- 1Collaborative Innovation Center of Nonferrous Metals of Henan Province, Henan Key Laboratory of High-Temperature Structural and Functional Materials, School of Materials Science and Engineering, Henan University of Science and Technology, Luoyang, China
- 2Henan Key Laboratory of Non-Ferrous Materials Science & Processing Technology, Henan University of Science and Technology, Luoyang, China
- 3School of Chemistry and Chemical Engineering, Yangzhou University, Yangzhou, China
Nowadays, wearable energy storage devices have been growing rapidly, but flexible systems with both excellent cycling stability and decent flexibility are still challenging. In this work, a flexible all-solid-state NH4NiPO4·H2O//graphene supercapacitor with remarkable performance was successfully assembled. When cycled at a current density of 5 mA cm−2, the device delivered 121 mF cm−2, and showed good cycling stability after 3,000 cycles. Moreover, the all-solid-state NH4NiPO4·H2O//graphene supercapacitor also exhibit high mechanical flexibility with well-maintained specific capacitance, even under bending to arbitrary angles (up to 180°) and different weights (up to 50 g).
Introduction
Recently, for the reason of environmental and energy issues, research on energy storage have become one of hot spots all over the world (Wang et al., 2017, 2019; Zheng et al., 2017; Huang et al., 2018a,b,c; Liu et al., 2018; Wang F. et al., 2018; Wang H. et al., 2018; Wang H. K. et al., 2018; Zhang et al., 2018a; Zhao et al., 2018). Among the energy storage systems, supercapacitors have sparked increasing attention due to their high power density and long cycle life (Conway, 1999; Aricò et al., 2005; Miller and Simon, 2008; Yuan et al., 2012; Bin Jiang et al., 2018; Zhu et al., 2018). One type of supercapacitors, pseudocapacitors, which include fast Faradic reactions on the electrodes, could deliver greater specific capacitance than electrochemical double-layer capacitors, which could make the device have higher energy density (Dai et al., 2018; Gao et al., 2018; Zhang et al., 2018b; Zhao et al., 2018a; Zheng et al., 2018). For the electrode materials of pseudocapacitors, electrochemical active materials are often used, such as transition-metal oxides [e.g., RuO2 (Zhai et al., 2018), NiO (Zuo et al., 2016), MnO2 (Yang et al., 2016), Co3O4 (Zhang et al., 2016a)], and conducting polymers (Xie and Wang, 2016). Nevertheless, the high price of Ruthenium makes it hardly be utilized as electrode materials for pseudocapacitors. In this case, it is crucial to synthesize an electrode material with low cost and high performance.
On the other hand, portable devices generally require small size, light weight, which the traditional capacitors could not achieve, and all of these limit the development of this area (Huang et al., 2018). As a newly developed energy-storage device, the flexible all-solid-state supercapacitors are small and light compared to the conventional capacitors (Lv et al., 2018). And flexible all-solid-state supercapacitor could deliver much higher energy density than conventional capacitors (Gao et al., 2014a; Wei et al., 2015; Yousaf et al., 2016; Zhang et al., 2016b; Wu et al., 2018). Furthermore, with two electrodes made of different materials, these asymmetric supercapacitors could show better performance in energy density. Together with their high power density, flexible asymmetric all-solid-state supercapacitor are promising for the wearable energy storage systems (Zhang et al., 2016b; Wu et al., 2018).
In the past few years, ammonium/transition metal phosphate NH4MPO4·H2O (M = Co2+, Ni2+) have been studied as electrodes in the field of supercapacitors (Pang et al., 2012; Zhao et al., 2013; Wang et al., 2014a). For instance, Wang and his colleagues utilized a facile hydrothermal method to synthesize layered NH4CoPO4·H2O microbundles which consist of 1D layered microrods (Wang et al., 2014a). The layered microbundle electrode showed good high-rate capability as well as excellent cycling stability. In the previous work, we have successful fabricated mesoporous NH4NiPO4·H2O nanostructures using one-pot hydrothermal method (Zhao et al., 2013). In this work, we assembled them into flexible all-solid-state asymmetric supercapacitors and studied their electrochemical performances. The specific capacitance of the device can reach 121 mF cm−2, and shows good long-term cycling stability. And this device exhibit excellent mechanical flexibility under bending to arbitrary angles (up to 180°) and different weights (even 50 g).
Materials and Methods
Synthesis of Mesoporous NH4NiPO4·H2O Nanostructures
NH4NiPO4·H2O nanostructures were synthesized by reacting 0.40 g Ni(NO3)2 and 0.40 g (NH4)3PO4 at 200°C for 45 h under hydrothermal condition in 20.0 mL ethylene glycol, and the autoclave was then cooled to room temperature as described elsewhere (Zhao et al., 2013). The green and yellow precipitates were obtained and filtered. After being washed with distilled water and ethanol repeatly, the final product was obtained after being dried in air for 24 h.
