- Laboratory of Green Synthetic Organic Chemistry, Dipartimento di Chimica, Biologia e Biotecnologie, Università di Perugia, Perugia, Italy
Herein we report the use of Rhodiasolv© Polarclean as a novel polar aprotic solvent for the synthesis of decorated heterocycles via dipolar cycloaddition (isooxazoles) or intramolecular C–H functionalization processes (benzo-fused chromenes). The use of Polarclean allowed to isolate the final products in good yields by simple solid filtration or liquid-liquid phase separation, avoiding the need for chromatographic purification. Moreover, since in the synthesis of benzo-fused chromenes, the metal catalyst is retained in Polarclean, the catalyst/reaction medium can be easily reused for consecutive reaction runs, without any apparent loss in efficiency. This methodology is associated with a limited waste production. These results extend the applicability of Polarclean as a promising reaction medium for the replacement of toxic petrol-based solvent.
Introduction
Heterocyclic compounds are ubiquitous and find multiple applications in different fields of applied chemistry such as in medicinal chemistry, as key motifs in pharmaceutically active ingredients (Gomtsyan, 2012), and in material science (Yin and Shreeve, 2017). Thus, chemists have always been looking for novel synthetic methodologies that would allow to access heterocyclic cores in more efficient, economical and selective ways and, as a result, many efficient examples are available in the literature. The most effective and straightforward way to access heterocyclic cores is probably still represented by cycloaddition reactions (Heravi et al., 2015; Padwa and Bur, 2016). These reactions typically occur with perfect atom economy and, since they allow the simultaneous formations of two bonds, they are generally also very efficient in terms of step economy. One of the possible limitations of cycloadditions reactions is that often they have rather strict structural requirements on the substrates for the cycloaddition to occur, which results in the potential need for subsequent transformations to decorate the heterocyclic core and access the target molecule.
In recent years, great advancements in transition metals catalyzed reactions provided synthetic organic chemists with many more tools to efficiently obtain heterocyclic molecules (Gulevich et al., 2013). In particular, the last decade saw enormous improvements in the available methodologies to activate and directly functionalize C–H bonds, and many of these methodologies indeed are specifically directed toward the synthesis of heterocycles (Thansandote and Lautens, 2009; Mei et al., 2012; Inamoto, 2013). However, these reactions typically require hazardous conditions and the use of common toxic organic solvents and suffer from procedural limitations such as the need of strictly anhydrous conditions. The need to develop more sustainable procedures for chemical production has recently brought some results also in the realm of C–H functionalization methodologies, particularly in the use of recoverable and reusable catalysts (Santoro et al., 2016) and of benign bio-based reaction media (Santoro et al., 2017, 2018). In fact, waste disposal represents one of the major issues related to chemical productions due to economic and environmental reasons. Increasingly stringent regulations impose strict limitations on the use of toxic organic solvents and more in general to the large use of potentially harmful substances and volatile organic compounds. Solvents constitute the largest portion of the waste associated to a chemical process and the prime responsible for the related CO2 emissions (Bruntland's report the World Commission on Environmental Development, 1987; Pollution Prevention Act, 1990; Anastas and Warner, 1998; Jiménez-González et al., 2004; Jimenez-Gonzalez et al., 2011).
As a part of our research program devoted to the search for novel environmentally benign reaction media, we are interested in the use of sustainable green solvents in the synthesis and functionalization of heterocycle systems (Rasina et al., 2016; Tian et al., 2016; Ferlin et al., 2017, 2018a; Bechtoldt et al., 2018; Vaccaro et al., 2018). In this context, we have recently reported the use of Rhodiasolv© Polarclean as an efficient system for the waste-minimized synthesis of fully decorated 1,2,3-triazoles (Luciani et al., 2018). Rhodiasolv© Polarclean, is composed by methyl-5-(dimethylamino)-2-methyl-5-oxopentanoate and its diamide derivative in a 20:1 ratio. It is commercially available and finds application as a solvent, co-solvent, or crystal growth inhibitor in agrochemical formulations (Vidal, 2012). It is miscible with water and has a boiling point of 278–282°C and a melting point of −60°C. Polarclean is industrially produced from methyleneglutarodinitrile (MDN), a by-product of Nylon-66 manufacturing, otherwise needed to be burnt to be disposed (Vidal, 2012). To the best of our knowledge Polarclean has been rarely used as a reaction medium and it has been tested among other solvents in metathesis polymerization (Lebarbé et al., 2014), olefin epoxidation (Mouret et al., 2014), and fiber membranes fabrication (Hassankiadeh et al., 2015).
