- School of Chemistry, Cardiff University, Cardiff, United Kingdom
Within the framework of designing new self-assembled metallosupramolecular architectures for drug delivery, seven [Pd2L4]4+ metallacages (L = 2,6-bis(pyridine-3-ylethynyl)pyridine) featuring different groups in exo-position, selected to enhance the cage solubility in aqueous environment, were synthesized. Thus, carboxylic acids, sugars, and PEG groups were tethered to the bispyridyl ligands directly or via disulfide bond formation, as well as via click chemistry. The ligands and respective cages were characterized by different methods, including NMR spectroscopy and high-resolution electrospray mass spectrometry (HR-ESI-MS). While the two ligands featuring carboxylic acid-functionalized groups showed improved solubility in water, the other ligands were soluble only in organic solvents. Unfortunately, all the respective self-assembled cages were also insoluble in water. Afterwards, the encapsulation properties of the anticancer drug cisplatin in selected [Pd2L4]X4 cages (X = NO, BF) were studied by 1H, 1H DOSY, and 195Pt NMR spectroscopy. The effect of the counter ions as well as of the polarity of the solvent in the drug encapsulation process were also investigated, and provided useful information on the host-guest properties of these experimental drug delivery systems. Our results provide further experimental support for previous studies that suggest the desolvation of guests from surrounding solvent molecules and the resulting solvent rearrangement may actually be the primary driving force for determining guest binding affinities in metallacages, in the absence of specific functional group interactions.
Introduction
Supramolecular chemistry has its roots in biology, and in recent years the unique and often advantageous properties of supramolecular materials have led to their extensive exploration in the fields of biomolecular recognition, drug delivery, disease diagnosis, and imaging. In this context, supramolecular coordination complexes (SCCs) hold great promise (Cook et al., 2013; Casini et al., 2017). In fact, the number of reports on the bioactivity of three-dimensional (3D) SCCs with different shapes has substantially increased (Kaner et al., 2016; Preston et al., 2016; Casini et al., 2017) and includes helicates (Schmitt et al., 2012), metallacages (Vajpayee et al., 2011), cubes (Ahmedova et al., 2016), prisms(Ahmad et al., 2015), and capsules (Therrien, 2013). These systems are of general formula MnLm, where M is usually Fe(II), Pd(II), Pt(II), or half-sandwich organometallic clips based on Ru(II), Os(II), or Ir(III) and Rh(III), and L is the ligand of the coordination complex.
In 2012, Lewis et al. reported on the encapsulation properties of the anticancer drug cisplatin within cationic [Pd2L4]4+ cages (L = 2,6-bis(pyridine-3-ylethynyl)pyridine as the bidentate ligand) studied by NMR and X-ray diffraction analysis (Lewis et al., 2012). Following these promising results, as part of our efforts to develop functional metallosupramolecular drug delivery vectors, we have recently expanded the family of exo-functionalized [Pd2L4]4+ cages coupled to fluorescent groups for imaging by fluorescence microscopy (Kaiser et al., 2016; Schmidt et al., 2016a,b), as well as to peptides for targeted drug delivery of cisplatin to cancer cells (Han et al., 2017, 2018). It is worth mentioning that while cisplatin occupies a crucial role in the treatment of various malignant tumors, its efficacy, and applicability are heavily restricted by severe systemic toxicities and drug resistance. Thus, our recent studies have exploited the host-guest properties of targeted metallacages to enhance the activity of cisplatin in cancer cells, protecting it from metabolism (Han et al., 2017). Of note, the reduced toxicity of cisplatin encapsulated in integrin targeted metallacages has also been demonstrated by us in an ex vivo model (Han et al., 2017).