Characterizations
The crystal structures of the samples were analyzed by X-ray diffraction (XRD) (Rigaku-Ultima III with Cu Kα radiation, λ = 1.5418 Å). The microstructures of as-prepared samples were revealed using a field-emission scanning electron microscope (FESEM; JEOL JSM-6701F, 5.0 kV), transmission electron microscopy (TEM) and high-resolution transmission electron microscopy (HRTEM) (JEM-2100, 200 kV). Nitrogen adsorption–desorption isotherms were measured on a Gemini VII 2390 Analyzer at 77 K, and the specific surface area was calculated by the Brunauer-Emmett-Teller (BET) method.
Fabrication of Flexible All-Solid-State NH4NiPO4·H2O//Graphene Supercapacitors
The PET substrates were first deposited with a layer of Pt film (about 3–5 nm thick) and then coated with the slurry containing the active materials (NH4NiPO4·H2O or graphene via a procedure similar to that in the three-electrode system and were used as the working electrode after drying). Meanwhile, 1.52 g PVA was mixed into 10 ml deionized water to form a mixture. After the mixture is clarified at the constant temperature of 75°C,the prepared 5 ml 3 mol/L KOH are slowly dripped into the mixture with continuous stirring. Then the gel-like electrolyte was obtained. Then, two pieces of such electrodes were immersed in the gel solution for 5–10 min to coat a layer of gel electrolyte. After the excess water was vaporized, two pieces of such electrodes containing electrolyte were pressed together with sandwiched structures. Finally, the stacked all-solid-state NH4NiPO4·H2O//graphene asymmetric supercapacitors were fabricated.
Electrochemical Measurements
Electrochemical study on all-solid-state NH4NiPO4·H2O//graphene asymmetric supercapacitor was carried out using an electrochemical working-station (CHI 660D, Shanghai Chenhua). The electrochemical performance measurements were conducted in a conventional two-electrode system with graphene electrode as counter and reference electrode. Cyclic voltammetry (CV) and galvanostatic charge-discharge methods were used to investigate capacitive properties of all-solid-state NH4NiPO4·H2O//graphene asymmetric supercapacitor with a potential window between 0 and 1.4 V. And electrochemical impedance spectroscopy (ESI) measurements were carried out by using PARSTAT2273 at 0.4 V over the frequency range of 100 kHz to 10 mHz with an amplitude of 5 mV.
Results and Discussions
As shown in Figure 1b, all peaks correspond well to those of NH4NiPO4·H2O (JCPD No. 50-0425, Figure 1a), indicating the good crystallinity of the samples. This is consistent with the observations reported elsewhere (Zhao et al., 2013). Figure S1b shows the typical N2 adsorption-desorption isotherms of mesoporous NH4NiPO4·H2O, and from the calculation, the specific surface area of the sample was ~418 m2 g−1 and pore-sizes were within the range about 2.0–18.5 nm (Figure S1a). The presence of mesoporous provides channels for the ion transport, and high specific surface area could facilitate the contact of electrolyte and electrode, which are beneficial to the electrochemical properties of electrode.
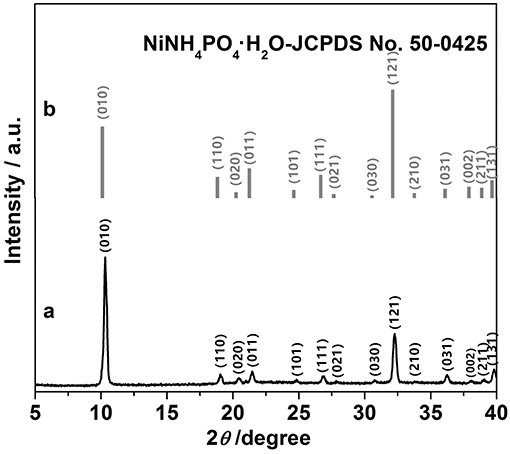
Figure 1. (a) X-ray diffraction patterns of as-prepared NH4NiPO4·H2O samples and (b) corresponding JCPDS No. 50-0425.