In this contribution, we report our results on the use of Polarclean for the synthesis of widely interesting heterocyclic such as isoxazoles and polycyclic fused 1,2,3-triazoles. These heterocyclic systems are rather common and, for instance, triazole moiety is present in active pharmaceutical ingredients (Wu et al., 2018) as well as in optoelectronics and material sciences (Marrocchi et al., 2016). Isoxazoles are recognized as privilege structures for the synthesis of beta-lactamase resistant antibiotics (Decuyper et al., 2018), and recently they found application in the field of lithium ion batteries (Yang et al., 2017).
Our investigations were directed toward the definition of protocols featuring recycle and reuse of solvent/catalyst systems, avoidance of wasteful chromatographic purification, and therefore minimization of waste production (Figure 1).
Results and Discussion
We started our investigation by testing the use of Polarclean in the representative reaction of phenylacetylene (1a) with 4-bromo-N-hydroxybenzimidoyl chloride (2a), using 2 mol% of CuSO4·5H2O as copper source together with 10 mol% of sodium ascorbate as a reductant (Himo et al., 2005) (Table 1). The reaction was tested at 70°C for 24 h in Polarclean 1 M as medium and the corresponding isoxazole 3a was obtained in 40% yield (Table 1, entry 1). In this case, due to high solubility of 3a in pure Polarclean, the pure product could only be isolated after a classic purification procedure (aqueous work-up followed by column chromatography). Slightly better results were achieved when a 9:1 mixture of Polarclean/water was used as medium at 70°C (Table 1, entry 2). In these conditions, the reaction mixture was partially heterogeneous and product 3a precipitated while forming and could be isolated in 50% yield by simple filtration. Increasing the amount of water by using a 4:1 Polarclean/H2O mixture lead to a further improvement in reaction yield, which reached 60% (Table 1, entry 3). An attempt to further increase the amount of water relative to the substrates and product while keeping the 4:1 Polarclean/H2O ratio, thus performing the reaction at 0.5 M concentration, resulted in drastically lower yield (33%, Table 1, entry 4). Finally, optimal results were obtained when Polarclean and water were used in 4:1 ratio at 1 M concentration and at 50°C (Table 1, entry 5). In these conditions in fact pure product 3a could be obtained in 70% yield by simple filtration as it precipitates in the reaction mixture. The beneficial effect of lowering the temperature can possibly be attributed to a reduced degradation of imidoyl chloride 2a.
The identified optimal reaction conditions were then applied to investigate the substrate scope. The protocol worked smoothly using combinations of aryl- or alkyl-substituted alkynes in combination with imidoyl chloride 2a, affording the products in good yields (Scheme 1). Importantly, the presence of halogen substituents on the aromatic rings was well-tolerated, potentially allowing for late stage transformations of these functionalities. Very importantly, the final work-up for the synthesized products was consistent with our initial intent. In fact, the products were insoluble in the reaction media and in all cases precipitated at the end of the reaction, thus allowing a very easy isolation by filtration and washing with water to remove solvent impurities.
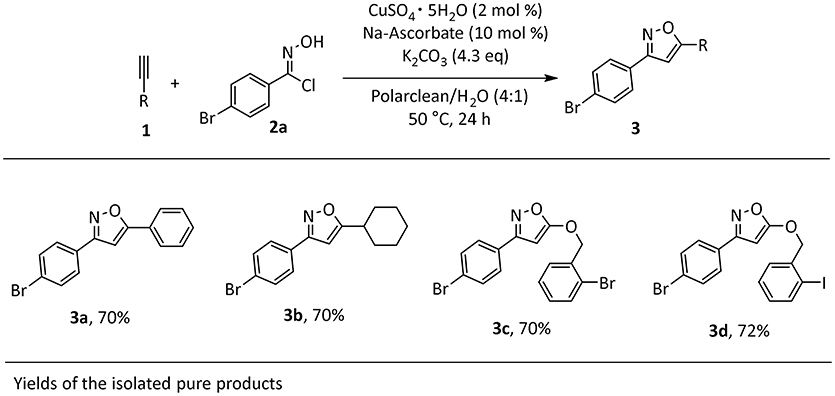
Scheme 1. Scope of isoxazole 3a-da. aReaction conditions: 1a (1 mmol), 2a (1 mmol), CuSO4 pentahydrate (2 mol%), Na-Ascorbate (10 mol %), K2CO3 (4.3 equivalent), Polarclean/H2O (4:1) 1 M, 1mL.