As the initially developed cage architectures displayed scarce water solubility, we were interested in improving their hydrophilicity via derivatization of the ligand scaffold. Thus, here, a small library of bis(pyridyl) ligands—of general scaffold 3,5-bis(3-ethynylpyridine)phenyl (Scheme 1) - exo-functionalized with chemical groups envisioned to improve the aqueous solubility, were synthesized and self-assembled into the corresponding [Pd2L4]4+ cages. The products were characterized by NMR, HR-ESI-MS, and IR. The solubility of the resulting metallacages was then tested in water, as well as in a range of common organic solvents. Furthermore, in order to elucidate the host-guest chemistry of the metallacages and the factors that favor cisplatin's retention in the supramolecular complexes, essential aspects to exploit the cages as drug delivery systems, the drug encapsulation was studied by 1H, 1H DOSY, and 195Pt NMR spectroscopy under different conditions.
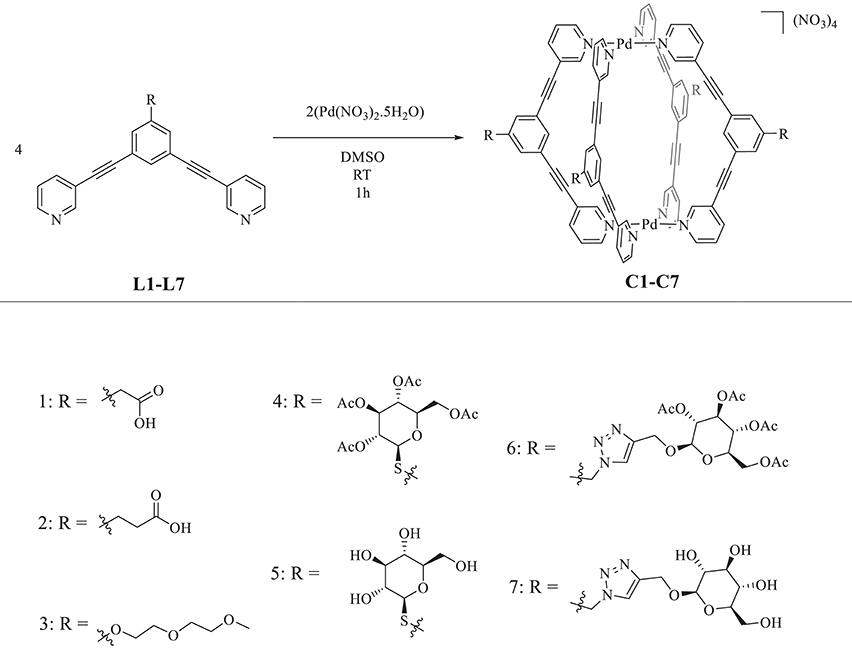
Scheme 1. Top: General scheme for [Pd2L4]4+ metallacage formation via self-assembly. Bottom: Numbered (n) chemical structures of the exo-functionalized groups envisioned to improve the cages' aqueous solubility.
Results and Discussion
Adapting previously reported procedures (Schmidt et al., 2016b), the synthesis of the exo-functionalized ligand scaffold with carboxylic acid groups, L1, and L2 was first achieved by Sonogashira cross-coupling of a benzyl-protected 1,3-dibromophenyl precursor with 3-ethynylpyridine, followed by basic deprotection of the benzyl ester to afford the free acid, forming the highly conjugated bispyridyl ligand scaffold in good yields (Scheme S1). A polyethylene glycol exo-functionalized ligand (L3) was also synthesized. In this case, a ligand bearing a central phenol aromatic ring was synthesized via Sonogashira cross-coupling, which was then was coupled to 1-chloro-2-(2-methoxyethoxy) ethane under basic conditions to achieve ligand L3 (Scheme S2). In addition, ligands L4 and L5, featuring water-soluble thio-β-D-glucose moieties, were synthesized for the first time. This was achieved by first synthesizing an alkyl bromide exo-functionalized ligand via classic Appel reaction using triphenylphosphine, carbon tetrabromide, and 3,5-bis(3-ethynylpyridine)-benzylalcohol; the latter having been synthesized via Sonogashira cross-coupling (Scheme S3). The 1-thio-β-D-glucose tetraacetate group was then conjugated under basic conditions following a SN2 type reaction to afford the ligand L4. Ligand L5 was achieved by deacetylation of L4 using Amberlite-IRA 401 (OH−) ion exchange beads, which were stirred together at room temperature overnight, following previously reported procedures (Lewis et al., 2014). Finally, the ligands L6 and L7 were synthesized using standard CuAAC “Click” reaction conditions (Scheme S4) following similar procedures reported for analogous tris-pyridyl ligand scaffolds (Lewis et al., 2013). Noteworthy, the latter were reported to be soluble in water, and therefore, offered a logical starting point for functionalization of the herein selected 3,5-bis(3-ethynylpyridine) phenyl ligands. All the ligands were characterized by NMR (1H, 13C) and HR-ESI-MS. A summary of the synthesized ligands and cages can be found in Scheme S5.