The morphologies of the as-prepared NH4NiPO4·H2O samples were examined by FESEM and TEM. As shown in Figures 2a,b, the samples are in uniform nano-almond structures, and even when the scale bar is 1.5 μm (Figure 2a), showing the high uniformity of nanostructures. The sizes of single nano-almond are in the range of 300~350 nm. Furthermore, the uniform shape and size were further proved by TEM, which are shown in Figures 2c,d. Figures 2e,f show the HRTEM and selected area electron diffraction (SAED) pattern of as-prepared NH4NiPO4·H2O samples. The d-spacing of lattice fringes in Figure 2e is ~0.278 nm, which is corresponding to the (121) lattice spacing of NH4NiPO4·H2O. The SAED patterns in Figure 2f confirm the polycrystalline nature of the samples, which show NH4NiPO4·H2O phase. As shown in Figure 2e, the measured diameters of pores are ~2.0 nm, and the porous structure may facilitate electrolyte access, resulting in fast ion intercalation and extraction.
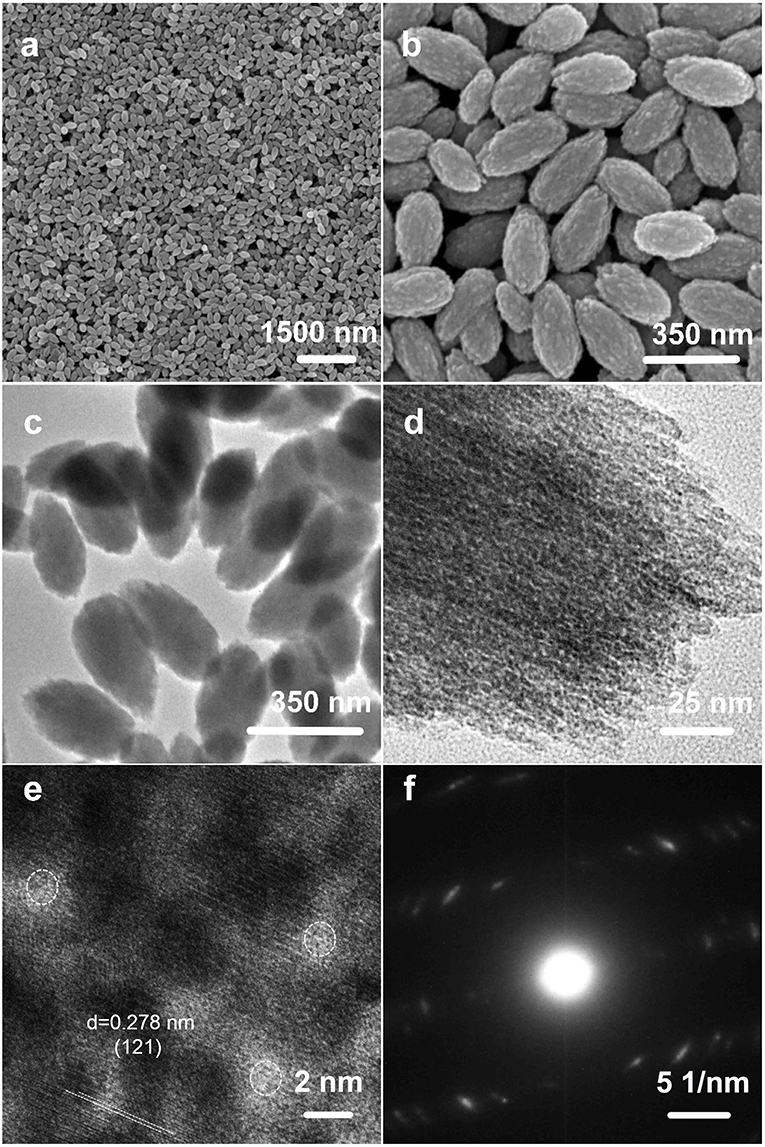
Figure 2. (a,b) SEM images, (c,d) TEM images, (e) HRTEM image, and (f) selected area electron diffraction patterns of as-prepared NH4NiPO4·H2O samples.
In this work, flexible all-solid-state hybrid supercapacitors were assembled using as-prepared NH4NiPO4·H2O and graphene as positive and negative electrode, respectively. The CV and galvanostatic charge and discharge tests were carried out to test the electrochemical properties of the samples. As the Figure 3A shows, the charging voltage of the device is 0 to 1.4V. When the scan rates range from 5 to 50 mV s−1. The curves show a quasi-rectangular geometry, which shows that the sample not only has the characteristic of pseudo capacitance, but also has the characteristics of electric double layer capacitance at these rates (Gao et al., 2014b). Furthermore, when rate is as high as 50 mV s−1, the shape of CV curve could still preserved, indicating that the hybrid supercapacitor has very good rate capability (Dai et al., 2018). When the hybrid supercapacitor was charged and discharged in the current density of 0.2, 0.5, 1.2, 2.0, 3.0, 5.0 mA cm−2, as the Figure 3B shows, these curves are approximately in triangular shape, which means the supercapacitor have excellent reversibility and capacitance at each current density. And the capacitances is calculated from galvanostatic charge-discharge curves by the following Formula:
Where I is the current density, t is the discharge time, V is the potential range (V = 1.4 V) and S is the area of the supercapacitors (Roldán et al., 2015). After calculation, we plotted the specific capacitance of the supercapacitor. As shown in Figure 3C. When the current density is 0.5 mA cm−2, its areal specific capacitances could achieve 180 mF cm−2. Remarkably, even at as high as 5 mA cm−2, this value can still reach 121 mF cm−2. The capacity retention rate is about 88.8%, after 3,000 cycles with the current density of 5 mA cm−2 (Figure 3D). This capacitance decay may be attributed to some irreversible reactions between the electrodes and electrolyte (Wang et al., 2014b). Noticeably, even after 3,000 cycles, the nanostructured morphology of the electrode material was well-sustained (Figure S2). A comparison of the electrochemical performance of the supercapacitors with other hybrid solid state devices are shown in Table S1.