Next, we began our investigation on the use of Polarclean as reaction medium in the cyclization reaction by C–H functionalization of 1,2,3-triazole 4a (Table 2). Starting from our experience in this transformation (Ferlin et al., 2018b), which suggested the use of simple Pd(OAc)2 as catalyst with a substoichiometric amount of 2,4,6-trimethylbenzoic acid as additive and potassium carbonate as base, we performed the reaction using Polarclean, pure or in combination with different amount of water, as reaction medium (Table 2).
In this process, the best selectivity was found when pure Polarclean was used as reaction medium (Table 2, entry 3). In fact, the presence of water influenced dramatically the final composition of the reaction mixture, favoring the formation of the de-halogenated side-product 6a (Table 2, entries 1 and 2). With the optimized reaction conditions, we further explored the scope of this process (Scheme 2). A wide range of substrates could be employed in the intramolecular C–H arylation of 1,2-3-triazole-based substrates 4, giving access to either triazolo-fused chromenes or triazolo-fused isoindoles, depending on the substitution pattern on the triazole substrate. The reaction is compatible with the presence of an oxygen atom in the side-chain, giving rapid access to benzo-fused chromene 5a in 87% yield. Aryl- or alkyl-substituted [1,2,3]-triazolo[5,1-a]isoindoles 5b, 5c, and 5e could also be obtained in good yields (78–84%). To our delight, the optimized reaction conditions proved effective on more complex substrates, giving access both to triazolo-fused benzazepine 5d and to the steroid-substituted [1,2,3]-triazolo[5,1-a]isoindoles 5f in 68 and 52% yields, respectively. In some cases (5b, 5d, and 5f) the formation of traces amount of dehalogenation products (<2%) was observed.
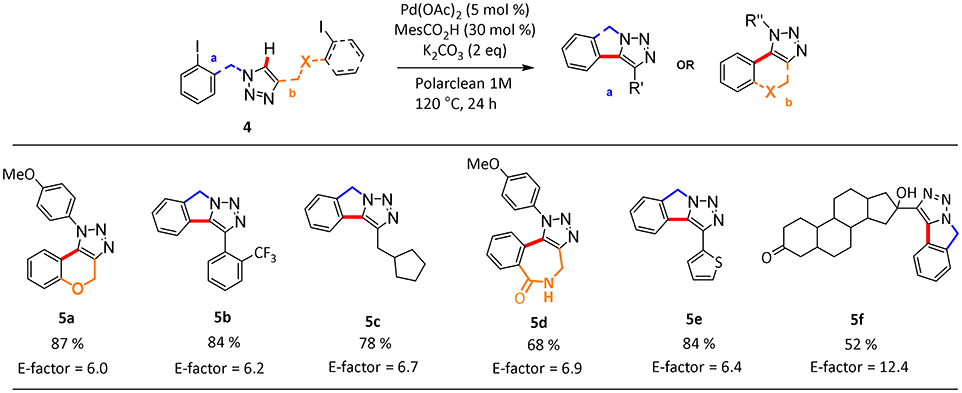
Scheme 2. Scope of hetero-fused triazoles 5a-fa. aData reported refer to the isolated yield of the pure product. aReaction conditions: 4a (1 mmol), MesCO2H (30 mol %, 0.3 mmol), K2CO3 (2 equivalent, 2 mmol), Pd(OAc)2 (5 mol %, 0.05 mmol), Polarclean 1 mL, 1 M.
Also in this case the isolation of the product was conducted via re-crystallization without the need for further purification, except in the case of the steroid product 5f in which filtration over a silica pad and precipitation in water were necessary to achieve the pure compound. Finally, we also investigated the recycle and reuse of the solvent/catalyst system (Table 3).