The solubility of the ligands in water was assessed by overnight sonication in D2O (10 mg/mL, 20°C), before filtration (pore size 450 μm). The filtrate was analyzed by 1H NMR to determine if detectable amounts of ligand were present. Unfortunately, the exo-functionalized bispyridyl ligand scaffolds were found to be scarcely soluble, and were all undetectable in D2O solution, except for the carboxylic acid functionalized ligands (L1 = 10 mg/mL, L2 > 100 mg/mL). The latter could be analyzed by 1H NMR in D2O (data not shown).
Afterwards, [Pd2L4]4+ cage formation was achieved via self-assembly by reacting two equivalents of Pd(II) nitrate pentahydrate with four equivalents of the functionalized ligand (L1-L7) in DMSO at room temperature, to form the corresponding homoleptic metallacages (C1-C7) (Scheme 1). The products were analyzed by NMR (1H, 13C, 11B, and 19F), HR-ESI-MS (Figures S1–S3, S5–S9) and IR. Following previously reported examples (Schmidt et al., 2016c), quantitative cage formation was monitored by 1H NMR, whereby the peaks corresponding to the protons alpha to the coordinating nitrogen of the terminal pyridine rings of the ligand undergo a large downfield shift, attributable to the withdrawal of electron density from the aromatic system following coordination to palladium (added in stoichiometric amounts). A representative 1H NMR spectra for cage C2 formation is shown in Figure S4.
In order to assess whether the synthesized metallacages were soluble in water, the products were suspended in D2O (8 mg per mL) and sonicated at 37°C for 24 h. The fine suspension was then filtered, and the filtrate was analyzed by 1H NMR. Despite the tetra-cationic nature of the metallacages, and the incorporation of solubilizing entities to the ligand scaffold, none of the metallacages (C1-C7) were soluble in D2O. In fact, the filtrate solution was colorless and no trace of a proton signal could be found in the 1H NMR spectra.
As a second attempt to water solubilization, and taking inspiration from previous work by Altmann and Pöthig (2016) on the synthesis of water soluble Ag(I) and Au(I) pillarplexes, we synthesized new cages featuring acetate as the counterion by the use of palladium(II) acetate as the precursor in the self-assembly. However, this attempt produced an insoluble residue that could not be dissolved in either organic solvents or water.
Concerning cisplatin encapsulation, in the case of previously reported [Pd2L4]BF4 cages (L = (2,6-bis(pyridin-3-ylethynyl)pyridine)) it was hypothesized that their central cavities are lined with four hydrogen bond accepting pyridine units which enable the encapsulation of two cisplatin molecules within the metallosupramolecular architecture through hydrogen bonding interactions between the cage and the amine ligands of the cisplatin guest (Lewis et al., 2012). This idea has been supported by NMR spectroscopy studies (Lewis et al., 2012), where chemical shifts of hydrogens in the trispyridyl ligands were observed upon cisplatin encapsulation. Control experiments confirm the importance of the hydrogen bonding interaction between the cage and the amine ligands of the cisplatin guest. It was also observed that upon addition of small amounts of D2O to a solution of the cisplatin-cage host-guest adduct in acetonitrile, the Ha, and Hb proton signals of the cage sharpen and shift upfield again, indicating evacuation of cisplatin from the cavity (Lewis et al., 2012).