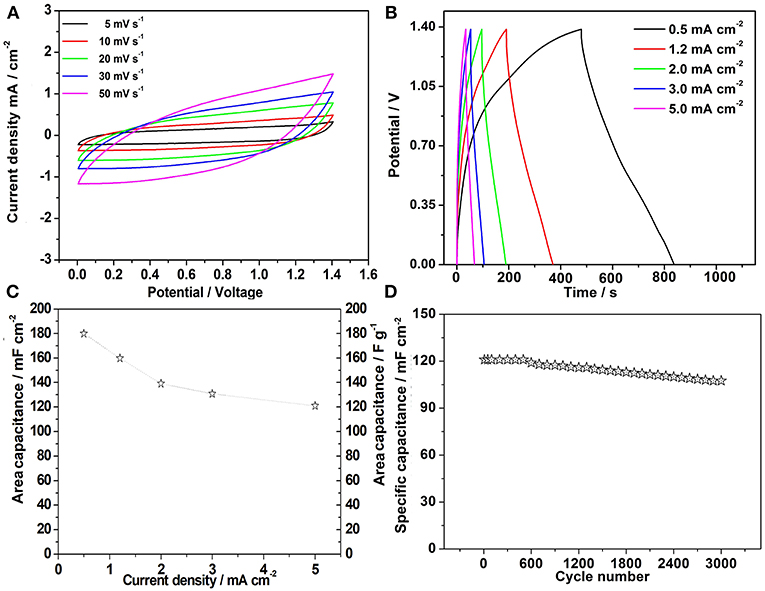
Figure 3. (A) Cyclic Voltammetry curves of the hybrid supercapacitor in different scan rates; (B) Galvanostatic charge-discharge curves with different current densities; (C) Specific capacitance with different current densities; (D) Charge-discharge cycling test at a current density of 5.0 mA cm−2.
To evaluate the potential of the all-solid-state hybrid supercapacitor for flexible energy storage under real conditions, the CV curves of the device at 5 mV s−1 were collected under normal and bent conditions. As shown in Figure 4a, when the hybrid supercapacitor was bent to 30°, 90°, 180°, the curves change slightly, suggesting the good capacitance stability of this flexible supercapacitor (Qin et al., 2018; Wang W. et al., 2018). Figures 4b–e show the all-solid-state hybrid supercapacitor under different weights and corresponding CV curves with 0–1.4 V range at a scan rate of 10 mV s−1. Similar to the device with different bending angles, the CV curves of the device under different weight (5, 20, and 50 g) change slightly, and the corresponding specific capacitance of the device is well-maintained. All the above results show that this hybrid supercapacitor has excellent mechanical flexibility (Qin et al., 2018; Wang W. et al., 2018).
