- 1Department of Chemistry and Catalysis Research Center, Technical University of Munich, Garching, Germany
- 2Department of Pharmaceutical Biosciences, School of Pharmacy, University of Oslo, Oslo, Norway
- 3Department of Pharmaceutical Chemistry, School of Pharmacy, University of Oslo, Oslo, Norway
The biological activity of four pillarplex compounds featuring different metals and anions was investigated. The toxicity of the compounds against four bacterial strains [Bacillus subtilis (ATCC6633), Staphylococcus aureus (ATCC6538), Escherichia coli (UVI isolate), Pseudomonas aeruginosa], one fungus (Candida albicans), and a human cell line (HepG2) was determined. Additionally, a UV-Vis titration study of the pillarplexes was carried out to check for stability depending on pH- and chloride concentration changes and evaluate the applicability in physiological media. All compounds are bioactive: the silver compounds showed higher activity against bacteria and fungi, and the corresponding gold pillarplexes were less toxic against human cells.
Introduction
Since the early 2000s, coinage metal complexes featuring N-heterocyclic carbenes (NHC)—a ligand class with a facile tunability toward sterics, electronics, and solubility—have been employed as bioactive compounds (Herrmann, 2002; Mercs and Albrecht, 2010; Hopkinson et al., 2014). As first examples, silver (I) NHC complexes have been used as antimicrobial compounds, pioneered by Youngs et al. (Kascatan-Nebioglu et al., 2004; Melaiye et al., 2004), and a respective applicability of such compounds has been shown for a variety of complexes ever since (Figure 1) (Kascatan-Nebioglu et al., 2007; Hindi et al., 2009; Oehninger et al., 2013; Liang et al., 2018). Hereby, a slow release of silver ions originating from the decomposition of the NHC complexes is expected to be the cause of their activity, which can be rationalized by the comparably labile metal-carbene bond (with respect to other late transition metal-NHC bonds) (Kascatan-Nebioglu et al., 2007). The more stable gold (I) NHC complexes were also employed in studies investigating their antibiotic potential (Lazreg and Cazin, 2014). One possible target are (seleno)-cysteine moieties in proteins, e.g., thioredoxin reductase, accompanied by the inhibition of the enzyme, which is similar to the mode of action proposed for the approved metallodrug Auranofin (Baker et al., 2005; Schuh et al., 2012). This is expected in particular for gold(I) mono-carbene complexes, which can dissociate one (labile non-NHC) ligand to coordinate the sulfur or selenium atom (Rubbiani et al., 2011, 2013; Cheng et al., 2014; Meyer et al., 2014; Arambula et al., 2016; Bertrand et al., 2017; Karaca et al., 2017a; Schmidt et al., 2017; Zhang et al., 2018). In case of the di-NHC complexes, which are more stable toward dissociation, a different mode of action can be observed. Casini and coworkers were able to show stacking of Au(I) di-caffeine NHC complexes in G4 quadruplex DNA structures, inhibiting telomerase activity (Bertrand et al., 2014; Bazzicalupi et al., 2016; Karaca et al., 2017b). Hereby, the overall structure of the intact complex (being planar, cationic, and possessing a conjugated system for stacking) determines the ability to interact in a non-covalent binding, forming supramolecular aggregates. A related supramolecular recognition of biomolecules causing bioactivity was discovered by Michael Hannon and coworkers, who were using cylindrical metal helicates—a class of supramolecular coordination complexes (SCCs, Figure 1)—to interact with different DNA structures (Meistermann et al., 2002; Oleksi et al., 2006; Hannon, 2007; Ducani et al., 2010; Phongtongpasuk et al., 2013; Malina et al., 2016). They showed, that the overall charge of the compounds (4+) as well as the aromatic parts of the ligands were crucial for supramolecular recognition of the negatively charged DNA. In general, such supramolecular coordination compounds are discussed as a promising class for future applications as metallodrugs or drug delivery systems (Casini et al., 2017).
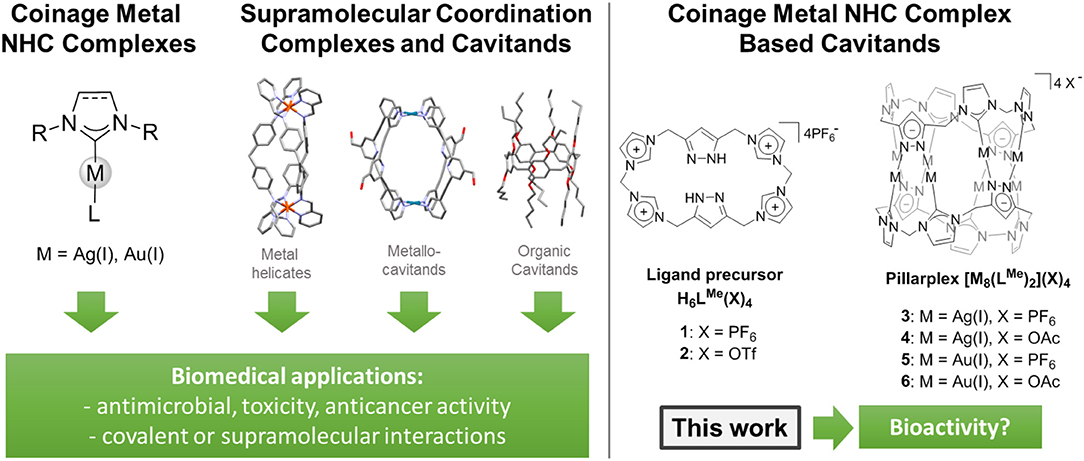
Figure 1. (Left) Overview of related known biomedical relevant compound classes. (Right) NHC based organometallocavitands including precursor investigated in this study.