We found that for almost all of the substrates it was possible to filtrate the reaction mixture on a Büchner funnel, collecting the product, and reuse the solvent system, which also retains the palladium catalyst, without any treatment for at least three consecutive cycles and with a limited loss in efficiency and selectivity (Table 3). The latter is likely caused by an increase in water content of the solvent/catalyst system over consecutive reaction runs, which was already demonstrated to be detrimental for the selectivity of the process.
We also calculated the green metrics associated with the C–H functionalization protocol to compare the results of the reactions conducted in Polarclean with those obtained using other media. We were pleased to find that, compared to other common synthetic protocols present in literature (see Supplementary Information), the use of our recyclable system for the intramolecular C–H activation allows us to achieve very low E-factor values around 6 for the synthesis of polycyclic heterocycles (Scheme 2). The only exception is represented by the cyclization of the steroid substituted substrate to give product 5f, for which recycling of the solvent/catalyst system was hampered by the necessity to add water to isolate the pure product.
Conclusion
In conclusion, we have reported that Polarclean, a novel solvent deriving from the waste valorization of Nylon 66 manufacturing, can be an effective alternative to common petrol-based solvents in the reactions object of the current investigation. Dipolar cycloadditions benefit from the use of Polarclean in terms of isolation of final products and therefore in achieving a waste minimized protocol for the synthesis of isoxazole 3. Intramolecular C–H activation also proved to be feasible using Polarclean allowing the synthesis of polycyclic heterocycles 5 in a step and atom economical fashion and with the reuse of the medium/catalyst system, thus effectively minimizing the waste generation.
Author Contributions
FF, LL, OV, FB, OP, and SS performed the experiments. FF and LV contributed to conception and design of the study. SS and LV wrote the manuscript. All authors contributed to manuscript revision, read and approved the submitted version.
Funding
MIUR Dipartimenti di Eccellenza-2018–2022: AMIS.
Conflict of Interest Statement
The authors declare that the research was conducted in the absence of any commercial or financial relationships that could be construed as a potential conflict of interest.
Acknowledgments
We gratefully acknowledge the Università degli Studi di Perugia and MIUR for financial support to the project AMIS, through the program Dipartimenti di Eccellenza-2018–2022. Solvay (Rhodiasolv©) is acknowledged for free samples of Polarclean.
Supplementary Material
The Supplementary Material for this article can be found online at: https://www.frontiersin.org/articles/10.3389/fchem.2018.00659/full#supplementary-material
References
Anastas, P. T., and Warner, J. C. (1998). Green Chemistry: Theory and Practice. New York, NY: Oxford University Press.
Bechtoldt, A., Baumert, M. E., Vaccaro, L., and Ackermann, L. (2018). Ruthenium(II) oxidase catalysis for C–H alkenylations in biomass-derived γ-valerolactone. Green Chem. 20, 398–402. doi: 10.1039/C7GC03353B
Bruntland's report the World Commission on Environmental and Development (1987). World Commission on Environmental and, Development: Our Common Future. Oxford: University Press.
