic absorption capability of Ni/Carbon based conductive and magnetic foams synthesized via a green one pot route. ACS Appl. Mater. Interfaces 8, 1468–1477. doi: 10.1021/acsami.5b10805
Zhou, J. H., He, J. P., Li, G. X., Wang, T., Sun, D., Ding, X. C., et al. (2010). Direct incorporation of magnetic constituents within ordered mesoporous carbon-silica nanocomposites for highly efficient electromagnetic wave absorbers. J. Phys. Chem. C 114, 7611–7617. doi: 10.1021/jp911030n
Zhu, C. L., Zhang, M. L., Qiao, Y. J., Xiao, G., Zhang, F., and Chen, Y. J. (2010). Fe3O4/TiO2 core/shell nanotubes: synthesis and magnetic and electromagnetic wave absorption characteristics. J. Phys. Chem. C 114, 16229–16235. doi: 10.1021/jp104445m
Keywords: porous, nickel sulfide, one-dimensional, dielectric loss, microwave absorption
Citation: Lu M, Wu Q, Guan X-H, Xu W, Zhang H-Y, Di X, Wang G-S and Dong S-H (2018) Synthesis and Microwave Absorbing Properties of Porous One-Dimensional Nickel Sulfide Nanostructures. Front. Chem. 6:405. doi: 10.3389/fchem.2018.00405
Received: 30 June 2018; Accepted: 20 August 2018;
Published: 11 October 2018.
Edited by:
Wenbo Wang, Lanzhou Institute of Chemical Physics (CAS), ChinaReviewed by:
Zheng Ren, University of Connecticut, United StatesAiwei Tang, Beijing Jiaotong University, China
Copyright © 2018 Lu, Wu, Guan, Xu, Zhang, Di, Wang and Dong. This is an open-access article distributed under the terms of the Creative Commons Attribution License (CC BY). The use, distribution or reproduction in other forums is permitted, provided the original author(s) and the copyright owner(s) are credited and that the original publication in this journal is cited, in accordance with accepted academic practice. No use, distribution or reproduction is permitted which does not comply with these terms.
*Correspondence: Guang-Sheng Wang, wanggsh@buaa.edu.cn
Shao-Hua Dong, shdong@cup.edu.cn