We recently introduced the pillarplexes (Figure 1), a new family of SCCs which are structurally similar to Hannon's cylindrical helicates yet additionally exhibit a pore which allows for encapsulation of guest molecules inside the complex (Altmann and Pöthig, 2016). These compounds are octanuclear coinage metal complexes with two coordinating macrocyclic NHC ligands. Due to their in-built functionality (e.g., luminescence, easy tunable solubility) caused by the metal-complex character, the pillarplexes are even more versatile than their highly successful organic relatives—the pillararenes (Ogoshi et al., 2008, 2016). The latter have also been applied in biomedical applications very recently, for reducing cytotoxicity, and improving the anticancer bioactivity of oxaliplatin (Hao et al., 2018). In case of the pillararenes and metallocage systems (Casini et al., 2017), the cavitand itself shows no bioactivity and therefore can be used to modulate the selectivity and activity of an actual metallodrug.
Our pillarplexes combine the possibilities to behave like NHC complexes, i.e., as a metallodrug themselves, with the possible applications of cavitands. Therefore, to explore the future potential of our pillarplexes in the biomedical context, we conducted a toxicity study. We tested the antimicrobial activity of the four metal complexes (3–6), the metal salts as well as the ligand precursor salts (1, 2) toward four different bacterial strains [Bacillus subtilis (ATCC6633), Staphylococcus aureus (ATCC6538), Escherichia coli (UVI isolate), Pseudomonas aeruginosa] as well as one fungus (Candida albicans). We also evaluated the toxicity of the complexes toward a human cell line (HepG2) in order to clarify if related future research directions might be promising to follow. Finally, we conducted a stability study of the pillarplexes toward changes in pH and chloride ion concentration, which has implications on the use of the compounds under physiological conditions.
Experimental Section
General Details
Compounds 1–6 were prepared according to the reported procedures (Altmann et al., 2015; Altmann and Pöthig, 2016). Chemicals were purchased from commercial suppliers and used without further purification if not stated otherwise. Liquid NMR spectra were recorded on a Bruker Avance DPX 400 and a Bruker DRX 400 at room temperature if not stated otherwise. Chemical shifts are given in parts per million (ppm) and the spectra were referenced by using the residual solvent shift as internal standards. Emission spectra were recorded on a Agilent Cary 60 UV-Vis. Nutrient agar plates were prepared according to the instructions provided by Oxoid where 28 g of nutrient agar (CM0003) was needed to make 1 L of nutrient agar broth. 11.2 g of the agar was added to three 400 ml glass bottles. Four hundred milliliters of distilled water was added into each bottle containing the nutrient agar and was dissolved by stirring. After sterilization, the nutrient agar bottles were cooled to 50°C and then placed into a 50°C water bath for the temperature to remain constant. The nutrient agar was then poured halfway into 9 cm sterile petri dishes in HEPA filtered laminar flow cabinets to minimize the risk of contamination. The nutrient agar plates were then left to solidify and were refrigerated at 4°C. Mueller-Hinton agar plates were prepared from Mueller-Hinton agar medium (Sigma-Aldrich) and agar (Oxoid LP0011). Twenty-two grams of the Mueller-Hinton medium was added into 1 L of distilled water in a volumetric flask and dissolved with a magnetic flea at speed 6–7 and temperature 300°C for ~10 min (IKA Labortechnik). Fifteen grams of agar was added to the mixture and the stirring continued at speed 5–6 and at temperatures between 200 and 250°C until the mixture began to boil. After sterilization, the Mueller-Hinton broth was cooled to 50°C and stirred slightly with a magnetic flea for ~1 min (IKA Labortechnik). Sixty milliliters of the Mueller-Hinton broth was poured into each 13 cm petri dish using the media dispensing machine (IBS Integra Biosciences Technomat) using aseptic techniques. The Mueller-Hinton plates were allowed to cool and then stored in a refrigerator at 4°C. Plates were sterilized and stored at 4°C in the refrigerator before use. One liter of a 0.9% solution of sodium chloride was prepared and sterilized at 121°C for 20 min at 1 atm. The bacteria were streaked onto a nutrient agar plate using a sterile loop and incubated at 37°C overnight. The fungus was streaked using a sterile loop onto a TSA plate and incubated at 25°C for 48 h. Fresh streaks were prepared for each disc diffusion assay.
Disc Diffusion Assays for Antimicrobial Activity
Antimicrobial activity was measured using the disc diffusion assay essentially as described in guidelines from Clinical and Laboratory Standards Institute CLSI (2012). The bacteria were maintained on Nutrient agar (Oxoid), while the fungus was maintained on Sabouraud dextrose agar (Oxoid). An inoculum of the test microorganisms were made by resuspending freshly overnight grown colonies into 2 mL of a sterile salt solution (0.9% NaCl). The test organism was diluted to McFarland standard density no. 2 and mixing thoroughly (McFarland, 1907). For the Gram-negative bacteria and fungus, 60 μL of the inoculum was added to 25 mL of sterile salt solution, while 120 μL was added for the Gram-positive bacteria.