Decuyper, L., Jukič, M., Sosič, I., Žula, A., D'hooghe, M., and Gobec, S. (2018). Antibacterial and β-lactamase inhibitory activity of monocyclic β-lactams. Med. Res. Rev. 38, 426–503. doi: 10.1002/med.21443
Ferlin, F., Luciani, L., Santoro, S., Marrocchi, A., Lanari, D., Bechtoldt, A., et al. (2018a). A continuous flow approach for the C–H functionalization of 1,2,3-triazoles in γ-valerolactone as a biomass-derived medium. Green Chem. 20, 2888–2893. doi: 10.1039/C8GC01115J
Ferlin, F., Santoro, S., Ackermann, L., and Vaccaro, L. (2017). Heterogeneous C–H alkenylations in continuous-flow: oxidative palladium-catalysis in a biomass-derived reaction medium. Green Chem. 19, 2510–2514. doi: 10.1039/C7GC01103B
Ferlin, F., Trombettoni, V., Luciani, L., Fusi, S., Piermatti, O., Santoro, S., et al. (2018b). A waste-minimized protocol for copper-catalyzed Ullmann-type reaction in a biomass derived furfuryl alcohol/water azeotrope. Green Chem. 20, 1634–1639. doi: 10.1039/C8GC00287H
Gomtsyan, A. (2012). Heterocycles in drugs and drug discovery. Chem. Heterocycl. Comp. 48, 7–10. doi: 10.1007/s10593-012-0960-z
Gulevich, A. V., Dudnik, A. S., Chernyak, N., and Gevorgyan, V. (2013). Transition metal-mediated synthesis of monocyclic aromatic heterocycles. Chem. Rev. 113, 3084–3213. doi: 10.1021/cr300333u
Hassankiadeh, N. T., Cui, Z., Kim, J. H., Shin, D. W., Lee, S. Y., Sanguineti, A., et al. (2015). Microporous poly(vinylidene fluoride) hollow fiber membranes fabricated with PolarClean as water-soluble green diluent and additives. J. Membr. Sci. 479, 204–212. doi: 10.1016/j.memsci.2015.01.031
Heravi, M. M., Ahmadi, T., Ghavidel, M., Heidari, B., and Hamidi, H. (2015). Recent applications of the hetero diels–alder reaction in the total synthesis of natural products. RSC Adv. 5, 101999–102075. doi: 10.1039/C5RA17488K
Himo, F., Lovell, T., Hilgraf, R., Rostovtsev, V. V., Noodleman, L., Sharpless, K. B., et al. (2005). Copper(I)-catalyzed synthesis of azoles. DFT study predicts unprecedented reactivity and intermediates. J. Am. Chem. Soc. 127, 210–216. doi: 10.1021/ja0471525
Inamoto, K. (2013). Synthesis of heterocyclic compounds through palladium-catalyzed C–H cyclization processes. Chem. Pharm. Bull. 61, 987–996. doi: 10.1248/cpb.c13-00420
Jiménez-González, C., Curzons, A. D., Constable, D. J. C., and Cunningham, V. L. (2004). Cradle-to-gate life cycle inventory and assessment of pharmaceutical compounds. Int. J. Life Cicle Assess. 9, 114–121. doi: 10.1007/BF02978570
Jimenez-Gonzalez, C., Ponder, C. S., Broxterman, Q. B., and Manley, J. B. (2011). Using the right green yardstick: why process mass intensity is used in the pharmaceutical industry to drive more sustainable processes. Org. Process Res. Dev. 15, 912–917. doi: 10.1021/op200097d
Lebarbé, T., More, A. S., Sane, P. S., Grau, E., Alfos, C., and Cramail, H. (2014). Bio-based aliphatic polyurethanes through ADMET polymerization in bulk and green solvent. Macromol. Rapid Commun. 35, 479–483. doi: 10.1002/marc.201300695
Luciani, L., Goff, E., Lanari, D., Santoro, S., and Vaccaro, L. (2018). Waste-minimised copper-catalysed azide–alkyne cycloaddition in polarclean as a reusable and safe reaction medium. Green Chem. 20, 183–187. doi: 10.1039/C7GC03022C
Marrocchi, A., Facchetti, A., Lanari, D., Santoro, S., and Vaccaro, L. (2016). Click-chemistry approaches to π-conjugated polymers for organic electronics applications. Chem. Sci. 7, 6298–6308. doi: 10.1039/C6SC01832G
Mei, T.-S., Kou, L., Ma, S., Engle, K. M., and Yu, J.-Q. (2012). Heterocycle formation via palladium-catalyzed C–H functionalization. Synthesis 44, 1778–1791. doi: 10.1055/s-0031-1289766
Mouret, A., Leclercq, L., Mühlbauer, A., and Nardello-Rataj, V. (2014). Eco-friendly solvents and amphiphilic catalytic polyoxometalate nanoparticles: a winning combination for olefin epoxidation. Green Chem. 16, 269–278. doi: 10.1039/C3GC42032A
Padwa, A., and Bur, S. (2016). Recent advances of 1,3-dipolar cycloaddition chemistry for alkaloid synthesis. Adv. Heterocycl. Chem. 119, 241–305. doi: 10.1016/bs.aihch.2016.03.005
Pollution Prevention Act (1990). Pollution Prevention Act. Washington, DC: US Government Printing Office, 617.