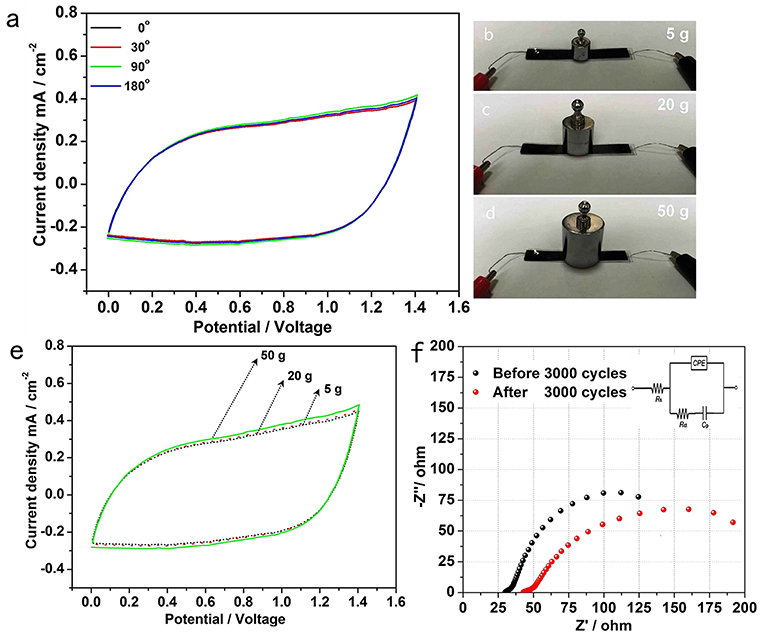
Figure 4. (a) CV curves within 0–1.4 V range at scan rate of 5 mV s−1 with different bending degrees. The optical photos of the device under different weights (b) 5 g; (c) 20 g; (d) 50 g, and corresponding (e) CV curves within 0–1.4 V range at scan rate of 10 mV s−1 when under different weights. (f) The Nyquist plots of the hybrid supercapacitor before and after 3,000 cycles at a current density of 5.0 mA cm−2, inset shows the EIS equivalent circuitry.
We test the electrochemical impedance spectra (EIS) of the supercapacitor before and after 3,000 cycles at a current density of 5.0 mA cm−2. An equivalent circuit was given in the inset of Figure 4f, which is similar to the circuit employed for the working electrode of a supercapacitor. The EIS data can be fitted by a bulk solution resistance Rs, a charge-transfer resistance Rct and a pseudocapacitive element Cp from the redox process of electrode materials, and a CPE to account for the double-layer capacitance (Pang et al., 2013). As shown in Figure 4f, the intrinsic resistance Rs of the device before and after 3,000 cycles are around 27.2 and 38.1 Ω, respectively. And the Rct after 3,000 cycles is around 218 ohms, which is higher than the 176 ohms of the initial Rct. The increase of charge transfer resistance may be due to the irreversible reaction between the electrodes and the electrolyte, which is consistent with the decrease in the capacitance after cycling (Figure 3D).
Conclusion
In summary, a flexible all-solid-state NH4NiPO4·H2O//graphene device was successfully assembled, which showed great performance. When cycled for 3,000 cycle at the current density of 5.0 mA cm−2, the hybrid supercapacitor shows 88.8% in capacitance retention rate. The device also showed excellent flexibility, especially when bent to various degrees and under different weights. The as-prepared flexible all-solid-state device could be integrated in to large scale flexible systems that require an energy storage unit. And further study will be focused on improving the device performance.
Author Contributions
YL, WZ, and HP conceived and designed the experiments. YL, XZ, FW, and KY performed the experiments. XZ, HW, and FR analyzed the data. YL and XZ wrote the paper. HP and WZ revised the paper, which could be found in the list of corrections we have submitted.
Funding
This work is supported by the Program for New Century Excellent Talents of the University in China (grant no. NCET-13-0645) and Natural Science Foundation of China (21671170), the Program for Changjiang Scholars and Innovative Research Team in University (IRT_16R21), Program for Innovative Research Team (in Science and Technology) in University of Henan Province (14IRTSTHN004), Open Fund of National Joint Engineering Research Center for abrasion control and molding of metal materials (HKDNM201807, HKDNM201802), Henan International Science and Technology Cooperation Project of China (134300510051), Scientific Research Starting Foundation for Ph.D. of Henan University of Science and Technology (13480065), the Scientific and Technological Project of Henan Province (182102210297), the Student Research Training Plan of Henan University of Science and Technology (2018029), Science Foundation for Youths of Henan University of Science and Technology (2013QN006).
Conflict of Interest Statement
The authors declare that the research was conducted in the absence of any commercial or financial relationships that could be construed as a potential conflict of interest.
Supplementary Material
The Supplementary Material for this article can be found online at: https://www.frontiersin.org/articles/10.3389/fchem.2019.00118/full#supplementary-material
References
Aricò, A. S., Bruce, P., Scrosati, B., Tarascon, J.-M., and van Schalkwijk, W. (2005). Nanostructured materials for advanced energy conversion and storage devices. Nat. Mater. 4, 366–377. doi: 10.1038/nmat1368
Bin Jiang, D., Zhang, B. Y., Zheng, T. X., Zhang, Y. X., and Xu, X. (2018). One-pot synthesis of eta-Fe2O3 nanospheres/diatomite composites for electrochemical capacitor electrodes. Mater. Lett. 215, 23–26. doi: 10.1016/j.matlet.2017.12.059
Conway, B. E. (1999). Electrochemical Supercapacitors: Scientific, Fundamentals and Technological Applications. New York, NY: Plenum.