To prepare the plates for the disc diffusion assay Mueller-Hinton agar 2 (Sigma-Aldrich) were covered with 5 mL of the freshly made inoculate. The surplus inoculate was removed and the plates were then left in a laminar flow hood until the surface of the plates were completely dry.
Six millimeter filter discs were impregnated with 10 μL volume of the ligand precursor compounds 1 [L(PF6)4] and 2 [L(OTf)4], silver pillarplexes 3 [Ag8L2(PF6)4] and 4 [Ag8L2(OAc)4], and gold pillarplexes 5 [Au8L2(PF6)4] and 6 [Au8L2(OAc)4]. The concentration of the compounds used were 10 mM. Further filter discs were also impregnated with 10 μl of: dimethyl sulfoxide (DMSO) acting as a negative control; 10 mM of silver nitrate, 10 mM of gold chloride acting as model compounds for free metal ions; and antibiotic discs including pre-impregnated 30 mg/ml gentamycin sulfate discs (BD BBL Sensi-Disk) (E. coli, S. aureus, P. aeruginosa), pre-impregnated 30 mg/ml tetracycline discs (BD Sensi-Disc) (B. subtilis), and 10 mM of Miconazole nitrate discs (Sigma-Aldrich) (C. albicans), acting as positive controls. The filter discs were placed evenly on 13 cm Mueller-Hinton agar plates separated to avoid overlapping inhibitions zones. The plates were incubated overnight at 32°C for the bacteria or 25°C for the fungus. The inhibition zones were measured with a caliper. All experiment was performed at least three times.
In vitro Toxicity in HepG2 Liver Cells
Human hepatocarcinoma cell line HepG2 (HB-8065, ATCC, Manassas, VA, USA) was cultured in MEM-Glutamax (5.5 mM glucose) supplemented with 10% fetal bovine serum (Gibco, Life Technologies AG, Basel, Switzerland), 100 μg/mL streptomycin, and 100 units/mL penicillin (both from Gibco, Life Technologies AG, Basle, Switzerland). Cells were incubated at 37°C under a 5% CO2 atmosphere. For viability assays, cells were seeded in white 96-well Nunc plates at a density of 20,000 cells/well and left overnight to adhere before experiments were conducted.
The compounds were dissolved in DMSO at concentrations ranging from 10−3 to 10−6 M and were added to white 96-well plates (maximum DMSO concentration in wells was lower than 1%) containing 20,000 HepG2 cells/well. Plates were incubated for 24 h at 37°C in a 5% CO2 atmosphere. After 24 h, AlamarBlue cell viability reagent (Thermo Fisher, Carlsbad, CA, USA) was added as a 10% solution, and plates were placed back in the incubator for 4 h. AlamarBlue is a redox indicator yielding a fluorescence signal proportional to the number of viable cells in each well (O'Brien et al., 2003). The fluorescence signal was measured in a microplate reader (Clariostar, BMG Labtech, Ortenberg, Germany) at 550 nm/603 nm (excitation/ emission). Data from four replicates were used to calculate the half-maximal inhibitory concentration (IC50) using Sigmoidal, 4PL, where X is log(concentration) analysis, and a four-parameter logistic regression from GraphPad Prism 7 (GraphPad Software Inc., USA). The experiment was repeated twice with similar results.
UV-Vis Experiments
Stability Tests of Pillarplexes Against Chloride
The titrations of the silver pillarplex 4 and gold pillarplex 6 against chloride ions were carried out by stepwise addition of an increasing volume of a 3.072 M sodium chloride solution to 2 mL of a 1.38 · 10−5 M aqueous pillarplex solution followed by thorough mixing in a quartz cuvette. The UV-Vis absorption spectra were recorded immediately after the addition. The measured absorbance was corrected for the increase of the sample volume.
Stability Tests of Pillarplexes Against pH
The stability of silver pillarplex 4 and gold pillarplexes 6 in different concentrations of trifluoromethanesulfonic acid (HOTf) was monitored by UV-Vis spectroscopy. One milliliter of a 2.76 · 10−5 M aqueous pillarplex solution were injected into an equal volume of 1 mL HOTf solution with pH-values 2, 4, 5, and 6 in the quartz cuvette. The absorption spectra were recorded after 1 min, 1, 7, 24, 48, and 72 h (see Supplementary Material).
Results and Discussion
Antimicrobial Activity Studies
The results of the antimicrobial studies are summarized in Table 1.