In this context, information on the encapsulation process of cisplatin within the more hydrophobic cavity of the bispydridyl ligand forming our [Pd2L4]4+ cages, as opposed to the less hydrophobic cavity of trispyridyl-ligand Pd2L4 metallacages (Lewis et al., 2012, 2014), needs to be achieved still. Thus, we investigated if the encapsulation of the anticancer drug cisplatin could occur in the metallacages using 1H NMR spectroscopy. In order to avoid DMSO as the solvent, since it is known to exchange cisplatin's chlorido ligands, the experiment was carried out in DMF-d7. Cage C1 [Pd2L4](NO3)4 was selected to study the encapsulation process due to its solubility in this solvent. Figure 1 shows the variation of the chemical shifts of selected cage protons upon cisplatin encapsulation. Specifically, we could clearly observe the chemical shifts of peaks Ha and Hb: the peak of Ha, a cavity-facing proton, showed a small upfield shift of δ −1.0 Hz. The exo-facing proton, Hb, showed a downfield shift upon cisplatin encapsulation, in accordance with previously reported studies (Lewis et al., 2012). Unfortunately, the cavity facing peak He, likely to interact with the guest Pt(II) complex, was masked by the peak of Hf, and therefore, could not be analyzed.
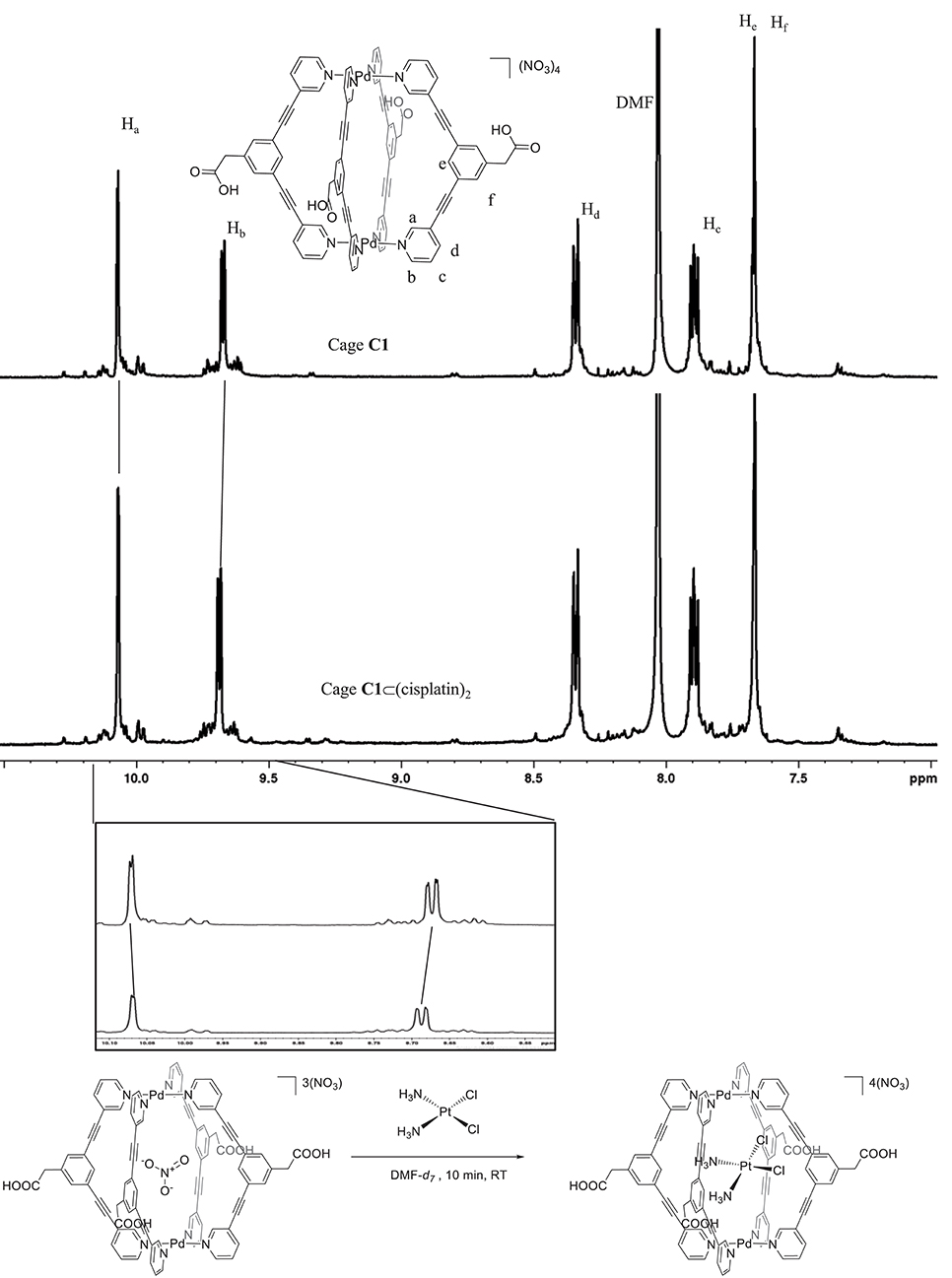
Figure 1. Cisplatin encapsulation in a metallacage following displacement of nitrate ions from the cavity. Stacked spectra of cage C1 in DMF-d7 (top) and cage C1 after the addition of 2 equation of cisplatin (bottom). Box: Zoom-in of signals for peaks Ha and Hb with chemical shifts of δ = −0.002 ppm (upfield) and +0.01 ppm (downfield), respectively.
It is worth mentioning that the observed smaller chemical shifts of the cavity facing proton Ha, with respect to those reported by Lewis et al. (2012) can be due to the lack of the H-bonding with the cisplatin guest (via N—H3N). Moreover, it has been previously reported that NO counterions are encapsulated within the cage architecture of trispyridyl Pd2L4 metallacages featuring a nitrogen in endo-position, while BF ions are not (Lewis and Crowley, 2014). Thus, we hypothesized that the observed small chemical