Dai, S., Liu, Z., Zhao, B., Zeng, J., Hu, H., Zhang, Q., et al. (2018). A high-performance supercapacitor electrode based on N-doped porous graphene. J. Power Sources 387, 43–48. doi: 10.1016/j.jpowsour.2018.03.055
Gao, M., Fu, J., Wang, M., Wang, K., Wang, S., Wang, Z, et al. (2018). A self-template and self-activation co-coupling green strategy to synthesize high surface area ternary-doped hollow carbon microspheres for high performance supercapacitors. J. Colloid Interface Sci. 524, 165–176. doi: 10.1016/j.jcis.2018.04.027
Gao, S., Sun, Y. F., Lei, F. C., Liang, L., Liu, J., Bi, W. T., et al. (2014a). Ultrahigh energy density realized by a single-layer beta-CO(OH)2 All-solid-state asymmetric supercapacitor. Angew. Chemie Int. Ed. 53, 12789–12793. doi: 10.1002/anie.201407836
Gao, Y., Zhao, J., Run, Z., Zhang, G., and Pang, H. (2014b). Microporous Ni-11(HPO3)8(OH)6 nanocrystals for high-performance flexible asymmetric all solid-state supercapacitors. Dalton Transact. 43, 17000–17005. doi: 10.1039/c4dt02831g
Huang, L., Santiago, D., Loyselle, P., and Dai, L. (2018). Graphene-based nanomaterials for flexible and wearable supercapacitors. Small 14:e1800879. doi: 10.1002/smll.201800879
Huang, Z.-D., Zhang, T.-T., Lu, H., Masese, T., Yamamoto, K., Liu, R.-Q., et al. (2018a). Grain-boundary-rich mesoporous NiTiO3 micro-prism as high tap-density, super rate and long life anode for sodium and lithium ion batteries. Energy Storage Mater. 13, 329–339. doi: 10.1016/j.ensm.2017.08.012
Huang, Z. D., Lu, H., Qian, K., Fang, Y. W., Du, Q. C., He, Y. B., et al. (2018b). Interfacial engineering enables Bi@C-TiOx microspheres as superpower and long life anode for lithium-ion batteries. Nano Energy 51, 137–145. doi: 10.1016/j.nanoen.2018.06.051
Huang, Z. D., Zhang, T. T., Lu, H., Yang, J. K., Bai, L., Chen, Y. H., et al. (2018c). Bimetal-organic-framework derived CoTiO3 mesoporous micro-prisms anode for superior stable power sodium ion batteries. Sci. China Mater. 61, 1057–1066. doi: 10.1007/s40843-017-9225-5
Liu, Y., Wei, H., Wang, C., Wang, F., Wang, H., Zhang, W., et al. (2018). Nitrogen-doped carbon coated ws 2 nanosheets as anode for high-performance sodium-ion batteries. Front. Chem. 6:236. doi: 10.3389/fchem.2018.00236
Lv, T., Liu, M., Zhu, D., Gan, L., and Chen, T. (2018). Nanocarbon-based materials for flexible all-solid-state supercapacitors. Adv. Mater. 30:17. doi: 10.1002/adma.201705489
Miller, J. R., and Simon, P. (2008). Materials science - Electrochemical capacitors for energy management. Science 321, 651–652. doi: 10.1126/science.1158736
Pang, H., Wang, S. M., Shao, W. F., Zhao, S. S., Yan, B., Li, X. R., et al. (2013). Few-layered CoHPO4 center dot 3H2O ultrathin nanosheets for high performance of electrode materials for supercapacitors. Nanoscale 5, 5752–5757. doi: 10.1039/c3nr01460f
Pang, H., Yan, Z. Z., Wang, W. Q., Chen, J., Zhang, J. S., and Zheng, H. H. (2012). Facile fabrication of NH4CoPO4 center dot H2O nano/microstructures and their primarily application as electrochemical supercapacitor. Nanoscale 4, 5946–5953. doi: 10.1039/c2nr31208e
Qin, J., Zhou, F., Xiao, H., Ren, R., and Wu, Z.-S. (2018). Mesoporous polypyrrole-based graphene nanosheets anchoring redox polyoxometalate for all-solid-state micro-supercapacitors with enhanced volumetric capacitance. Sci. China Mater. 61, 233–242. doi: 10.1007/s40843-017-9132-8
Roldán, S., Barreda, D., Granda, M., Menéndez R., Santamaría, R., and Blanco, C. (2015). An approach to classification and capacitance expressions in electrochemical capacitors technology. Phys. Chem. Chem. Phys. 17, 1084–1092. doi: 10.1039/c4cp05124f