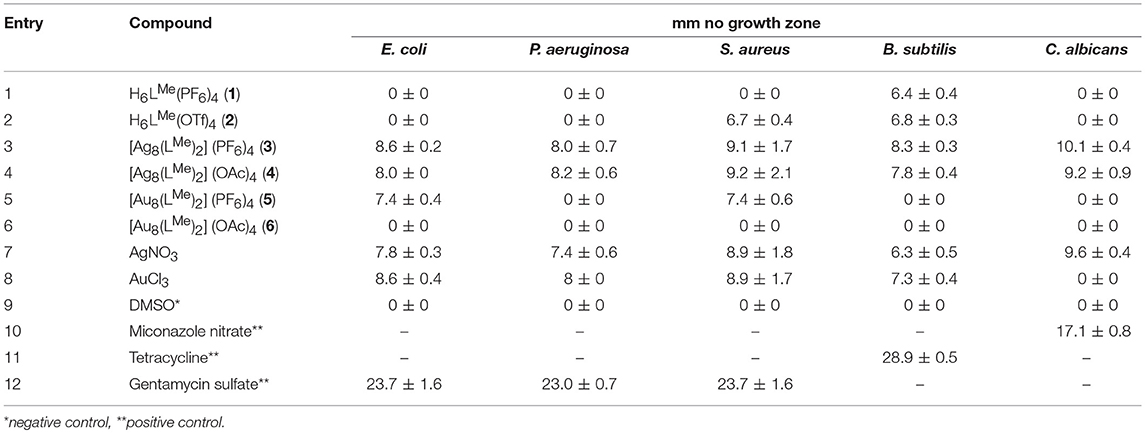
Table 1. Results from the disc diffusion assay for compounds 1–8 (10 mM) as well as reference substrates (30 mg/ml for tetracycline and gentamycine sulfate, and 10 mM for miconazole nitrate).
Both silver compounds (entries 3 and 4) show antimicrobial activity against all bacterial strains as well as the fungus. Hereby, the activity is independent of the anion present, as the results are identical within the margin of errors. In comparison to the positive controls (entries 10–12) the overall activity is moderate, however, (by means of statistic uncertainty) it is identical to that of AgNO3 (entry 7), which has been used as an antibiotic since ancient times (Danscher and Locht, 2010). Hence we suspect the release of silver ions via decomposition of the pillarplexes, which is in agreement with the general behavior of silver(I) NHC complexes, as stated above.
The gold pillarplexes show lower to no activity (entries 5 and 6). Compound 6, the completely water soluble acetate, shows no activity against any of the microbes, whereas, the more lipophilic compound 5 shows a selective moderate activity against Gram-negative E. coli and Gram-positive S. aureus. In contrast, AuCl3 shows activity against all bacterial strains (interestingly not against the fungus), which of course might be additionally influenced by the redox activity of the gold(III) ion. However, we suspect the gold pillarplexes being more stable in the physiological environment, therefore not releasing uncoordinated metal ions, which would explain the lower activity. Similarly, if the gold complexes would decompose, a similar toxicity as in case of the free ligand precursors would be expected. In general, such imidazolium salts are known to be potentially toxic, depending on different factors, e.g., lipophilicity or anions (Gravel and Schmitzer, 2017). In our case, the two macrocyclic polyimidazolium ligand precursors (entries 1 and 2) show only moderate and very selective toxicity only against the Gram-positive bacteria S. aureus and B. subtilis. Gram-positive bacteria lacks the outer membrane surrounding the cell wall. This outer membrane excludes, by various mechanisms, certain drugs from penetrating the bacterial cell (Hancock, 1997) and could be the reason for antimicrobial selectivity of compound 2. For the latter, no activity at all was observed in case of the gold pillarplexes, why we rule out a possible decomposition.
Cell Toxicity Studies
The results for the toxicity study of the compounds against human HepG2 liver cells are summarized in Table 2. The IC50-values were determined for all compounds, however, the silver pillarplexes (3 and 4) as well as AgNO3 and AuCl3 all showed precipitation to some degree. This can influence both uptake of the compounds by the cells and the absorbance read, resulting in ambiguous measurement results, which we have pointed out by an asterisk in Table 2.

Table 2. IC50-values for compounds 1–6 as well as reference salts against human liver cells (HepG2).
In general, all tested compounds exhibit biological activity. Both ligand precursors (1 and 2) exhibited low toxicity levels which corresponds to the determined IC50−values. In contrast, high cell toxicity was observed in concentration higher than 100 μM for all pillarplexes (see figures in the Supplementary Material). According to the determined IC50-values, the gold congeners are more active within the pairs of pillarplexes with the same anions (3 vs. 5 and 4 vs. 6). However, they also show a higher base RFU compared to the silver compounds, indicating the gold compounds to be less toxic. With regard to the effect of the anions, the more water-soluble compounds (2, 4, 6: triflates or acetates) show higher activity than the less water-soluble hexafluorophosphate salts (1, 3, 5). In general, the same trend as in the antimicrobial assay are observed with the HepG2 cells. The silver pillarplexes appear to be more toxic and more active than their gold counterparts. Precipitation was observed in case of the silver pillarplexes as well as for AgNO3 and AuCl3, whereas the gold pillarplexes did not exhibit any stability or solubility issues.
Stability Tests
To evaluate possible reasons for the observations made during the bacterial and cell tests, we conducted an UV-Vis titration study. In detail, we checked the influence of a varying chloride and proton concentration on the stability or solubility of the pillarplex compounds. Therefore, we first evaluated the absorption properties of the two water-soluble pillarplex acetates 4 and 6 in aqueous solution, as well as the ligand precursor (Figure 2A). All compounds absorb in the UV range: the silver complex 4 shows an absorption maximum at 226 nm whereas the gold complex 6 absorbs at 245 nm. The ligand precursor absorbs at 209 nm. The molar extinction coefficients for the pillarplex compounds at the wavelengths of the individual maximal absorption are 9.33 · 104 ± 6.41 · 102 M−1cm−1 (4) and 1.21 · 105 ± 4.64 · 103 M−1cm−1 (6).