shift of Ha following cisplatin encapsulation was due to the presence of NO ions inside the cage, which already induced chemical shifts of Ha and Hb and thus, acted to “mask” the effect of the cisplatin encapsulation process. In fact, upon evacuation of the anionic NO counterion, the “de-shielding” effect of the cavity facing protons from NO would be reduced as the neutral guest, cisplatin, occupies the metallacage cavity.
Afterwards, we also investigated if cisplatin would preferentially be encapsulated within our bispyridyl type Pd2L4 metallacages featuring a different counter ion. Therefore, an analogous metallacage was synthesized with BF as the counterion, C1.BF4 and studied for its cisplatin encapsulation properties by 1H NMR spectroscopy. Supporting Figure S10 shows the stacked 1H NMR spectrum of C1.BF4, alone or encapsulating cisplatin [C1.BF4⊂(cisplatin)] in DMF-d7. Indeed, both Ha and Hb show marked downfield shifts upon cisplatin encapsulation of 0.044 and 0.033 ppm, respectively. In addition, He also shows a downfield shift of 0.03 ppm. Furthermore, the peaks of both Ha and Hb of C1 (before addition of cisplatin) are already shifted further downfield (Figure 1, ca. 0.095 and 0.001 ppm, respectively) when compared to Ha and Hb of C1.BF4 (Figure S10) consistent with encapsulation of NO.
To continue our investigation, the effect of the solvent polarity was hypothesized to be the driving force of the cisplatin encapsulation process. Thus, the latter was also tested using C1.BF4 in MeCN-d3, with reduced polarity with respect to DMF-d7. However, upon addition of 2 eq. cisplatin to the MeCN-d3 solution of C1.BF4 (1 equation) a precipitate was formed, even after several hours of sonication. The suspension was filtered, and the resulting solution was analyzed by 1H NMR spectroscopy. However, the resulting spectra showed no indication of cisplatin encapsulation (data not shown). Instead, the large upfield shift of the peaks corresponding to the α-protons of the coordinating pyridyl is indicative of the ligands no longer coordinated to Pd(II) ions. Moreover, the NMR spectrum suggests that the structure of the ligand has also been altered.