Rasina, D., Lombi, A., Santoro, S., Ferlin, F., and Vaccaro, L. (2016). Searching for novel reusable biomass-derived solvents: furfuryl alcohol/water azeotrope as a medium for waste-minimised copper-catalysed azide–alkyne cycloaddition. Green Chem. 18, 6380–6386. doi: 10.1039/C6GC01941B
Santoro, S., Ferlin, F., Luciani, L., Ackermann, L., and Vaccaro, L. (2017). Biomass-derived solvents as effective media for cross-coupling reactions and C–H functionalization processes. Green Chem. 19, 1601–1612. doi: 10.1039/c7gc00067g
Santoro, S., Kozhushkov, S. I., Ackermann, L., and Vaccaro, L. (2016). Heterogeneous catalytic approaches in C–H activation reactions. Green Chem. 18, 3471–3493. doi: 10.1039/c6gc00385k
Santoro, S., Marrocchi, A., Lanari, D., Ackermann, L., and Vaccaro, L. (2018). Towards sustainable C–H functionalization reactions: the emerging role of bio-based reaction media. Chem. Eur. J. 24, 13383–13390. doi: 10.1002/chem.201801114
Thansandote, P., and Lautens, M. (2009). Construction of nitrogen-containing heterocycles by C–H bond functionalization. Chem. Eur. J. 15, 5874–5883. doi: 10.1002/chem.200900281
Tian, X., Yang, F., Rasina, D., Bauer, M., Warratz, S., Ferlin, F., et al. (2016). C–H arylations of 1,2,3-triazoles by reusable heterogeneous palladium catalysts in biomass-derived γ-valerolactone. Chem. Commun. 52, 9777–9780. doi: 10.1039/C6CC03468C
Vaccaro, L., Curini, M., Ferlin, F., Lanari, D., Marrocchi, A., Piermatti, O., et al. (2018). Definition of green synthetic tools based on safer reaction media, heterogeneous catalysis, and flow technology. Pure Appl. Chem. 90, 21–33. doi: 10.1515/pac-2017-0409
Vidal, T. (2012). Sustainable Solvents, Products and Process Innovations. RSC Symposium. Available online at: http://www.rscspecialitychemicals.org.uk/docs/rsc-symposium/Sustainable-Solvents-Products-and-Process-Innovations_Thierry-Vidal_-RSC-Symposium-2012.pdf (Accessed September 2017).
Wu, G., Gao, Y., Kang, D., Huang, B., Huo, Z., Liu, H., et al. (2018). Design, synthesis and biological evaluation of tacrine-1,2,3-triazole derivatives as potent cholinesterase inhibitors. Med. Chem. Commun. 9, 149–159. doi: 10.1039/C7MD00457E
Yang, S.-J., Qin, X.-Y., He, R., Shen, W., Li, M., and Zhao, L.-B. (2017). A density functional theory study on the thermodynamic and dynamic properties of anthraquinone analogue cathode materials for rechargeable lithium ion batteries. Phys. Chem. Chem. Phys. 19, 12480–12489. doi: 10.1039/C7CP01203A
Keywords: heterocycles, green-synthesis, isoxazoles, triazoles, C–H activation
Citation: Ferlin F, Luciani L, Viteritti O, Brunori F, Piermatti O, Santoro S and Vaccaro L (2019) Polarclean as a Sustainable Reaction Medium for the Waste Minimized Synthesis of Heterocyclic Compounds. Front. Chem. 6:659. doi: 10.3389/fchem.2018.00659
Received: 18 September 2018; Accepted: 18 December 2018;
Published: 29 January 2019.
Edited by:
Manoj B. Gawande, Palacky University Olomouc, CzechiaReviewed by:
Rafael Luque, Universidad de Córdoba, SpainFabio Arico', Università Ca' Foscari, Italy
Copyright © 2019 Ferlin, Luciani, Viteritti, Brunori, Piermatti, Santoro and Vaccaro. This is an open-access article distributed under the terms of the Creative Commons Attribution License (CC BY). The use, distribution or reproduction in other forums is permitted, provided the original author(s) and the copyright owner(s) are credited and that the original publication in this journal is cited, in accordance with accepted academic practice. No use, distribution or reproduction is permitted which does not comply with these terms.
*Correspondence: Luigi Vaccaro, bHVpZ2kudmFjY2Fyb0B1bmlwZy5pdA==