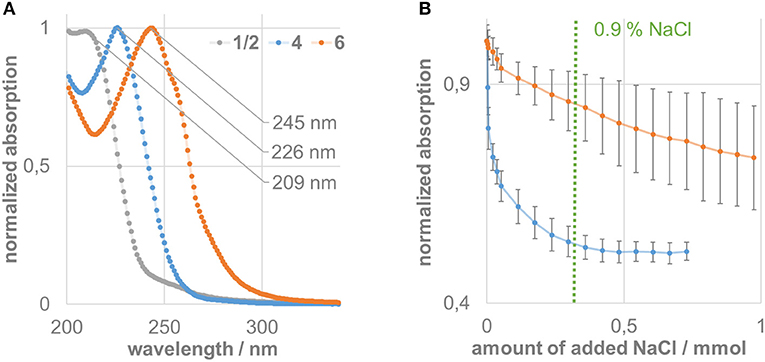
Figure 2. (A) Normalized UV-Vis absorption spectra of the ligand precursor (gray) and pillarplexes 4 (blue), and 6 (orange). (B) Titration experiment of compounds 4 (blue) and 6 (orange) against sodium chloride, showing the decay of the normalized signal at the individual absorption maximum (226 nm for 4, 245 nm for 6) including statistical uncertainties.
The titration results of the pillarplexes against an increasing amount of chloride ions present in aqueous solution show a very different behavior of the silver compared to the gold compound (Figure 2B). The absorption signal of silver complex 4 immediately drops up to addition of 0.5 mmol NaCl (which is about 17,000-fold excess of chloride). After that, no significant change can be observed in the absorption spectra upon addition of more equivalents of chloride. Apparently, this is close to the physiological concentration of chloride (0.9%) which might be a possible explanation why precipitation was observed in the biological tests for the silver containing pillarplexes. The gold compound 6 also shows a decay if the absorption signal upon chloride addition. However, the drop is less pronounced and at 0.9% chloride concentration, there is still a significant absorption (85% of the initial value). At higher chloride contents we observed a higher variation of the measured values, which we cannot explain up to now. However, even after addition of 1 mmol NaCl (about 35,000-fold excess) the characteristic absorption band at 245 nm can be observed for compound 6 (see Supplementary Material), strongly indicating that the gold complex is significantly less effected by chloride addition and still present in solution under physiological conditions.
Figure 3 shows the pH-dependent decay of pillarplex compounds 4 and 6 over time. From our previous work on pillarplex rotaxanes we already knew, that in case of silver, the metal ions can be released quickly in the presence of an excess of the strong trifluoromethanesulfonic acid (Altmann and Pöthig, 2017). This was reproduced also in case of the empty pillarplex 4 (Figure 3A) for which an immediate drop of absorption signal at 226 nm was observed at pH 2, indicating very fast decomposition to the protonated imidazolium precursor. The resulting UV-Vis spectrum is also in agreement to that measured for the ligand precursor (Figure 2A). At higher pH-values, the decomposition of 4 is significantly slower and almost identical for pH 4–6. A similar behavior was observed for the gold complex 6 although the decay at pH 2 is significantly slower than that of its silver analog (Figure 3B). Interestingly, at the higher pH-values the relative drop of the absorption signal is more pronounced compared to the silver complex. However, in case of 6 the resulting absorption spectrum after the assumed decomposition is not resembling that of the ligand precursor, and rather corresponds to the spectrum of 6 just with lower absorption intensity. Therefore, we additionally conducted a NMR experiment to check for protonation of the NHC ligands at pH 2. As a result, no protonated species was detected strongly indicating that the gold pillarplexes are stable even at low pH (see Supplementary Information Figure S15).
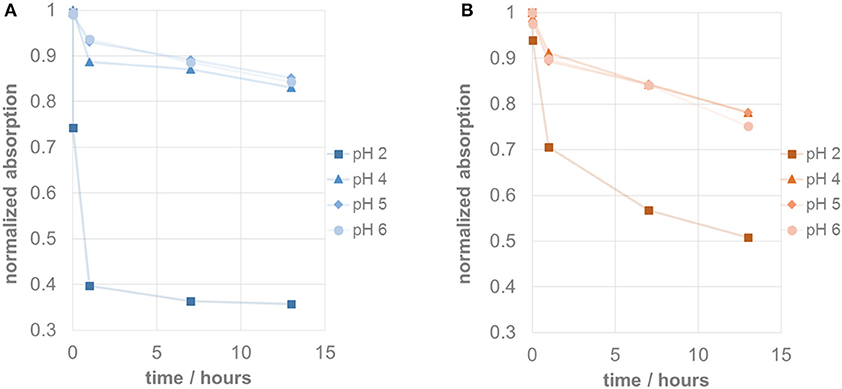
Figure 3. (A) Stability test at different pH-values showing decay of normalized absorption of silver pillarplex 4 (blue) at 226 nm over time. (B) Stability test at different pH-values showing decay of normalized absorption of gold pillarplex 6 (orange) at 245 nm over time.