Wang, F., Liu, Y., Zhao, Y. F., Wang, Y., Wang, Z. J., Zhang, W. H., et al. (2018). Facile synthesis of two-dimensional porous MgCO2O4 nanosheets as anode for lithium-ion batteries. Appl. Sci. Basel 8:22. doi: 10.3390/app8010022
Wang, H., Yang, X. M., Wu, Q., Zhang, Q., Chen, H., Jing, H., et al. (2018). Encapsulating silica/antimony into porous electrospun carbon nanofibers with robust structure stability for high-efficiency lithium storage. Acs Nano 12, 3406–3416. doi: 10.1021/acsnano.7b09092
Wang, H. K., Wang, J., Xie, S., Liu, W. X., and Niu, C. (2018). Template synthesis of graphitic hollow carbon nanoballs as supports for SnOx nanoparticles towards enhanced lithium storage performance. Nanoscale 10, 6159–6167. doi: 10.1039/c8nr00405f
Wang, H. K., Wang, J. K., Cao, D. X., Gu, H. Y., Li, B. B., Lu, X., et al. (2017). Honeycomb-like carbon nanoflakes as a host for SnO2 nanoparticles allowing enhanced lithium storage performance. J. Mater. Chem. A 5, 6817–6824. doi: 10.1039/c7ta00772h
Wang, S. M., Pang, H., Zhao, S. S., Shao, W. F., Zhang, N. N., Zhang, J. S., et al. (2014a). NH4CoPO4 center dot H2O microbundles consisting of one-dimensional layered microrods for high performance supercapacitors. RSC Adv. 4, 340–347. doi: 10.1039/c3ra45977b
Wang, W., Yuan, Y., Yang, J., Meng, L., Tang, H., Zeng, Y., et al. (2018). Hierarchical core-shell Co3O4/graphene hybrid fibers: potential electrodes for supercapacitors. J. Mater. Sci. 53, 6116–6123. doi: 10.1007/s10853-017-1971-z
Wang, X., Liu, B., Liu, R., Wang, Q., Hou, X. J., Chen, D., et al. (2014b). Fiber-based flexible all-solid-state asymmetric supercapacitors for integrated photodetecting system. Angew. Chem. Int. Ed. 53, 1849–1853. doi: 10.1002/anie.201307581
Wang, X., Yang, C., Xiong, X., Chen, G., Huang, M., Wang, J.-H., et al. (2019). A robust sulfur host with dual lithium polysulfide immobilization mechanism for long cycle life and high capacity Li-S batteries. Energy Storage Mater. 16, 344–353. doi: 10.1016/j.ensm.2018.06.015
Wei, C. Z., Cheng, C., Zhou, B. B., Yuan, X., Cui, T. T., Wang, S. S., et al. (2015). Hierarchically porous NaCoPO4-Co3O4 hollow microspheres for flexible asymmetric solid-state supercapacitors. Part. Part. Syst. Character. 32, 831–839. doi: 10.1002/ppsc.201500018
Wu, S. X., San Hui, K., and Hui, K. N. (2018). Carbon nanotube@manganese oxide nanosheet core-shell structure encapsulated within reduced graphene oxide film for flexible all-solid-state asymmetric supercapacitors. Carbon 132, 776–784. doi: 10.1016/j.carbon.2017.12.051
Xie, Y. B., and Wang, D. (2016). Supercapacitance performance of polypyrrole/titanium nitride/polyaniline coaxial nanotube hybrid. J. Alloys Compd. 665, 323–332. doi: 10.1016/j.jallcom.2016.01.089
Yang, L. F., Cheng, S., Wang, J. H., Ji, X., Jiang, Y., Yao, M. H., et al. (2016). Investigation into the origin of high stability of delta-MnO2 pseudo-capacitive electrode using operando Raman spectroscopy. Nano Energy 30, 293–302. doi: 10.1016/j.nanoen.2016.10.018
Yousaf, M., Shi, H. T. H., Wang, Y. S., Chen, Y. J., Ma, Z. M., Cao, A. Y., et al. (2016). Novel pliable electrodes for flexible electrochemical energy storage devices: recent progress and challenges. Adv. Energy Mater. 6:17. doi: 10.1002/aenm.201600490
Yuan, C., Yang, L., Hou, L., Shen, L., Zhang, X., and Lou, X. W. (2012). Growth of ultrathin mesoporous Co3O4 nanosheet arrays on Ni foam for high-performance electrochemical capacitors. Energy Environ. Sci. 5, 7883–7887. doi: 10.1039/c2ee21745g
Zhai, S. L., Wang, C. J., Karahan, H. E., Wang, Y. Q., Chen, X. C., Sui, X., et al. (2018). Nano-RuO2-decorated holey graphene composite fibers for micro-supercapacitors with ultrahigh energy density. Small 14:29. doi: 10.1002/smll.201800582