Therefore, in order to study the effects of the solvent on cisplatin encapsulation, we synthesized a derivative of cage C1.BF4, but where the carboxylic function was protected by a benzyl group, namely cage C1Bn.BF4. Thus, cisplatin encapsulation was followed by 1H NMR in MeCN-d3 and the results are presented in Figure S11. As it can be observed, no chemical shifts could be seen for any of the cage's proton peaks even after 24 h incubation of cisplatin at room temperature. We could conclude that in acetonitrile, cisplatin encapsulation is not favored. In order to check that metallodrug encapsulation was not prevented by the presence of the benzyl group on the cage, we also monitored the process once more in DMF-d7. The results are depicted in Figure S12 and indicate that, in these conditions, cisplatin encapsulation occurs, as shown by the chemical shifts of Ha (downfield, δ = +0.009 ppm), Hb (downfield, δ = +0.021 ppm), and a small shift for the cavity-facing proton He (upfield, δ = −0.002 ppm). The encapsulation of cisplatin in cage C1Bn.BF4 was further studied in DMF-d7 using 195Pt NMR to see if a complementary shift could be observed for the guest cisplatin molecules. Indeed, an upfield shift of −5 ppm was observed upon addition of either 1 eq. or 2 eq. of cisplatin in the presence of metallacage C1Bn.BF4 (Figure S13), supporting cisplatin encapsulation. The obtained results are in agreement with previously reported ones from our group on similar cage systems exo-functionalized with different type of ligands (Schmidt et al., 2016c), indicating that surface functionalization does not prevent the guest from entering the cavity.
The encapsulation of cisplatin in C1Bn.BF4 was also studied by 1H DOSY NMR in DMF-d7. Cisplatin alone shows a broad signal at 4.18 ppm, HNH3 with a diffusion coefficient = −8.9 × 10−10 m2/s (Figure S14, red traces). Clear quenching of this signal was observed upon addition of 1 eq. of metallacage C1Bn.BF4 (Figure S14, blue traces). The cisplatin peak reappears slightly upon addition of a second equivalent of cisplatin to C1Bn.BF4⊂(cisplatin) (Figure S14, black traces), which may suggest that the metallacage cavity has been saturated to form C1Bn.BF4⊂(cisplatin)2 and residual cisplatin remains free in solution, or that upon addition of a second equivalent of cisplatin, a dynamic equilibrium occurs.
Conclusions
In conclusion, we report here on a series of exo-functionalized [Pd2L4]4+ metallacages featuring bispydridyl ligands. Despite the addition of hydrophilic groups to the ligands' scaffold, or the use of different counter ions, the increase in water solubility of the resulting cages was not achieved. Thus, different strategies are necessary to address this problem, including the conjugation of the ligands to peptides. The latter approach has already proved to be successful (Han et al., 2017).
Furthermore, the encapsulation of the anticancer drug cisplatin in selected cages has been studied by NMR spectroscopy, and the obtained results show that if the solvent is of sufficient polarity, metallodrug encapsulation can easily occur in the hydrophobic cavity of the cage despite the absence of hydrogen-bond accepting central pyridine units (Lewis and Crowley, 2014). Conversely, polar solvent molecules capable of forming hydrogen-bond networks (including DMF and water) are likely to prefer not to be encapsulated by hydrophobic cage cavities, as in our case, and will not compete with the cisplatin molecules. This hypothesis is corroborated by previous studies on M4L6 metallacages for which guest encapsulation in polar protic solvents, such as water, appears to be driven by initial desolvation of the guest with concomitant rearrangement of the hydrogen bond networks in solution, more than by host-guest interactions alone (Leung et al., 2008). In general, energetically favorable solvent rearrangement during guest desolvation also explains the ability of the supramolecular host to encapsulate neutral guests such as cisplatin, in spite of the lack of possible electrostatic attraction or H-bond interactions with the charged host, as in our case. Further studies are ongoing in our lab to validate this hypothesis.