Conclusion
In general, the silver pillarplexes behave like similar silver complexes and show antimicrobial and antifungal activity as well as moderate toxicity toward human HepG2 cells. The corresponding gold complexes were inactive against most bacterial strains and fungi, as well as had lower HepG2 toxicity. The observed effects originate most likely from the increased stability of the gold pillarplexes compared to the silver pillarplexes, as evident by the UV-Vis titration and the 1H NMR experiment. The fact that the gold complexes seem comparably non-toxic and stable opens up the possibility of them being used as drug carriers for selective drug delivery or modified release of drugs that could fit inside the cavity in the pillarplexes.
Author Contributions
AP: project conception and supervision, manuscript composition, and writing. PA: synthesis and characterization of pillarplexes. SG: synthesis and characterization of pillarplexes, UV-Vis studies. JK: UV-Vis studies. OH: biological testing supervision, data analysis, manuscript writing. SA biological testing, data analysis. HWL: biological testing, supervision, data analysis. AS: biological testing, data analysis. TG: biological testing supervision, data analysis.
Funding
This work was funded by FCI (AP), CSC (SG), TUM-GS (SG), and RCN (OH).
Conflict of interest statement
The authors declare that the research was conducted in the absence of any commercial or financial relationships that could be construed as a potential conflict of interest.
Acknowledgments
AP thanks the Fonds der chemischen Industrie (FCI) for funding of the project (Sachkostenzuschuss) as well as the Leonhard-Lorenz-Stiftung for financial support. SG thanks the CSC for a personal scholarship and the TUM Graduate School for financial support. OH thanks the Research Council of Norway (RCN) for a mobility grant (grant number: 240215).
Supplementary Material
The Supplementary Material for this article can be found online at: https://www.frontiersin.org/articles/10.3389/fchem.2018.00584/full#supplementary-material
Additional information on the IC50 determinations as well as the UV-Vis experiments is available as supplementary material.
References
Altmann, P. J, and Pöthig, A. (2017). A pH-dependent, mechanically interlocked switch: organometallic [2]rotaxane vs. organic [3]rotaxane. Angew. Chem. Int. Ed. 56, 15733–15736. doi: 10.1002/anie.201709921
Altmann, P. J., Jandl, C., and Pöthig, A. (2015). Introducing a pyrazole/imidazole based hybrid cyclophane: a hydrogen bond sensor and binucleating ligand precursor. Dalton Trans. 44, 11278–11281. doi: 10.1039/C5DT01775K
Altmann, P. J., and Pöthig, A. (2016). Pillarplexes: a metal–organic class of supramolecular hosts. J. Am. Chem. Soc. 138, 13171–13174. doi: 10.1021/jacs.6b08571
Arambula, J. F., McCall, R., Sidoran, K. J., Magda, D., Mitchell, N. A., Bielawski, C. W., et al. (2016). Targeting antioxidant pathways with ferrocenylated N-heterocyclic carbene supported gold(i) complexes in A549 lung cancer cells. Chem. Sci. 7, 1245–1256. doi: 10.1039/C5SC03519H
Baker, M. V., Barnard, P. J., Berners-Price, S. J., Brayshaw, S. K., Hickey, J. L., Skelton, B. W., et al. (2005). Synthesis and structural characterization of linear Au(I) N-heterocyclic carbene complexes: new analogues of the Au(I) phosphine drug auranofin. J. Organomet. Chem. 690, 5625–5635. doi: 10.1016/j.jorganchem.2005.07.013
Bazzicalupi, C., Ferraroni, M., Papi, F., Massai, L., Bertrand, B., Messori, L., et al. (2016). Determinants for tight and selective binding of a medicinal dicarbene gold(I) complex to a telomeric DNA G-quadruplex: a joint ESI MS and XRD investigation. Angew. Chem. Int. Ed. 55, 4256–4259. doi: 10.1002/anie.201511999
Bertrand, B., Romanov, A. S., Brooks, M., Davis, J., Schmidt, C., Ott, I., et al. (2017). Synthesis, structure and cytotoxicity of cyclic (alkyl)(amino) carbene and acyclic carbene complexes of group 11 metals. Dalton Trans. 46, 15875–15887. doi: 10.1039/C7DT03189K
Bertrand, B., Stefan, L., Pirrotta, M., Monchaud, D., Bodio, E., Richard, P., et al. (2014). Caffeine-based gold(I) N-heterocyclic carbenes as possible anticancer agents: synthesis and biological properties. Inorg. Chem. 53, 2296–2303. doi: 10.1021/ic403011h
Casini, A., Woods, B., and Wenzel, M. (2017). The promise of self-assembled 3D supramolecular coordination complexes for biomedical applications. Inorg. Chem. 56, 14715–14729. doi: 10.1021/acs.inorgchem.7b02599
Cheng, X., Holenya, P., Can, S., Alborzinia, H., Rubbiani, R., Ott, I., et al. (2014). A TrxR inhibiting gold(I) NHC complex induces apoptosis through ASK1-p38-MAPK signaling in pancreatic cancer cells. Mol. Cancer 13:221. doi: 10.1186/1476-4598-13-221
CLSI (2012). Methods for Dilution Antimicrobial Susceptibility Tests for Bacteria That Grow Aerobically; Approved Standard, 9th Edn. Wayne, PA: CLSI.