Zhang, J., Lin, J., Wu, J., Xu, R., Lai, M., Gong, C., et al. (2016a). Excellent electrochemical performance hierarchical Co3O4@Ni3S2 core/shell nanowire arrays for asymmetric supercapacitors. Electrochim. Acta 207, 87–96. doi: 10.1016/j.electacta.2016.04.068
Zhang, Q., Liu, Z., Zhao, B., Cheng, Y., Zhang, L., Wu, H., et al. (2018b). Design and understanding of dendritic mixed-metal hydroxide nanosheets@N-doped carbon nanotube array electrode for high-performance asymmetric supercapacitors. Energy Storage Mater. 16, 632–645. doi: 10.1016/j.ensm.2018.06.026
Zhang, Q. B., Chen, H. X., Luo, L. L., Zhao, B. T., Luo, H., Han, X., et al. (2018a). Harnessing the concurrent reaction dynamics in active Si and Ge to achieve high performance lithium-ion batteries. Energy Environ. Sci. 11, 669–681. doi: 10.1039/c8ee00239h
Zhang, Y. Z., Cheng, T., Wang, Y., Lai, W. Y., Pang, H., and Huang, W. (2016b). A simple approach to boost capacitance: flexible supercapacitors based on manganese oxides@MOFs via chemically induced in situ self-transformation. Adv. Mater. 28:5242. doi: 10.1002/adma.201600319
Zhao, B., Zhang, L., Zhang, Q., Chen, D., Cheng, Y., Deng, X., et al. (2018a). Rational design of nickel hydroxide-based nanocrystals on graphene for ultrafast energy storage. Advanced Energy Materials 8:9. doi: 10.1002/aenm.201702247
Zhao, J., Pang, H., Deng, J., Ma, Y., Yan, B., Li, X., et al. (2013). Mesoporous uniform ammonium nickel phosphate hydrate nanostructures as high performance electrode materials for supercapacitors. CrystEngComm 15, 5950–5955. doi: 10.1039/c3ce40712h
Zhao, M., Huang, Y., Peng, Y. W., Huang, Z., Ma, Q., and Zhang, H. (2018). Two-dimensional metal-organic framework nanosheets: synthesis and applications. Chem. Soc. Rev. 47, 6267–6295. doi: 10.1039/c8cs00268a
Zheng, S., Li, X., Yan, B., Hu, Q., Xu, Y., Xiao, X., et al. (2017). Transition-metal (Fe, Co, Ni) based metal-organic frameworks for electrochemical energy storage. Adv. Energ. Mater. 7:18. doi: 10.1002/aenm.201602733
Zheng, S., Xue, H., and Pang, H. (2018). Supercapacitors based on metal coordination materials. Coord. Chem. Rev. 373, 2–21. doi: 10.1016/j.ccr.2017.07.002
Zhu, S., Li, L., Liu, J., Wang, H. T., Wang, T., Zhang, Y., et al. (2018). Structural directed growth of ultrathin parallel birnessite on beta-MnO2 for high-performance asymmetric supercapacitors. ACS Nano 12, 1033–1042. doi: 10.1021/acsnano.7b03431
Keywords: NH4NiPO4·H2O, flexible supercapacitor, asymmetrical, all-solid-state, electrochemical performances
Citation: Liu Y, Zhai X, Yang K, Wang F, Wei H, Zhang W, Ren F and Pang H (2019) Mesoporous NH4NiPO4·H2O for High-Performance Flexible All-Solid-State Asymmetric Supercapacitors. Front. Chem. 7:118. doi: 10.3389/fchem.2019.00118
Received: 31 August 2018; Accepted: 15 February 2019;
Published: 07 March 2019.
Edited by:
Qiaobao Zhang, Xiamen University, ChinaReviewed by:
Zhen-Dong Huang, Nanjing University of Posts and Telecommunications, ChinaYuxin Zhang, Chongqing University, China
Hongkang Wang, Xi'an Jiaotong University, China
Copyright © 2019 Liu, Zhai, Yang, Wang, Wei, Zhang, Ren and Pang. This is an open-access article distributed under the terms of the Creative Commons Attribution License (CC BY). The use, distribution or reproduction in other forums is permitted, provided the original author(s) and the copyright owner(s) are credited and that the original publication in this journal is cited, in accordance with accepted academic practice. No use, distribution or reproduction is permitted which does not comply with these terms.
*Correspondence: Yong Liu, liuyong209@haust.edu.cn
Wanhong Zhang, zhangwh@haust.edu.cn
†These authors have contributed equally to this work