Author Contributions
AC and BW contributed conception and design of the study. BW, MW, and ST contributed to the design, synthesis, and characterization of the ligands, and of the palladium cages. TW contributed to the HR-ESI-MS analysis of the cages. BW and RJ performed the encapsulation studies by NMR spectroscopy. AC and BW wrote the first draft of the manuscript, while MW wrote sections of the manuscript. All authors contributed to manuscript revision, read, and approved the submitted version.
Conflict of Interest Statement
The authors declare that the research was conducted in the absence of any commercial or financial relationships that could be construed as a potential conflict of interest.
Acknowledgments
Authors thank Cardiff University for funding.
Supplementary Material
The Supplementary Material for this article can be found online at: https://www.frontiersin.org/articles/10.3389/fchem.2019.00068/full#supplementary-material
References
Ahmad, N., Younus, H. A., Chughtai, A. H., and Verpoort, F. (2015). Metal–organic molecular cages: applications of biochemical implications. Chem. Soc. Rev. 44, 9–25. doi: 10.1039/C4CS00222A
Ahmedova, A., Momekova, D., Yamashina, M., Shestakova, P., Momekov, G., Akita, M., et al. (2016). Anticancer potencies of Pt(II)-and Pd(II)-linked M2L4 coordination capsules with improved selectivity. Chem. Asian J. 11, 474–477. doi: 10.1002/asia.201501238
Altmann, P. J., and Pöthig, A. (2016). Pillarplexes: a metal-organic class of supramolecular hosts. J. Am. Chem. Soc. 138, 13171–13174. doi: 10.1021/jacs.6b08571
Casini, A., Woods, B., and Wenzel, M. (2017). The promise of self-assembled 3D supramolecular coordination complexes for biomedical applications. Inorg. Chem. 56, 14715–14729. doi: 10.1021/acs.inorgchem.7b02599
Cook, T. R., Vajpayee, V., Lee, M. H., Stang, P. J., and Chi, K. W. (2013). Biomedical and biochemical applications of self-assembled metallacycles and metallacages. Acc. Chem. Res. 46, 2464–74. doi: 10.1021/ar400010v.
Han, J., Rader, A., Reichart, F., Aikman, B., Wenzel, M. N., Woods, B., et al. (2018). Bioconjugation of supramolecular metallacages to integrin ligands for targeted delivery of cisplatin. Bioconjug. Chem. 29, 3856–3865. doi: 10.1021/acs.bioconjchem.8b00682
Han, J., Schmidt, A., Zhang, T., Permentier, H., Groothuis, G. M. M., Bischoff, R., et al. (2017). Bioconjugation strategies to couple supramolecular: exo -functionalized palladium cages to peptides for biomedical applications. Chem. Commun. 53, 1405–1408. doi: 10.1039/c6cc08937b
Kaiser, F., Schmidt, A., Heydenreuter, W., Altmann, P. J., Casini, A., Sieber, S. A., et al. (2016). Self-assembled palladium and platinum coordination cages: photophysical studies and anticancer activity. Eur. J. Inorg. Chem. 2016:5189–5196. doi: 10.1002/ejic.201601282
Kaner, R. A., Allison, S. J., Faulkner, A. D., Phillips, R. M., Roper, D. I., Shepherd, S. L., et al. (2016). Anticancer metallohelices: nanomolar potency and high selectivity. Chem. Sci. 7, 951–958. doi: 10.1039/C5SC03677A
Leung, D. H., Bergman, R. G., and Raymond, K. N. (2008). Enthalpy-entropy compensation reveals solvent reorganization as a driving force for supramolecular encapsulation in water. J. Am. Chem. Soc. 130, 2798–2805. doi: 10.1021/ja075975z