Danscher, G., and Locht, L. J. (2010). In vivo liberation of silver ions from metallic silver surfaces. Histochem. Cell Biol. 133, 359–366. doi: 10.1007/s00418-009-0670-5
Ducani, C., Leczkowska, A., Hodges, N. J., and Hannon, M. J. (2010). Noncovalent DNA-binding metallo-supramolecular cylinders prevent DNA transactions in vitro. Angew. Chem. Int. Ed. 49, 8942–8945. doi: 10.1002/anie.201004471
Gravel, J., and Schmitzer, A. R. (2017). Imidazolium and benzimidazolium-containing compounds: from simple toxic salts to highly bioactive drugs. Org. Biomol. Chem. 15, 1051–1071. doi: 10.1039/C6OB02293F
Hancock, R. E. (1997). The bacterial outer membrane as a drug barrier. Trends Microbiol. 5, 37–42. doi: 10.1016/S0966-842X(97)81773-8
Hannon, M. J. (2007). Supramolecular DNA recognition. Chem. Soc. Rev. 36, 280–295. doi: 10.1039/B606046N
Hao, Q., Chen, Y., Huang, Z., Xu, J.-F., Sun, Z., and Zhang, X. (2018). Supramolecular chemotherapy: carboxylated pillar[6]arene for decreasing cytotoxicity of oxaliplatin to normal cells and improving its anticancer bioactivity against colorectal cancer. ACS Appl. Mater. Interfaces 10, 5365–5372. doi: 10.1021/acsami.7b19784
Herrmann, W. A. (2002). N-heterocyclic carbenes: a new concept in organometallic catalysis. Angew. Chem. Int. Ed. 41, 1290–1309. doi: 10.1002/1521-3773(20020415)41:8<1290::AID-ANIE1290>3.0.CO;2-Y
Hindi, K. M., Panzner, M. J., Tessier, C. A., Cannon, C. L., and Youngs, W. J. (2009). The medicinal applications of imidazolium carbene-metal complexes. Chem. Rev. 109, 3859–3884. doi: 10.1021/cr800500u
Hopkinson, M. N., Richter, C., Schedler, M., and Glorius, F. (2014). An overview of N-heterocyclic carbenes. Nature 510, 485–496. doi: 10.1038/nature13384
Karaca, Ö., Meier-Menches, S. M., Casini, A., and Kuehn, F. E. (2017b). On the binding modes of metal NHC complexes with DNA secondary structures: implications for therapy and imaging. Chem. Commun. 53, 8249–8260. doi: 10.1039/C7CC03074F
Karaca, Ö., Scalcon, V., Meier-Menches, S. M., Bonsignore, R., Brouwer, J. M. J. L., Tonolo, F., et al. (2017a). Characterization of hydrophilic gold(I) N-heterocyclic carbene (NHC) complexes as potent TrxR inhibitors using biochemical and mass spectrometric approaches. Inorg. Chem. 56, 14237–14250. doi: 10.1021/acs.inorgchem.7b02345
Kascatan-Nebioglu, A., Panzner, M. J., Garrison, J. C., Tessier, C. A., and Youngs, W. J. (2004). Synthesis and structural characterization of N-heterocyclic carbene complexes of silver(I) and rhodium(I) from caffeine. Organometallics 23, 1928–1931. doi: 10.1021/om030689r
Kascatan-Nebioglu, A., Panzner, M. J., Tessier, C. A., Cannon, C. L., and Youngs, W. J. (2007). N-Heterocyclic carbene–silver complexes: a new class of antibiotics. Coord. Chem. Rev. 251, 884–895. doi: 10.1016/j.ccr.2006.08.019
Lazreg, F., and Cazin, C. S. J. (2014). “Medical applications of NHC-gold and -copper complexes,” in N-Heterocyclic Carbenes : Effective Tools for Organometallic Synthesis, ed S. Nolan (Weinheim: Wiley-VCH Verlag GmbH & Co. KGaA), 173–198.