Lewis, J. E., John McAdam, C., Gardiner, M. G., and Crowley, J. D. (2013). A facile “click” approach to functionalised metallosupramolecular architectures. Chem. Commun. 49, 3398–3400. doi: 10.1039/c3cc41209a
Lewis, J. E. M., and Crowley, J. D. (2014). Exo- and endo-hedral interactions of counteranions with tetracationic Pd2L4 metallosupramolecular architectures. Supramol. Chem. 26, 173–181. doi: 10.1080/10610278.2013.842644
Lewis, J. E. M., Elliott, A. B. S., McAdam, C. J., Gordon, K. C., and Crowley, J. D. (2014). ‘Click' to functionalise: synthesis, characterisation and enhancement of the physical properties of a series of exo- and endo-functionalised Pd2 L4 nanocages. Chem. Sci. 5, 1833–1843. doi: 10.1039/C4SC00434E
Lewis, J. E. M., Gavey, E. L., Cameron, S. A., and Crowley, J. D. (2012). Stimuli-responsive Pd2L4 metallosupramolecular cages: towards targeted cisplatin drug delivery. Chem. Sci. 3, 778–784. doi: 10.1039/c2sc00899h
Preston, D., McNeill, S. M., Lewis, J. E. M., Giles, G. I., and Crowley, J. D. (2016). Enhanced kinetic stability of [Pd2 L4]4+ cages through ligand substitution. Dalton Trans. 45, 8050–8060. doi: 10.1039/C6DT00133E
Schmidt, A., Hollering, M., Drees, M., Casini, A., and Kühn, F. E. (2016a). Supramolecular exo-functionalized palladium cages: fluorescent properties and biological activity. Dalton Trans. 45 8556–8565. doi: 10.1039/C6DT00654J
Schmidt, A., Hollering, M., Han, J., Casini, A., and Kühn, F. E. (2016b). Self-assembly of highly luminescent heteronuclear coordination cages. Dalton Trans. 45, 12297–12300. doi: 10.1039/c6dt02708c
Schmidt, A., Molano, V., Hollering, M., Pöthig, A., Casini, A., and Kühn, F. E. (2016c). Evaluation of new palladium cages as potential delivery systems for the anticancer drug cisplatin. Chem. A Eur. J. 22, 2253–2256. doi: 10.1002/chem.201504930
Schmitt, F., Barry, N. P. E., Juillerat-Jeanneret, L., and Therrien, B. (2012). Efficient photodynamic therapy of cancer using chemotherapeutic porphyrin-ruthenium metalla-cubes. Bioorg. Med. Chem. Lett. 22, 178–180. doi: 10.1016/j.bmcl.2011.11.058
Therrien, B. (2013). Drug delivery by water-soluble organometallic cages. Top. Curr. Chem. 11, 13–35. doi: 10.1007/128
Keywords: metallacages, supramolecular coordination complexes, water solubility, host-guest chemistry, cisplatin
Citation: Woods B, Wenzel MN, Williams T, Thomas SR, Jenkins RL and Casini A (2019) Exo-Functionalized Metallacages as Host-Guest Systems for the Anticancer Drug Cisplatin. Front. Chem. 7:68. doi: 10.3389/fchem.2019.00068
Received: 05 November 2018; Accepted: 24 January 2019;
Published: 18 February 2019.
Edited by:
Leyong Wang, Nanjing University, ChinaReviewed by:
Xiao-Yu Hu, Nanjing University of Aeronautics and Astronautics, ChinaLaia Vilà Nadal, University of Glasgow, United Kingdom
Robert Elmes, Maynooth University, Ireland
Copyright © 2019 Woods, Wenzel, Williams, Thomas, Jenkins and Casini. This is an open-access article distributed under the terms of the Creative Commons Attribution License (CC BY). The use, distribution or reproduction in other forums is permitted, provided the original author(s) and the copyright owner(s) are credited and that the original publication in this journal is cited, in accordance with accepted academic practice. No use, distribution or reproduction is permitted which does not comply with these terms.
*Correspondence: Angela Casini, Y2FzaW5pYUBjYXJkaWZmLmFjLnVr