Liang, X., Luan, S., Yin, Z., He, M., He, C., Yin, L., et al. (2018). Recent advances in the medical use of silver complex. Eur. J. Med. Chem. 157, 62–80. doi: 10.1016/j.ejmech.2018.07.057
Malina, J., Hannon, M. J., and Brabec, V. (2016). Iron(II) supramolecular helicates interfere with the HIV-1 Tat-TAR RNA interaction critical for viral replication. Sci. Rep. 6, 29674. doi: 10.1038/srep29674
McFarland, J. (1907). The nephelometer:an instrument for estimating the number of bacteria in suspensions used for calculating the opsonic index and for vaccines. J. Am. Med. Assoc. 49, 1176–1178. doi: 10.1001/jama.1907.25320140022001f
Meistermann, I., Moreno, V., Prieto, M. J., Moldrheim, E., Sletten, E., Khalid, S., et al. (2002). Intramolecular DNA coiling mediated by metallo-supramolecular cylinders: differential binding of P and M helical enantiomers. Proc. Natl. Acad. Sci. U.S.A. 99, 5069–5074. doi: 10.1073/pnas.062634499
Melaiye, A., Simons, R. S., Milsted, A., Pingitore, F., Wesdemiotis, C., Tessier, C. A., et al. (2004). Formation of water-soluble pincer silver(I)–carbene complexes: a novel antimicrobial agent. J. Med. Chem. 47, 973–977. doi: 10.1021/jm030262m
Mercs, L., and Albrecht, M. (2010). Beyond catalysis: N-heterocyclic carbene complexes as components for medicinal, luminescent, and functional materials applications. Chem. Soc. Rev. 39, 1903–1912. doi: 10.1039/b902238b
Meyer, A., Oehninger, L., Geldmacher, Y., Alborzinia, H., Woelfl, S., Sheldrick, W. S., et al. (2014). Gold(I) N-heterocyclic carbene complexes with naphthalimide ligands as combined thioredoxin reductase inhibitors and DNA intercalators. ChemMedChem 9, 1794–1800. doi: 10.1002/cmdc.201402049
O'Brien, J., Wilson, I., Orton, T., and Pognan, F. (2003). Investigation of the alamar blue (resazurin) fluorescent dye for the assessment of mammalian cell cytotoxicity. Eur. J. Biochem. 267, 5421–5426. doi: 10.1046/j.1432-1327.2000.01606.x
Oehninger, L., Rubbiani, R., and Ott, I. (2013). N-Heterocyclic carbene metal complexes in medicinal chemistry. Dalton Trans. 42, 3269–3284. doi: 10.1039/C2DT32617E
Ogoshi, T., Kanai, S., Fujinami, S., Yamagishi, T.-A., and Nakamoto, Y. (2008). para-Bridged symmetrical pillar[5]arenes: their lewis acid catalyzed synthesis and host–guest property. J. Am. Chem. Soc. 130, 5022–5023. doi: 10.1021/ja711260m
Ogoshi, T., Yamagishi, T. A., and Nakamoto, Y. (2016). Pillar-shaped macrocyclic hosts pillar[n]arenes: new key players for supramolecular chemistry. Chem. Rev. 116, 7937–8002. doi: 10.1021/acs.chemrev.5b00765
Oleksi, A., Blanco, A. G., Boer, R., Uson, I., Aymami, J., Rodger, A., et al. (2006). Molecular recognition of a three-way DNA junction by a metallosupramolecular helicate. Angew. Chem. Int. Ed. 45, 1227–1231. doi: 10.1002/anie.200503822
Phongtongpasuk, S., Paulus, S., Schnabl, J., Sigel, R. K. O., Spingler, B., Hannon, M. J., et al. (2013). Binding of a designed anti-cancer drug to the central cavity of an RNA three-way junction. Angew. Chem. Int. Ed. 52, 11513–11516. doi: 10.1002/anie.201305079
Rubbiani, R., Can, S., Kitanovic, I., Alborzinia, H., Stefanopoulou, M., Kokoschka, M., et al. (2011). Comparative in vitro evaluation of n-heterocyclic carbene gold(I) complexes of the benzimidazolylidene type. J. Med. Chem. 54, 8646–8657. doi: 10.1021/jm201220n
Rubbiani, R., Schuh, E., Meyer, A., Lemke, J., Wimberg, J., Metzler-Nolte, N., et al. (2013). TrxR inhibition and antiproliferative activities of structurally diverse gold N-heterocyclic carbene complexes. MedChemComm 4, 942–948. doi: 10.1039/c3md00076a
Schmidt, C., Karge, B., Misgeld, R., Prokop, A., Franke, R., Broenstrup, M., et al. (2017). Gold(I) NHC complexes: antiproliferative activity, cellular uptake, inhibition of mammalian and bacterial thioredoxin reductases, and gram-positive directed antibacterial effects. Chem. Eur. J. 23, 1869–1880. doi: 10.1002/chem.201604512
Schuh, E., Pfluger, C., Citta, A., Folda, A., Rigobello, M. P., Bindoli, A., et al. (2012). Gold(I) carbene complexes causing thioredoxin 1 and thioredoxin 2 oxidation as potential anticancer agents. J. Med. Chem. 55, 5518–5528. doi: 10.1021/jm300428v
Keywords: NHC, silver, gold, antimicrobial, cytotoxicity, organometallic, supramolecular, stability
Citation: Pöthig A, Ahmed S, Winther-Larsen HC, Guan S, Altmann PJ, Kudermann J, Santos Andresen AM, Gjøen T and Høgmoen Åstrand OA (2018) Antimicrobial Activity and Cytotoxicity of Ag(I) and Au(I) Pillarplexes. Front. Chem. 6:584. doi: 10.3389/fchem.2018.00584
Received: 30 September 2018; Accepted: 08 November 2018;
Published: 27 November 2018.
Edited by:
Angela Casini, Cardiff University, United KingdomReviewed by:
Zoe Pikramenou, University of Birmingham, United KingdomStephane Bellemin-Laponnaz, Centre National de la Recherche Scientifique (CNRS), France
Copyright © 2018 Pöthig, Ahmed, Winther-Larsen, Guan, Altmann, Kudermann, Santos Andresen, Gjøen and Høgmoen Åstrand. This is an open-access article distributed under the terms of the Creative Commons Attribution License (CC BY). The use, distribution or reproduction in other forums is permitted, provided the original author(s) and the copyright owner(s) are credited and that the original publication in this journal is cited, in accordance with accepted academic practice. No use, distribution or reproduction is permitted which does not comply with these terms.
*Correspondence: Alexander Pöthig, YWxleGFuZGVyLnBvZXRoaWdAdHVtLmRl
Ove Alexander Høgmoen Åstrand, by5hLmguYXN0cmFuZEBmYXJtYXNpLnVpby5ubw==